ABSTRACT
Acute lung injury (ALI)/acute respiratory distress syndrome (ARDS) is a critical and life-threatening illness that causes severe dyspnea, and respiratory distress and is often caused by a variety of direct or indirect factors that damage the alveolar epithelium and capillary endothelial cells, leading to inflammation factors and macrophage infiltration. Macrophages play a crucial role in the progression of ALI/ARDS, exhibiting different polarized forms at different stages of the disease that control the disease outcome. MicroRNAs (miRNA) are conserved, endogenous, short non-coding RNAs composed of 18–25 nucleotides that serve as potential markers for many diseases and are involved in various biological processes, including cell proliferation, apoptosis, and differentiation. In this review, we provide a brief overview of miRNA expression in ALI/ARDS and summarize recent research on the mechanism and pathways by which miRNAs respond to macrophage polarization, inflammation, and apoptosis. The characteristics of each pathway are also summarized to provide a comprehensive understanding of the role of miRNAs in regulating macrophage polarization during ALI/ARDS.
Acute lung injury (ALI) is a clinical syndrome characterized by alveolar epithelial and lung capillary endothelial cell damage, increased alveolar-capillary permeability, and gas exchange dysfunction. It can be caused by various direct or indirect factors, such as severe infection, septicemia, trauma, shock, burn, and poisoning (). Neutrophil infiltration, inflammation, and oxidative stress are hallmarks of ALI [Citation1–3], and uncontrolled inflammation can result in acute respiratory distress syndrome (ARDS), and eventually multi-organ dysfunction [Citation2].
Table 1. Related factors causing ALI/ARDS.
Microribonucleic acid (miRNA) is a conserved, endogenous, short non-coding ribonucleic acid composed of 18–25 nucleotides. It is abundant in the body and post-transcriptionally regulates gene expression by incomplete complementary base-pairing with target sites in the 3´ -terminal untranslated region of the mRNA, reducing its stability, mediating mRNA cleavage, or inhibiting the process of translation. miRNA is a key regulator involved in various biological processes, including cell proliferation, differentiation, metabolism, apoptosis, inflammation, tumorigenesis, and vascularization [Citation4–9]. Numerous studies have shown that miRNAs play a role in ALI through different mechanisms. Therefore, miRNA is closely linked to the occurrence and development of ALI.
This review summarizes some specific miRNAs associated with ALI, including their role in macrophage polarization, inflammation, and apoptosis, as well as specific regulatory mechanisms. It also describes the relationship between miRNAs and related therapeutic modalities, as well as in vivo miRNAs delivery pathways.
1 Macrophage polarization and acute lung injury
The pathogenesis of ALI is well-known to exhibit both early and late stages of lung injury. In the early stage, occurring from the first few hours to several days, histopathological changes in the lungs include alveolar epithelial and capillary endothelial cell damage, increased microvascular permeability, exudation of inflammatory cells and cytokines, and tiny alveolar consolidation and edema. The late stage, occurring several days later, is characterized by lung tissue fibrosis [Citation10–12]. Despite the numerous scientific and technological advancements, ALI still poses a significant threat to human life and health, given its rapid clinical development, high pathogenic rate, lack of effective treatment, poor prognosis, and high mortality rate. Therefore, research focusing on potential clinical solutions to improve and even eradicate ALI is essential.
Recently, there has been an increasing amount of evidence indicating that macrophages play a key role in the pathogenesis of ALI [Citation13,Citation14]. Macrophages are relatively long-lived cells with many important functions. They are responsible for the destruction of invading microorganisms through phagocytosis, which is an important first line of defense in innate immunity. Additionally, they modulate adaptive immune responses to different pathogens via antigen processing and presentation [Citation15]. Macrophages not only participate in the early stages of lung injury but also play a vital role in the repair and fibrosis stages of lung injury [Citation16]. Macrophages can be classified into two types: M1 (classical activation) and M2 (alternative activation). M1, also referred to as pro-inflammatory phenotype, primarily produces pro-inflammatory cytokines such as tumor necrosis factor, IL-6, IL-1 β, and IL-8, etc., and mainly functions in the early stages of inflammation. M2 type, or the anti-inflammatory phenotype, mainly releases a variety of cytokines such as IL-10, TGF-β, and VEGF, exerting anti-inflammatory effects in the late inflammatory response and promoting wound repair and fibrosis. M2 macrophages can be further divided into subtypes such as M2a, M2b, M2c, and M2d [Citation17]. Therefore, different macrophage types play different roles in the occurrence and development of lung damage at different stages. When subjected to severe infection or inflammation, macrophages first exhibit the M1 phenotype to release TNF-α, IL-1β, IL-12, and IL-23 to fight stimuli. However, if the M1 phase continues, it can lead to tissue damage. Thus, M2 macrophages secrete large amounts of IL-10 and TGF-β to inhibit inflammation, contribute to tissue repair, remodeling, angiogenesis, and preserve homeostasis [Citation18,Citation19]. Therefore, exploring the mechanism of macrophage polarization transformation, and regulating the initiation, development, and end of the inflammatory response by targeting signaling pathways and molecular changes in the local microenvironment to transform macrophages into appropriate phenotypes will have excellent therapeutic prospects.
Macrophages play a crucial role in acute lung injury. Firstly, nonendogenous pathogen-associated molecular patterns (PAMPs), such as lipopolysaccharides and lipoteichoic acid, bind to pattern recognition receptors (PRRs), initiating an intra-inflammatory signaling cascade that results in the release of pro-inflammatory cytokines, such as tumor necrosis factor α (TNF-α), interleukin (IL)-1β, and IL-8 [Citation20]. Secondly, mitochondrial damage-associated molecular patterns (DAMPs) released from damaged cells, such as cardiolipin, formyl peptides, and mitochondrial DNA [Citation21,Citation22], further stimulate the production of the pro-inflammatory cytokine IL-8, which in turn activates neutrophils, which migrate to the alveolus [Citation23]. Activated neutrophils secrete matrix metalloproteinases (MMPs), reactive oxygen species (ROS), and neutrophil extracellular traps (NETs) [Citation24,Citation25], which exert bactericidal effects. MMPs are the primary proteolytic enzymes in extracellular matrix (ECM) and are involved in cell migration and invasion [Citation26]. Numerous studies have shown that MMPs are closely linked to tumor progression. Under the influence of cytokines such as IL-1 β, and TNF-α, MMPs act on microvascular endothelial cells, transforming them into interstitial fibroblasts. This process is known as endothelial mesenchymal transformation (EndMT) [Citation27,Citation28]. In the late stage of acute lung injury, MMPs may play a vital role in the formation of pulmonary fibrosis, which is also associated with the participation of transforming growth factor β (TGF-β) [Citation29,Citation30].
Macrophages are not only directly involved in the progression of ALI, but also indirectly induce other inflammatory cells to participate in the occurrence and development of ALI. Therefore, exploring macrophage function will further reveal the mechanism of acute lung injury. The schematic diagram presented in shows cellular changes at different stages during the development of acute lung injury, including the type of macrophage polarization, neutrophils, and monocyte infiltration, which secrete large amounts of inflammatory cytokines, and thus directly or indirectly participate in the occurrence and development of acute lung injury.
Figure 1. Cell response plots of acute lung injury. When infected bacteria or viruses, macrophages act as the first line of defense, the alveolar macrophages identify PAMPs (such as bacterial lipopolysaccharides, phosphate acid, etc.), and polarize to M1 type, release proinflammatory cytokines, including IL-6, IL-8, TNF-α in response to damage, then cell endogenous damage product DAMPs (such as mitochondrial DNA, etc.), combined with macrophage surface receptor, further stimulate the production of pro-inflammatory cytokines. The release of a large number of inflammatory factors then leads to tissue damage.Therefore, in the late stage of injury, macrophages are polarized to M2 type to cope with the pro-inflammatory changes of M1 type, release anti-inflammatory cytokines such as IL-10, and maintain the counterbalance of inflammation in the body. When uncontrolled inflammation breaks the balance between pro-inflammatory and anti-inflammatory conditions, it then leads to epithelial and endothelial cell damage, which eventually develops into acute lung injury. On the other hand, the proinflammatory cytokine IL-8 promotes neutrophil activation and migration to the alveoli, and releases MMPs, MPO, as well as NETs, to exert antibacterial effects. In the later stage of the disease, under the combination of proinflammatory cytokines such as IL-1 β and TNF- α, MMPs released by neutrophils act on microvascular endothelial cells to transform endothelial cells into stromal fibroblasts, further disrupt microvascular structure, affect gas exchange, and eventually develop into ARDS. PAMPs, nonendogenous pathogen-associated molecular patterns; DAMPs, damage associated molecular patterns; MMPs, matrix metalloproteinases; MPO, myeloperoxidase; NETs, neutrophil extracellular traps; ARDS, acute respiratory distress syndrome.
