ABSTRACT
Pathological cardiac hypertrophy (referred to as cardiac hypertrophy) is a maladaptive response of the heart to a variety of pathological stimuli, and cardiac hypertrophy is an independent risk factor for heart failure and sudden death. Currently, the treatments for cardiac hypertrophy are limited to improving symptoms and have little effect. Elucidation of the developmental process of cardiac hypertrophy at the molecular level and the identification of new targets for the treatment of cardiac hypertrophy are crucial. In this review, we summarize the research on multiple active substances related to the pathogenesis of cardiac hypertrophy and the signaling pathways involved and focus on the role of transforming growth factor-β (TGF-β) and bone morphogenetic protein (BMP) signaling in the development of cardiac hypertrophy and the identification of potential targets for molecular intervention. We aim to identify important signaling molecules with clinical value and hope to help promote the precise treatment of cardiac hypertrophy and thus improve patient outcomes.
1. Introduction
Pathological cardiac hypertrophy (referred to as cardiac hypertrophy) is a maladaptive response of the heart to a variety of pathological stimuli. This condition mainly manifests as increased heart volume, which is accompanied by cardiomyocyte apoptosis, extracellular interstitial deposition, and cardiac dysfunction, resulting in decreased myocardial contractility, increased oxygen consumption, and decreased compliance and eventually leading to heart failure and sudden cardiac death [Citation1–3] (). The clinical manifestations of cardiac hypertrophy are fatigue, dizziness, chest pain, and dyspnea, which ultimately lead to heart failure in approximately 25% of all deaths [Citation4,Citation5]. Currently, the treatments for myocardial hypertrophy are limited to improving symptoms and have little effect. Therefore, there is an urgent need to further explore the pathogenesis of myocardial hypertrophy and identify the key targets of its occurrence. Although some progress has been made in the pathogenesis of cardiac hypertrophy, its molecular mechanism is still unclear due to its extremely complex pathogenesis, which is mainly related to the activation of multiple cell signaling pathways.
Figure 1. Diagram of the pathological mechanism of the development of pathological cardiac hypertrophy. Pathological stimuli act on normal cardiomyocytes, leading to increase in the cardiomyocyte size and cardiomyocyte apoptosis. Ultimately, these effects lead to the development of pathological cardiac hypertrophy. Ang-II, angiotensin II; BMP, bone morphogenetic protein; EGF, epidermal growth factor; ERK1/2, extracellular signal-regulated kinase 1/2; ET-1, endothelin-1; ISO, isoprenaline; JNK, c-Jun N-terminal kinase; mTOR, mammalian target of rapamycin; NE, norepinephrine; NFAT, nuclear factor of activated T cells; PKC, protein kinase C; RHOA, Ras homolog gene family member A; ROCK, Rho kinase; Smad, small mother against decapentaplegic; TAK1, transforming growth factor-β (TGF-β) activated kinase 1; TGF-β, transforming growth factor-β.
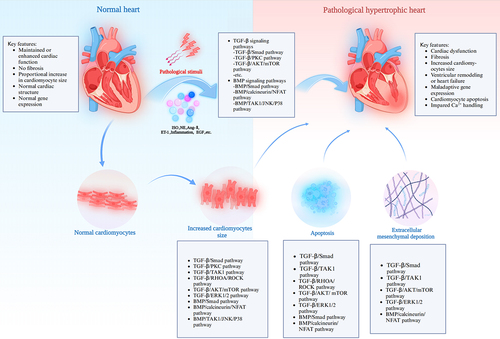
Figure 2. TGF-β signaling pathways in the development of pathological cardiac hypertrophy. The TGF-β signaling pathways include the TGF-β/small mother against decapentaplegic (Smad) signaling pathway and the Smad-independent signaling pathways. The latter includes the TGF-β/PKC, TGF-β/TAK1, TGF-β/RHOA/ROCK, TGF-β/Ras/MERK1/2/ERK1/2, and TGF-β/AKT/mTOR signaling pathways. ALK7, activin receptor-like kinase 7; CAPE, caffeic acid phenethyl ester; CTRP3, c1q-tumor necrosis factor-related protein-3; DAPA, dapagliflozin; DUSP14, dual-specificity phosphatase 14; DUSP26, dual-specificity phosphatase 26; EGCG, epigallocatechin-3-gallate; GCN5, general control nonrepressed protein 5; GPER1, G protein-coupled estrogen receptor 1; HTR2A, 5-hydroxytryptamine receptor 2A; MERK1/2, mitogen-activated extracellular signal-regulated kinase 1/2; NF-κB, nuclear factor kappa B; NO, nitric oxide; PICOT, protein kinase C (PKC)-interacting cousin of thioredoxin; THs, thyroid hormones; P70S6K, protein 70 S6 kinase.
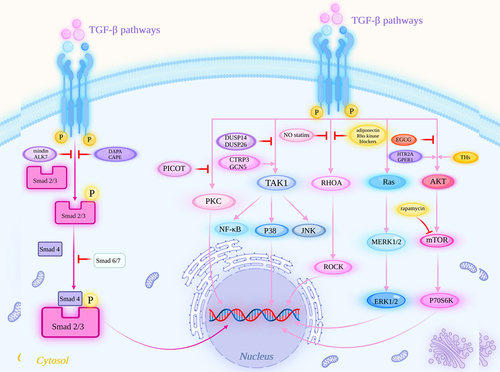
Figure 3. BMP signaling pathways in the development of pathological cardiac hypertrophy. The BMP signaling pathways include the BMP/Smad signaling pathway and BMP/Smad-independent signaling pathways. The latter includes the BMP/calcineurin/NFAT and BMP/TAK1/JNK/P38 signaling pathways. Ca2+, calcium ions; CaM, calmodulin.
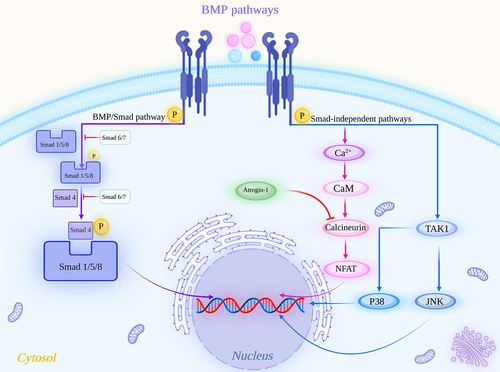
Cardiac hypertrophy is an early hallmark during the clinical course of heart failure and is regulated by various signaling pathways [Citation6]. At present, a growing number of studies have shown that several signaling pathways are involved in the occurrence and development of cardiac hypertrophy, such as the signaling pathway mediated by calcium ions (Ca2+) [Citation7,Citation8], which is the most fundamental signal leading to cardiac hypertrophy; the Wnt signaling pathway [Citation9–11]; the nuclear factor kappa B (NF-κB) signaling pathway [Citation12] and noncoding RNA [Citation13,Citation14]. In addition to these widely known signaling pathways, the transforming growth factor-β (TGF-β) and bone morphogenetic protein (BMP) signaling pathways play integral roles in the occurrence and development of cardiac hypertrophy, such as promoting cardiomyocyte hypertrophy, ventricular fibrosis, cell proliferation, differentiation and cardiomyocyte apoptosis [Citation15–17]. These signaling pathways involve multiple target molecules and interact with each other during the development and outcome of cardiac hypertrophy, so it is necessary to clarify the mechanism of their effect on cardiac hypertrophy ().
The highly pleiotropic cytokine TGF-β is an important contributor to the control of apoptosis, angiogenesis, wound healing, immune regulation, and tumor biology [Citation18]. TGF-β exists in three isoforms (TGF-β1, TGF-β2, and TGF-β3) [Citation19], and virtually all cells have receptors for TGF-βs. Ligands of the TGF-β family bind to various TGF-β receptors, leading to the recruitment and activation of small mother against decapentaplegic (Smad) family transcription factors that regulate gene expression [Citation20]. Myocardial TGF-β expression is upregulated in experimental models of myocardial infarction and cardiac hypertrophy and plays an important role in the pathogenesis of cardiac remodeling [Citation17]. In the myocardium, TGF-β is expressed during cardiac hypertrophy and after myocardial infarction [Citation21] and contributes to remodeling processes by enhancing cardiac fibrosis [Citation17]. TGF-β leads to cardiac fibrosis via activation of the Smad-dependent pathway [Citation22]. In experimental models, TGF-β is particularly expressed in the hypertrophic myocardium during the transition from persistent hypertrophy to heart failure (HF) [Citation23]. The overexpression of TGF-β in transgenic mice results in cardiac hypertrophy characterized by both interstitial fibrosis and hypertrophic growth of cardiomyocytes [Citation24,Citation25]. BMP members of the TGF-β superfamily bind to two different serine/threonine kinase receptors, are widely found in various organs and systems, and play important roles. The BMP signaling pathway plays key roles in the regulation of cell proliferation, differentiation and apoptosis in the cardiovascular system [Citation15,Citation26].
This article reviews the TGF-β and BMP signaling pathways related to the occurrence and development process of cardiac hypertrophy to reveal the mechanism of cardiac hypertrophy at the molecular level and provide a detailed theoretical basis for the treatment of cardiac hypertrophy and the search for new drug treatment targets, which may prevent the development and progression of cardiac hypertrophy and be conducive to reducing the incidence and mortality of cardiovascular diseases.
2. TGF-β signaling pathways and therapeutics in pathological cardiac hypertrophy
2.1. Classical TGF-β/Smad signaling pathway in pathological cardiac hypertrophy
TGF-β expression is induced in the myocardium during the transition from compensated hypertrophy to HF [Citation27]. The TGF-β signaling pathways, which include the Smad-dependent classical TGF-β (TGF-β/Smad) signaling pathway and Smad-independent signaling pathways, play an important role in the development of pathological cardiac hypertrophy [Citation28]. The classical TGF-β/Smad signaling pathway is integral to cardiac hypertrophy and ventricular remodeling [Citation29–32]. TGF-β-induced Smad binding activity, Smad2 phosphorylation and the expression of Smad7 were increased after TGF-β stimulation and blocked with a TGF-β receptor antagonist, which contributed to cardiac hypertrophy and heart failure progression [Citation31]. In addition, some drugs block the TGF-β1/Smad signaling pathway to alleviate cardiac remodeling; for example, an Ang II-induced rat cardiac remodeling model shows that dapagliflozin (DAPA) improves Ang II-induced cardiac remodeling by inhibiting TGF-β1/Smad signaling and inhibits collagen production in cardiac fibroblasts (CFs) in vitro [Citation33]. Heart-specific overexpression of mindin, a secreted extracellular matrix (ECM) protein, attenuates pathological cardiac hypertrophy by blocking TGF-β/Smad signaling, which occurs through the interaction of cardiomyocytes and other heart cell types [Citation32]. Caffeic acid phenethyl ester (CAPE) attenuates cardiomyocyte hypertrophy by inhibiting TGF-β1/Smad signaling pathway [Citation30]. TGF-β1/Smad signaling pathway can also interact with other pathways, and studies have shown that in neonatal rat cardiomyocytes (NRCMs), activin receptor-like kinase 7 (ALK7) may reduce pathological cardiac hypertrophy by blocking mitogen-activated extracellular signal-regulated kinase (MEK)/extracellular signal-regulated kinase 1/2 (ERK1/2) signaling and subsequent inhibition of Smad2/3 signaling [Citation34]. These studies demonstrate the indispensability of the TGF-β1/Smad signaling pathway in cardiac hypertrophy. Of course, a growing number of studies have shown that the nonclassical TGF-β signaling pathways have the same status as the TGF-β1/Smad pathway in the development of pathological cardiac hypertrophy ().
