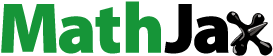
ABSTRACT
It was to investigate the neuroprotective mechanism of tanshinone after cerebral infarction via the Kelch-like ECH-associated protein 1 (Keap1)-nuclear factor erythroid 2-related factor 2 (Nrf2)/antioxidant reaction element (ARE) signaling pathway. Forty specific pathogen-free (SPF) Sprague Dawley (SD) rats were selected, all of which were male, approximately seven weeks old, weighing 250 ± 25 g. They were randomly divided into a model group, a non-model operation group, a positive control group, and an experimental group with ten SD rats in each group. The model of cerebral infarction in rats was established by the wire occlusion method. The model group and non-model operation group (control group) were injected with normal saline daily, the negative control group was injected with Keap1 gene inhibitor daily, and the experimental group was injected with tanshinone IIA (10 mg·kg−1·d−1) daily. Animal behavior analysis was performed on the 7th day after the operation, and pathology and the neuroprotective effects of tanshinone IIA on cells were assessed, including cell proliferation, autophagy, oxidative damage, and mitochondrial membrane permeability. The neuroprotective mechanism based on the Keap1-Nrf2/ARE pathway was explored and analyzed. Compared with the model group, the number of Keap1 proteins in the experimental group and the control group was substantially reduced (P < 0.05), and the experimental group was substantially different from the model group (P < 0.01). The protein expression of Nrf2, HO-1, and NQO1 increased substantially (P < 0.05), and the experimental group was substantially different from the model group (P < 0.01). In summary, tanshinone IIA promoted the proliferation of nerve cells, inhibited the production of cellular reactive oxygen species, inhibited the change in mitochondrial membrane potential, and activated the Keap1-Nrf2/ARE signaling pathway. It also induced and regulated the upregulation of downstream NQO1, HO-1, etc. and protected cells from cerebral infarction.
1. Introduction
Cerebral infarction, also known as cerebral ischemic stroke, is a condition that causes difficulty in supplying blood to areas of the brain. Ischemia leads to necrosis of brain cells and is the second leading cause of death in the world. Clinically, cerebral infarction accounts for 80% of all cerebral apoplexy cases, and cerebral thrombosis is the most common type of cerebral infarction, accounting for approximately 60% of all cerebral infarction cases [Citation1–4]. Cerebral infarction is an acute disease, and its morbidity and mortality are very high. In this case, intravenous thrombolysis therapy is best used within 3 4.5 hours after the onset of the disease, and there is a risk of intracranial hemorrhage, but there is no other effective treatment. Therefore, the combination of reperfusion and neuroprotection is the best treatment scheme at present [Citation5,Citation6]. According to studies, when ischemic stroke occurs, the body’s antioxidant emergency mechanism is activated, and the endogenous Kelch-like ECH-associated protein 1 (Keap1)-nuclear factor erythroid 2-related factor 2 (Nrf2)/antioxidant reaction element (ARE) signaling pathway is activated immediately to rapidly protect ischemic and hypoxic brain cells. This mechanism is one of the most important stress channels in the antioxidative stress mechanism of cerebral infarction [Citation7].
Keap1 is a polypeptide anchored to the cytoplasmic actin skeleton of the cell. It regulates the Nrf2 factor and binds to it in the cytoplasm. When the body produces oxidative stress, Keap1 will be unstable in combination with Nrf2, and Nrf2 will be released, then transferred to the nucleus and combined with ARE to activate the transcription of downstream genes. Then, a variety of proteases, such as antioxidant proteases, ubiquitinases, and phase II detoxification enzymes, are translated. As a result, the expression levels of antioxidants such as oxidoreductase 1 (NQO1), glutathione peroxidase (GSH-Px), and heme oxygenase-1 (HO-1) are upregulated. These antioxidants will be important substances to protect cells, and they will scavenge a batch of toxic substances, such as oxygen free radicals [Citation7,Citation8]. Therefore, finding a substance that can activate the Keap1-Nrf2/ARE signaling pathway is an ideal drug for “cell protection”.