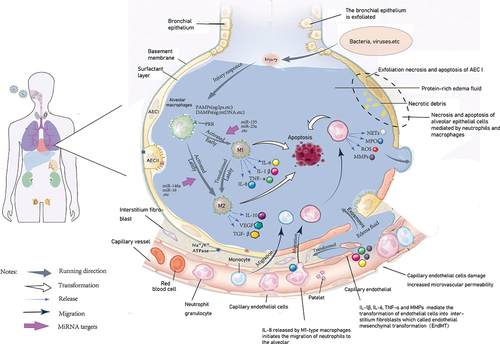
2 miRNA and acute lung injury
2.1 miRNA basics
MiRNAs function is similar to that of endogenous or synthetic short interfering RNA (siRNAs); however, miRNAs play more complex and subtle roles than suggested by the “switch” model [Citation1,Citation4,Citation31]. miRNA expression is highly tissue-dependent. For instance, miR-451 is expressed the most in the kidney, miR-181a, miR-181b, and miR-214 in the liver, and miR-214, miR-24, miR-9, and miR-124 in the brain [Citation32], whereas other miRNAs are expressed in the heart tissue [Citation33]. Therefore, differential expression of miRNAs can be useful as potential biomarkers for monitoring various diseases [Citation34–36].
MicroRNA undergoes a lengthy and intricate process from generation to function, involving dynamic changes from initial transcription to binding with target mRNA. miRNAs play a predominant role in the complex network of balanced relationships between pro-inflammatory and anti-inflammatory factors, adapting and coordinating with different clinical manifestations of high or low expression of inflammatory factors. The dynamic changes in RNA can monitor the state of health and disease in real-time, allowing for early identification of risks, intervention, and improved pathological and clinical performance at the initial stages of the disease. MiRNAs also play an indispensable role in the prognosis, diagnosis, and treatment of the disease [Citation37]. However, the conclusions of many studies involving various miRNAs are not uniform, as the expression of miRNAs can be either upregulated or downregulated. Additionally, the response mechanisms and pathways to inflammation and apoptosis can also differ. In this review, we summarize the entire process of miRNA from generation to function, emphasizing that up or down-regulation of miRNA in acute lung injury can subsequently inhibit or strengthen downstream inflammatory or apoptotic pathways by interfering with the target mRNA (as depicted in ).
Figure 2. A schematic illustration of miRNA biogenesis and functions in ALI. RNA polymerase II (RNA Pol II) ordinate the synthesis of pre-miRNA. Then pre-miRNA was exported to the cytoplasm where pre-miRNA generates a mature miRNA.Mature miRNAs mediate the transcription and translation inhibition of target mRNAs and the degradation of their own mRNAs. In the development of ALI, miRNA itself is up-regulated or down-regulated, leading to the reduction or improvement of the level of target genes, which then leads to the enhancement or inhibition of downstream inflammatory and apoptotic pathways, and mediates macrophage polarization, thereby aggravating or alleviating the progress of ALI. Pri-miRNA, primitive miRNA; miRNA,microRNA; pre-miRNA, recursor-miRNA;DROSHA,RNase III endonuclease;DGCR8,DiGeorge syndrome critical region 8;XPO-5,exportin 5;TRBP, transactivation-responsive RNA-binding protein;DICER,RNase III;RISC,RNA-induced silencing complex. Created with BioRender.com.
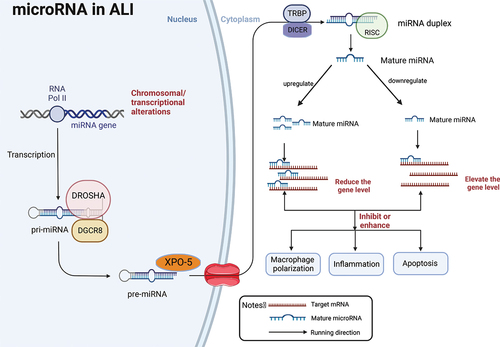
2.2 Regulation of the miRNA expression in acute lung injury
The expression level of miRNA expression directly influences the developmental outcome of ALI. How is the miRNA expression regulated? In response to bacterial or viral stimulation, alveolar macrophages first exhibit the M1 type and produce numerous pro-inflammatory cytokines, such as IL-6, IL-1 β, IL-8 and TNF- α, which subsequently stimulates the expression of pro-inflammatory microRNAs, such as miRNA-155, miRNA-23a, and miRNA-34a. These miRNAs further aggravate inflammatory and apoptotic signals, promoting the progression of lung injury. However, at the same time, the expression of some anti-inflammatory miRNAs, such as miRNA-223, miRNA-146a, miRNA-135a, and miRNA-21, is also up-regulated. This may be due to the passive up-regulation response of the body to achieve a balance between pro-inflammatory and anti-inflammatory signals, which exerts a protective effect. In addition, some non-coding RNAs, such as lncRNA and cicRNA [Citation38–40], can adsorb specific miRNAs to increase the levels of downstream target genes, which also indirectly affects miRNA expression.
This paper briefly describes the expression of miRNAs in ALI, along with the response mechanisms and pathways of miRNAs to macrophage polarization, inflammation, and apoptosis. However, there are conflicting conclusions (as summarized in ).
Table 2. miRNAs in ALI.
3 miRNA associated with macrophage polarization in lung injury
3.1 miRNA 223 and miRNA 142
miR-223, originally identified through bioinformatics and characterized in the hematopoietic system, is highly expressed in neutrophil lines and is considered a specific miRNA for neutrophils [Citation67,Citation68]. It plays a key role in controlling excessive inflammatory responses and neutrophil activation [Citation69,Citation70]. Recent studies have demonstrated that EV-derived miR-223 inhibits liver inflammation and fibrosis gene expression, facilitates neutrophil-hepatocyte communication, and slows the progression of the nonalcoholic steatohepatitis (NASH) [Citation71]. Another RNA that is crucial for neutrophil differentiation and maturation is miRNA-142, which has been shown to result in abnormal reduction and over-maturation of neutrophils during eventual myelogenesis, as well as impaired inflammatory migration of neutrophils during fetal production [Citation72]. Investigating the function of neutrophils, including activation, maturation, and migration, using a combination of miR-223 and miR-142 may lead to significant breakthroughs.
In ALI/ARDS, there is still no agreement on the upregulation or downregulation of miR-223/142 expression. Some studies have shown that miR-223/142 is down-regulated, and its over-expression inhibits macrophage activation and lung inflammation, and apoptosis [Citation41,Citation73]. With regards to neutrophil function, Ly6G+ neutrophils can enhance the expression of miR-223, and the elevated miR-223 can negatively regulate bone marrow-derived Ly6G+ neutrophil differentiation and inhibits peripheral cytokine release. MiR-223 also targets the inhibition of NLRP3 expression and IL-1β release, ultimately alleviating MTD-induced ALI [Citation42]. Although most studies suggest that overexpression of miR-233 is beneficial for improving acute lung injury, whether it is upregulated or downregulated remains unclear. However, most research has focused on the role of miR-233 and miR-142 in neutrophil differentiation and maturation. Future studies can concentrate on the pivotal role of neutrophil migration to the alveoli, which may reveal new therapeutic targets for the treatment of acute lung injury.
3.2 MiRNA-146a
Inflammatory conditions have been associated with elevated expression of miRNA-146a in white adipose tissue. Transfection of miR-146a into white adipocytes has been demonstrated to down-regulate the expression of inflammatory cytokine [Citation74]. This miRNA has been found to inhibit the release of inflammatory factors such as tumor necrosis factor-αand interleukin-6, and has been shown to play a crucial role in reducing inflammation [Citation75–77], alleviate excessive inflammation and multi-organ function impairment, reduce mortality, and regulate immune response responses in splenic macrophages [Citation78]. Recent studies have demonstrated that miRNA-146a regulates the M2-type polarization of macrophages by targeting Notch1, thereby enhancing the anti-inflammatory effect [Citation43]. In addition, miR-146a controls the immune response and promotes the M2 phenotype by inhibiting the expression of proteins such as IRAK1 and IRF5 [Citation44,Citation45]. Interestingly, miRNA-146a is highly expressed in patients with acute lung injury due to sepsis, and has the potential to serve as an early diagnostic marker [Citation79].
It is evident that miRNA-146a not only directly participates in the occurrence of lung injury inflammation, but also indirectly regulates macrophages to M2-type polarization and exerts anti-inflammatory effects. Upregulation of miRNA-146a can be beneficial in improving acute lung injury. Several studies have demonstrated that miRNA-146a can distinguish between sepsis and systemic inflammatory response syndrome, making it a highly specific and sensitive biomarker [Citation80,Citation81]. Moreover, the levels of miRNA-146a correlate with the severity of inflammation and the levels of inflammatory factors [Citation82]. However, the limited sample size of some studies has lowered the credibility of the findings. Therefore, research on miRNAs cannot be used as an independent individual study, and the study of target sites of miRNAs cannot be confined to a single regulatory level.
3.3 MiRNA-181a
miRNA-181a belongs to the miRNA-181 family, which is characterized by highly conserved gene sequences [Citation83]. Studies have shown conflicting results regarding miRNA-181a expression in the LPS-induced acute lung injury (ALI) model [Citation84]. While some studies suggest that overexpression of miRNA-181a promotes the expression of caspase-3, inhibits BCL-2, and aggravates the progression of lung injury, others report that the downregulation of miRNA-181a expression improves LPS-induced apoptosis and inflammation. By targeting to SIRT1, it can be protected against sepsis-induced acute lung injury in mice by inhibiting miR-181a expression [Citation46]. Recently, Liu et al [Citation38] have reported that the downregulation of miR-181a-5p was detected in ALI/ARDS patients, and in vitro experiments suggest that overexpression of miR-181a-5p can target Fax and improve LPS-induced apoptosis and inflammation. In addition, miR-181a can inhibit the expression of TLR-4, reduce LPS-induced inflammation in vivo and in vitro, and improve acute lung injury [Citation85]. In a rat model of pulmonary hypertension, upregulation of miRNA-181a-5p expression significantly reduced right ventricular remodeling and lung injury [Citation86].
