ABSTRACT
Background
Long non-coding RNAs (lncRNAs) have been proved to play a vital role in pancreatic cancer (PC). However, the role of lncRNA FAM83A-AS1 in PC remains unclear. In this study, we explored the biological function and underlying mechanism of FAM83A-AS1 in PC cells.
Methods
The FAM83A-AS1 expression was assessed via public databases and validated by qRT-PCR. The biofunction and immune cell infiltration of FAM83A-AS1 were analyzed through GO, KEGG, GESA and ssGSEA. The migration, invasion and proliferation abilities of PC cells were examined by Transwell, wound healing, CCK8 and colony formation. The EMT and Hippo pathway markers were evaluated by western blot.
Results
FAM83A-AS1 expression was higher in PC tissues and cells than normal. Additionally, FAM83A-AS1 was associated with poor prognosis of PC and involved in cadherin binding and immune infiltration. Subsequently, we proved FAM83A-AS1 overexpression enhanced the migration, invasion and proliferation abilities of PC cells, whereas FAM83A-AS1 downregulation inhibited those. Moreover, western blot results showed that FAM83A-AS1 knockdown increased the E-cadherin expression and decreased the expression of N-cadherin, β-catenin, Vimentin, Snail and Slug. On the contrary, FAM83A-AS1 upregulation results in the opposite effects. Besides, FAM83A-AS1 overexpression inhibited the expression of p-YAP, p-MOB1, p-Lats1, SAV1, MST1 and MST2 as well as the results of FAM83A-AS1 knockdown were opposite.
Conclusion
FAM83A-AS1 promoted EMT of PC cells via Hippo signaling inactivation and may be a potential diagnosis and prognosis target.
1. Introduction
Pancreatic cancer (PC) is a lethal and aggressive malignancy with a poor 5-y relative survival due to metastasis [Citation1]. Most patients are diagnosed at an advanced stage and lack successful therapeutic strategies leading to high mortality [Citation2]. Currently, molecular biology research is developing rapidly but clinical outcome is still unsatisfactory [Citation2]. Therefore, it is necessary to find out the underlying molecular mechanism in PC progression.
Long non-coding RNAs (lncRNAs) have no protein-coding ability, and the length is longer than 200 nucleotides (nt) [Citation3]. Various studies have proved that lncRNAs play an important role in biological processes, such as migration and invasion, cell growth, protein metabolism and transcriptional control [Citation3]. Recently, increasing attention has been paid to the lncRNAs participation in various cancer progression. For example, Chen identified that HOXA10-AS regulated oral cancer growth as a modular scaffold for TP63 mRNA processing [Citation4]. In PC, LncRNA PVT1 promoted gemcitabine resistance through modulating the miR-619-5p/Pygo2 and miR-619-5p/ATG14 axes [Citation5]. LncRNA FAM83A-AS1 is FAM83A gene antisense strand in chromosome 8q24, and the length is 1,572 nucleotides [Citation6,Citation7]. FAM83A-AS1 has been proved to play a critical role in the progression and development of lung adenocarcinoma [Citation8], esophageal carcinoma [Citation9] and hepatocellular [Citation10]. For instance, FAM83A-AS1 facilitated lung adenocarcinoma proliferation and stemness via the HIF-1α/glycolysis axis [Citation7]. In PC, only few studies indicated FAM83A served as on oncogene [Citation11]. However, the role of FAM83A-AS1 in PC is still unknown.
In this study, we are the first to explore the role of FAM83A-AS1 in PC. Firstly, we found the FAM83A-AS1 expression was aberrant in PC tissues and cells. Subsequently, we proved FAM83A-AS1 enhanced the migration, invasion and proliferation abilities of PC cells. Also, FAM83A-AS1 promoted EMT in PC cells. Mechanically, the study provided evidence that FAM83A-AS1 may inhibit Hippo pathway to active YAP to promote EMT in PC cells. Thus, FAM83A-AS1 may serve as a candidate target for PC diagnosis and prognosis.
2. Materials and methods
2.1 Data source
The RNA sequencing and clinical data (survival time, survival status, tumor grade, and pathological stage) of patients with pancreatic cancer and other tumors were collected from the Cancer Genome Atlas (TCGA) database and the Genotype Tissue Expression Project (GTEx) database.