Table 1. Ligands and inhibitors of TGF-β and BMP signaling pathways associated with pathological cardiac hypertrophy.
2.2. Therapeutics targeting the TGF-β/Smad signaling pathway in pathological cardiac hypertrophy
Better knowledge of the intracellular signals of TGF-β can provide novel therapeutic approaches for cardiovascular diseases [Citation35]. One of the mechanisms of action by which verapamil ameliorates cardiac hypertrophy is reducing the level of TGF-β [Citation36]. Zhang Yuze et al. found that pretreatment with DAPA, a sodium-glucose cotransporter 2 inhibitor, inhibits myocardial hypertrophy and fibrosis, increases collagen synthesis, and inhibits the activation of TGF-β1/Smad signaling to improve Ang II-induced cardiac remodeling [Citation37]. Diabetic cardiomyopathy (DCM) is characterized by functional malfunctioning of the heart, myocyte hypertrophy and myocardial fibrosis [Citation38]. Syringaresinol acts on the TGF-β/Smad signaling pathway and inhibits inflammation and fibrosis of cardiomyocytes, which makes it a potential therapeutic agent for the treatment of DCM [Citation39].
Previous studies found that ARBs, such as losartan [Citation40], and lipid-lowering agents, such as rosuvastatin or pitavastatin [Citation41,Citation42], exert antihypertrophic effects, which are associated with the TGF-β/Smad signaling pathway [Citation40]. CAPE and propolis extracts also target this signaling pathway to reduce pathological cardiac hypertrophy induced by pressure overload [Citation30,Citation43]. Targeting the TGF-β/Smad signaling pathway is also observed with the compound Qidan formula, which is composed of Chinese herbs, and the plant extracts daphnetin, aloin and aloe-emodin [Citation44–47]. These substances have promising therapeutic potential against pathological cardiac hypertrophy.
These results demonstrate the importance of the TGF-β/Smad signaling pathway and its potential value as a therapeutic target for pathological myocardial hypertrophy.
2.3. Nonclassical TGF-β signaling pathways and therapeutics in pathological cardiac hypertrophy
2.3.1. TGF-β/PKC signaling pathway in pathological cardiac hypertrophy
The TGF-β/protein kinase C (PKC) signaling pathway is implicated in the development of pathological cardiac hypertrophy [Citation48–52]. In cardiac tissue, PKC activity is increased following pressure overload, suggesting that it is involved in the development of cardiac hypertrophy [Citation53]. PICOT (PKC-Interacting Cousin Of Thioredoxin), a putative PKC inhibitor, inhibits cardiac remodeling and increases ventricular function and cardiomyocyte contractility by blocking the hypertrophic response of NRCMs [Citation54]. PICOT-mediated inhibition of mitogen-activated protein kinase (MAPK) activation was investigated using antibodies against phospho-ERK1/2 and phospho-c-Jun N-terminal kinase (JNK), and the results suggested that it may counteract hypertrophic stimulation by inhibiting PKC and the downstream ERK1/2 and JNK signaling pathways. M Uenoyama and colleagues used an immunohistochemical method and found that PKC-α expression is increased on the right ventricular plasma membrane of mice exposed to a hypobaric hypoxic environment (HHE) for 3 days, indicating that PKC-α is associated with right ventricular hypertrophy in HHE [Citation52].
2.3.2. Therapeutics targeting the TGF-β/PKC signaling pathway in pathological cardiac hypertrophy
Potential therapeutics targeting the TGF-β/PKC signaling pathway have also been researched. For example, endothelin receptor antagonists, such as bosentan, attenuate right ventricular hypertrophy and fibrosis in a chronic hypoxia model of pulmonary hypertension by altering PKC activity [Citation55]. Chinese medicine ingredients, such as breviscapine and sodium ferulate, also act on PKC targets [Citation56–58]. The progression of paricalcitol treatment in preventing pathological cardiac hypertrophy in rats has also been found to be associated with the PKC signaling pathway [Citation59]. In general, the TGF-β/PKC signaling pathway has great research value as a therapeutic target to improve pathological cardiac hypertrophy in the future.
2.3.3. Positive regulators of the TGF-β/TAK1 signaling pathway in pathological cardiac hypertrophy
A series of studies have shown that activation of the TGF-β/TGF-β activated kinase 1 (TAK1) signaling pathway plays an important role in the pathogenesis of pathological cardiac hypertrophy [Citation60–66]. TAK1 is a MAP kinase kinase kinase (MAPKKK) involved in numerous signaling pathways and is strongly implicated in pathological cardiac hypertrophy and heart failure [Citation67]. TGF-β/TAK1 is a component of the TGF-β nonclassical (Smad-independent) signaling pathways. In some mouse models of thoracic aortic constriction (TAC)-induced cardiomyocyte hypertrophy, the phosphorylation levels of TAK1 are increased, which can induce pathological cardiac hypertrophy [Citation60,Citation68]. The phosphorylation of TAK1 and its downstream kinase JNK by tomoregulin-1, a growth factor primarily expressed in cardiomyocytes but not in CFs, are inhibited when it was overexpressed and are significantly activated in tomoregulin-1-knockdown mice [Citation60]. In addition, TAK1 mediates the activation of the c-JNK and p38 pathways and NF-κB, which are also involved in the development of cardiac hypertrophy [Citation69]. An activating mutation of TAK1 in the myocardium of transgenic mice is sufficient to induce p38 mitogen-activated protein kinase phosphorylation in vivo and cardiac hypertrophy [Citation70]. Once TAK1 is activated by TGF-β [Citation69], ERK, JNK and p38 translocate to the nucleus, where they phosphorylate a variety of intracellular transcription factors and are involved in the development of pathological myocardial hypertrophy. Cardiomyocyte-specific C1q-tumor necrosis factor-related protein-3 (CTRP3) overexpression promotes pressure overload-induced cardiac hypertrophy via activation of the TAK1/JNK signaling pathway [Citation67]. General control nonrepressed protein 5 (GCN5) exacerbates cardiac hypertrophy via excessive activation of the TAK1-JNK/p38 signaling pathway in isolated NRCMs [Citation71]. JNK activation has also been found to be associated with load-induced cardiac hypertrophy and heart failure [Citation72].
2.3.4. Negative regulators of the TGF-β/TAK1 signaling pathway in pathological cardiac hypertrophy
There are related studies on the negative regulators of these pathways. For instance, dual-specificity phosphatase 14 (DUSP 14) and DUSP26, which are important negative modulators of mitogen-activated protein kinase (MAPK) signaling pathways, protect against pressure overload-induced hypertrophic growth of cardiomyocytes by modulating the TAK1-JNK/p38 signaling axis [Citation64,Citation73]. He Huang et al. showed that tumor suppressor A20 expression markedly blocks the TAK1-JNK/p38 signaling cascade to inhibit apoptosis and pathological cardiac hypertrophy in cultured NRCMs and blocks TGF-β1-induced collagen synthesis in CFs [Citation65]. These studies reflect the importance of the TGF-β/TAK1 signaling pathway in the occurrence and development of cardiac hypertrophy.
2.3.5. Therapeutics targeting the TGF-β/TAK1 signaling pathway in pathological cardiac hypertrophy
Cellular and molecular biology studies have indicated that TAK1 is an effective therapeutic target for pathological cardiac hypertrophy, which is essential for pathological cardiac hypertrophy and heart failure. D Zhang et al. found that TAK1 kinase activity is upregulated in the adult mouse myocardium to induce hypertrophy and TGF-β expression [Citation70]. Previous studies have found that arjunolic acid-induced peroxisome proliferator-activated receptor α (PPARα) upregulation inhibits TGF-β signaling, and the mechanism involves inhibiting TAK1 phosphorylation, reducing p38 MAPK activation and ultimately ameliorating cardiac hypertrophy [Citation74]. A recent study showed that salidroside, as a Toll-like receptor 4 (TLR4) inhibitor, displays antihypertrophic action by acting on the TAK1/JNK/p38 pathway [Citation75,Citation76]. Tabersonine, an indole alkaloid mainly isolated from roses, targets TAK1, blocks downstream NF-κB and JNK/P38 MAPK signaling activation and decreases cardiac inflammation and fibrosis [Citation77,Citation78].
2.3.6. TGF-β/RHOA/ROCK signaling pathway in pathological cardiac hypertrophy
The TGF-β/Ras homolog gene family member A (RhoA)/Rho kinase (ROCK) signaling pathway is involved in the development of various diseases, such as hypertension [Citation79], myocardial infarction [Citation80], cardiac hypertrophy [Citation81–85], and acute lung injury [Citation86]. Among the molecules involved in the signaling pathways causing cardiac hypertrophy, RhoA has been widely studied. RhoA is a dubious molecule in cardiac pathophysiology [Citation87]. The plethora of transcriptional mediators downstream of RhoA suggest specific mechanisms by which RhoA contributes to cardiomyocyte hypertrophic gene expression [Citation88]. ROCK is an effector of RhoA [Citation83,Citation89]. Some studies have shown that blocking the TGF-β/RhoA/ROCK pathway can improve cardiac hypertrophy [Citation84,Citation85,Citation90]. J Craig Hunter et al. found that the anti-cardiomyocyte hypertrophy effect of nitric oxide (NO) is due, in part, to cGMP-dependent inhibition of the TGF-β/RhoA/ROCK signaling pathway [Citation84]. Wared Nour-Eldine et al. showed that adiponectin could prevent Ang II-induced apoptosis of human endothelial cells (ECs) and inhibit vascular smooth muscle cells (VSMCs) hypertrophy through NO-dependent inhibition of the RhoA/ROCK pathway [Citation85]. In addition, some drugs exert their protective effects on the cardiovascular system through RhoA signaling pathway [Citation91,Citation92]. One study used statins or Rho kinase blockers to assess RhoA as a target of interventional approaches to blunt hypertrophy or heart failure [Citation88]. Simvastatin prevents the cardiomyocyte hypertrophy response in hypertensive rats by inhibiting RhoA pathway [Citation93]. These studies indicate that activation of the TGF-β/RhoA/ROCK signaling pathway plays a pivotal role in the development of cardiomyocyte hypertrophy.