Further research is needed to clarify the exact relationship and specific mechanism between miRNA-181a and lung injury. However, it is clear that miRNA-181a is only a member of the miRNA-181 family, and the relationship between independent individuals and the whole, as well as the mutual causal relationship between miRNAs and acute lung injury, cannot be ignored. Therefore, it is essential to continue studying miRNA-181a as an independent entity while considering the broader context of the miRNA-181 family. Nevertheless, the aforementioned relationship between different miRNAs and their causal relationship with acute lung injury cannot be ignored.
3.4 MiRNA-155
MiRNA-155 was first identified in the LPS-induced ALI model by Jesper Worm [Citation47], and later studies showed that pre-treatment with antisense oligonucleotides could inhibit its expression [Citation49]. Several studies have reported that miRNA-155 is upregulated in the LPS-induced ALI model and that inhibiting its expression can produce anti-inflammatory effects by suppressing the release of pro-inflammatory factors [Citation87–89], including interleukin-6, interleukin-8, and tumor necrosis factor- α [Citation48,Citation90]. This can also affect the expression of heat shock proteins, such as heat shock protein-10, in endothelial cells and exert anti-inflammatory effects, playing an important role in sepsis-induced lung injury [Citation91]. Additionally, Kangfeng Jiang. et al [Citation92]found that miR-155 is found to promote M1 macrophage activation, exacerbating lung inflammation and tissue damage in macrophages. Specifically, miR-155 targets and down-regulates C/EBPβ, which leads to increased activation of M1 response [Citation49,Citation93]. Inhibition of miRNA-155 expression has been shown to reduce inflammation and intestinal barrier dysfunction by inactivating NF-κB signaling during sepsis [Citation94].
Moreover, miRNA-155 can inhibit the activation of the JAK/STAT signaling pathway [Citation95], reduce the levels of transaminase and urea nitrogen, and delay the damage to the liver, kidney, and other organs [Citation96]. By increasing the proportion of T-lymphocytes, miRNA-155 also plays a critical role in the immune system and immune response, accelerating the recovery process of ALI [Citation49,Citation97]. Additionally, it has been suggested that miRNA-155 can serve as a predictor of the prognosis of lung injury [Citation98]. Thus, inhibiting miRNA-155 expression can play a positive role in the treatment of ALI.
3.5 MiRNA-135a
During ALI, miRNA-135a expression is significantly upregulated. Inhibition of miRNA-135a expression enhances the expression of the anti-inflammatory factor BCL-2 and ameliorated LPS-induced lung injury [Citation5]. miRNA-135a is involved in inflammation, oxidative stress, and apoptosis by reducing the levels of pro-inflammatory factors, reactive oxygen species, and pro-apoptotic protein factors, thus maintaining the integrity of the endothelial barrier function and protecting vascular endothelial cells from ventilator-induced lung injury [Citation50].
Despite the promising results, the current number of studies on miRNA-135a is limited, and further research is necessary to demonstrate its practical role. Increasing the variety of miRNA types and tissues studied, and combining in vivo and in vitro research, while considering the possible synergistic or antagonistic effects of various miRNAs, may overcome some of the limitations of existing research. In-depth studies of the relevant mechanisms and therapeutic targets using both in vivo and in vitro models are needed to achieve unified and positive results, and to fully realize the clinical potential of miRNA-135a.
3.6 MiRNA-23a and miRNA-23b
The expression of miRNA-23a in ALI models remains controversial. While database results and quantitative fluorescence PCR detection have revealed that miRNA-23a is down-regulated in ALI models with high confidence, other studies have reported that miRNA-23a is upregulated during ALI and can improve ALI symptoms by promoting a reduction in apoptosis, affecting the cell cycle [Citation99], and elevating macrophage polarization to M1 via regulation of polo-like kinase 1(PLK1) in vivo and in vitro models [Citation100].
In contrast, miRNA-23b expression was found to be downregulated in A549 and vascular endothelial cells, which promoted S. pneumoniae-induced apoptosis and inflammatory responses [Citation51,Citation101]. Inhibition of miRNA-23b expression in cardiomyocytes has been shown to alleviate the progression of myocardial fibrosis in late sepsis, alleviate the progression of myocardial fibrosis in late sepsis, alleviate myocardial dysfunction, and improve survival rates [Citation102].
Although miRNA-23a and miRNA-23b belong to different clusters and have distinct sites, miRNA-23a and miRNA23b are often studied as joint-related factors, such as in non-small cell lung cancer and osteoarthritis. Therefore, results of miRNA-23a and miRNA-23b expression are considered more reliable when studied together.
3.7 MiRNA-21
miRNA-21 was first identified as an oncogenic RNA highly expressed in cancerous tissues. However, recent studies have revealed that miR-21 is also involved in the development of multiple inflammatory conditions. Inhibition of miRNA-21 expression has been shown to promote the recovery of motor function and inhibit the inflammatory response by blocking the IL-6 R/JAK/STAT pathway, facilitating the recovery and reconstruction of spinal cord nerves after spinal cord injury [Citation52]. Inhibition of the JAK/STAT signaling pathway, thereby alleviating the release of tumor necrosis factor- α in acute pancreatitis and controlling the inflammatory response [Citation103,Citation104]. miRNA-21 has also been reported to improve sepsis survival by specific inhibitory effects at appropriate concentrations without causing obvious organ dysfunction and liver injury [Citation105].
In ALI, most studies have found that miRNA-21 is upregulated, by inhibiting the TLR-4/NF-κB signaling pathway can help alleviate inflammation, reinforcing the first-line defense role of alveolar macrophages in lung tissue [Citation1]. Moreover, miRNA-21 may reduce lung tissue damage by inhibiting the target gene PTEN, improving the inflammatory response and oxidative stress in septic mice [Citation106]. However, simultaneous inhibition of PGE2 and miRNA-21 induces macrophage M2-type polarization via inhibiting STAT-3 expression [Citation53]. It has also been shown that miRNA-21 and its downstream signaling pathway can inhibit sodium ion channels in alveolar epithelial cells and aggravate pulmonary edema caused by sepsis-induced ALI [Citation107]. In addition, miRNA-21 plays an important protective role in chronic lung injuries such as radiation lung injury, as reported by Pengtao Bao et al [Citation108]. Animal experiments have also further confirmed the protective effect of miRNA-21 overexpression. However, unlike in ALI, miRNA-21 inhibits M1 polarization of alveolar macrophages in the chronic phase.
It is evident that miRNA-21 can have different roles in lung injury depending on the stage of the injury, and the regulation of macrophage polarization can also vary. Moreover, the protective effect of miRNA-21 on lung injury depends upon the balance between anti-inflammatory and pro-inflammatory pathways. Understanding the mechanisms influencing this balance will be a major research focus in the future, and further research is required to clarify the role of miRNA-21 in lung injury.
3.8 MiRNA-15a and miRNA-16
MiRNA-15a and miRNA-16 have emerged as biomarkers for sepsis and systemic inflammatory response syndromes, and appear to be superior to inflammatory factors such as procalcitonin, C reactive protein, and interleukin-6, of which the discriminatory advantage of miRNA-15a is more pronounced [Citation109].
In neonatal sepsis, upregulated expression of miRNA-15a/miRNA-16 can inhibit the inflammatory response [Citation39,Citation54], as demonstrated in a mouse model [Citation110]. MiRNA-15a/miRNA-16 could bear potential as a marker for the diagnostic and prognostic outcome of neonatal sepsis, but whether it can be a biomarker for adult sepsis as well as its role in inducing acute lung injury remains to be verified. Moreover, miRNA-16 can promote the polarization of M2 macrophages and reduce lung damage in mice with sepsis via suppressing TLR4 [Citation55]. Additionally, the mechanism by which the host allows the harmful upregulation of miRNA levels deserves further study, as shown by the conflicting results reported in Moon et al [Citation111].
3.9 MiRNA-138-5p
miRNA-138-5p is a well-studied tumor suppressor that inhibits the proliferation of various cancer cells [Citation112,Citation113] and plays an important role in inflammation. Recent studies have demonstrated that miRNA-138-5p improved LPS-induced hippocampal neuronal inflammation in rats by inhibiting NLRP3/Caspase1 activation [Citation114].
Recent studies have found that miRNA-138-5p is significantly downregulated in patients with sepsis-induced ARDS and that its overexpression can promote the production of M2 macrophages while inhibiting M1 macrophage polarization, thereby improving acute lung injury [Citation56]. However, these findings are based on the regulation of circular RNAs(circRNAs), which along with lncRNAs, are a part of a growing research area focused on circular networks based on circRNA/miRNA/mRNA. Further research is needed to identify the upstream target of miRNA-138-5p and explore its full biological potential. The limited number of studies on miRNA-138-5p and macrophage function in ALI reduces its current credibility, but it lays a foundation for further research in this area.
3.10 MiRNA-27a
miRNA-27a expression is elevated in patients with sepsis and serves as a potential biomarker for diagnosis and prognosis in patients with sepsis [Citation115]. In addition, miRNA-27a alleviates lung tissue damage by inhibiting TLR4/MyD88/NF-κB signaling pathway, which subsequently inhibits lung tissue inflammation and apoptosis [Citation57]. In vitro and in vitro experiments as well as studies on macrophage function have shown that miRNA-27a-3p expression is elevated in LPS-induced acute lung injury, and promotes M2 polarization of macrophages to further relieve lung tissue damage [Citation58].