Traditional Chinese medicine believes that cerebral infarction is often caused by organ dysfunction, negative emotions, other congestion blocks, and finally cerebral pulse paralysis. TCM treatment mainly focuses on warming Yang and tonifying qi, dredging collateral and activating arteries, promoting blood circulation and removing blood stasis, dredging collateral and clearing heat and detoxifying [Citation9,Citation10]. In Chinese medicine treatment, the most common treatment is the Chinese medicine Salvia miltiorrhiza. Salvia miltiorrhiza is the dry rhizome of Salvia miltiorrhiza, which has the functions of promoting blood circulation and removing blood stasis, transcending menstruation and relieving pain, cooling blood, and eliminating carbuncles [Citation11]. Salvia miltiorrhiza contains many chemical components, such as diterpenoid quinones, phenolic acids, and glycosides. Tanshinone IIA is the most commonly used component in cerebral infarction. Studies have suggested that tanshinone IIA is effective in treating cerebral infarction in many aspects, including anti-inflammatory, anti-itching free radical, anti-apoptosis, and improving blood flow. In the early stage of cerebral infarction, such as calcium overload, it also has a considerable effect. Moreover, studies have proven that tanshinone IIA is a strong Nrf2 activator that can enhance the antioxidant activity of Nrf2. Therefore, tanshinone IIA has a protective effect on cells in cerebral infarction. However, there is a lack of specific in vivo research data to support the specific mechanism [Citation12–15]. This study used a cerebral infarction rat model to evaluate whether tanshinone IIA has a protective effect on the cerebral infarction nerve and its effect on the Keap1-Nrf2/ARE signaling pathway.
2. Materials and methods
2.1 Experimental animals
The experimental models were male SD rats, SPF grade, 7–8 years old, weighing 250 ± 25 g, purchased from Beijing University Animal Experiment Center. Before the experiment, all rats were subjected to environmental adaptation, feeding, and water on time, and sawdust was changed. The specific research process was shown in .
2.2 Main drugs and reagents
The reagents and instruments used in this experiment included Tanshinone IIA (Sigma, USA), silicone-coated suture (Jiangsu Xinmai), phosphate-buffered saline (PBS) buffer (extracted from Chinese double helix), 2,3,5-triphenyltetrazolium chloride (TTC) (China Lab), Tissue Mitochondrial Isolation Kit (Bilaibao, China), JC-1 Mitochondrial Membrane Potential Detection Kit (Suzhou Yuheng), whole protein extraction kit, primary and secondary antibody removal solution, sodium dodecyl sulfate – polyacrylamide gel electrophoresis (SDS – PAGE) gel rapid preparation kit, SDS – PAGE electrophoresis solution, SDS – PAGE protein sample buffer, western-style washing solution, ECL luminescent solution (China Wanlei Bio), poly(vinylidene fluoride) (PVDF) membrane (Millipore, USA), skim milk powder (China Mengniu), absolute ethanol (Sinopharm), DYY-6D electrophoresis apparatus, gel imaging system (Beijing Liuyi), Tanon 5200 automatic chemiluminescence image analysis system (Beijing Yuanpinghao), and benchtop cryogenic high-speed centrifuge (Eppendorf, Germany).
2.3 Grouping and administration
Forty SD rats were randomly divided into a model group, a non-model operation group, a positive control group, and an experimental group, with ten rats in each group. The model group and non-model operation group were injected with normal saline daily (10 mg·kg−1·d−1).The negative control group was injected with Keap1 gene inhibitor daily, and the experimental group was injected with tanshinone IIA (10 mg·kg−1·d−1) daily. All groups were given intraperitoneal injection once a day for seven consecutive days.
2.4 Establishment of a rat model of cerebral infarction
In this study, a rat cerebral infarction model was established by the wire occlusion method [Citation16,Citation17]. First, ten rats in the experimental group were anesthetized with 10%chloral hydrate (the reason for using chloral hydrate for mouse anesthesia was as follows. First, the drug acted rapidly and improved the experimental efficiency; second, it was a nonclinical experiment. However, large doses of anesthesia can easily lead to death, and it was rarely used. Follow-up studies would consider other anesthetics) injected intraperitoneally according to the standard of 3.5 mL/kg. The anesthetized rats were fixed in the supine position on the operating table. The incision was made to the right of the center of the neck of the rat to fully display the blood vessels. The cervical vessels, right common carotid artery, external carotid artery, and internal carotid artery were successively separated. The right common carotid artery near the heart was ligated with the external carotid artery with surgical sutures, and then the common carotid artery was opened near the heart. A suppository line was inserted at the opening approximately 22.0 ± 2.0 mm and entered the skull from the bifurcation of the common carotid artery. When there was resistance under the finger, the thrombus blocked the cerebral artery blood flow. After the thrombus was fixed, the wound of the rats was sutured. The non-model surgery group underwent the same operation without blocking the cerebral artery blood flow.