2.3.7. Therapeutics targeting the TGF-β/RHOA/ROCK signaling pathway in pathological cardiac hypertrophy
RhoA/ROCK targets participate in the progression of pathological cardiac hypertrophy. A previous experiment demonstrated that fasudil, a specific Rho-kinase inhibitor, significantly suppresses hypertrophic changes in rat vascular smooth muscle and cardiomyocytes [Citation94]. Fasudil treatment improves ventricular hypertrophy [Citation95,Citation96]. A study using ROCK inhibitors, such as fasudil, showed that the RhoA/ROCK signaling pathway plays a pivotal role in cardiac hypertrophy and ventricular remodeling [Citation97]. Some substances exert antihypertrophic effects by targeting multiple pathways; for instance, KMUP-1, a synthetic xanthine-based derivative, alleviates ET-1-induced cardiac hypertrophy by inhibiting RhoA/ROCK pathway [Citation98]. RhoA/ROCK-targeted therapy interventions for the treatment of pathological cardiac hypertrophy are valuable.
2.3.8. Positive regulators of the TGF-β/AKT/mTOR signaling pathway in pathological cardiac hypertrophy
AKT, which is also known as protein kinase B, is a serine/threonine protein kinase that participates in the mammalian target of rapamycin (mTOR) signaling pathway, which controls the assembly of the eukaryotic translation initiation factor 4F (eIF4E) complex, and this pathway responds to extracellular signals from growth factors and cytokines and is dysregulated in many diseases, including cardiac hypertrophy [Citation73,Citation99–104]. 5-Hydroxytryptamine receptor 2A (HTR2A) can activate TGF-β/AKT/mTOR signaling in cardiomyocytes to promote cardiac hypertrophy [Citation105]. Stimulation of cardiac hypertrophy by lysophosphatidic acid (LPA) is associated with the AKT/mTOR pathway within cardiomyocytes [Citation100]. Using in vivo gene delivery of specific short hairpin RNAs against stromal interaction molecule 1 (STIM1) revealed that STIM1 regulates AKT kinase activity through activation of mTOR complex 2 in cardiomyocytes, which impairs mal-adaptive hypertrophy and promotes heart failure through inactivation of mTORC2/AKT signaling [Citation106]. Using thyroid hormone (TH)-induced hypertrophy in mice and neonatal cardiomyocytes, James A Kuzman et al. found that hypertrophy was mediated by the AKT/mTOR signaling pathway [Citation107]. Pei Hui et al. found that G protein-coupled estrogen receptor 1 (GPER1) activation affects Ang II-induced cardiomyocyte hypertrophy by upregulating the AKT/mTOR signaling pathway [Citation99].
2.3.9. Negative regulators of the TGF-β/AKT/mTOR signaling pathway in pathological cardiac hypertrophy
However, numerous studies have shown that blocking the TGF-β/AKT/mTOR pathway alleviates cardiac hypertrophy. Rapamycin is an inhibitor of mTOR and can prevent cardiac hypertrophy [Citation108]. In a mouse model of cardiac hypertrophy induced by transverse aortic constriction (TAC), AKT/mTOR signaling pathway activation is inhibited by epigallocatechin-3-gallate (EGCG) to inhibit cardiomyocyte apoptosis and CF proliferation, which alleviates pathological cardiac hypertrophy [Citation104]. Allicin and galangin attenuate pathological cardiomyocyte hypertrophy by activating TGF-β/AKT/mTOR [Citation109,Citation110]. Gabriela Placona Diniz et al. found that angiotensin type 1 receptor (AT1R) silencing or blockade attenuated or completely prevented the activation of this signaling pathway [Citation111]. Phosphorylated AKT, mTOR and S6 kinase (S6K) are increased in the hearts of TAC mice, and by using siRNA-mediated gene knockdown, Di Zhao et al. found that protein kinase D (PKD) knockdown inhibits activation of the AKT/mTOR/S6K pathway and attenuates the cardiomyocyte hypertrophy response and cardiac remodeling during pressure overload [Citation112]. These studies suggest that factors that alleviate cardiac hypertrophy can exert common effects by inhibiting the TGF-β/AKT/mTOR signaling pathway.
2.3.10. Therapeutics targeting the TGF-β/AKT/mTOR signaling pathway in pathological cardiac hypertrophy
Many drugs, such as allicin, xanthohumol, resveratrol, epigallocatechin-3-gallate, and melatonin, act on pathological cardiac hypertrophy through the AKT/mTOR signaling pathway [Citation101,Citation109,Citation113–115]. Some traditional Chinese medicines, such as the Yiqi Fumai lyophilized injection, a traditional compound preparation of Chinese medicine, and pinoresinol diglucoside, can also act on this signaling pathway [Citation116,Citation117]. Studies have found the potential value of AKT/mTOR in developing treatments for pathological myocardial hypertrophy.
2.3.11. TGF-β/ERK1/2 signaling pathway in pathological cardiac hypertrophy
Mouse models of aortic band (AB)-induced cardiac hypertrophy revealed that TGF-β/extracellular signal-regulated kinase (ERK) signaling pathway plays important roles in the regulation of cardiac hypertrophy caused by stress overload [Citation30]. ERK acts in a signaling cascade that regulates various cellular processes, such as proliferation, differentiation, and cell cycle progression, in response to a variety of extracellular signals [Citation118]. Researchers have used transgenic or knockout mouse models to investigate the relationship between ERK signaling and cardiac hypertrophy. A review of the recent literature shows that various stimulatory factors, such as epidermal growth factor (EGF), act through protein tyrosine kinase (PTK), activate Ras protein and then act on the MAPK system, and ERK1/2 is an important signaling molecule in the MAPK signaling pathway [Citation119]. In addition, ERK1/2 has been identified as a downstream nuclear mediator of ROCK during myocardial cell hypertrophy [Citation120]. ERK2 directly phosphorylates GATA binding protein 4 (GATA4), which is a known regulator of hypertrophic gene expression in cardiomyocytes in response to hypertrophic agonist stimulation [Citation88,Citation119]. Sustained angiotensin II receptor (ATR) stimulation upregulates the expression of growth factors in human CFs by TGF-β/ERK signaling, which is associated with pathological cardiac hypertrophy [Citation121].
2.3.12. Therapeutics targeting the TGF-β/ERK1/2 signaling pathway in pathological cardiac hypertrophy
Silybin is known to exert a protective effect on liver cells, and a previous study found that this substance also attenuates the phenylephrine-induced hypertrophic response of H9c2 cells by inhibiting the signaling pathways mediated by ERK1/2 MAPKs [Citation122]. Hui Meng et al. found that Baoyuan decoction, a Chinese medicinal formulation, ameliorates cardiac hypertrophy and improves heart failure by acting on the ERK/GATA4 pathway [Citation123], providing a novel therapeutic option. The potential therapeutic target of the Yi-Xin-Shu capsule in protecting against cardiac hypertrophy is also GATA4 [Citation124]. Experimental studies have found that drugs acting on ERK1/2 targets for the treatment of cardiac hypertrophy also include puerarin and the renin inhibitor aliskiren [Citation125,Citation126].
3. BMP signaling pathways and therapeutics in pathological cardiac hypertrophy
In addition to the TGF-β signaling pathway, a series of signaling pathways mediated by BMP also play an important role in the occurrence and development of pathological cardiac hypertrophy. BMP mediates signals through the Smad-dependent pathway and Smad-independent pathways [Citation127]. Cardiac hypertrophy has been linked to aberrant BMP signaling [Citation16]. Among them, the BMP/Smad signaling pathway is a typical signaling pathway leading to cardiac hypertrophy. In addition to this canonical signaling pathway, noncanonical BMP signaling pathways independent of Smad have also been identified, and these include the various branches of the MAPK pathway; moreover, JNK/p38 have been implicated as important regulators of cardiomyocyte hypertrophic growth in culture [Citation128]. BMPs and their receptors are needed for pulmonary arterial hypertension (PAH)-induced right ventricular hypertrophy, and the restoration of BMP type II receptor (BMPR2) signaling is a promising alternative that could prevent and reverse pulmonary vascular remodeling [Citation129]. Here, we summarize the roles of different signaling pathways mediated by BMP in myocardial hypertrophy ().
3.1. BMP/Smad signaling pathway in pathological cardiac hypertrophy
Among more than 20 ligands in the BMP family, the best-characterized BMPs in the cardiovascular system are BMP2, BMP4, BMP7 and BMP9 [Citation16]. BMPs form heterotetrameric signaling complexes with type I and two type II receptors, and then the type II receptors phosphorylate the type I receptor, causing type I receptor activation and the phosphorylation of receptor-regulated Smad (R-Smad) effector proteins [Citation130]. The R-Smad/Co-Smad complexes then translocate into the nucleus and regulate the transcription of target genes by interacting with various transcription factors and transcriptional coactivators or corepressors [Citation127]. Smad1 and Smad5 primarily mediate BMP signals [Citation131]. Smad1, Smad5 and Smad8 are R-Smads that are activated by BMP type I receptors, and Smad2 and Smad3 are activated by activin and TGF-β type I receptors [Citation132,Citation133]. Guangwang Liu et al. noted that the Smad3 pathway is a potential therapeutic target for reducing the fibrotic response following myocardial infarction (MI) and hypoxia/reperfusion injury [Citation134], and a similar finding was reported by Marcin Bujak [Citation135]. Smad4 is activated by the BMP and TGF-β/activin signaling pathways [Citation136]. The BMP/Smad signaling pathway is a typical signaling pathway leading to cardiac hypertrophy; a study demonstrated the proapoptotic effect of BMP4 and Smad1/5/8 on rat neonatal cardiomyocytes exposed to hypoxia/reoxygenation [Citation137]. Activation of BMP signaling with BMP2 or BMP4 induced hypertrophy in neonatal rat cardiomyocytes [Citation15]. M Izumi’s team found that BMP2 and Smad1 enhanced the expression of the antiapoptotic molecule Bcl-x(L), and BMP2 prevented apoptosis in myocytes by inducing Bcl-x(L) via the Smad1 pathway without any hypertrophic effect on myocytes [Citation26]. This study shows that BMP2 alone does not induce cardiomyocyte hypertrophy and apoptosis but antagonizes BMP4-induced cardiomyocyte hypertrophy and apoptosis. BMP4-induced cardiomyocyte hypertrophy and apoptosis might occur through activating AKT and inhibiting JNK activation [Citation138]. Of course, the role of Smad-independent pathways in cardiac hypertrophy should not be underestimated.