Based on these studies, miRNA-27a is upregulated in ALI and can reduce ALI-associated inflammation and lung tissue damage by affecting macrophage M2 polarization and downstream inflammatory pathways. However, further studies are required to understand the specific role of miRNA-27a in macrophage polarization.
3.11 MiRNA-9-5p and miRNA-30d-5p
Studies of miR-9-5p in lung tissues have mainly focused on its role in promoting the progression of lung adenocarcinoma [Citation116]. However, recent research has highlighted its involvement in acute lung injury, where its expression was found to be significantly increased in both ALI model mice and patients. Moreover, miR-9-5p deficiency was shown to alleviate LPS-induced inflammatory infiltration in lung tissue by promoting the polarization of alveolar macrophages to the M2 phenotype [Citation59]. Despite the limited number of studies on miR-9-5p in acute lung injury, these findings offer valuable insights into the specific mechanisms underlying macrophage polarization.
Another important microRNA that affects macrophage polarization is miR-30d-5p. Accumulating evidence has revealed its role in the development and progression of different cancer types. For example, miR-30d-5p targeting suppresses the cellular activity of non-small cell lung cancer cancer [Citation117]and is involved in the progression of esophageal squamous cell carcinoma [Citation118]. Moreover, miR-30d-5p is considered a potential critical tumor biomarker. Recently, it was found that exosome-delivered miR-30d-5p promotes lung inflammation by targeting SOCS-1 and SIRT 1 to enhance M1 macrophage polarization and induces macrophage pyroptosis [Citation60]. In contrast, Li W.et al [Citation61] found that the expression of miR-30d-5p was decreased in lung tissues of mice with ALI, and its overexpression could improve lung inflammation, oxidative stress, and LPS-induced dysfunction via activation of AMPK α. The results of these two studies are contradictory. However, the number of studies on miR-30d-5p in lung injury is limited, and further research is required to establish the credibility of these findings.
3.12 MiRNA-33 and miRNA-34a
Recent studies have revealed that miRNA-33 and miRNA-34a are upregulated in ALI, contributing to the development of lung inflammation and pathological damage. MiRNA-33 has been shown to play important roles in inflammation [Citation119], metabolism [Citation120], and mitochondrial function. Inflammatory macrophage polarization mediated by NLRP3 has been identified as a key function of miRNA-33 [Citation121]. Previous research has shown that Er miao San (EMS) downregulates miRNA-33 expression in rheumatoid arthritis and promotes M1-type macrophage polarization via NLRP3 [Citation122,Citation123]. Similar results were observed in ALI, where miR-33 was found to be highly expressed in M1 type macrophages, and in vivo experiments demonstrated that miR-33 promotes M1 type polarization by inhibiting MEF 2 in alveolar macrophages [Citation62]. Similarly, miR-34a expression was found to be elevated in mice with acute LPS-induced lung injury. Inhibition of miR-34a resulted in significant amelioration of lung histopathological changes and promoted M2 type macrophage polarization [Citation63].
Despite the promising findings, few studies have been conducted on miRNA-33 and miRNA-34a, and the limited sample size also reduces the confidence of the study. Nonetheless, these findings have updated our understanding of miRNA-33 and miRNA-34a in the context of ALI and provided insight into the disease regulatory mechanisms that drive its progression.
3.13 MiRNA-451
The expression of miRNA-451 is increased in burn-induced ALI, but it is known to increase endothelial cell permeability and suppresses angiogenesis [Citation124]. Interestingly, a study has found that miRNA-451 is upregulated in exosomes of human umbilical cord MSCs [Citation125] and can alleviate burn-induced acute lung injury by regulating macrophage M2 polarization via the MIF-PI3K-AKT signaling pathway [Citation64]. These findings appear to contradict each other, as the former focuses on the effects of miRNA-451 on nonimmune cells while the latter explores the specific mechanism of immune cell response to acute lung injury.
Similarly, several other miRNAs are involved in macrophage polarization in acute lung injury. For example, miR-429 promotes the M1 phenotype by inhibiting the DUSP-1 protein, which is a negative regulator of p38 MAPK signaling, leading to the activation of inflammatory response and M1 polarization [Citation65]. MiR-127 also enhances M1 type polarization and promotes the release of pro-inflammatory cytokines by inhibiting BCL-6/Dusp-1 [Citation66]. Overall, microRNAs are either upregulated or downregulated in lung injury, regulated through different mechanisms in macrophage polarization and inflammation, which can either aggravate or alleviate ALI. Thus, up-regulated or downregulated microRNAs can become therapeutic targets for ALI. Furthermore, various interactions among microRNAs constitute a complex closed loop, which jointly regulates the occurrence and development of ALI.
4 MicroRnas-The treatment targets for acute lung injury
As previously mentioned, microRNAs play an essential role in the development of ALI, regulating the disease prognosis through their upregulation or downregulation. Numerous studies have shown that microRNAs modulate ALI progression by regulating apoptosis, inflammatory response, and oxidative stress. Differential expression of miRNAs also highlights their potential as therapeutic targets for ALI. Additionally, overexpression or knockdown of miRNAs have been demonstrated to ameliorate acute lung injury through various mechanisms. In this review, we summarize the current approaches for microRNA substitution, including inhibitory therapy, and in vitro and vivo delivery of microRNA, to either enhance or suppress downstream target gene expression and alleviate ALI ().
Figure 3. Schematic diagram of miRNAs regulation and therapeutic targets in ALI. With respect to miRNA delivery, there are four commonly used delivery methods to transfer miRNA modifications into cells. The level of miRNA can be restored by modification of miRNA. miRNA binds to target mRNA and then regulates its level, thus participating in pathological processes such as macrophage polarization, inflammation and apoptosis. In conclusion, miRNA plays an integral role in acute lung injury. Created with BioRender.com.
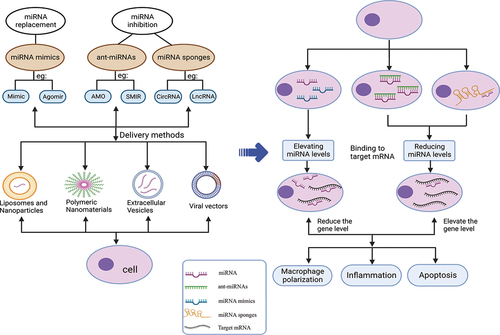
At present, the application of miRNAs in regulating disease progression mainly includes miRNA mimics, miRNA inhibitors, and miRNA sponges. miRNA mimics are double-stranded RNA molecules that mimic endogenous miRNA duplexes and are processed into single-stranded RNA upon entering the cells. They can restore the downregulated RNA levels and the biological function of specific RNA, thereby treating diseases. However, the transfection of RNA mimics may not always achieve the desired therapeutic effects due to the failure of the guide strand of the transfected miRNA mimic to integrate into the RNA-induced silencing complex [Citation9]. Moreover, miRNA mimics are unstable in cells and may be prone to degradation by endogenous nucleases. Therefore, chemically modified miRNA agomirs have been developed through numerous experimental reforms, and are more widely used in clinical practice. MiRNA inhibitors are more stable than mimics since they do not require cell treatment to exert their effects, and they also have relatively few transfection problems. The miRNA inhibitors mainly include anti-miRNA oligonucleotides (AMOs) and small molecular inhibitors of specific miRNAs (SMIRs). AMOs are perfectly complementary to the target miRNA, bind to the guide strand, and inactivate the miRNA by inhibiting its binding to the target mRNA or promoting its degradation by recruitment of RNase H [Citation126].
MiRNA sponges are an important tool for studying miRNA function in vivo and in vitro. They consist of repetitive miRNA antisense sequences acting as competitive inhibitors and separating the endogenous miRNA from its target to affect the biological function of the endogenous miRNA [Citation127]. Natural endogenous miRNA sponges, such as long non-coding RNAs (lncRNAs), circular RNAs (circRNAs), and mRNAs, bind to miRNAs and competitively isolate them from target cells. These are called competitive endogenous RNAs (ceRNAs) [Citation40].
Currently, there are various therapeutic vectors for delivering miRNAs, such as viruses, exosomes [Citation39], nanometer particles [Citation128], and other synthetic materials [Citation129–131]. Synthetic materials can be cation and condensed through electrostatic interaction with negative electric poly (nucleic acid). Compared with virus-based vectors, synthetic delivery systems have considerable advantages, such as the ability to control their molecular composition, simplified manufacturing, modification, and analysis, cargo size tolerance, and relatively low immunogenicity. However, their efficiency may be lower than that of virus-based vectors [Citation129]. In the future, more exploration of clinically efficient delivery methods of microRNAs may be needed to target tissues more efficiently.
5 Preclinical stage of microRnas therapy in ALI/ARDS
ALI is a challenging condition to treat, and currently, there are no effective radical measures available. Conservative treatment measures such as lung-protective ventilation, restricted fluid management, combined use of glucocorticoids, and extracorporeal membrane oxygenation [Citation132,Citation133] have been adopted, but long-term mechanical ventilation can lead to complications such as ventilator-associated pneumonia and pulmonary fibrosis.