2.2 Enrichment analysis
The R package DESeq2 was used to perform the differentially expressed genes (DEGs) analysis. Adjusted P < 0.05 and |log2-fold-change (FC)|>1 were set as the thresholds of DEGs, which were visualized by volcano plot. Then, Spearman correlation analysis was used to evaluate the correlation between the top 10 DEGs and FAM83A-AS1 expression. Furthermore, functional enrichment analysis of DEGs was performed using the R package cluster profiler, including Gene Ontology (GO) and Kyoto Encyclopedia of Genes and Genomes (KEGG) analysis to investigate the biological characteristics of FAM83A-AS1 in pancreatic cancer. Gene Set Enrichment Analysis (GSEA) was to analyze the enrichment of the dataset between the high and low expressing group, with a false discovery rate (FDR) <25% and a nominal P value < 5% set as cutoff criteria.
2.3 Immune infiltration analysis
The cohorts were sorted into high-risk and low-risk segments based on the median FAM83A-AS1 expression to explore the correlation between prognostic gene expression immune infiltrations of pancreatic cancer using Spearman correlation analysis via the “gsva” R package [Citation12].
2.4 Cell culture
The human immortalized pancreatic ductal epithelial cell line H6C7 and pancreatic cancer cell lines BxPC-3, PANC-1 and PaTu8988 were obtained from the American Type Culture Collection (ATCC, Manassas, VA, USA). All cells were tested and authenticated by short tandem repeat analysis. Cells were cultured in DMEM medium (KeyGEN, Nanjing, China) that contains 10% fetal bovine serum, 1% penicillin and streptomycin (Hyclone, South Logan, UT, USA) and in a humidified incubator at 37°C and 5% CO2 conditions.
2.5 Cell transfection
The si-FAM83A-AS1 and si-control were synthesized by GenePharma (Suzhou, China. si-FAM83A-AS1-sense: 5’-GGCCCUGGGCUGAAUAAUUTT-3’ and si-FAM83A-AS1-antisense: 5’-AAUUAUUCAGCCCAGGGCCTT-3’; si-control-sense: 5’-UGACCUCAACUACAUGGUUTT-3’; si-control-antisense: 5’-AACCAUGUAGUUGAGGUCATT-3’). The plasmids pcDNA3.1-FAM83A-AS1 and pcDNA3.1 were bought from Future Biotherapeutics (Suzhou, China). The sequence was confirmed through DNA sequencing. Lipofectamine 2000 reagent (Invitrogen, Carlsbad, CA, USA) was used for cell transfection based on the protocols. Each well of 6-plate need 2 μg siRNA or plasmids and 10 μL Lipofectamine 2000.
2.6 Quantitative real-time polymerase chain reaction (qRT-PCR)
RNAiso Plus (Invitrogen) was to extract total RNA according to the manufacturer’s protocol. Complementary DNAs (cDNAs) were synthesized from RNA samples (1 μg) by RevertAid First Strand cDNA Synthesis Kit (Thermo Scientific). qRT-PCR was performed via SYBR Green Mix kit (Bio Rad Laboratories, Hercules, CA). Analysis of the relative expression was based on 2−ΔΔCt method. The primers are as follows: U6‐forward: 5“‐CTCGCTTCGGCAGCACA‐3” and U6‐reverse: 5“‐AACGCTTCACGAATTTGCGT‐3”; and FAM83A-AS1‐forward: 5“‐AACATACCCAGTCCAGTCCCTGAC‐3” and FAM83A-AS1‐reverse: 5“‐CCCGAGCCCTCTTATCCTCTATGG‐3”; MMP2‐forward: 5“‐CACAGGAGGAGAAGGCTGTG‐3” and MMP2‐reverse: 5“‐GAGCTTGGGAAAGCCAGGAT‐3”; MMP9‐forward: 5“‐TTCAGGGAGACGCCCATTTC‐3” and MMP9‐reverse: 5“‐TGTAGAGTCTCTCGCTGGGG‐3”.
2.7 Cell migration and invasion assay
Transwell assay was performed via transwell chamber (pore diameter, 8 µm; MilliporeSigma). Transfected pancreatic cancer cells were resuspended by serum-free medium and subsequently seeded into the upper layer of the chamber (6 × 104 cells/100 µl) while complete medium was added to the chamber bottom. After 20 h, the cells on the lower surface were fixed by 4% polyformaldehyde (Aladdin, Shanghai, China) and stained with 0.05% crystal violet (Beyotime, Shanghai, China) for 30 min at room temperature whereas the cells on the upper surface were wiped slightly. An inverted light microscope (Olympus Corporation) was to capture the images. Cell invasion assay was similar to migration assay except that cells were seeded in the matrigel-coated transwell inserts (BD Bioscience, Corning, NY, USA).