2.5 Animal behavior analysis
After the operation, animal behavior analysis was performed in each group, and neurological impairment was judged by scores.
The integration and coordination of anterior and posterior limb movements were evaluated.
Rat forelimbs were evaluated by the foot fault test [Citation18]. A 100 cm × 60 cm × 25 cm grid platform was utilized, with a single grid of 5 cm × 3 cm × 25 cm. Most normal rats crawled with their forelimbs attached to the mesh railings. If the rat was abnormal, the forelimbs could not climb to the railing normally and slipped into the grid, and it was recorded as one foot fault. During the experiment, three experiments were performed on the rats, with each time being 2 min and the interval 5 min. The number and total steps of the two forelimbs of the rats were recorded. The full error rate Z was calculated as follows.
whereX1 is the number of abnormal forelimb error steps, X2 is the normal forelimb error step count, and B is the total number of steps on both forelimbs.
The hind limbs of the rats were evaluated using Fenny’s score criteria in the balance beam experiment. A crossbar 125 cm long, 2.2 cm wide, and 40 cm above the ground was used. A black box 31 cm × 24 cm × 17 cm was placed in front of the crossbar, and the rats were guided through the crossbar into the black box. Each rat was tested three times and scored according to the specific performance of the rat. The specific scoring table was shown in .
Table 1. Fenny score sheet for the balance beam test.
2.6 Rat brain tissue extraction
After the experiment, rats in each group were sacrificed by intraperitoneal injection of pentobarbital sodium 50 mg/kg. The skull of the rat was dissected to obtain the complete brain tissue of the rat, and the hippocampus and cerebral cortex tissues of the rat were collected, avoiding damage during removal.
2.7 Calculation of cerebral infarction volume
TTC staining was used to measure the infarct volume. The extracted rat brain tissues were cleaned with normal saline and then frozen in a refrigerator at −20degree centigrade for 30 min. The frozen rat brain tissue was cut coronally into 2 mm slices under low temperature. The staining was performed in 2% TTC for 30 min at 37degree centigrade in a dark environment, with continuous turnover to ensure complete dyeing. The normal area was bright red, while the infarcted tissue was pale. Finally, the evenly stained sections were photographed and input into the computer, and the volume of cerebral infarction was calculated using Image J analysis software. The specific calculation was as follows.
V% is the percentage of cerebral infarction, V1 is the volume of cerebral infarction, and V2 is the volume of brain tissue slice.
2.8 Brain water content measurement
Untreated samples of rat brain tissue were dried with filter paper and weighed. After weighing, the brain tissues were placed in a petri dish, quickly placed in a 100 degree centigrade incubator, and dried for 72hto obtain the dry weight of the tissues. The equation for measuring brain water content was expressed as follows.
Z is the water content of the brain, G1 is the wet weight of the brain tissue, and G2 is the dry weight of the brain tissue.
2.9 Mitochondrial membrane potential measurement
Then,100 to 200 mg of rat brain tissue was weighed and rinsed with phosphate buffer, and the tissue surface was drained with filter paper. After crushing, the samples were placed into a homogenizer, 1 mL of cell lysis buffer was added, and the samples were ground in an ice bath for 5–10 min. The lapping tissue was placed in a centrifuge tube at 4degree centigrade at 1,000 r/min and centrifuged for 5 min. The supernatant was taken and centrifuged again with the same parameters in a new tube. The same operation was performed again, with a total of three rounds of centrifugation. At that time, the supernatant in the centrifuge tube contained cytoplasmic components, which were used for the extraction of cytoplasmic proteins, and the lower precipitate was mitochondria.
Then, 0.5 mL rinse buffer was added to precipitate the suspended mitochondria, and centrifugation was repeated. Then, the supernatant of the centrifugal liquid was taken and centrifuged for 10 min at 4degrees centigrade at 12,000 r/min with a new centrifugal tube. At that point, high-purity mitochondria precipitated at the bottom. The mitochondrial precipitate was resuspended in 50 100 μL of store buffer.
The fluorescence intensity of the mitochondrial membrane was measured by JC-1 (0.9 mL).