3.2. Therapeutics targeting the BMP/Smad signaling pathway in pathological cardiac hypertrophy
Traditional Chinese medicine is effective in treating cardiovascular diseases. Astragalus polysaccharides, a component extracted from Astragalus membranaceus, alleviate cardiac hypertrophy in DCM by inhibiting the BMP-mediated signaling pathway [Citation139]. Although clinical research is limited, its protective effect on cardiac hypertrophy has a potential translational effect. Further research is needed on the mechanism of Smad-targeted action on the BMP signaling pathway for the treatment of pathological cardiac hypertrophy.
3.3. BMP/independent Smad signaling pathways and therapeutics in pathological cardiac hypertrophy
3.3.1. BMP/calcineurin/NFAT signaling pathway in pathological cardiac hypertrophy
The Ca2+/calmodulin (CaM)-activated calcineurin-nuclear factor of activated T cells (NFAT) signaling pathway has been shown to play a role in the development of pathological cardiac hypertrophy [Citation140,Citation141]. Ca2+ regulates cardiac hypertrophic processes via two primary Ca2+-related signaling pathways, calcineurin-NFAT and Ca2+/calmodulin-dependent protein kinase II (CaMKII)-myocyte enhancer factor 2 [Citation142]. The treatment of neonatal rat ventricular myocytes with phenylephrine for 72 hours increases calcineurin activity and the introduction of NFAT proteins into the nuclei of cardiomyocytes, which mediates the transcription of targets involved in hypertrophic growth [Citation140]. BMP signaling stimulates the calcineurin/NFAT pathway in cardiomyocytes, which upregulates the calcineurin/nuclear factor of the activated T-cell pathway via the BMP type I receptor activin receptor-like kinase-2 (ALK2), contributing to cardiac hypertrophy and fibrosis [Citation15]. Atrogin-1 is a ubiquitin ligase that targets calcineurin for degradation [Citation16]. However, BMP signaling inhibits atrogin-1 expression, upregulates the calcineurin/NFAT pathway and contributes to the development of cardiac hypertrophy [Citation15].
3.3.2. Therapeutics targeting the BMP/calcineurin/NFAT signaling pathway in pathological cardiac hypertrophy
Calcineurin/NFAT provides a therapeutic target for the treatment of cardiac hypertrophy [Citation143]. Phosphodiesterase-5A (PDE5A) inhibition deactivates calcineurin/NFAT signaling pathways; for example, sildenafil, tacrolimus (FK506) and cyclosporine can prevent cardiac hypertrophy and fibrosis by directly targeting the calcineurin signaling pathway [Citation144–146]. Similarly, betulinic acid and cisapride exert therapeutic effects through this signaling pathway [Citation147–149]. Therefore, targeting the calcineurin/NFAT pathway may provide new approaches for the treatment of pathological cardiac hypertrophy.
3.3.3. BMP/TAK1/JNK/P38 signaling pathway in pathological cardiac hypertrophy
TAK1 is a MAPKKK that is activated by TGF-β, BMP and other MAPK signaling components [Citation150,Citation151]. During development, BMP and TGF-β signaling use a Smad1/5-independent mechanism to activate the expression of TAK1, which is known to be a potent inhibitor of apoptosis [Citation152]. Lea M Gunnell et al. showed that the loss of TAK1 leads to reduced activation of BMP/Smad signaling during cartilage and joint development [Citation150]. The correlation between BMP and TAK1 activation in pathological cardiac hypertrophy has not been systematically elucidated. Thus, the relationship between the BMP/TAK1 signaling pathway in the development of pathological cardiac hypertrophy and ventricular remodeling still needs to be further explored.
3.3.4. Therapeutics targeting the BMP/TAK1/JNK/P38 signaling pathway in pathological cardiac hypertrophy
Phytochemicals, such as tabersonine, salidroside and genistein, have gained attention as alternative therapeutics for cardiovascular diseases, and their underlying mechanism is the regulation of TAK1. The administration of 6-gingerol protects against pressure overload-induced cardiac hypertrophy in TAC mice in a p38-dependent manner [Citation74,Citation76,Citation78,Citation153,Citation154]. These studies provide insights into the development of therapies targeting TAK1 for the treatment of pathological cardiac hypertrophy. Further research is needed to explore drugs that target this signaling pathway to more accurately prevent the occurrence of pathological cardiac hypertrophy.
4. Other signaling pathways in pathological cardiac hypertrophy
Protein phosphatase 2A (PP2A) is one of the four major Ser/Thr phosphatases and is implicated in the negative control of cell growth and division. Some studies have shown that TGF-β/PP2A is associated with the development of pathological cardiac hypertrophy [Citation155–157]. p21-activated kinase-1 (Pak-1) is a serine/threonine protein kinase that activates PP2A [Citation158]. Active PAK-1 is a natural inhibitor of ERK1/2 and a novel antihypertrophic signaling molecule that is upstream of PP2A [Citation156]. PP2A may inhibit cardiomyocyte hypertrophy by dephosphorylating ERK1/2 [Citation156]. Somy Yoon et al. suggested that PP2A is a critical regulator of pathological cardiac hypertrophy [Citation157]. The inhibition of pathological cardiac hypertrophy by casein kinase-2 interacting protein-1 (CKIP-1), a regulator of cell proliferation and apoptosis and an important regulator of pathological cardiac remodeling, was achieved by increasing the dephosphorylation of histone deacetylase 4 (HDAC4) through the recruitment of PP2A, and this signaling cascade was associated with pathological hypertrophy [Citation155,Citation159]. The specific mechanisms by which PP2A exerts its antihypertrophic effects via TGF-β to regulate the development of myocardial hypertrophy and the other downstream signaling molecules involved in this signaling pathway need to be further investigated. The extent to which the effect of TGF-β on pathological myocardial hypertrophy is mediated through PP2A signaling is unclear. Further studies on the downstream molecules and specific mechanisms of TGF-β/PP2A may be important for the treatment and prognosis of pathological cardiac hypertrophy.
In addition to these signaling pathways, many other pathways play important roles in the development of pathological cardiac hypertrophy. For example, obesity leads not only to myocardial hypertrophy but also to ventricular dysfunction, and its molecular mechanisms include Ca2+ homeostasis, myocardial fibrosis, and endothelial damage [Citation160]. Macrophage doublecortin-like kinase 1 promotes obesity-induced cardiomyopathy by activating TAK1 targeting [Citation161]. Empagliflozin may improve obesity-related cardiac dysfunction by targeting mTOR [Citation162]. Clarifying the signaling pathways involved in obesity-related cardiac hypertrophy is crucial, and studying the related molecular mechanisms will provide a theoretical basis for intervention and treatment.
5. Conclusions and perspectives
In this review, we summarize and analyze the TGF-β and BMP signaling pathways involved in the development of pathological cardiac hypertrophy. The development of cardiac hypertrophy is accompanied by a change in signals in complex cascades, and each signaling pathway is not regulated independently, which forms a complex regulatory network. Therefore, it is crucial to further strengthen the overall understanding of the signaling pathways associated with cardiac hypertrophy to provide effective targets for the diagnosis and treatment of patients with HF, seek more effective prevention and treatment measures for new targets, prevent the progression of pathological cardiac hypertrophy, and contribute to the prevention and treatment of human cardiac hypertrophy and related diseases.
Author contributions
Ling Zhu and Yuanyuan Sun conceived the review. Jing Wen and Guixiang Liu analyzed the current literature and wrote the first draft of the manuscript. Mingjie Liu, Huarui Wang, Yunyan Wan, Zhouhong Yao, and Nannan Gao wrote sections of the manuscript. All authors have read and agreed to the published version of the manuscript.
Institutional review board statement
This study does not involve ethical approval.
Acknowledgements
We apologize to the many authors of the exceptional papers not directly cited due to space limitations.
Data availability statement
The research data are not involved in this study.
Disclosure statement
The authors declare that the research was conducted in the absence of any commercial or financial relationships that could be construed as a potential conflict of interest.
Additional information
Funding
References
- Heineke J, Molkentin JD. Regulation of cardiac hypertrophy by intracellular signalling pathways. Nat Rev Mol Cell Biol. 2006 Aug;7(8):589–600. doi: 10.1038/nrm1983
- Artham SM, Lavie CJ, Milani RV. et al. Clinical impact of left ventricular hypertrophy and implications for regression. Prog Cardiovasc Dis. 2009 Sep-Oct;52(2):153–167.
- Kavey RE. Left ventricular hypertrophy in hypertensive children and adolescents: predictors and prevalence. Curr Hypertens Rep. 2013 Oct;15(5):453–457. doi: 10.1007/s11906-013-0370-3
- Sabbah HN. Silent disease progression in clinically stable heart failure. Eur J Heart Fail. 2017 Apr;19(4):469–478. doi: 10.1002/ejhf.705
- Michels M, Olivotto I, Asselbergs FW, et al. Life-long tailoring of management for patients with hypertrophic cardiomyopathy: awareness and decision-making in changing scenarios. Neth Heart J. 2017 Mar;25(3):186–199. doi: 10.1007/s12471-016-0943-2
- Bisserier M, Berthouze-Duquesnes M, Breckler M, et al. Carabin protects against cardiac hypertrophy by blocking calcineurin, Ras, and Ca2+/calmodulin-dependent protein kinase II signaling. Circulation. 2015 Jan 27;131(4):390–400. discussion 400. doi: 10.1161/circulationaha.114.010686
- Gul R, Shawl AI, Kim SH, et al. Cooperative interaction between reactive oxygen species and Ca2+ signals contributes to angiotensin II-induced hypertrophy in adult rat cardiomyocytes. Am J Physiol Heart Circ Physiol. 2012 Feb 15;302(4):H901–909. doi: 10.1152/ajpheart.00250.2011
- Ruwhof C, van der Laarse A. Mechanical stress-induced cardiac hypertrophy: mechanisms and signal transduction pathways. Cardiovasc Res. 2000 Jul;47(1):23–37. doi: 10.1016/s0008-6363(00)00076-6
- Stylianidis V, Hermans KCM, Blankesteijn WM. Wnt signaling in cardiac remodeling and heart failure. Handb Exp Pharmacol. 2017;243:371–393. doi: 10.1007/164_2016_56
- Li Q, Jiang W, Wan Z, et al. Polyphyllin I attenuates pressure over-load induced cardiac hypertrophy via inhibition of Wnt/beta-catenin signaling pathway. Life Sci. 2020 Jul 1;252:117624. doi: 10.1016/j.lfs.2020.117624
- Malekar P, Hagenmueller M, Anyanwu A, et al. Wnt signaling is critical for maladaptive cardiac hypertrophy and accelerates myocardial remodeling. Hypertension. 2010 Apr;55(4):939–945. doi: 10.1161/HYPERTENSIONAHA.109.141127
- Li Y, Ha T, Gao X, et al. NF-κB activation is required for the development of cardiac hypertrophy in vivo. Am J Physiol Heart Circ Physiol. 2004 Oct;287(4):H1712–H1720.