2.8 Wound healing assay
Transfected pancreatic cancer cells (2 × 105/well) were seeded in 24-well plates for 24 h and then scratched in wells with a 10 μl micropipette tip. Nonadherent cells were washed away by phosphate buffer saline (PBS), and fresh medium was added. Pictures of wounds were imaged at 0 h and 20 h with a microscope. The wound-healing rate was calculated as: 100% × [(wound width at 0 h − width at 20 h)/width at 0 h].
2.9 Western blotting
Cells were rinsed with cold PBS and then lysed with RIPA buffer containing 1% PMSF, 1% protease inhibitor, 5% 2‐mercaptoethanol and 93% 2× loading buffer. The cell lysate boiled at 100°C for 10 min was centrifuged at 12 000 g for 10 min. Protein concentration was tested by the BCA assay. Subsequently, the total protein was separated by SDS-PAGE and transferred onto PVDF membranes for immunoblot assays. Membranes were blocked with 5% BSA for 1 h at room temperature and incubated with primary antibodies overnight at 4°C. The membranes were washed and incubated with the respective HRP-conjugated secondary antibody for 1 h at room temperature and then visualized with ECL detection system (Amersham Pharmacia Biotech, Little Chalfont, UK). The antibodies were rabbit anti-MMP2 (ImmunoWay, CAT YT2798), rabbit anti-MMP9 (ImmunoWay, CAT YT1892), rabbit anti-E-cadherin (Cell Signaling, CAT 3195), rabbit anti-N-cadherin (Cell Signaling, CAT 13,116), rabbit anti-β-catenin (Cell Signaling, CAT 8480), rabbit anti-Vimentin (Cell Signaling, CAT 5741), rabbit anti-Snail (Cell Signaling, CAT 3879), rabbit anti-Slug (Cell Signaling, CAT 9585), rabbit anti-β-Tubulin (Abcam, CAT 21,058) and Hippo Signaling Antibody Sampler Kit (Cell Signaling, CAT 8579).
2.10 Cell Counting Kit (CCK)-8 assay
After 48 h transfection, 1 × 103 cells/100 μL were seeded into 96-well plates each well. Then, cells were incubated with 100 μl CCK8 reagent mixture (10 μl CCK8 reagent: 90 μl DMEM) without light for 2 h at 37°C. The results were measured at 450 nm absorbance by a microplate reader (Bio-Rad, Hercules, CA, USA) and analyzed via GraphPad Prism version 7.
2.11 Colony formation assay
Single-cell suspensions were plated in 6-well plates at 1 × 103 cells/well and maintained at 37°C and 5% CO2 for 10 – 14 d. The supernatant was changed every 3 d. Finally, 4% paraformaldehyde was used to fix colonies and 0.5% crystal violet was for staining for 30 min. The number of visible colonies was counted by Image J.
2.12 Statistical analysis
Each experiment was performed separately at least three times. The data were presented as the mean ± standard deviation (SD). Student’s t-test determined the statistical significance between the two groups, while a two-way analysis of variance (ANOVA) was used to analyze the data more than three groups. The results were analyzed via GraphPad Prism 7 software. P < 0.05 was considered statistically significant.
3. Results
3.1 Fam83A-AS1 is abnormally expressed in PC and associated with patients’ diagnosis and prognosis
To investigate the role of FAM83A-AS1, we firstly analyzed the relative expression of FAM83A-AS1 in human tumors and found that FAM83A-AS1 was abnormal in gastrointestinal tumors, especially PC (). Subsequently, we explored the FAM83A-AS1 significance in PC tissues through analyzing TCGA and GTEx databases, confirming that FAM83A-AS1 expression was higher in PC tissues than normal tissues () and associated with pathological stages (T stage) (). In addition, we evaluated the prognosis value of FAM83A-AS1 in PC and found that high expression of FAM83A-AS1 resulted in poor prognosis both at survival and disease-free survival rates (, f). Then, we proved that FAM83A-AS1 had good predictive value due to the area under the curve (AUC) exceeded 0.75 in the prediction model (). We also studied the relationship between FAM83A-AS1 expression and 1, 3 and 5 y survival. The 1 y AUC was 0.652, and 3 y was 0.743, 5 y was 0.933 (), which indicated that FAM83A-AS1 played a vital role in predicting prognosis of pancreatic cancer.
Figure 1. The expression and prognosis of FAM83A-AS1 in human pancreatic cancer.