To address this challenge, mesenchymal stromal cells (MSCs) have emerged as a research hotspot for the treatment of various diseases. These cells are found in various tissues, such as bone marrow, muscle, adipose tissue, dental tissue, synovial fluid, skin, and placenta [Citation134], and can be detected in all vascular tissues of the body, including the upper and lower respiratory tract of lung tissue [Citation135]. Additionally, they can induce macrophages to polarize to the M2 type, which indirectly exerts anti-inflammatory effects and further promotes lung tissue repair [Citation136–139]. Mechanistically, MSCs can reduce the expression of miR-193b-5p and improve sepsis-induced acute lung injury [Citation140]. Therefore, MSCs have good therapeutic prospects for acute lung injury, and MSCs can be used as target delivery cells to make miRNAs. This approach is free from the degradation of human nucleases, making it more efficient in targeting lung tissue. However, further clinical trials are required to validate this potential therapy.
6 Development and outlook
miRNA expression can be either up-regulated or down-regulated in the same model, and the effect of these changes on the host is still unknown. Furthermore, there may be antagonism or synergistic effects between miRNAs, and the difference between the leading and auxiliary effects of miRNAs cannot be ignored. More studies are needed to further summarize the complex interactions between miRNAs in different disease states and processes.
The interaction between miRNAs and macrophage polarization is particularly relevant in regulating the balance between anti-inflammatory and pro-inflammatory responses, and miRNAs are being increasingly recognized as having an important role in many diseases, including ALI. Because miRNA expression is tissue-specific, different disease states and different disease processes may have different effects, and macrophages of different tissue origins may exhibit different polarization responses to the same miRNA. Because diseases change dynamically over time and in different environments, macrophages may also exhibit different phenotypes, making the identification of the dominant polarized phenotypes their controlling miRNAs a complex challenge. Therefore, the dynamic monitoring of miRNA levels poses a great challenge to the research work.
ALI/ARDS is an acute and critical life-threatening illness in clinical work, often accompanied by secondary damage to distant organ tissues in addition to local tissue damage. As mentioned above, exploring miRNA regulatory pathways and finding suitable targets may help in effectively alleviating disease progression and improving patient survival. Macrophages as the first line of defense in immunity, its polarized form also determines the development process of the disease, so finding microRNAs to control macrophage activation will also achieve the purpose of treating the disease from the source. This is also the main aim of most microRNA studies. However, there are still many unanswered questions that must be addressed. For example, most studies focus on a single miRNA pathway and do not consider the interaction and leading role of each miRNA in disease occurrence. Additionally, the number of studies on miRNAs affecting macrophage polarization is relatively small, reducing the confidence of the study. Finally, differentially expressed miRNAs in animal models must be validated in patients with established ALI/ARDS for extrapolation in humans, making the development of miRNA therapy a significant challenge.
To address these limitations, future research should focus on identifying the key factors that affect macrophage polarization and identifying miRNAs that favor the polarization and dominant role of M2-type macrophages. Interventions to strengthen the effects of these miRNAs may help promote disease recovery. Additionally, research should explore whether miRNAs can be used to control the occurrence of complications in the treatment of local clinical symptoms. Further in-depth research is required to explore the clinical value of miRNAs in the future.
Disclosure statement
No potential conflict of interest was reported by the authors.
Additional information
Funding
References
- Zhu WD, Xu J, Zhang M, et al. MicroRNA‑21 inhibits lipopolysaccharide‑induced acute lung injury by targeting nuclear factor‑κB. Exp Ther Med. 2018;16(6):4616–4622. doi: 10.3892/etm.2018.6789
- Suo T, Chen G, Huang Y, et al. MiRNA-1246 suppresses acute lung injury-induced inflammation and apoptosis via the NF-κB and Wnt/β-catenin signal pathways. Biomed Pharmacother. 2018;108:783–791. doi: 10.1016/j.biopha.2018.09.046
- Fu L, Zhu P, Qi S, et al. MicroRNA-92a antagonism attenuates lipopolysaccharide (LPS)-induced pulmonary inflammation and injury in mice through suppressing the PTEN/AKT/NF-κB signaling pathway. Biomed Pharmacother. 2018;107:703–711. doi: 10.1016/j.biopha.2018.08.040
- Vaporidi K, Vergadi E, Kaniaris E, et al. Pulmonary microRNA profiling in a mouse model of ventilator-induced lung injury. Am J Physiol Lung Cell Mol Physiol. 2012;303(3):L199–207. doi: 10.1152/ajplung.00370.2011
- Zhao J, Li X, Zou M, et al. MiR-135a inhibition protects A549 cells from LPS-induced apoptosis by targeting Bcl-2. Biochem Biophys Res Commun. 2014;452(4):951–957. doi: 10.1016/j.bbrc.2014.09.025
- Xiao B, Liu Z, Li B, et al. Induction of microRNA-155 during Helicobacter pylori infection and its negative regulatory role in the inflammatory response. J Infect Dis. 2009;200(6):916–925. doi: 10.1086/605443
- Fang Y, Gao F, Hao J, et al. MicroRNA-1246 mediates lipopolysaccharide-induced pulmonary endothelial cell apoptosis and acute lung injury by targeting angiotensin-converting enzyme 2. Am J Transl Res. 2017;9(3):1287–1296.
- McCoy CE. The role of miRnas in cytokine signaling. Front Biosci. 2011;16(1):2161–2171. doi: 10.2741/3845. Landmark Ed.
- Lu TX, Rothenberg ME. MicroRNA. MicroRna J Allergy Clin Immunol. 2018;141(4):1202–1207. doi: 10.1016/j.jaci.2017.08.034
- Kushimoto S, Taira Y, Kitazawa Y, et al. The clinical usefulness of extravascular lung water and pulmonary vascular permeability index to diagnose and characterize pulmonary edema: a prospective multicenter study on the quantitative differential diagnostic definition for acute lung injury/acute respiratory distress syndrome. Crit Care. 2012;16(6):R232. doi: 10.1186/cc11898
- Han S, Mallampalli RK. The acute respiratory distress syndrome: from mechanism to translation. J Immunol. 2015;194(3):855–860. doi: 10.4049/jimmunol.1402513
- Qin S, Wang H, Liu G, et al. miR‑21‑5p ameliorates hyperoxic acute lung injury and decreases apoptosis of AEC II cells via PTEN/AKT signaling in rats. Mol Med Rep. 2019;20(6):4953–4962. doi: 10.3892/mmr.2019.10779
- Kumar V. Pulmonary innate immune response determines the outcome of inflammation during pneumonia and sepsis-associated acute lung injury. Front Immunol. 2020;11:1722. doi: 10.3389/fimmu.2020.01722
- Huang X, Xiu H, Zhang S, et al. The role of macrophages in the pathogenesis of ALI/ARDS. Mediators Inflamm. 2018;2018:1–8. doi: 10.1155/2018/1264913
- Epelman S, Lavine KJ, Randolph GJ. Origin and functions of tissue macrophages. Immunity. 2014;41(1):21–35. doi: 10.1016/j.immuni.2014.06.013
- Cheng P, Li S, Chen H. Macrophages in lung injury, repair, and fibrosis. Cells. 2021;10(2):436. doi: 10.3390/cells10020436
- Hao NB, Lu MH, Fan YH, et al. Macrophages in tumor microenvironments and the progression of tumors.Clin Dev Immunol 2012;2012:1–11. doi:10.1155/2012/948098
- Mantovani A, Biswas S, Galdiero M, et al. Macrophage plasticity and polarization in tissue repair and remodelling. J Pathol. 2013;229(2):176–185. doi: 10.1002/path.4133
- Artyomov MN, Sergushichev A, Schilling JD. Integrating immunometabolism and macrophage diversity. Semin Immunol. 2016;28(5):417–424. doi: 10.1016/j.smim.2016.10.004
- Takeuchi O, Akira S. Pattern recognition receptors and inflammation. Cell. 2010;140(6):805–820. doi: 10.1016/j.cell.2010.01.022
- Zhang Q, Raoof M, Chen Y, et al. Circulating mitochondrial DAMPs cause inflammatory responses to injury. Nature. 2010;464(7285):104–107. doi: 10.1038/nature08780
- Ray NB, Durairaj L, Chen B, et al. Dynamic regulation of cardiolipin by the lipid pump Atp8b1 determines the severity of lung injury in experimental pneumonia. Nat Med. 2010;16(10):1120–1127. doi: 10.1038/nm.2213
- Simmons JD, Lee YL, Mulekar S, et al. Elevated levels of plasma mitochondrial DNA DAMPs are linked to clinical outcome in severely injured human subjects. Ann Surg. 2013;258(4):591–596. discussion 596-8. doi: 10.1097/SLA.0b013e3182a4ea46
- Douda DN, Jackson R, Grasemann H, et al. Innate immune collectin surfactant protein D simultaneously binds both neutrophil extracellular traps and carbohydrate ligands and promotes bacterial trapping. J Immunol. 2011;187(4):1856–1865. doi: 10.4049/jimmunol.1004201
- Butt Y, Kurdowska A, Allen TC. Acute lung injury: a clinical and molecular review. Arch Pathol Lab Med. 2016;140(4):345–350. doi: 10.5858/arpa.2015-0519-RA
- Kessenbrock K, Plaks V, Werb Z. Matrix metalloproteinases: regulators of the tumor microenvironment. Cell. 2010;141(1):52–67. doi: 10.1016/j.cell.2010.03.015
- Sobierajska K, Ciszewski WM, Sacewicz-Hofman I, et al. Endothelial cells in the tumor microenvironment. Adv Exp Med Biol. 2020;1234:71–86. doi: 10.1007/978-3-030-37184-5_6
- Pérez L, Muñoz-Durango N, Riedel CA, et al. Endothelial-to-mesenchymal transition: cytokine-mediated pathways that determine endothelial fibrosis under inflammatory conditions. Cytokine Growth Factor Rev. 2017;33:41–54. doi: 10.1016/j.cytogfr.2016.09.002
- Hu HH, Chen DQ, Wang YN, et al. New insights into TGF-β/Smad signaling in tissue fibrosis. Chem Biol Interact. 2018;292:76–83. doi: 10.1016/j.cbi.2018.07.008
- Isaka Y. Targeting TGF-β signaling in kidney fibrosis. Int J Mol Sci. 2018;19(9):2532. doi: 10.3390/ijms19092532
- Mohr AM, Mott JL. Overview of microRNA biology. Semin Liver Dis. 2015;35(1):003–011. doi: 10.1055/s-0034-1397344
- Ludwig N, Leidinger P, Becker K, et al. Distribution of miRNA expression across human tissues. Nucleic Acids Res. 2016;44(8):3865–3877. doi: 10.1093/nar/gkw116
- Cai ZG, Zhang SM, Zhang Y, et al. MicroRNAs are dynamically regulated and play an important role in LPS-induced lung injury. Can J Physiol Pharmacol. 2012;90(1):37–43. doi: 10.1139/y11-095
- Meng F, Henson R, Wehbe–Janek H, et al. MicroRNA-21 regulates expression of the PTEN tumor suppressor gene in human hepatocellular cancer. Gastroenterology. 2007;133(2):647–658. doi: 10.1053/j.gastro.2007.05.022
- Pu QF, Liu GY, Wang HL, et al. MicroRNAs progress in the relationship with acute respiratory distress syndrome. Journal Of Zunyi Medical College. 2016;39(5):535–538+546. doi: 10.14169/j.cnki.zunyixuebao.2016.0124
- Cao YM, Li YC. MicroRNA—— Novel regulatory molecules of acute lung injury. Journal Of Shanghai Jiao Tong University. 2014;34(10): 1539–1542. Medical edition.