- Zhang Q, Wang F, Wang F. et al. Long noncoding RNA MAGI1-IT1 regulates cardiac hypertrophy by modulating miR-302e/DKK1/Wnt/beta-catenin signaling pathway. J Cell Physiol. 2020 Jan;235(1):245–253.
- Silva J, da Costa Martins PA. Non-coding RNAs in the therapeutic landscape of pathological cardiac hypertrophy. Cells. 2022 May 31;11(11):1805. doi: 10.3390/cells11111805
- Shahid M, Spagnolli E, Ernande L, et al. BMP type I receptor ALK2 is required for angiotensin II-induced cardiac hypertrophy. Am J Physiol Heart Circ Physiol. 2016 Apr 15;310(8):H984–994. doi: 10.1152/ajpheart.00879.2015
- Luo JY, Zhang Y, Wang L, et al. Regulators and effectors of bone morphogenetic protein signalling in the cardiovascular system. J Physiol. 2015 Jul 15;593(14):2995–3011. doi: 10.1113/JP270207
- Dobaczewski M, Chen W, Frangogiannis NG. Transforming growth factor (TGF)-beta signaling in cardiac remodeling. J Mol Cell Cardiol. 2011 Oct;51(4):600–606. doi: 10.1016/j.yjmcc.2010.10.033
- Prud’homme GJ. Pathobiology of transforming growth factor beta in cancer, fibrosis and immunologic disease, and therapeutic considerations. Lab Invest. 2007 Nov;87(11):1077–1091. doi: 10.1038/labinvest.3700669
- Morikawa M, Derynck R, Miyazono K. TGF-β and the TGF-β family: context-dependent roles in Cell and tissue physiology. Cold Spring Harb Perspect Biol. 2016 May 2;8(5):a021873. doi: 10.1101/cshperspect.a021873
- Gomez-Bernal F, Quevedo-Abeledo JC, Garcia-Gonzalez M, et al. Serum levels of transforming growth factor beta 1 in systemic lupus erythematosus patients. Biomolecules.2022 Dec 29;13(1):73. doi: 10.3390/biom13010073
- Hanna A, Frangogiannis NG. The role of the TGF-beta superfamily in myocardial infarction. Front Cardiovasc Med. 2019 Sep 18;6:140. doi: 10.3389/fcvm.2019.00140
- Yue Y, Meng K, Pu Y. et al. Transforming growth factor beta (TGF-β) mediates cardiac fibrosis and induces diabetic cardiomyopathy. Diabet Res Clin Pract. 2017 Nov;133:124–130.
- Boluyt MO, Bing OH, Lakatta EG. The ageing spontaneously hypertensive rat as a model of the transition from stable compensated hypertrophy to heart failure. Eur Heart J. 1995 Dec;16(suppl N):19–30. doi: 10.1093/eurheartj/16.suppl_n.19
- Lijnen PJ, Petrov VV, Fagard RH. Induction of cardiac fibrosis by transforming growth factor-beta(1). Mol Genet Metab. 2000 Sep-Oct;71(1–2):418–435. doi: 10.1006/mgme.2000.3032
- Rosenkranz S, Flesch M, Amann K, et al. Alterations of β-adrenergic signaling and cardiac hypertrophy in transgenic mice overexpressing TGF-β 1. Am J Physiol Heart Circ Physiol. 2002 Sep;283(3):H1253–H1262.
- Izumi M, Fujio Y, Kunisada K, et al. Bone morphogenetic protein-2 inhibits serum deprivation-induced apoptosis of neonatal cardiac myocytes through activation of the Smad1 pathway. J Biol Chem. 2001 Aug 17;276(33):31133–31141. doi: 10.1074/jbc.M101463200
- Heger J, Schulz R, Euler G. Molecular switches under TGFβ signalling during progression from cardiac hypertrophy to heart failure. Br J Pharmacol. 2016 Jan;173(1):3–14. doi: 10.1111/bph.13344
- Frangogiannis NG. Transforming growth factor-β in myocardial disease. Nat Rev Cardiol. 2022 Jul;19(7):435–455. doi: 10.1038/s41569-021-00646-w
- Koitabashi N, Danner T, Zaiman AL, et al. Pivotal role of cardiomyocyte TGF-beta signaling in the murine pathological response to sustained pressure overload. J Clin Invest. 2011 Jun;121(6):2301–2312.
- Ren J, Zhang N, Liao H, et al. Caffeic acid phenethyl ester attenuates pathological cardiac hypertrophy by regulation of MEK/ERK signaling pathway in vivo and vitro. Life Sci. 2017 Jul 15;181:53–61. doi: 10.1016/j.lfs.2017.04.016
- Heger J, Warga B, Meyering B, et al. TGFbeta receptor activation enhances cardiac apoptosis via SMAD activation and concomitant NO release. J Cell Physiol. 2011 Oct;226(10):2683–2690.
- Yan L, Wei X, Tang QZ, et al. Cardiac-specific mindin overexpression attenuates cardiac hypertrophy via blocking AKT/GSK3beta and TGF-beta1-Smad signalling. Cardiovasc Res. 2011 Oct 1;92(1):85–94. doi: 10.1093/cvr/cvr159
- Zhang Y, Lin X, Chu Y, et al. Dapagliflozin: a sodium-glucose cotransporter 2 inhibitor, attenuates angiotensin II-induced cardiac fibrotic remodeling by regulating TGFbeta1/Smad signaling. Cardiovasc Diabetol.2021 Jun 11;20(1):121. doi: 10.1186/s12933-021-01312-8
- Huang H, Tang Y, Wu G, et al. ALK7 protects against pathological cardiac hypertrophy in mice. Cardiovasc Res.2015 Oct 1;108(1):50–61. doi: 10.1093/cvr/cvv206
- Ruiz-Ortega M, Rodríguez-Vita J, Sanchez-Lopez E, et al. TGF-beta signaling in vascular fibrosis. Cardiovasc Res. 2007 May 1;74(2):196–206. doi: 10.1016/j.cardiores.2007.02.008
- Mendes AS, Blascke de Mello MM, Parente JM, et al. Verapamil decreases calpain-1 and matrix metalloproteinase-2 activities and improves hypertension-induced hypertrophic cardiac remodeling in rats. Life Sci. 2020 Mar 1;244:117153. doi: 10.1016/j.lfs.2019.117153
- Zhang Y, Lin X, Chu Y, et al. Dapagliflozin: a sodium-glucose cotransporter 2 inhibitor, attenuates angiotensin II-induced cardiac fibrotic remodeling by regulating TGFβ1/Smad signaling. Cardiovasc Diabetol.2021 Jun 11;20(1):121. doi: 10.1186/s12933-021-01312-8
- Seksaria S, Mehan S, Dutta BJ. et al. Oxymatrine and insulin resistance: focusing on mechanistic intricacies involve in diabetes associated cardiomyopathy via SIRT1/AMPK and TGF-β signaling pathway. J Biochem Mol Toxicol. 2023 May;37(5):e23330.
- Li G, Yang L, Feng L, et al. Syringaresinol protects against type 1 diabetic cardiomyopathy by alleviating inflammation responses, cardiac fibrosis, and oxidative stress. Mol Nutr Food Res. 2020 Sep;64(18):e2000231. doi: 10.1002/mnfr.202000231
- Kovács MG, Kovács ZZA, Varga Z, et al. Investigation of the antihypertrophic and antifibrotic effects of losartan in a rat model of radiation-induced heart disease. Int J Mol Sci. 2021 Nov 30;22(23):12963. doi: 10.3390/ijms222312963
- Wang P, Luo L, Shen Q, et al. Rosuvastatin improves myocardial hypertrophy after hemodynamic pressure overload via regulating the crosstalk of Nrf2/ARE and TGF-β/smads pathways in rat heart. Eur J Pharmacol. 2018 Feb 5;820:173–182. doi: 10.1016/j.ejphar.2017.12.013
- Yagi S, Aihara K, Ikeda Y, et al. Pitavastatin, an HMG-CoA reductase inhibitor, exerts eNOS-independent protective actions against angiotensin II induced cardiovascular remodeling and renal insufficiency. Circ Res. 2008 Jan 4;102(1):68–76. doi: 10.1161/circresaha.107.163493
- Fu YK, Wang BJ, Tseng JC, et al. Combination treatment of docetaxel with caffeic acid phenethyl ester suppresses the survival and the proliferation of docetaxel-resistant prostate cancer cells via induction of apoptosis and metabolism interference. J Biomed Sci.2022 Feb 23;29(1):16. doi: 10.1186/s12929-022-00797-z
- Yuan P, Liu J, Xiong S, et al. Effects and mechanism of compound qidan formula on rats with HFpEF induced by hypertension and diabetes mellitus based on Ang II/TGF-β1/Smads signaling pathway. J Ethnopharmacol. 2023 Sep 15;313:116558. doi: 10.1016/j.jep.2023.116558
- Syed AM, Kundu S, Ram C. et al. Up-regulation of Nrf2/HO-1 and inhibition of TGF-β1/Smad2/3 signaling axis by daphnetin alleviates transverse aortic constriction-induced cardiac remodeling in mice. Free Radic Biol Med. 2022 Jun;186:17–30.