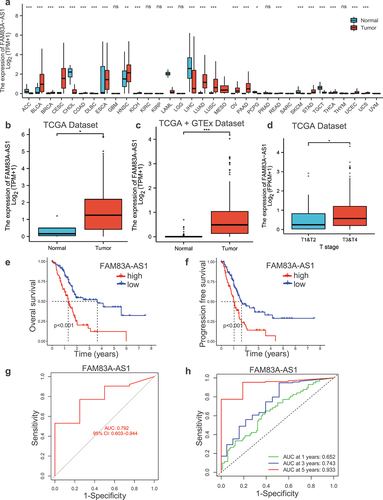
3.2 Fam83A-AS1-related differentially expressed genes (DEGs) and functional enrichment analysis was identified in PC
Comparing tumor tissues with adjacent nontumorous tissues in TCGA database, a total of 353 genes were differentially expressed between the FAM83A-AS1 high-group and low-group, including 64 upregulated DEGs and 289 downregulated DEGs (adjusted P value < 0.05, |Log2-FC| > 2) (). And then, we got the top 10 FAM83A-AS1-related DEGs, such as CASP14, KLK2, UPK2, MUC21, FAM83A, SLURP1, C6orf15, KLK3, A2ML1 and KRT6A (). Subsequently, GO enrichment analysis was done, containing biological processes (BP), cellular compositions (CC) and molecular functions (MF), to reveal that DEGs were enriched in different GO terms such as epidermis development in BP, cell-substrate junction and focal adhesion in CC, cadherin binding in MF (). Meanwhile, KEGG pathway analysis showed that significantly DEGs-enriched pathways contained protoglycans in cancer, axon guidance, focal adhesion, ECM–receptor interaction, glycolysis/gluconeogenesis, HIF-1 signaling pathway, central carbon metabolism in cancer, p53 signaling pathway and nucleotide metabolism (). Moreover, GSEA analyses were applied to explore the potential biological mechanism related to DEGs. The results indicated that spliceosome and cell cycle were relative to high-FAM83A-AS1 expression as well as calcium signaling pathway, maturity onset diabetes of the young and neuroactive ligand receptor interaction were related to low-FAM83A-AS1 expression (). Additionally, we analyzed the relationship between FAM83A-AS1 expression and immune cell infiltration in pancreatic cancer and found that macrophages M0 and B cell memory were positively correlated to FAM83A-AS1 expression, while T cells CD8 was negative correlation ().
Figure 2. Differentially expressed genes and functional enrichment analysis.
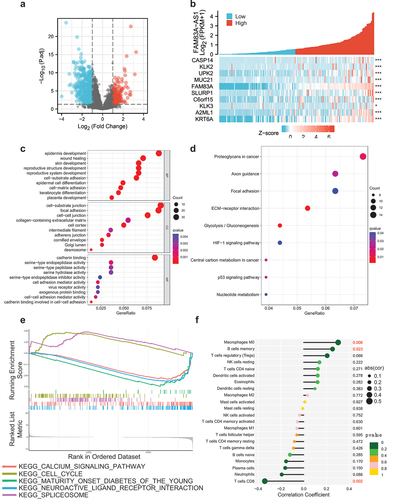
3.3 Fam83A-AS1 was profiled and promoted migration in PC cells
To investigate whether FAM83A-AS1 is overexpressed in PC cells, we detected the expression of FAM83A-AS1 in human immortalized pancreatic ductal epithelial cell line H6C7 and PC cell lines PaTu8988, PANC-1 and BxPC-3. The results showed that FAM83A-AS1 was higher in PC cell than pancreatic ductal epithelial cell and was relatively highest in BxPC-3, second in PANC-1 and lowest in PaTu8988 cell (). Then, si-FAM83A-AS1 was transfected in BxPC-3 and PANC-1 cells while pcDNA3.1-FAM83A-AS1 in PANC-1 and PaTu8988 cells and the results displayed that plasmid construction was successful (). Therefore, transwell experiment was performed to detect the effect of FAM83A-AS1 in PC cells. The results demonstrated that the number of migrated cells was significantly reduced in si-FAM83A-AS1 group of BxPC-3 and PANC-1 cells () whereas much more migrated cells in pcDNA3.1-FAM83A-AS1 group of PANC-1 and PaTu8988 cells (). In addition, the wound healing assay indicated that FAM83A-AS1 downregulation weakened the migratory capacity of BxPC-3 and PANC-1 cells (), and in comparison, FAM83A-AS1 upregulation increased motility of PANC-1 and PaTu8988 cells (). The results above showed that FAM83A-AS1 promoted the migration of PC cells.
Figure 3. Fam83A-AS1 is upregulated in pancreatic cancer cells.