2.10 Western blot method to measure Keap1, Nrf2, ARE, and NQO1 protein expression levels
50 100 mg of cortical tissue on the pathological side of the rats was taken, and 1 mL of cell lysis buffer was added after the solid tissue was broken and then ground in an ice bath for 5–10 min. Then, 0.5 mL of the grinding homogenate was added to a centrifuge tube, and the remaining homogenate was discarded. A total of 0.5 mL of tissue homogenate was added to 1 mL of the extraction reagent, fully mixed, and then incubated at 4degrees centigrade for 10 minutes. Centrifugation was performed at 10,000 × g for 10 min at 4degrees centigrade. Between the two phases of the solution was a protein membrane. The upper and lower phases were aspirated with a needle, and the intermediate protein membrane was kept intact and dried at room temperature for 10 min. An appropriate amount of 2% SDS was added to dry the protein, which was heated to 95% for 10 min to dissolve and precipitate at room temperature to obtain total protein. The protein concentration was measured and balanced by bicinchoninic acid (BCA) assay.
A 30 μL sample was loaded. Ten percent separated glue and 5% concentrated glue were mixed and subjected to gel electrophoresis. Then, the proteins were transferred to PVDF by the electrotransfer method. After the PVDF membrane was removed, 5% skimmed milk powder sealing solution was used to seal the membrane at room temperature for 1 hour. After blocking, a specific primary antibody was added and incubated overnight at 4degrees centigrade. Then, they were rinsed with phosphate buffer five times for 5 min each time. Secondary antibody was added and incubated at room temperature for 1 hour. ECL luminescent liquid was added to the gel imaging system, and the gray value was analyzed by Image software.
2.11 Statistical methods
Data processing in this study was analyzed by SPSS 19.0. Animal behavior score, cerebral infarct volume, water content of brain tissue, Western blot results, and immunohistochemical results were compared between groups. Univariate analysis of variance was used to determine the difference between groups. All experimental data were tested for homogeneity of variance, and a nonparametric test was used if the variance was not homogeneous. P < 0. 05 indicated a significant difference.
3. Results
3.1 Animal behavior research
The number of foot errors in animal behavior in the model group was substantially higher than that in the non-model surgery group (P < 0.01). Except for these two groups, the foot error rates of the other two groups decreased with time. Especially in the last two inspections, the changes were particularly obvious. This proved that tanshinone IIA can indeed restore and promote the exercise and coordination ability of rats. The specific foot error rate changes were shown in .
The animal behavior balance beam score of the model group was substantially lower than that of the non-model surgery group (P < 0.01). Except for these two groups, the scores of the rats in the other two groups gradually increased over time and returned to the level of the non-model surgery group on the 7th day, which proved that the rats’ self-balancing ability can gradually recover. The scores of the experimental group and the control group were higher than those of the model group over time, which proved that tanshinone IIA did restore the balance ability of rats. The specific scores were shown in .
3.2 Calculation of cerebral infarction volume
The specific cerebral infarction volume calculation data were shown in . No cerebral infarction occurred in the rats in the non-model operation group, and the staining of the brain tissue was red. The staining display of the remaining groups was shown in . The brain tissues of the model group, the experimental group, and the control group had obvious pale areas, and the cerebral infarction volume was obviously larger (P < 0.01). The pale area in the model group was substantially larger than that in the experimental group and the control group; that is, the cerebral infarction volume of the experimental group and the control group was substantially reduced (P < 0.01). The cerebral infarction volume of rats in the experimental group was not substantially different from that in the control group (P > 0.05). The results showed that tanshinone IIA reduced the volume of cerebral infarction in brain tissue and protected the brain tissue.
Figure 4. Proportion of cerebral infarction volume.
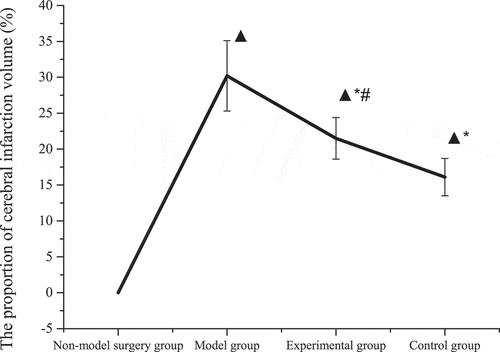
(A: Non-model surgery group, B: rat brain tissue in the model group; C: rat brain tissue in the control group; D: rat brain tissue in the experimental group. (The brain tissue of the mice in the non-model operation group was normal, and there was no cerebral infarction, so it was not shown in the figure.)