- Syed AM, Kundu S, Ram C, et al. Aloin alleviates pathological cardiac hypertrophy via modulation of the oxidative and fibrotic response. Life Sci. 2022 Jan 1;288:120159. doi: 10.1016/j.lfs.2021.120159
- Yu J, Zhao X, Yan X, et al. Aloe-emodin ameliorated MI-induced cardiac remodeling in mice via inhibiting TGF-β/SMAD signaling via up-regulating SMAD7. Phytomedicine. 2023 Jun;114:154793. doi: 10.1016/j.phymed.2023.154793
- Lim JY, Park SJ, Hwang HY, et al. TGF-beta1 induces cardiac hypertrophic responses via PKC-dependent ATF-2 activation. J Mol Cell Cardiol. 2005 Oct;39(4):627–636.
- Braz JC, Bueno OF, De Windt LJ, et al. PKC alpha regulates the hypertrophic growth of cardiomyocytes through extracellular signal-regulated kinase1/2 (ERK1/2). J Cell Bio. 2002 Mar 4;156(5):905–919. doi: 10.1083/jcb.200108062
- Pan J, Singh US, Takahashi T. et al. PKC mediates cyclic stretch-induced cardiac hypertrophy through Rho family GTPases and mitogen-activated protein kinases in cardiomyocytes. J Cell Physiol. 2005 Feb;202(2):536–553.
- Li J, Huang J, Lu J, et al. Sirtuin 1 represses PKC-zeta activity through regulating interplay of acetylation and phosphorylation in cardiac hypertrophy. Br J Pharmacol. 2019 Feb;176(3):416–435.
- Uenoyama M, Ogata S, Nakanishi K. et al. Protein kinase C mRNA and protein expressions in hypobaric hypoxia-induced cardiac hypertrophy in rats. Acta Physiol (Oxf). 2010 Apr;198(4):431–440.
- Braun MU, LaRosée P, Schön S, et al. Differential regulation of cardiac protein kinase C isozyme expression after aortic banding in rat. Cardiovasc Res. 2002 Oct;56(1):52–63.
- Jeong D, Cha H, Kim E, et al. PICOT inhibits cardiac hypertrophy and enhances ventricular function and cardiomyocyte contractility. Circ Res. 2006 Aug 4;99(3):307–314. doi: 10.1161/01.RES.0000234780.06115.2c
- Choudhary G, Troncales F, Martin D. et al. Bosentan attenuates right ventricular hypertrophy and fibrosis in normobaric hypoxia model of pulmonary hypertension. J Heart Lung Transplant. 2011 Jul;30(7):827–833.
- Wang M, Zhang WB, Zhu JH, et al. Breviscapine ameliorates hypertrophy of cardiomyocytes induced by high glucose in diabetic rats via the PKC signaling pathway. Acta Pharmacol Sin. 2009 Aug;30(8):1081–1091. doi: 10.1038/aps.2009.95
- Yan L, Huang H, Tang QZ, et al. Breviscapine protects against cardiac hypertrophy through blocking PKC-alpha-dependent signaling. J Cell Biochem. 2010 Apr 15;109(6):1158–1171. doi: 10.1002/jcb.22495
- Luo M, Chen PP, Yang L. et al. Sodium ferulate inhibits myocardial hypertrophy induced by abdominal coarctation in rats: involvement of cardiac PKC and MAPK signaling pathways. Biomed Pharmacother. 2019 Apr;112:108735.
- Bae S, Yalamarti B, Ke Q, et al. Preventing progression of cardiac hypertrophy and development of heart failure by paricalcitol therapy in rats. Cardiovasc Res. 2011 Sep 1;91(4):632–639. doi: 10.1093/cvr/cvr133
- Bao D, Lu D, Liu N, et al. Tomoregulin-1 prevents cardiac hypertrophy after pressure overload in mice by inhibiting TAK1-JNK pathways. Dis Model Mech. 2015 Aug 1;8(8):795–804. doi: 10.1242/dmm.021303
- Si L, Xu J, Yi C, et al. Asiatic acid attenuates cardiac hypertrophy by blocking transforming growth factor-beta1-mediated hypertrophic signaling in vitro and in vivo. Int J Mol Med. 2014 Aug;34(2):499–506.
- Yan X, Zhao R, Feng X, et al. Sialyltransferase7A promotes angiotensin II-induced cardiomyocyte hypertrophy via HIF-1alpha-TAK1 signalling pathway. Cardiovasc Res. 2020 Jan 1;116(1):114–126. doi: 10.1093/cvr/cvz064
- Huang XY, Chen CX. Effect of oxymatrine, the active component from radix sophorae flavescentis (Kushen), on ventricular remodeling in spontaneously hypertensive rats. Phytomedicine. 2013 Feb 15;20(3–4):202–212. doi: 10.1016/j.phymed.2012.10.012
- Zhao J, Jiang X, Liu J, et al. Dual-specificity phosphatase 26 protects against cardiac hypertrophy through TAK1. J Am Heart Assoc. 2021 Feb 16;10(4):e014311. doi: 10.1161/JAHA.119.014311
- Huang H, Tang QZ, Wang AB, et al. Tumor suppressor A20 protects against cardiac hypertrophy and fibrosis by blocking transforming growth factor-beta-activated kinase 1-dependent signaling. Hypertension. 2010 Aug;56(2):232–239.
- Chen L, Huang J, Ji YX, et al. Tripartite Motif 8 contributes to pathological cardiac hypertrophy through enhancing transforming growth factor beta-activated kinase 1-dependent signaling pathways. Hypertension. 2017 Feb;69(2):249–258.
- Ma ZG, Yuan YP, Zhang X, et al. C1q-tumour necrosis factor-related protein-3 exacerbates cardiac hypertrophy in mice. Cardiovasc Res. 2019 May 1;115(6):1067–1077. doi: 10.1093/cvr/cvy279
- Guo S, Liu Y, Gao L, et al. TBC1D25 regulates cardiac remodeling through TAK1 signaling pathway. Int J Biol Sci. 2020 Feb 21;16(8):1335–1348. doi: 10.7150/ijbs.41130
- Dai L, Aye Thu C, Liu XY, et al. TAK1, more than just innate immunity. IUBMB Life. 2012 Oct;64(10):825–834. doi: 10.1002/iub.1078
- Zhang D, Gaussin V, Taffet GE, et al. TAK1 is activated in the myocardium after pressure overload and is sufficient to provoke heart failure in transgenic mice. Nat Med. 2000 May;6(5):556–563. doi: 10.1038/75037
- Li J, Yan C, Wang Y, et al. GCN5-mediated regulation of pathological cardiac hypertrophy via activation of the TAK1-JNK/p38 signaling pathway. Cell Death Dis.2022 Apr 30;13(4):421. doi: 10.1038/s41419-022-04881-y
- Choukroun G, Hajjar R, Fry S, et al. Regulation of cardiac hypertrophy in vivo by the stress-activated protein kinases/c-Jun NH(2)-terminal kinases. J Clin Invest. 1999 Aug;104(4):391–398.
- Li CY, Zhou Q, Yang LC, et al. Dual-specificity phosphatase 14 protects the heart from aortic banding-induced cardiac hypertrophy and dysfunction through inactivation of TAK1-P38MAPK/-JNK1/2 signaling pathway. Basic Res Cardiol. 2016 Mar;111(2):19. doi: 10.1007/s00395-016-0536-7
- Bansal T, Chatterjee E, Singh J, et al. Arjunolic acid, a peroxisome proliferator-activated receptor α agonist, regresses cardiac fibrosis by inhibiting non-canonical TGF-β signaling. J Biol Chem. 2017 Oct 6;292(40):16440–16462. doi: 10.1074/jbc.M117.788299
- Mian MOR, He Y, Bertagnolli M, et al. TLR (toll-like receptor) 4 antagonism prevents left ventricular hypertrophy and dysfunction caused by neonatal hyperoxia exposure in rats. Hypertension. 2019 Oct;74(4):843–853. doi: 10.1161/hypertensionaha.119.13022
- Guo Z, Liu FY, Yang D. et al. Salidroside ameliorates pathological cardiac hypertrophy via TLR4-TAK1-dependent signaling. Phytother Res. 2023 May;37(5):1839–1849.
- Chen Y, Lin W, Chen P. et al. Tabersonine alleviates obesity-induced cardiomyopathy by binding to transforming growth factor activated kinase 1 (TAK1) and inhibiting TAK1-mediated inflammation. Phytother Res. 2023 Mar;37(3):860–871.
- Dai C, Luo W, Chen Y, et al. Tabersonine attenuates angiotensin II-induced cardiac remodeling and dysfunction through targeting TAK1 and inhibiting TAK1-mediated cardiac inflammation. Phytomedicine. 2022 Aug;103:154238. doi: 10.1016/j.phymed.2022.154238
- Wang X, Jiang S, Fei L, et al. Tacrolimus causes hypertension by increasing vascular contractility via RhoA (Ras homolog family member A)/ROCK (Rho-associated protein kinase) pathway in mice. Hypertension. 2022 Oct;79(10):2228–2238. doi: 10.1161/HYPERTENSIONAHA.122.19189
- Zhang Q, Wang L, Wang S, et al. Signaling pathways and targeted therapy for myocardial infarction. Signal Transduct Target Ther.2022 Mar 10;7(1):78. doi: 10.1038/s41392-022-00925-z
- Zhou Q, Wei SS, Wang H, et al. Crucial role of ROCK2-mediated phosphorylation and upregulation of FHOD3 in the pathogenesis of angiotensin II-Induced cardiac hypertrophy. Hypertension. 2017 Jun;69(6):1070–1083.
- Zeidan A, Javadov S, Chakrabarti S, et al. Leptin-induced cardiomyocyte hypertrophy involves selective caveolae and RhoA/ROCK-dependent p38 MAPK translocation to nuclei. Cardiovasc Res. 2008 Jan;77(1):64–72.
- Loirand G, Guerin P, Pacaud P. Rho kinases in cardiovascular physiology and pathophysiology. Circ Res. 2006 Feb 17;98(3):322–334. doi: 10.1161/01.RES.0000201960.04223.3c
- Hunter JC, Zeidan A, Javadov S. et al. Nitric oxide inhibits endothelin-1-induced neonatal cardiomyocyte hypertrophy via a RhoA-ROCK-dependent pathway. J Mol Cell Cardiol. 2009 Dec;47(6):810–818.
- Nour-Eldine W, Ghantous CM, Zibara K, et al. Adiponectin attenuates angiotensin II-Induced vascular smooth muscle Cell remodeling through nitric oxide and the RhoA/ROCK pathway. Front Pharmacol. 2016 Apr 7;7:86. doi: 10.3389/fphar.2016.00086
- Chen B, Gong S, Li M. et al. Protective effect of oxyberberine against acute lung injury in mice via inhibiting RhoA/ROCK signaling pathway. Biomed Pharmacother. 2022 Sep;153:113307.