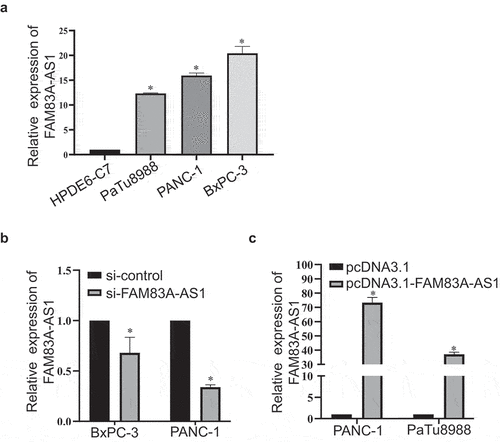
Figure 4. Fam83A-AS1 enhances the migration ability of pancreatic cancer cells.
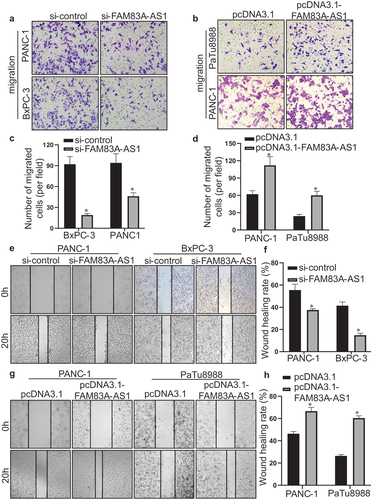
3.4 Fam83A-AS1 enhanced the invasion ability of PC cells
To further explore the effect of FAM83A-AS1 on pancreatic cancer cell invasion, we performed transwell BD assay to detect the invasive capability of PC cells. As shown in , the number of invasive cells transfected with si-FAM83A-AS1 decreased in BxPC-3 and PANC-1 cells (). Meanwhile, FAM83A-AS1 overexpression increased the number of invasive cells in PANC-1 and PaTu8988 cells (). Besides, western blot and qRT-PCR were performed to confirm the role of FAM83A-AS1 on PC cell invasion. At both protein and mRNA level, the FAM83A-AS1 knockdown decreased MMP2 and MMP9 expression of BxPC-3 and PANC-1 cells (). On the contrary, the FAM83A-AS1 upregulation increased MMP2 and MMP9 expression of PANC-1 and PaTu8988 cells at protein and mRNA level (). The above results demonstrated that FAM83A-AS1 enhanced the invasive ability of PC cells.
Figure 5. Fam83A-AS1 enhances the invasion ability of pancreatic cancer cells.
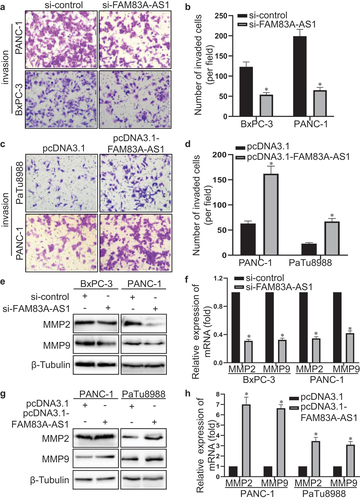
3.5 Fam83A-AS1 stimulated the proliferation of PC cells
Furthermore, CCK-8 assay and colony formation test were used to assess the function of FAM83A-AS1 on pancreatic cancer cell proliferation. It was found that FAM83A-AS1 downregulation slowed down the growth of BxPC-3 and PANC-1 cells (). In turn, the upregulation of FAM83A-AS1 accelerated the growth of PANC-1 and PaTu8988 cells (). In addition, the colony-forming capacity of BxPC-3 and PANC-1 cells transfected with si-FAM83A-AS1 was decreased () while the colony-forming ability of PANC-1 and PaTu8988 cells with FAM83A-AS1 upregulation was enhanced (). The above results suggested that FAM83A-AS1 promoted the proliferation of PC cells.
Figure 6. Fam83A-AS1 promotes the proliferation of pancreatic cancer cells.