3.3 Brain water content measurement results
The specific results of the brain tissue water content of rats in each group were shown in . Compared with the non-model surgery group, the brain tissue water content of rats in the model group, experimental group, and control group increased substantially (P < 0.05), especially in the model group (P < 0.01). The water content of the control group and the experimental group was substantially lower than that of the model group (P < 0.05). However, there was no considerable difference in the brain tissue water content of the control group and the experimental group (P > 0.05).
3.4 Mitochondrial membrane potential test results
The comparison results of the fluorescence intensity of the brain tissue membrane potential of each group of rats were shown in . The membrane potential fluorescence intensity of rats in the model group, the control group, and the experimental group was substantially lower than that of the non-model surgery group (P < 0.05), among which the model group and the experimental group were much lower (P < 0.01). The membrane potential fluorescence intensity of rats in the experimental group and the control group was higher than that of the model group (P < 0.05), of which the control group was much higher (P < 0.01). There was no considerable difference between the control group and the experimental group in the fluorescence intensity of the rats (P > 0.05).
3.5 Western blot method to detect Keap1, Nrf2, HO-1, and NQO1 protein expression levels
Compared with the model group, the non-model surgery group, the expression of Keap1 protein in the experimental group and the control group decreased substantially, the expression of Nrf2, HO-1, and NQO1 protein increased substantially (P < 0.01), and the expression of HO-1 protein in the control group increased (P < 0.05). Compared with the control group, the non-model surgery group and the experimental group had significant differences in the expression of Keap1, Nrf2, HO-1, and NQO1 (P < 0.05) ().
n the model group, the control group, and the experimental group was substantially lower than that of the non-model surgery group (P < 0.05)
(1 4 represented the non-model surgery group, model group, experimental group, and control group, respectively. A: Keap1 protein expression test result; B: Nrf2 protein expression test result; C: HO-1 protein expression test result; D: NQO1 protein expression test result)
Note: compared with the model group, ▲P < 0.05, *P < 0.01; compared with the control group #P < 0.05.
4. Discussion
The high disability rate, high mortality rate, high morbidity rate, and high recurrence rate of cerebral infarction have brought incurable pain and heavy economic burden to thousands of families [Citation19–21]. Therefore, effectively alleviating the sequelae of cerebral infarction has become the focus of clinical research. Modern pharmacological studies have found that tanshinone has a curative effect on the clinical treatment of cerebral infarction. To obtain a more detailed understanding of Salvia miltiorrhiza, this work investigated the mechanism of Salvia miltiorrhiza on the neuroprotective Keap1-Nrf2/ARE signaling pathway of cerebral infarction from a cellular perspective, providing a detailed reference and basis for clinical medication.
In this work, the rat cerebral infarction model was established by the suture method, and the rat cerebral infarction model was verified by the balance beam Fenny score and the wrong foot rate. The results showed that the foot error rate of the model group was significantly higher than that of the other groups, while the Finney score of the balance beam was significantly lower than that of the other groups, with statistical significance (P < 0.01), indicating that the rat cerebral infarction model was successfully established and that follow-up studies could be conducted. Fei et al. (2017) [Citation22] believed that Salvia miltiorrhiza had obvious effects in inhibiting thrombosis and preventing cerebral ischemia injury. Wang et al. (2017) [Citation23] concluded through a clinical trial involving 1044 patients who Danhong injection combined with conventional therapy has a very good effect on neurological protection and clinical cure rate. The results of this work also showed that, compared with the model group, the foot error rate of the control group and the experimental group decreased at 1, 3, 5, and 7 days after surgery, while the Fenny score increased, which was statistically significant (P < 0.01). However, there was no significant difference in the evaluation between the control group and the experimental group (P > 0.05), indicating that tanshinone IIA may improve the nervous system of rats with cerebral infarction by repairing brain tissue. Then, the role of tanshinone IIA in cytology was explored.