- Kilian LS, Voran J, Frank D, et al. RhoA: a dubious molecule in cardiac pathophysiology. J Biomed Sci.2021 Apr 28;28(1):33. doi: 10.1186/s12929-021-00730-w
- Brown JH, Del Re DP, Sussman MA. The Rac and Rho hall of fame: a decade of hypertrophic signaling hits. Circ Res. 2006 Mar 31;98(6):730–742. doi: 10.1161/01.RES.0000216039.75913.9e
- Noma K, Oyama N, Liao JK. Physiological role of ROCKs in the cardiovascular system. Am J Physiol Cell Physiol. 2006 Mar;290(3):C661–668. doi: 10.1152/ajpcell.00459.2005
- Zeidan A, Javadov S, Karmazyn M. Essential role of Rho/ROCK-dependent processes and actin dynamics in mediating leptin-induced hypertrophy in rat neonatal ventricular myocytes. Cardiovasc Res. 2006 Oct 1;72(1):101–111. doi: 10.1016/j.cardiores.2006.06.024
- Oka M, Fagan KA, Jones PL. et al. Therapeutic potential of RhoA/Rho kinase inhibitors in pulmonary hypertension. Br J Pharmacol. 2008 Oct;155(4):444–454.
- Zhu L, Liu F, Hao Q, et al. Dietary geranylgeranyl pyrophosphate counteracts the benefits of statin therapy in experimental pulmonary hypertension. Circulation.2021 May 4;143(18):1775–1792. doi: 10.1161/CIRCULATIONAHA.120.046542
- Takayama N, Kai H, Kudo H, et al. Simvastatin prevents large blood pressure variability induced aggravation of cardiac hypertrophy in hypertensive rats by inhibiting RhoA/Ras-ERK pathways. Hypertens Res. 2011 Mar;34(3):341–347.
- Higashi M, Shimokawa H, Hattori T, et al. Long-term inhibition of Rho-kinase suppresses angiotensin II-induced cardiovascular hypertrophy in rats in vivo: effect on endothelial NAD(P)H oxidase system. Circ Res. 2003 Oct 17;93(8):767–775. doi: 10.1161/01.Res.0000096650.91688.28
- Abe K, Shimokawa H, Morikawa K, et al. Long-term treatment with a Rho-kinase inhibitor improves monocrotaline-induced fatal pulmonary hypertension in rats. Circ Res. 2004 Feb 20;94(3):385–393. doi: 10.1161/01.Res.0000111804.34509.94
- Li FH, Xia W, Li AW, et al. Inhibition of rho kinase attenuates high flow induced pulmonary hypertension in rats. Chin Med J (Engl). 2007 Jan 5;120(1):22–29. doi: 10.1097/00029330-200701010-00005
- Ren J, Fang CX. Small guanine nucleotide-binding protein Rho and myocardial function. Acta Pharmacol Sin. 2005 Mar;26(3):279–285. doi: 10.1111/j.1745-7254.2005.00059.x
- Liou SF, Hsu JH, Chen YT, et al. KMUP-1 attenuates endothelin-1-induced cardiomyocyte hypertrophy through activation of heme oxygenase-1 and suppression of the Akt/GSK-3β, calcineurin/NFATc4 and RhoA/ROCK pathways. Molecules. 2015 Jun 5;20(6):10435–10449. doi: 10.3390/molecules200610435
- Pei H, Wang W, Zhao D, et al. G protein-coupled estrogen receptor 1 inhibits angiotensin II-Induced cardiomyocyte hypertrophy via the regulation of PI3K-Akt-mTOR signalling and autophagy. Int J Biol Sci. 2019 Jan 6;15(1):81–92. doi: 10.7150/ijbs.28304
- Yang J, Xu J, Han X, et al. Lysophosphatidic Acid Is Associated With Cardiac Dysfunction and Hypertrophy by Suppressing Autophagy via the LPA3/AKT/mTOR Pathway. Front Physiol. 2018 Sep 18;9:1315. doi: 10.3389/fphys.2018.01315
- Guan P, Sun ZM, Wang N, et al. Resveratrol prevents chronic intermittent hypoxia-induced cardiac hypertrophy by targeting the PI3K/AKT/mTOR pathway. Life Sci. 2019 Sep 15;233:116748. doi: 10.1016/j.lfs.2019.116748
- Gao W, Guo N, Zhao S, et al. Carboxypeptidase A4 promotes cardiomyocyte hypertrophy through activating PI3K-AKT-mTOR signaling. Biosci Rep. 2020 May 29;40(5): BSR20200669. 10.1042/BSR20200669
- Clemente CF, Xavier-Neto J, Dalla Costa AP. et al. Focal adhesion kinase governs cardiac concentric hypertrophic growth by activating the AKT and mTOR pathways. J Mol Cell Cardiol. 2012 Feb;52(2):493–501.
- Cui Y, Wang Y, Liu G. Epigallocatechin gallate (EGCG) attenuates myocardial hypertrophy and fibrosis induced by transverse aortic constriction via inhibiting the Akt/mTOR pathway. Pharm Biol. 2021 Dec;59(1):1305–1313. doi: 10.1080/13880209.2021.1972124
- Gao W, Guo N, Zhao S. et al. HTR2A promotes the development of cardiac hypertrophy by activating PI3K-PDK1-AKT-mTOR signaling. Cell Stress Chaperones. 2020 Nov;25(6):899–908.
- Benard L, Oh JG, Cacheux M, et al. Cardiac Stim1 silencing impairs adaptive hypertrophy and promotes heart failure through inactivation of mTorc2/akt signaling. Circulation. 2016 Apr 12;133(15):1458–1471. discussion 1471. doi: 10.1161/CIRCULATIONAHA.115.020678
- Kuzman JA, O’Connell TD, Gerdes AM. Rapamycin prevents thyroid hormone-induced cardiac hypertrophy. Endocrinology. 2007 Jul;148(7):3477–3484. doi: 10.1210/en.2007-0099
- Bodine SC, Stitt TN, Gonzalez M, et al. Akt/mTOR pathway is a crucial regulator of skeletal muscle hypertrophy and can prevent muscle atrophy in vivo. Nat Cell Biol. 2001 Nov;3(11):1014–1019. doi: 10.1038/ncb1101-1014
- Ba L, Gao J, Chen Y, et al. Allicin attenuates pathological cardiac hypertrophy by inhibiting autophagy via activation of PI3K/Akt/mTOR and MAPK/ERK/mTOR signaling pathways. Phytomedicine. 2019 May;58:152765. doi: 10.1016/j.phymed.2018.11.025
- Wang HB, Huang SH, Xu M, et al. Galangin ameliorates cardiac remodeling via the MEK1/2-ERK1/2 and PI3K-AKT pathways. J Cell Physiol. 2019 Sep;234(9):15654–15667.
- Diniz GP, Carneiro-Ramos MS, Barreto-Chaves ML. Angiotensin type 1 receptor mediates thyroid hormone-induced cardiomyocyte hypertrophy through the Akt/GSK-3beta/mTOR signaling pathway. Basic Res Cardiol. 2009 Nov;104(6):653–667. doi: 10.1007/s00395-009-0043-1
- Zhao D, Wang W, Wang H, et al. PKD knockdown inhibits pressure overload-induced cardiac hypertrophy by promoting autophagy via AKT/mTOR pathway. Int J Biol Sci. 2017 Feb 12;13(3):276–285. doi: 10.7150/ijbs.17617
- Sun TL, Li WQ, Tong XL, et al. Xanthohumol attenuates isoprenaline-induced cardiac hypertrophy and fibrosis through regulating PTEN/AKT/mTOR pathway. Eur J Pharmacol. 2021 Jan 15;891:173690. doi: 10.1016/j.ejphar.2020.173690
- Li HL, Huang Y, Zhang CN, et al. Epigallocatechin-3 gallate inhibits cardiac hypertrophy through blocking reactive oxidative species-dependent and -independent signal pathways. Free Radic Biol Med. 2006 May 15;40(10):1756–1775. doi: 10.1016/j.freeradbiomed.2006.01.005
- Xu CN, Kong LH, Ding P, et al. Melatonin ameliorates pressure overload-induced cardiac hypertrophy by attenuating Atg5-dependent autophagy and activating the Akt/mTOR pathway. Biochim Biophys Acta Mol Basis Dis. 2020 Oct 1;1866(10):165848. doi: 10.1016/j.bbadis.2020.165848
- Wan M, Yin K, Yuan J, et al. YQFM alleviated cardiac hypertrophy by apoptosis inhibition and autophagy regulation via PI(3)K/AKT/mTOR pathway. J Ethnopharmacol. 2022 Mar 1;285:114835. doi: 10.1016/j.jep.2021.114835
- Chen Y, Pan R, Zhang J, et al. Pinoresinol diglucoside (PDG) attenuates cardiac hypertrophy via AKT/mTOR/NF-κB signaling in pressure overload-induced rats. J Ethnopharmacol. 2021 May 23;272:113920. doi: 10.1016/j.jep.2021.113920
- Lavoie H, Gagnon J, Therrien M. ERK signalling: a master regulator of cell behaviour, life and fate. Nat Rev Mol Cell Biol. 2020 Oct;21(10):607–632. doi: 10.1038/s41580-020-0255-7
- Bueno OF, Molkentin JD. Involvement of extracellular signal-regulated kinases 1/2 in cardiac hypertrophy and cell death. Circ Res. 2002 Nov 1;91(9):776–781. doi: 10.1161/01.res.0000038488.38975.1a
- Yanazume T, Hasegawa K, Wada H, et al. Rho/ROCK pathway contributes to the activation of extracellular signal-regulated kinase/GATA-4 during myocardial cell hypertrophy. J Biol Chem. 2002 Mar 8;277(10):8618–8625. doi: 10.1074/jbc.M107924200
- Duangrat R, Parichatikanond W, Morales NP, et al. Sustained AT(1)R stimulation induces upregulation of growth factors in human cardiac fibroblasts via G(alphaq)/TGF-beta/ERK signaling that influences myocyte hypertrophy. Eur J Pharmacol. 2022 Dec 15;937:175384. doi: 10.1016/j.ejphar.2022.175384
- Anestopoulos I, Kavo A, Tentes I. et al. Silibinin protects H9c2 cardiac cells from oxidative stress and inhibits phenylephrine-induced hypertrophy: potential mechanisms. J Nutr Biochem. 2013 Mar;24(3):586–594.