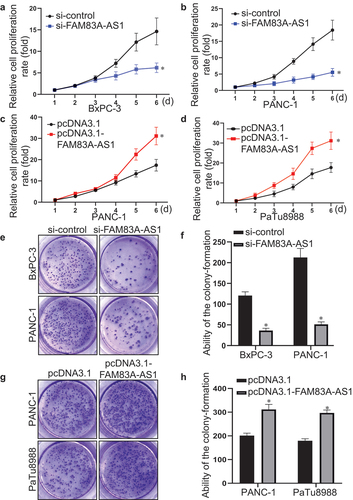
3.6 Fam83A-AS1 induced EMT via Hippo/YAP pathway inactivation in PC cells
The activation of EMT was associated with enhanced invasion, migration and proliferation ability [Citation13]. Moreover, our previous bioinformatics analysis showed that FAM83A-AS1 was related to cadherin binding (). Therefore, the protein markers of EMT were detected in PC cells. The western blot data displayed that the knockdown of FAM83A-AS1 resulted in E-cadherin upregulation and downregulation of N-cadherin, β-catenin, Vimentin, Snail and Slug (). Meanwhile, the FAM83A-AS1 overexpression contributed to the decrease of E-cadherin and increase of N-cadherin, β-catenin, Vimentin, Snail and Slug (). As Hippo pathway plays a vital role in malignant transformation and cell proliferation [Citation14], western blot was used to study the relationship between FAM83A-AS1 and Hippo signaling. Compared to control group, FAM83A-AS1 downregulation increased the expression of p-YAP, p-MOB1, p-Lats1, SAV1, MST1 and MST2 and decreased the expression of YAP, MOB1 and Lats1 in BxPC-3 and PANC-1 cells (). In contrary, the increase of FAM83A-AS1 reduced the expression of p-YAP, p-MOB1, p-Lats1, SAV1, MST1 and MST2 and increased the expression of YAP, MOB1 and Lats1 in PANC-1 and PaTu8988 cells (). It is indicated that FAM83A-AS1 can inhibit the activation of Hippo/YAP signaling pathway to promote EMT in PC cells.
Figure 7. Fam83A-AS1 promotes the EMT in pancreatic cancer cells via the activation of Hippo/YAP pathway.
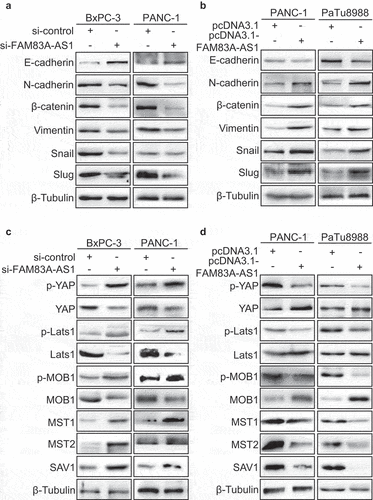
4. Disscusion
The present study demonstrates that FAM83A-AS1 expression was higher in PC tissues and cells than normal. Then, the bioinformatic analysis showed that FAM83A-AS1 high expression was related to poor overall survival of PC and participated in various biological processes, especially cadherin binding and immune infiltration. Subsequently, we proved FAM83A-AS1 enhanced the migration, invasion and proliferation abilities of PC cells. Furthermore, it was verified that FAM83A-AS1 inhibited Hippo pathway to active YAP to promote EMT in PC cells.
Pancreatic cancer is a fatal disease with poor therapeutic effect, and the study of its mechanism is urgent [Citation15,Citation16]. LncRNAs are noncoding transcripts longer than 200 nucleotides [Citation17]. LncRNAs were once thought to be transcriptional noise and now have been proved to participate in various mechanisms, such as transcription, translation, protein modification and RNA-protein or protein–protein complex formation [Citation18]. Many studies have demonstrated that aberrant lncRNA expression may play an oncogene or antioncogene role. For example, LncRNA CEBPA-DT interacted with hnRNPC to promote liver cancer metastasis [Citation19], while LncRNA ELF3-AS1 inhibited gastric cancer via interacting with ILF2/ILF3 complex [Citation20]. LncRNA FAM83A-AS1 is the antisense transcript of FAM83A. Previous studies discovered that FAM83A-AS1 served as an oncogene in lung cancer [Citation8], hepatocellular carcinoma [Citation10] and esophageal cancer [Citation9]. However, the role of FAM83A-AS1 in pancreatic cancer is unclear. In this study, we explored the FAM83A-AS1 function in pancreatic cancer cells. Firstly, we analyzed FAM83A-AS1 expression in TCGA database and found that FAM83A-AS1 overexpressed in PC tissues and cells than normal. Then, we found FAM83A-AS1 was related to poor prognosis and involved in cadherin binding and immune infiltration in pancreatic cancer. It is implied that FAM83A-AS1 may serve as an oncogene in pancreatic cancer.
Epithelial–mesenchymal transition (EMT) is a critical progress in pancreatic cancer, increasing cancer cell motility and invasiveness through enabling epithelial cells to lose polarity and tight junction and acquire a mesenchymal phenotype feature [Citation21]. In addition, lncRNAs have been found to participate in the regulation of EMT [Citation22]. For example, lncRNA SNHG6 could promote the EMT process in breast cancer [Citation23]. In pancreatic cancer, PMSB8-AS1 contributed to proliferation, migration, invasion and EMT [Citation24]. In order to explore the effect of FAM83A-AS1 on pancreatic cancer cell, we did the migration and invasion assays and found that FAM83A-AS1 enhanced the abilities of migration and invasion. Meanwhile, the proliferation of pancreatic cancer cell was also promoted by FAM83A-AS1. In addition, FAM83A-AS1 could induce the EMT in pancreatic cancer cell. However, the underlying mechanism is still unclear.