- Goodwin AJ, Guo C, Cook JA, et al. Plasma levels of microRNA are altered with the development of shock in human sepsis: an observational study. Crit Care. 2015;19(1):440. doi: 10.1186/s13054-015-1162-8
- Liu Y, Wang X, Li P, et al. Targeting MALAT1 and miRNA-181a-5p for the intervention of acute lung injury/acute respiratory distress syndrome. Respir Res. 2021;22(1):1. doi: 10.1186/s12931-020-01578-8
- Wang W, Lou C, Gao J, et al. LncRNA SNHG16 reverses the effects of miR-15a/16 on LPS-induced inflammatory pathway. Biomed Pharmacother. 2018;106:1661–1667. doi: 10.1016/j.biopha.2018.07.105
- Alkan AH, Akgül B. Endogenous miRNA sponges. Methods Mol Biol. 2022;2257:91–104.
- He N, Tan H, Deng X, et al. MiR-223-3p-loaded exosomes from bronchoalveolar lavage fluid promote alveolar macrophage autophagy and reduce acute lung injury by inhibiting the expression of STK39. Hum Cell. 2022;35(6):1736–1751. doi: 10.1007/s13577-022-00762-w
- Feng Z, Qi S, Zhang Y, et al. Ly6g+ neutrophil-derived miR-223 inhibits the NLRP3 inflammasome in mitochondrial DAMP-induced acute lung injury. Cell Death Dis. 2017;8(11):e3170. doi: 10.1038/cddis.2017.549
- Huang C, Liu XJ, Zhou Q, et al. MiR-146a modulates macrophage polarization by inhibiting notch1 pathway in RAW264.7 macrophages. Int Immunopharmacol. 2016;32:46–54. doi: 10.1016/j.intimp.2016.01.009
- Zeng Z, Gong H, Li Y, et al. Upregulation of miR-146a contributes to the suppression of inflammatory responses in LPS-induced acute lung injury. Exp Lung Res. 2013;39(7):275–282. doi: 10.3109/01902148.2013.808285
- Vergadi E, Vaporidi K, Theodorakis EE, et al. Akt2 deficiency protects from acute lung injury via alternative macrophage activation and miR-146a induction in mice. J Immunol. 2014;192(1):394–406. doi: 10.4049/jimmunol.1300959
- Li Y, Jiang QD, Mei ST, et al. Effects and action mechanism of miR-181a targeting SIRT 1 on sepsis-induced acute lung injury in mice. Journal Of Xi’an Jiaotong University. 2020;41(4):516–524. Medical Edition.
- Worm J, Stenvang J, Petri A, et al. Silencing of microRNA-155 in mice during acute inflammatory response leads to derepression of c/ebp Beta and down-regulation of G-CSF. Nucleic Acids Res. 2009;37(17):5784–5792. doi: 10.1093/nar/gkp577
- Zhang Y. Role of microRNA-155 in smoke inhalation-induced acute lung injury. Zhejiang University of Technology; 2020. doi: 10.27463/d.cnki.gzgyu.2020.001429
- Guo Z, Wen Z, Qin A, et al. Antisense oligonucleotide treatment enhances the recovery of acute lung injury through IL-10-secreting M2-like macrophage-induced expansion of CD4+ regulatory T cells. J Immunol. 2013;190(8):4337–4348. doi: 10.4049/jimmunol.1203233
- Yan X, Li W, Yang L, et al. MiR-135a protects vascular endothelial cells against ventilator-induced lung injury by inhibiting PHLPP2 to activate PI3K/Akt pathway. Cell Physiol Biochem. 2018;48(3):1245–1258. doi: 10.1159/000492010
- Wu M, Sun L, Zhu J, et al. MicroRNA-23b regulates the expression of inflammatory factors in vascular endothelial cells during sepsis. Exp Ther Med. 2015;9(4):1125–1132. doi: 10.3892/etm.2015.2224
- Ning SL, Zhu H, Shao J, et al. MiR-21 inhibitor improves locomotor function recovery by inhibiting IL-6R/JAK-STAT pathway-mediated inflammation after spinal cord injury in model of rat. Eur Rev Med Pharmacol Sci. 2019;23(2):433–440. doi: 10.26355/eurrev_201901_16852
- Wang Z, Brandt S, Medeiros A, et al. MicroRNA 21 is a homeostatic regulator of macrophage polarization and prevents prostaglandin E2-mediated M2 generation. PLoS One. 2015;10(2):e0115855. doi: 10.1371/journal.pone.0115855
- Wang X, Wang X, Liu X, et al. MiR-15a/16 are upregulated in the serum of neonatal sepsis patients and inhibit the LPS-induced inflammatory pathway. Int J Clin Exp Med. 2015;8(4):5683–5690.