The pale area of the brain tissue of the mice in the model group was significantly larger than that in the experimental group and the control group (P < 0.01), but there was no significant difference between the experimental group and the control group (P > 0.05). In the work, the method for detecting cerebral infarction in rat brain tissue was TTC staining, which can stain the cells red with the reduction reaction of enzymes in the mitochondria of the cells, but the cells in the cerebral infarction area are ischemia and hypoxia, and the mitochondrial activity is damaged [Citation24], which can’t be dyed. Moreover, some studies have shown that the mitochondrial damage-mediated apoptosis pathway is the main pathway of neuronal apoptosis after cerebral ischemia [Citation25]. Based on the above results, Tanshinone IIA can reduce the infarct volume and protect the brain. When mitochondria are damaged, the level of adenosine triphosphate (ATP) decreases, which in turn reduces Ca2+ in brain tissue cells, resulting in a decrease in the stability of mitochondrial membrane potential [Citation26]. By measuring the membrane potential fluorescence intensity, it was found that compared with the model group, the membrane potential fluorescence intensity of the experimental group and the control group increased (P < 0.05), and there was no significant difference in the fluorescence intensity between the control group and the experimental group (P > 0.05). Experiments have shown that mitochondrial membrane potential is significantly reduced during cerebral infarction, while tanshinone IIA can alleviate the symptoms of cerebral infarction in rats and protect the brain by increasing mitochondrial membrane potential.
In addition, clinical studies suggest that patients with cerebral infarction are prone to cerebral edema in the early stage, and cerebral edema is also one of the most serious causes of cerebral infarction and high mortality [Citation27]. The main reason for the formation of brain edema after cerebral infarction is the destruction of the blood – brain barrier. To maintain the integrity of the blood – brain barrier, it is necessary to increase the expression of claudin-5, which is the main component of tight junction proteins in the blood – brain barrier [Citation28]. Some studies have suggested that tanshinone IIA can inhibit the inflammatory response and apoptosis after cerebral infarction, thereby reducing the volume of cerebral infarction, improving the occurrence of cerebral edema, and exerting neuroprotective effects [Citation29,Citation30]. The results of this work revealed that compared with the non-model surgery group, the brain tissue water content in the model group, experimental group and control group was significantly increased (P < 0.05), especially in the model group (P < 0.01). The water content of the control group and the experimental group was significantly lower than that of the model group (P < 0.05). There was no significant difference in brain tissue water content between the control group and the experimental group (P > 0.05). It has also been proven that tanshinone IIA can effectively reduce the water content of brain tissue, thereby reducing the symptoms of cerebral edema, which is in line with the above research results.
As the main endogenous antioxidant regulator in the human body that maintains the balance of oxidation and antioxidants in the body, Nrf2 inhibits inflammation and apoptosis [Citation31]. Under normal conditions, Nrf2-Keap1 coupling mainly exists stably in the cytoplasm and mediates the body’s antioxidant process. Once stimulated, Nrf2 will bind to the specific recognition site of ARE in the nucleus, the expressions of NQO1 and HO-1 will increase, and the resistance to oxidative stress will also be enhanced [Citation32,Citation33]. The results of this work found that compared with the model group, the amount of Keap1 protein in the experimental group and the control group was significantly reduced (P < 0.05), and the difference was significant (P < 0.01). The protein expression levels of Nrf2, HO-1 and NQO1 were significantly increased (P < 0.05), and the difference between the experimental groups was significant (P < 0.01). This result indicated that tanshinone IIA was indeed involved in the Keap1-Nrf2/ARE signaling pathway and could inhibit the expression of Keap1 protein and activate Nrf2 to induce the expression of downstream HO-1 and NQO1. The above results suggest that tanshinone IIA can stimulate the Keap1-Nrf2/ARE signaling pathway, improve the antioxidant activity of brain tissue, and reduce the occurrence of cerebral infarction.
5. Conclusion
Tanshinone IIA can repair and protect the brain tissue under cerebral infarction, improve the symptoms of cerebral edema, and reduce the damage to brain tissue cells caused by cerebral ischemia and hypoxia. This process may be related to the Keap1-Nrf2/ARE signaling pathway. Further study of theKeap1-Nrf2/ARE signaling pathway will be helpful to improve the understanding of the onset warning and treatment of cerebral infarction to reduce the incidence of cerebral infarction and improve postoperative recovery. However, in this experiment, few animal samples were selected, and a few proteins related to theKeap1-Nrf2/ARE signaling pathway were detected. In addition, this study only focused on the protective effect of tanshinone IIA. Other components, such as tanshinone I and cryptoTanshinone, have not been studied, which may have certain limitations in the discussion of the results. In this study, it was verified that tanshinone participates in the signaling pathway to regulate oxidative stress and protect the nervous system in a cerebral infarction model, providing strong data support for the treatment of cerebral infarction by Salvia miltiorrhiza.