Many studies show that Hippo pathway is associated with various diseases, most notably cancer [Citation25]. Hippo pathway was first discovered to suppress the tissues growth in Drosophila [Citation26]. In mammals, the Hippo pathway includes four tumor suppressor genes (LATS1/2, SAV1, MOB1, MST1/2) and downstream effectors YAP and TAZ [Citation26]. These tumor suppressors constitute a kinase cascade, MST1/2–SAV1 complex phosphorylating to activate the LATS1/2–MOB1A/B complex, and then phosphorylates and inactivates YAP and TAZ [Citation26]. These components of the Hippo pathway play an important role in regulating cell proliferation, regeneration and organ size control [Citation26]. And that several lncRNAs were proved to take effect through participating in Hippo pathway activation and inactivation. Liu et al. found that MNX1-AS1 facilitates tumorigenesis of gallbladder via inactivation of Hippo pathway [Citation27]. In pancreatic cancer, LINC00941 resulted in Hippo pathway activation to enhance glycolysis [Citation28], and Zhang et al. reported that lncUCA1 promotes migration and invasion via the Hippo pathway [Citation29]. Previous studies have showed that Hippo pathway may play an important role in pancreatic cancer. Therefore, we hypothesized that FAM83A-AS1 may promote EMT of pancreatic cancer cells through Hippo signaling. As expected, the results of our experiments indicated that FAM83A-AS1 could inactive the Hippo pathway to promote the proliferation and EMT of pancreatic cancer cells.
To conclude, our study indicated that FAM83A-AS1 expression was higher in PC tissues and cells than normal. FAM83A-AS1 was related to poor overall survival of PC and participated in various biological processes, especially cadherin binding and immune infiltration. The data from the present study demonstrated that FAM83A-AS1 promoted the migration, invasion, proliferation and EMT in PC cells. Mechanistically, FAM83A-AS1 inhibited Hippo pathway activation to active YAP to promote EMT in PC cells. Our study suggested that FAM83A-AS1 may serve as a candidate target for pancreatic cancer diagnosis and prognosis.
Author Contributions
All authors contributed to the study conception and design. Material preparation, data collection and analysis were performed by Huizhi Wang, Yuntao Ding, Qiuming Zhu, Zhengyue Yu and Qi Wang. Aihua Gong and Min Xu designed the experiment. The first draft of the manuscript was written by Huizhi Wang and all authors commented on previous versions of the manuscript. All authors read and approved the final manuscript.
Disclosure statement
No potential conflict of interest was reported by the authors.
Data availability statement
The authors confirm that the data supporting the findings of this study are available within the article.
Additional information
Funding
References
- Siegel RL, Miller KD, Fuchs HE, et al. Cancer statistics, 2022. CA Cancer J Clin. 2022;72(1):7–33.
- Wood LD, Canto MI, Jaffee EM, et al. Pancreatic cancer: pathogenesis, screening, diagnosis, and treatment. Gastroenterology. 2022;163(2):386–402.e1.
- Nojima T, Proudfoot NJ. Mechanisms of lncRNA biogenesis as revealed by nascent transcriptomics. Nat Rev Mol Cell Biol. 2022;23(6):389–406.
- Chen YT, Kan CH, Liu H, et al. Modular scaffolding by lncRNA HOXA10-AS promotes oral cancer progression. Cell Death Dis. 2022;13(7):629.
- Zhou C, Yi C, Yi Y, et al. LncRNA PVT1 promotes gemcitabine resistance of pancreatic cancer via activating Wnt/β-catenin and autophagy pathway through modulating the miR-619-5p/Pygo2 and miR-619-5p/ATG14 axes. Mol Cancer. 2020;19(1):118.
- Shi R, Jiao Z, Yu A, et al. Long noncoding antisense RNA FAM83A-AS1 promotes lung cancer cell progression by increasing FAM83A. J Cell Biochem. 2019;120(6):10505–10512.
- Chen Z, Hu Z, Sui Q, et al. LncRNA FAM83A-AS1 facilitates tumor proliferation and the migration via the HIF-1α/glycolysis axis in lung adenocarcinoma. Int J Biol Sci. 2022;18(2):522–535.
- Zhao H, Wang Y, Wu X, et al. FAM83A antisense RNA 1 (Fam83A-AS1) silencing impairs cell proliferation and induces autophagy via MET-AMPKα signaling in lung adenocarcinoma. Bioengineered. 2022;13(5):13312–13327.