- Tian J, Cui X, Sun J, et al. Exosomal microRNA-16-5p from adipose mesenchymal stem cells promotes TLR4-mediated M2 macrophage polarization in septic lung injury. Int Immunopharmacol. 2021;98:107835. doi:10.1016/j.intimp.2021.107835
- Zhao D, Wang C, Liu X, et al. CircN4bp1 facilitates sepsis-induced acute respiratory distress syndrome through mediating macrophage polarization via the miR-138-5p/EZH2 axis. Mediators Inflamm. 2021;2021:1–14. doi:10.1155/2021/7858746
- Ju M, Liu B, He H, et al. MicroRNA-27a alleviates LPS-induced acute lung injury in mice via inhibiting in?ammation and apoptosis through modulating TLR4/MyD88/NF-κB pathway. Cell Cycle. 2018;17(16):2001–2018. doi: 10.1080/15384101.2018.1509635
- Wang J, Huang R, Xu Q, et al. Mesenchymal stem cell-derived extracellular vesicles alleviate acute lung injury via transfer of miR-27a-3p. Crit Care Med. 2020;48(7):e599–e610. doi: 10.1097/CCM.0000000000004315
- Li H, Hu W, Lin Y, et al. MicroRNA-9-5p is involved in lipopolysaccharide-induced acute lung injury via the regulation of macrophage polarization. Int J Toxicol. 2023;42(2):156–164. doi: 10.1177/10915818221146446
- Jiao Y, Zhang T, Zhang C, et al. Exosomal miR-30d-5p of neutrophils induces M1 macrophage polarization and primes macrophage pyroptosis in sepsis-related acute lung injury. Crit Care. 2021;25(1):356. doi: 10.1186/s13054-021-03775-3
- Li W, Hou G, Lv J, et al. MicroRNA-30d-5p ameliorates lipopolysaccharide-induced acute lung injury via activating AMPKα. Immunopharmacol Immunotoxicol. 2021;43(4):431–442. doi: 10.1080/08923973.2021.1933517
- Sun Q, Xia Y, Qin H, et al. MEF2 intervened LPS-induced acute lung injury by binding to KLF2 promoter and modulating macrophage phenotype. Int Immunopharmacol. 2022;108:108873. doi:10.1016/j.intimp.2022.108873
- Khan MJ, Singh P, Dohare R, et al. Inhibition of miRNA-34a promotes M2 macrophage polarization and improves LPS-Induced lung injury by targeting Klf4. Genes (Basel). 2020;11(9):966. doi: 10.3390/genes11090966
- Liu J, Xing F, Fu Q, et al. Huc-MSCs exosomal miR-451 alleviated acute lung injury by modulating macrophage M2 polarization via regulating MIF-PI3K-AKT signaling pathway. Environ Toxicol. 2022;37(12):2819–2831. doi: 10.1002/tox.23639
- Xiao J, Tang J, Chen Q, et al. MiR-429 regulates alveolar macrophage inflammatory cytokine production and is involved in LPS-induced acute lung injury. Biochem J. 2015;471(2):281–291. doi: 10.1042/BJ20131510
- Ying H, Kang Y, Zhang H, et al. MiR-127 modulates macrophage polarization and promotes lung inflammation and injury by activating the JNK pathway. J Immunol. 2015;194(3):1239–1251. doi: 10.4049/jimmunol.1402088
- Ward JR, Heath PR, Catto JW, et al. Regulation of neutrophil senescence by microRnas. PLoS One. 2011;6(1):e15810. doi: 10.1371/journal.pone.0015810
- Wang X, He Y, Mackowiak B, et al. MicroRNAs as regulators, biomarkers and therapeutic targets in liver diseases. Gut. 2021;70(4):784–795. doi: 10.1136/gutjnl-2020-322526
- Yuan X, Berg N, Lee JW, et al. MicroRNA miR-223 as regulator of innate immunity. J Leukocyte Biol. 2018;104(3):515–524. doi: 10.1002/JLB.3MR0218-079R
- Johnnidis JB, Harris MH, Wheeler RT, et al. Regulation of progenitor cell proliferation and granulocyte function by microRNA-223. Nature. 2008;451(7182):1125–1129. doi: 10.1038/nature06607
- He Y, Rodrigues RM, Wang X, et al. Neutrophil-to-hepatocyte communication via LDLR-dependent miR-223-enriched extracellular vesicle transfer ameliorates nonalcoholic steatohepatitis. J Clin Invest. 2021;131(3). doi: 10.1172/JCI141513
- Fan HB, Liu YJ, Wang L, et al. MiR-142-3p acts as an essential modulator of neutrophil development in zebrafish. Blood. 2014;124(8):1320–1330. doi: 10.1182/blood-2013-12-545012
- Zhang D, Lee H, Wang X, et al. A potential role of microvesicle-containing miR-223/142 in lung inflammation. Thorax. 2019;74(9):865–874. doi: 10.1136/thoraxjnl-2018-212994
- Roos J, Enlund E, Funcke JB, et al. MiR-146a-mediated suppression of the inflammatory response in human adipocytes. Sci Rep. 2016;6(1):38339. doi: 10.1038/srep38339
- Brudecki L, Ferguson DA, McCall CE, et al. MicroRNA-146a and RBM4 form a negative feed-forward loop that disrupts cytokine mRNA translation following TLR4 responses in human THP-1 monocytes. Immunol Cell Biol. 2013;91(8):532–540. doi: 10.1038/icb.2013.37
- Meng JB, Sun LP, Wa YL, et al. Mechanism of action of miRNA-146a in acute lung injury induced by sepsis. The PLA Medical Journal. 2017;42(3):207–210.
- Zeng ZG. Role and mechanism of miR-146a in lipopolysaccharide-induced acute lung injury in rats. Nanchang University; 2012. doi: 10.7666/d.Y2427628
- Funahashi Y, Kato N, Masuda T, et al. MiR-146a targeted to splenic macrophages prevents sepsis-induced multiple organ injury. Lab Invest. 2019;99(8):1130–1142. doi: 10.1038/s41374-019-0190-4
- Juriaiti Y, Abudureheman Y, Yang YD, et al. Expression of miR-146a in sepsis-associated acute lung injury and its early diagnostic value. Practical Shock Journal (Chinese And English). 2020;4(4):215–218.
- Wang JF, Yu ML, Yu G, et al. Serum miR-146a and miR-223 as potential new biomarkers for sepsis. Biochem Biophys Res Commun. 2010;394(1):184–188. doi: 10.1016/j.bbrc.2010.02.145
- Wu Y, Li C, He Y, et al. [Relationship between expression of microRNA and inflammatory cytokines plasma level in pediatric patients with sepsis]. Zhonghua Er Ke Za Zhi. 2014;52(1):28–33.
- Aziz F. The emerging role of miR-223 as novel potential diagnostic and therapeutic target for inflammatory disorders. Cell Immunol. 2016;303:1–6. doi: 10.1016/j.cellimm.2016.04.003
- Zhu J, Wang FL, Wang HB, et al. TNF-α mRNA is negatively regulated by microRNA-181a-5p in maturation of dendritic cells induced by high mobility group box-1 protein. Sci Rep. 2017;7(1):12239. doi: 10.1038/s41598-017-12492-3
- Li W, Qiu X, Jiang H, et al. Downregulation of miR-181a protects mice from LPS-induced acute lung injury by targeting Bcl-2. Biomed Pharmacother. 2016;84:1375–1382. doi: 10.1016/j.biopha.2016.10.065
- Jiang K, Guo S, Zhang T, et al. Downregulation of TLR4 by miR-181a provides negative feedback regulation to lipopolysaccharide-induced inflammation. Front Pharmacol. 2018;9:142. doi: 10.3389/fphar.2018.00142
- Zhao H, Guo Y, Sun Y, et al. MiR-181a/b-5p ameliorates inflammatory response in monocrotaline-induced pulmonary arterial hypertension by targeting endocan. J Cell Physiol. 2020;235(5):4422–4433. doi: 10.1002/jcp.29318
- Wang ZH, Liang YB, Tang H, et al. Dexamethasone down-regulates the expression of microRNA-155 in the livers of septic mice. PLoS One. 2013;8(11):e80547. doi: 10.1371/journal.pone.0080547
- Nie C. Experimental study that upregulation of autophagy induced by miR-155 protects the lung of septic rat. Nanchang University; 2014. doi: 10.3969/j.issn.2095-4344.1438
- Li HZ, Yang K, Liu YW, et al. Effect of miR-155 on acute lung injury in burned rats: changes of nuclear factor-kappa B pathway. Chin J Tissue Eng Res. 2020;24(2):204–208.
- Wang Y. Protective effect of miR-155 on acute lung injury induced by sepsis and its mechanism in mice. Nanchang University; 2016. doi: 10.7666/d.D01016628
- Pfeiffer D, Roßmanith E, Lang I, et al. MiR-146a, miR-146b, and miR-155 increase expression of IL-6 and IL-8 and support HSP10 in an in vitro sepsis model. PLoS One. 2017;12(6):e0179850. doi: 10.1371/journal.pone.0179850
- Jiang K, Yang J, Guo S, et al. Peripheral circulating exosome-mediated delivery of miR-155 as a novel mechanism for acute lung inflammation. Mol Ther. 2019;27(10):1758–1771. doi: 10.1016/j.ymthe.2019.07.003
- Rajasekaran S, Pattarayan D, Rajaguru P, et al. MicroRNA regulation of acute lung injury and acute respiratory distress syndrome. J Cell Physiol. 2016;231(10):2097–2106. doi: 10.1002/jcp.25316
- Cao YY, Wang Z, Wang ZH, et al. Inhibition of miR-155 alleviates sepsis-induced inflammation and intestinal barrier dysfunction by inactivating NF-κB signaling. Int Immunopharmacol. 2021;90:107218. doi: 10.1016/j.intimp.2020.107218
- Lv X, Zhang Y, Cui Y, et al. Inhibition of microRNA‑155 relieves sepsis‑induced liver injury through inactivating the JAK/STAT pathway. Mol Med Rep. 2015;12(4):6013–6018. doi: 10.3892/mmr.2015.4188
- Ma F, Liu F, Ding L, et al. Anti-inflammatory effects of curcumin are associated with down regulating microRNA-155 in LPS-treated macrophages and mice. Pharm Biol. 2017;55(1):1263–1273. doi: 10.1080/13880209.2017.1297838
- Wang P, Hou J, Lin L, et al. Inducible microRNA-155 feedback promotes type I IFN signaling in antiviral innate immunity by targeting suppressor of cytokine signaling 1. J Immunol. 2010;185(10):6226–6233. doi: 10.4049/jimmunol.1000491
- Hao JX, Xu JX, Liang Y, et al. Expression of miR-127 and miR-155 in patients with acute lung injury and its effect on prognosis. Clinical Emergency Journal. 2020;21(8):654–658.
- Yang J, Mao M, Zhen YY. MiRNA-23a has effects to improve lung injury induced by sepsis in vitro and vivo study. Biomed Pharmacother. 2018;107:81–89. doi: 10.1016/j.biopha.2018.07.097
- Jiang T, Sun L, Zhu J, et al. MicroRNA-23a-3p promotes macrophage M1 polarization and aggravates lipopolysaccharide-induced acute lung injury by regulating PLK1/STAT1/STAT3 signalling. Int J Exp Pathol. 2022;103(5):198–207. doi: 10.1111/iep.12445
- Dai CT, Zhang SF, Huang YJ, et al. Down-regulation of miR-23b-3p protects vascular endothelial cell from injury induced by Streptococcus pneumoniae through targeting PALM3. Chinese Journal Of Laboratory Diagnosis. 2020;24(6):996–1002.