Ethics approval and consent to participate
All the animal experiments were performed in line with the National Medical Advisory Committee (NMAC) guidelines using approved procedures of the Institutional Animal Care and Use committee at Beijing University of Chinese Medicine.
Availability of data and materials
The dataset used and/or analyzed during the current study are available from the corresponding author on reasonable request.
Disclosure statement
No potential conflict of interest was reported by the author(s).
Additional information
Funding
References
- Regenhardt RW, Das AS, Lo EH, et al. Advances in understanding the pathophysiology of lacunar stroke: a review. JAMA Neurol. 2018 Oct 1;75(10):1273–1281. DOI:10.1001/jamaneurol.2018.1073
- Barantsevich YR, Emanuel YV. Vosstano vlenieposleishemicheskogoinsul’ta v molodomvozraste [Recovery from ischemic stroke at a young age]. ZhNevrolpsikhiatrim S Skorsakova. 2020 Russian;120(2):49–55.
- Khaku AS, Tadi P. Cerebrovascular Disease. 2021 Jan 31. In: StatPearls [Internet]. Treasure Island (FL): StatPearls Publishing; 2021Jan. PMID: 28613677.
- Diener HC, Hankey GJ. Primary and secondary prevention of ischemic stroke and cerebral hemorrhage: jACC focus seminar. J Am Coll Cardiol. 2020 Apr 21;75(15):1804–1818. DOI:10.1016/j.jacc.2019.12.072
- Paul S, Candelario-Jalil E. Emerging neuroprotective strategies for the treatment of ischemic stroke: an overview of clinical and preclinical studies. Exp Neurol. 2021 Jan;335:113518.
- Hurford R, Sekhar A, Hughes TAT, et al. Diagnosis and management of acute ischaemic stroke. Pract Neurol. 2020 Aug;20(4):304–316.
- Dovinova I, Kvandová M, Balis P, et al. The role of Nrf2 and PPARgamma in the improvement of oxidative stress in hypertension and cardiovascular diseases. Physiol Res. 2020 Dec 31;69(Suppl 4):S541–S553. DOI:10.33549/physiolres.934612
- Kopacz A, Kloska D, Forman HJ, et al. Beyond repression of Nrf2: an update on Keap1. Free Radic Biol Med. 2020 Sep;157:63–74.
- Liu J, Xu F, Mohammadtursun N, et al. The analysis of constitutions of traditional ChineSe medicine in relation to cerebral infarction in a Chinese sample. J Altern Complement Med. 2018 May;24(5):458–462.
- He J, Kwon Y, Li C, et al. Several considerations in using traditional Chinese patent medicine for cerebral infarction. Chin J Integr Med. 2012 Aug;18(8):571–574.
- Meim XD, Cao YF, Che YY, et al. Danshen: a phytochemical and pharmacological overview. Chin J Nat Med. 2019 Jan;17(1):59–80.
- Zhou L, Zhang J, Wang C, et al. Tanshinone inhibits neuronal cell apoptosis and inflammatory response in cerebral infarction rat model. Int J Immunopathol Pharmacol. 2017 Jun;30(2):123–129.
- Liu S, Wang K, Duan X, et al. EfficaCy of danshen class injection in the treatment of acute cerebral infarction: a bayesian network meta-analysis of randomized controlled trials. Evid Based Complement Alternat Med. 2019 Feb 3;2019:5814749.
- Tang Q, Han R, Xiao H, et al. Protective effect of tanshinone IIA on the brain and its therapeutic time window in rat models of cerebral ischemia-reperfusion. Exp Ther Med. 2014 Nov;8(5):1616–1622.
- Yang G, Wang F, Wang Y, et al. Protective effect of tanshinone IIA on H2O2-induced oxidative stress injury in rat cardiomyocytes by activating Nrf2 pathway. J Recept Signal Transduct Res. 2020 Jun;40(3):264–272.
- Zhang P, Huang Z, Yan HQ, et al. Improvement of the suture-occluded method in rat models of focal cerebral ischemia-reperfusion. Exp Ther Med. 2014 Mar;7(3):657–662.