- Meng H, Du Z, Lu W, et al. Baoyuan decoction (BYD) attenuates cardiac hypertrophy through ANKRD1-ERK/GATA4 pathway in heart failure after acute myocardial infarction. Phytomedicine. 2021 Aug;89:153617. doi: 10.1016/j.phymed.2021.153617
- Zhang M, Guo F, Li X, et al. Yi-Xin-shu capsule ameliorates cardiac hypertrophy by regulating RB/HDAC1/GATA4 signaling pathway based on proteomic and mass spectrometry image analysis. Phytomedicine. 2022 Aug;103:154185. doi: 10.1016/j.phymed.2022.154185
- Chen G, Pan SQ, Shen C, et al. Puerarin inhibits angiotensin II-induced cardiac hypertrophy via the redox-sensitive ERK1/2, p38 and NF-κB pathways. Acta Pharmacol Sin. 2014 Apr;35(4):463–475. doi: 10.1038/aps.2013.185
- Weng LQ, Zhang WB, Ye Y, et al. Aliskiren ameliorates pressure overload-induced heart hypertrophy and fibrosis in mice. Acta Pharmacol Sin. 2014 Aug;35(8):1005–1014. doi: 10.1038/aps.2014.45
- Miyazono K, Maeda S, Imamura T. BMP receptor signaling: transcriptional targets, regulation of signals, and signaling cross-talk. Cytokine Growth Factor Rev. 2005 Jun;16(3):251–263. doi: 10.1016/j.cytogfr.2005.01.009
- Bueno OF, De Windt LJ, Tymitz KM, et al. The MEK1-ERK1/2 signaling pathway promotes compensated cardiac hypertrophy in transgenic mice. EMBO J. 2000 Dec 1;19(23):6341–6350. doi: 10.1093/emboj/19.23.6341
- Xin Z, Wang J, Li S, et al. A review of BMP and Wnt signaling pathway in the pathogenesis of pulmonary arterial hypertension. Clin Exp Hypertens. 2022 Feb 17;44(2):175–180. doi: 10.1080/10641963.2021.1996590
- Morrell NW, Bloch DB, ten Dijke P, et al. Targeting BMP signalling in cardiovascular disease and anaemia. Nat Rev Cardiol. 2016 Feb;13(2):106–120. doi: 10.1038/nrcardio.2015.156
- Umans L, Cox L, Tjwa M. et al. Inactivation of Smad5 in endothelial cells and smooth muscle cells demonstrates that Smad5 is required for cardiac homeostasis. Am J Pathol. 2007 May;170(5):1460–1472.
- Miyazono K. Signal transduction by bone morphogenetic protein receptors: functional roles of Smad proteins. Bone. 1999 Jul;25(1):91–93. doi: 10.1016/s8756-3282(99)00113-1
- Pardali K, Kowanetz M, Heldin CH, et al. Smad pathway-specific transcriptional regulation of the cell cycle inhibitor p21(WAF1/Cip1). J Cell Physiol. 2005 Jul;204(1):260–272.
- Liu G, Ma C, Yang H. et al. Transforming growth factor β and its role in heart disease. Exp Ther Med. 2017 May;13(5):2123–2128.
- Bujak M, Ren G, Kweon HJ, et al. Essential role of Smad3 in infarct healing and in the pathogenesis of cardiac remodeling. Circulation. 2007 Nov 6;116(19):2127–2138. doi: 10.1161/circulationaha.107.704197
- Derynck R, Zhang YE. Smad-dependent and Smad-independent pathways in TGF-beta family signalling. Nature. 2003 Oct 9;425(6958):577–584. doi: 10.1038/nature02006
- Ogura Y, Ouchi N, Ohashi K, et al. Therapeutic impact of follistatin-like 1 on myocardial ischemic injury in preclinical models. Circulation.2012 Oct 2;126(14):1728–1738. doi: 10.1161/CIRCULATIONAHA.112.115089
- Lu J, Sun B, Huo R. et al. Bone morphogenetic protein-2 antagonizes bone morphogenetic protein-4 induced cardiomyocyte hypertrophy and apoptosis. J Cell Physiol. 2014 Oct;229(10):1503–1510.
- Sun S, Yang S, Zhang N, et al. Astragalus polysaccharides alleviates cardiac hypertrophy in diabetic cardiomyopathy via inhibiting the BMP10-mediated signaling pathway. Phytomedicine. 2023 Jan;109:154543. doi: 10.1016/j.phymed.2022.154543
- Ding W, Dong M, Deng J, et al. Polydatin attenuates cardiac hypertrophy through modulation of cardiac Ca2+ handling and calcineurin-NFAT signaling pathway. Am J Physiol Heart Circ Physiol. 2014 Sep 1;307(5):H792–802. doi: 10.1152/ajpheart.00017.2014
- Saito T, Fukuzawa J, Osaki J. et al. Roles of calcineurin and calcium/calmodulin-dependent protein kinase II in pressure overload-induced cardiac hypertrophy. J Mol Cell Cardiol. 2003 Sep;35(9):1153–1160.
- Du Y, Demillard LJ, Ren J. Catecholamine-induced cardiotoxicity: a critical element in the pathophysiology of stroke-induced heart injury. Life Sci. 2021 Dec 15;287:120106. doi: 10.1016/j.lfs.2021.120106
- Shimoyama M, Hayashi D, Takimoto E, et al. Calcineurin plays a critical role in pressure overload-induced cardiac hypertrophy. Circulation. 1999 Dec 14;100(24):2449–2454. doi: 10.1161/01.cir.100.24.2449
- Takimoto E, Champion HC, Li M, et al. Chronic inhibition of cyclic GMP phosphodiesterase 5A prevents and reverses cardiac hypertrophy. Nat Med. 2005 Feb;11(2):214–222. doi: 10.1038/nm1175
- Takeda Y, Yoneda T, Demura M, et al. Calcineurin inhibition attenuates mineralocorticoid-induced cardiac hypertrophy. Circulation. 2002 Feb 12;105(6):677–679. doi: 10.1161/hc0602.104675
- Fiedler B, Wollert KC. Interference of antihypertrophic molecules and signaling pathways with the Ca2±calcineurin-NFAT cascade in cardiac myocytes. Cardiovasc Res. 2004 Aug 15;63(3):450–457. doi: 10.1016/j.cardiores.2004.04.002
- Hong MH, Na SW, Jang YJ, et al. Betulinic acid improves cardiac-renal dysfunction caused by hypertrophy through calcineurin-NFATc3 signaling. Nutrients.2021 Sep 30;13(10):3484. doi: 10.3390/nu13103484
- Yoon JJ, Son CO, Kim HY, et al. Betulinic acid protects DOX-Triggered cardiomyocyte hypertrophy response through the GATA-4/Calcineurin/NFAT pathway. Molecules. 2020 Dec 24;26(1):53. doi: 10.3390/molecules26010053
- Zhou X, Zhang Q, Zhao T, et al. Cisapride protects against cardiac hypertrophy via inhibiting the up-regulation of calcineurin and NFATc-3. Eur J Pharmacol. 2014 Jul 15;735:202–210. doi: 10.1016/j.ejphar.2014.04.012
- Gunnell LM, Jonason JH, Loiselle AE, et al. TAK1 regulates cartilage and joint development via the MAPK and BMP signaling pathways. J Bone Miner Res. 2010 Aug;25(8):1784–1797. doi: 10.1002/jbmr.79
- Yamaguchi K, Nagai S, Ninomiya-Tsuji J, et al. XIAP, a cellular member of the inhibitor of apoptosis protein family, links the receptors to TAB1-TAK1 in the BMP signaling pathway. EMBO J. 1999 Jan 4;18(1):179–187. doi: 10.1093/emboj/18.1.179
- Augeri DJ, Langenfeld E, Castle M, et al. Inhibition of BMP and of TGFβ receptors downregulates expression of XIAP and TAK1 leading to lung cancer cell death. Mol Cancer. 2016 Apr 6;15(1):27. doi: 10.1186/s12943-016-0511-9
- Qin W, Du N, Zhang L. et al. Genistein alleviates pressure overload-induced cardiac dysfunction and interstitial fibrosis in mice. Br J Pharmacol. 2015 Dec;172(23):5559–5572.
- Ma SQ, Guo Z, Liu FY, et al. 6-gingerol protects against cardiac remodeling by inhibiting the p38 mitogen-activated protein kinase pathway. Acta Pharmacol Sin. 2021 Oct;42(10):1575–1586. doi: 10.1038/s41401-020-00587-z
- Ling S, Sun Q, Li Y, et al. CKIP-1 inhibits cardiac hypertrophy by regulating class II histone deacetylase phosphorylation through recruiting PP2A. Circulation. 2012 Dec 18;126(25):3028–3040. doi: 10.1161/CIRCULATIONAHA.112.102780
- Taglieri DM, Monasky MM, Knezevic I. et al. Ablation of p21-activated kinase-1 in mice promotes isoproterenol-induced cardiac hypertrophy in association with activation of Erk1/2 and inhibition of protein phosphatase 2A. J Mol Cell Cardiol. 2011 Dec;51(6):988–996.
- Yoon S, Kook T, Min HK, et al. PP2A negatively regulates the hypertrophic response by dephosphorylating HDAC2 S394 in the heart. Exp Mol Med. 2018 Jul 26;50(7):1–14. doi: 10.1038/s12276-018-0121-2
- Ke Y, Wang L, Pyle WG, et al. Intracellular localization and functional effects of P21-activated kinase-1 (Pak1) in cardiac myocytes. Circ Res. 2004 Feb 6;94(2):194–200. doi: 10.1161/01.RES.0000111522.02730.56
- Zhao Y, Ling S, Zhong G, et al. Casein kinase-2 interacting protein-1 regulates physiological cardiac hypertrophy via inhibition of histone deacetylase 4 phosphorylation. Front Physiol. 2021 Jun 15;12:678863. doi: 10.3389/fphys.2021.678863
- Ren J, Wu NN, Wang S, et al. Obesity cardiomyopathy: evidence, mechanisms, and therapeutic implications. Physiol Rev. 2021 Oct 1;101(4):1745–1807. doi: 10.1152/physrev.00030.2020
- Yang B, Zhao Y, Luo W, et al. Macrophage DCLK1 promotes obesity-induced cardiomyopathy via activating RIP2/TAK1 signaling pathway. Cell Death Dis.2023 Jul 13;14(7):419. doi: 10.1038/s41419-023-05960-4
- Sun X, Han F, Lu Q, et al. Empagliflozin ameliorates obesity-related cardiac dysfunction by regulating Sestrin2-mediated AMPK-mTOR signaling and Redox homeostasis in high-fat diet-induced obese mice. Diabetes. 2020 Jun;69(6):1292–1305.