- Zhang H, Caudle Y, Shaikh A, et al. Inhibition of microRNA-23b prevents polymicrobial sepsis-induced cardiac dysfunction by modulating TGIF1 and PTEN. Biomed Pharmacother. 2018;103:869–878. doi: 10.1016/j.biopha.2018.04.092
- Wang G, Zhang J, Dui D, et al. High mobility group box 1 induces the activation of the Janus kinase 2 and signal transducer and activator of transcription 3 (JAK2/STAT3) signaling pathway in pancreatic acinar cells in rats, while AG490 and rapamycin inhibit their activation. Bosn J Of Basic Med Sci. 2016;16(4):307–312. doi: 10.17305/bjbms.2016.1442
- Liu H, Yao YM, Yu Y, et al. Role of Janus kinase/signal transducer and activator of transcription pathway in regulation of expression and inflammation-promoting activity of high mobility group box protein 1 in rat peritoneal macrophages. Shock. 2007;27(1):55–60. doi: 10.1097/01.shk.0000233197.40989.31
- Peña G, Cai B, Deitch EA, et al. JAK2 inhibition prevents innate immune responses and rescues animals from sepsis. J Mol Med (Berl). 2010;88(8):851–859. doi: 10.1007/s00109-010-0628-z
- Ge C, Liu JX, Fu Y, et al. Protective effect of microRNA-21 on acute lung injury mice induced by sepsis and its relationship with PETN. Shandong Med J. 2022;62(2):30–34.
- Qi W, Li H, Cai XH, et al. Lipoxin A4 activates alveolar epithelial sodium channel gamma via the microRNA-21/PTEN/AKT pathway in lipopolysaccharide-induced inflammatory lung injury. Lab Invest. 2015;95(11):1258–1268. doi: 10.1038/labinvest.2015.109
- Bao P, Zhao W, Mou M, et al. MicroRNA-21 mediates bone marrow mesenchymal stem cells protection of radiation-induced lung injury during the acute phase by regulating polarization of alveolar macrophages. Transl Cancer Res. 2020;9(1):231–239. doi: 10.21037/tcr.2019.12.77
- Wang H, Zhang P, Chen W, et al. Evidence for serum miR-15a and miR-16 levels as biomarkers that distinguish sepsis from systemic inflammatory response syndrome in human subjects. Clin Chem Lab Med. 2012;50(8):1423–1428. doi: 10.1515/cclm-2011-0826
- Cai ZG, Zhang SM, Zhang H, et al. Expression and function of miR-16 in lipopolysaccharide-induced acute lung injury. Journal Of Nanchang University(medical Sciences). 2011;51(2):1–5.
- Moon HG, Yang J, Zheng Y, et al. MiR-15a/16 regulates macrophage phagocytosis after bacterial infection. J Immunol. 2014;193(9):4558–4567. doi: 10.4049/jimmunol.1401372
- Rasoolnezhad M, Safaralizadeh R, Hosseinpourfeizi MA, et al. MiRNA-138-5p: a strong tumor suppressor targeting PD-L-1 inhibits proliferation and motility of breast cancer cells and induces apoptosis. Eur J Pharmacol. 2021;896:173933. doi: 10.1016/j.ejphar.2021.173933
- Zhao L, Yu H, Yi S, et al. The tumor suppressor miR-138-5p targets PD-L1 in colorectal cancer. Oncotarget. 2016;7(29):45370–45384. doi: 10.18632/oncotarget.9659
- Feng X, Hu J, Zhan F, et al. MicroRNA-138-5p regulates hippocampal neuroinflammation and cognitive impairment by NLRP3/Caspase-1 signaling pathway in rats. J Inflamm Res. 2021;14:1125–1143. doi: 10.2147/JIR.S304461
- Ou Y, An R, Wang H, et al. Oxidative stress-related circulating miRNA-27a is a potential biomarker for diagnosis and prognosis in patients with sepsis. BMC Immunol. 2022;23(1):14. doi: 10.1186/s12865-022-00489-1
- Lu Y, Zheng W, Rao X, et al. MicroRNA-9-5p facilitates lung adenocarcinoma cell malignant progression via targeting STARD13. Biochem Genet. 2022;60(6):1865–1880. doi: 10.1007/s10528-022-10191-x
- Kranjc T, Milojević M, Kocjan T, et al. Plasma levels of miR-30d-5p are decreased in regularly exercising postmenopausal women. Menopause. 2020;27(3):319–325. doi: 10.1097/GME.0000000000001454
- Zhu Y, Liu J, Fan L, et al. Serum expression and significance of MicroRNA-30d-5p in esophageal squamous cell carcinoma. Int J Clin Exp Pathol. 2017;10(8):8677–8685.
- Lai L, Azzam KM, Lin WC, et al. MicroRNA-33 regulates the innate immune response via ATP binding cassette transporter-mediated remodeling of membrane microdomains. J Biol Chem. 2016;291(37):19651–19660. doi: 10.1074/jbc.M116.723056
- Näär AM. MiR-33: a metabolic conundrum. Trends Endocrinol Metab. 2018;29(10):667–668. doi: 10.1016/j.tem.2018.04.004
- Xie Q, Wei M, Zhang B, et al. MicroRNA‑33 regulates the NLRP3 inflammasome signaling pathway in macrophages. Mol Med Rep. 2018;17(2):3318–3327. doi: 10.3892/mmr.2017.8224
- Liu M, Wang J, Chen S, et al. Exploring the effect of Er miao San-containing serum on macrophage polarization through miR-33/NLRP3 pathway. J Ethnopharmacol. 2023;307:116178. doi:10.1016/j.jep.2023.116178
- Liu M, Meng X, Xuan Z, et al. Effect of Er Miao San on peritoneal macrophage polarisation through the miRNA-33/NLRP3 signalling pathway in a rat model of adjuvant arthritis. Pharm Biol. 2022;60(1):846–853. doi: 10.1080/13880209.2022.2066700
- Zhou J, Lian H, Xu G, et al. MicroRNA-451 increases vascular permeability and suppresses angiogenesis in pulmonary burn injury in a rat model. Adv Clin Exp Med. 2020;29(11):1241–1248. doi: 10.17219/acem/126299
- Liu JS, Du J, Cheng X, et al. Exosomal miR-451 from human umbilical cord mesenchymal stem cells attenuates burn-induced acute lung injury. J Chin Med Assoc. 2019;82(12):895–901. doi: 10.1097/JCMA.0000000000000189
- Simonson B, Das S. MicroRNA therapeutics: the next magic bullet? Mini Rev Med Chem. 2015;15(6):467–474. doi: 10.2174/1389557515666150324123208
- Kluiver J, Gibcus JH, Hettinga C, et al. Rapid generation of microRNA sponges for microRNA inhibition. PLoS One. 2012;7(1):e29275. doi: 10.1371/journal.pone.0029275
- Lee SWL, Paoletti C, Campisi M, et al. MicroRNA delivery through nanoparticles. J Control Release. 2019;313:80–95. doi:10.1016/j.jconrel.2019.10.007
- Zhang Y, Wang Z, Gemeinhart RA. Progress in microRNA delivery. J Control Release. 2013;172(3):962–974. doi: 10.1016/j.jconrel.2013.09.015
- Misiak P, Markiewicz KH, Szymczuk D, et al. Polymeric drug delivery systems bearing cholesterol moieties: a review. Polymers. 2020;12(11):2620. doi: 10.3390/polym12112620
- Paunovska K, Loughrey D, Dahlman JE. Drug delivery systems for RNA therapeutics. Nat Rev Genet. 2022;23(5):265–280. doi: 10.1038/s41576-021-00439-4
- Lewis SR, Pritchard MW, Thomas CM, et al. Pharmacological agents for adults with acute respiratory distress syndrome. Cochrane Database Syst Rev. 2019;7(7):Cd004477. doi: 10.1002/14651858.CD004477.pub3
- Nanchal RS, Truwit JD. Recent advances in understanding and treating acute respiratory distress syndrome. F1000Res. 2018;7. doi: 10.12688/f1000research.15493.1
- Klingemann H, Matzilevich D, Marchand J. Mesenchymal stem cells – sources and clinical applications. Transfus Med Hemother. 2008;35(4):2–2. doi: 10.1159/000142333
- Hennrick KT, Keeton AG, Nanua S, et al. Lung cells from neonates show a mesenchymal stem cell phenotype. Am J Respir Crit Care Med. 2007;175(11):1158–1164. doi: 10.1164/rccm.200607-941OC
- Lee JH, Tammela T, Hofree M, et al. Anatomically and functionally distinct lung mesenchymal populations marked by Lgr5 and Lgr6. Cell. 2017;170(6):1149–1163.e12. doi: 10.1016/j.cell.2017.07.028
- Laffey JG, Matthay MA. Fifty years of research in ards. cell-based therapy for acute respiratory distress Syndrome.Biology and potential therapeutic value. Am J Respir Crit Care Med. 2017;196(3):266–273. doi: 10.1164/rccm.201701-0107CP
- Shi L, Wang L, Xu R, et al. Mesenchymal stem cell therapy for severe COVID-19. Signal Transduct Target Ther. 2021;6(1):339. doi: 10.1038/s41392-021-00754-6
- Deng H, Wu L, Liu M, et al. Bone marrow mesenchymal stem cell-derived exosomes attenuate LPS-Induced ARDS by modulating macrophage polarization through inhibiting glycolysis in macrophages. Shock. 2020;54(6):828–843. doi: 10.1097/SHK.0000000000001549
- Dos Santos CC, Amatullah H, Vaswani CM, et al. Mesenchymal stromal (stem) cell therapy modulates miR-193b-5p expression to attenuate sepsis-induced acute lung injury. Eur Respir J. 2022;59(1):2004216. doi: 10.1183/13993003.04216-2020