- Liu QS, Zhang WW, Yin XY, et al. [Neuro-Protective effects and mechanism of Terminalia chebula extract HZ4 on cerebral infarction models]. Zhongguo Zhong Yao Za Zhi. 2016 May;41(10):1910–1915. Chinese10.4268/cjcmm20161025
- Barth TM, Stanfield BB. The recovery of forelimb-placing behavior in rats with neonatal unilateral cortical damage involves the remaining hemisphere. J Neurosci. 1990 Oct;10(10):3449–3459.
- van Veluw SJ, Shih AY, Smith EE, et al. Detection, risk factors, and functional consequences of cerebral microinfarcts. Lancet Neurol. 2017 Sep;16(9):730–740. DOI:10.1016/S1474-4422(17)30196-5.
- Sveinsson OA, Kjartansson O, Em V. Heilablóðþurrð/heiladrep: faraldsfræði, orsakirogeinkenni [Cerebral ischemia/infarction - epidemiology, causes and symptoms]. Laeknabladid. 2014 May;100(5):271–279. Icelandic10.17992/lbl.2014.05.543
- Zhang Y, Hu Y, Li M, et al. ThE traditional Chinese medicine compound, GRS, alleviates blood-brain barrier dysfunction. Drug Des Devel Ther. 2020 Feb 28;14:933–947.
- Fei YX, Wang SQ, Yang LJ, et al. Salvia miltiorrhiza Bunge (Danshen) extract attenuates permanent cerebral ischemia through inhibiting platelet activation in rats. J Ethnopharmacol. 2017 Jul 31;207:57–66. DOI:10.1016/j.jep.2017.06.023.
- Wang K, Zhang D, Wu J, et al. A comparative study of Danhong injection and Salvia miltiorrhiza injection in the treatment of cerebral infarction: a systematic review and meta-analysis. Medicine (Baltimore). 2017 Jun;96(22):e7079.
- Gong Z, Pan J, Shen Q, et al. Mitochondrial dysfunction induces NLRP3 inflammasome activation during cerebral ischemia/reperfusion injury. J Neuroinflammation. 2018 Aug 28;15(1):242. DOI:10.1186/s12974-018-1282-6
- Pan G, Zhang H, Zhu A, et al. Treadmill exercise attenuates cerebral ischaemic injury in rats by protecting mitochondrial function via enhancement of caveolin-1. Life Sci. 2021 Jan 1;264:118634. DOI:10.1016/j.lfs.2020.118634.
- Wang H, Chen S, Zhang Y, et al. Electroacupuncture ameliorates neuronal injury by Pink1/Parkin-mediated mitophagy clearance in cerebral ischemia-reperfusion. Nitric Oxide. 2019 Oct 1;91:23–34. DOI:10.1016/j.niox.2019.07.004.
- Huang T, Chen B, Zeng ZM. VEGF-Transfected hBmsCs aggravate early brain edema in cerebral hemorrhage rats. Sichuan Da XueXue Bao Yi Xue Ban. 2020 Sep;51(5):622–629.
- Gholamzadeh R, Ramezani F, Tehrani PM, et al. Apelin-13 attenuates injury following ischemic stroke by targeting matrix metalloproteinases (MMP), endothelin- B receptor, occludin/claudin-5 and oxidative stress. J Chem Neuroanat. 2021 Dec;118:102015.
- Huang Y, Long X, Tang J, et al. The attenuation of traumatic brain injury via inhibition of oxidative stress and apoptosis by tanshinone IIA. Oxid Med Cell Longev. 2020 May 1;2020:4170156.
- Wang L, Xiong X, Zhang X, et al. Sodium tanshinone IIA sulfonate protects against cerebral ischemia-reperfusion injury by inhibiting autophagy and inflammation. Neuroscience. 2020 Aug 10;441:46–57. DOI:10.1016/j.neuroscience.2020.05.054.
- Bellezza I, Giambanco I, Minelli A, et al. Nrf2-Keap1 signaling in oxidative and reductive stress. Biochim Biophys Acta Mol Cell Res. 2018 May;1865(5):721–733.
- Baird L, Yamamoto M. ThE molecular mechanisms regulating the KEAP1-NRF2 pathway. Mol Cell Biol. 2020 Jun 15;40(13):20–e00099. DOI:10.1128/MCB.00099-20
- Tu W, Wang H, Li S, et al. The anti-inflammatory and anti-oxidant mechanisms of the Keap1/Nrf2/ARE signaling pathway in chronic diseases. Aging Dis. 2019 Jun 1;10(3):637–651. DOI:10.14336/AD.2018.0513