- Jia J, Li H, Chu J, et al. LncRNA FAM83A-AS1 promotes ESCC progression by regulating miR-214/CDC25B axis. J Cancer. 2021;12(4):1200–1211.
- He J, Yu J. Long noncoding RNA FAM83A-AS1 facilitates hepatocellular carcinoma progression by binding with NOP58 to enhance the mRNA stability of FAM83A. Biosci Rep. 2019;39(11):BSR20192550.
- Zhou C, Liang Y, Zhou L, et al. TSPAN1 promotes autophagy flux and mediates cooperation between WNT-CTNNB1 signaling and autophagy via the MIR454-FAM83A-TSPAN1 axis in pancreatic cancer. Autophagy. 2021;17(10):3175–3195.
- Hänzelmann S, Castelo R, Guinney J. GSVA: gene set variation analysis for microarray and RNA-seq data. BMC Bioinf. 2013;14:7.
- Liu L, Han S, Xiao X, et al. Glucocorticoid-induced microRNA-378 signaling mediates the progression of pancreatic cancer by enhancing autophagy. Cell Death Dis. 2022;13(12):1052.
- Li F, Negi V, Yang P, et al. TEAD1 regulates cell proliferation through a pocket-independent transcription repression mechanism. Nucleic Acids Res. 2022;gkac1063(22):12723–12738.
- Papadakos SP, Dedes N, Gkolemi N, et al. The EPH/ephrin system in Pancreatic Ductal Adenocarcinoma (PDAC): from pathogenesis to treatment. Int J Mol Sci. 2023;24(3):3015.
- Papadakos SP, Dedes N, Pergaris A, et al. Exosomes in the treatment of pancreatic cancer: a moonshot to PDAC treatment? Int J Mol Sci. 2022;23(7):3620.
- Bridges MC, Daulagala AC, Kourtidis A. Lnccation: lncRNA localization and function. J Cell Bio. 2021;220(2):e202009045.
- Herman AB, Tsitsipatis D, Gorospe M. Integrated lncRNA function upon genomic and epigenomic regulation. Mol Cell. 2022;82(12):2252–2266.
- Cai Y, Lyu T, Li H, et al. LncRNA CEBPA-DT promotes liver cancer metastasis through DDR2/β-catenin activation via interacting with hnRNPC. J Exp Clin Cancer Res. 2022;41(1):335.
- Li D, Shen L, Zhang X, et al. LncRNA ELF3-AS1 inhibits gastric cancer by forming a negative feedback loop with SNAI2 and regulates ELF3 mRNA stability via interacting with ILF2/ILF3 complex. J Exp Clin Cancer Res. 2022;41(1):332.
- Kwon MR, Lee JH, Park J, et al. NCK-associated protein 1 regulates metastasis and is a novel prognostic marker for colorectal cancer. Cell Death Discov. 2023;9(1):7.
- Hashemi M, Hajimazdarany S, Mohan CD, et al. Long non-coding RNA/epithelial-mesenchymal transition axis in human cancers: tumorigenesis, chemoresistance, and radioresistance. Pharmacol Res. 2022;186:106535.
- Khan MI, Ahmad A. LncRNA SNHG6 sponges miR-101 and induces tamoxifen resistance in breast cancer cells through induction of EMT. Front Oncol. 2022;12:1015428.
- Zhang H, Zhu C, He Z, et al. LncRNA PSMB8-AS1 contributes to pancreatic cancer progression via modulating miR-382-3p/STAT1/PD-L1 axis. J Exp Clin Cancer Res. 2020;39(1):179.
- Sharma U, Tuli HS, Uttam V, et al. Role of Hedgehog and Hippo signaling pathways in cancer: a special focus on non-coding RNAs. Pharmacol Res. 2022;186:106523.
- Fu M, Hu Y, Lan T, et al. The Hippo signalling pathway and its implications in human health and diseases. Signal Transduct Target Ther. 2022;7(1):376.
- Liu S, Li H, Zhu Y, et al. LncRNA MNX1-AS1 sustains inactivation of Hippo pathway through a positive feedback loop with USP16/IGF2BP3 axis in gallbladder cancer. Cancer Lett. 2022;547:215862.
- Xu M, Cui R, Ye L, et al. LINC00941 promotes glycolysis in pancreatic cancer by modulating the Hippo pathway. Mol Ther Nucleic Acids. 2021;26:280–294.
- Zhang M, Zhao Y, Zhang Y, et al. LncRNA UCA1 promotes migration and invasion in pancreatic cancer cells via the Hippo pathway. Biochim Biophys Acta Mol Basis Dis. 2018;1864(5):1770–1782.