ABSTRACT
Objective: Run-off-road events occur frequently and can result in severe consequences. Several potential injury-causing mechanisms can be observed in the diverse types of run-off-road events. Real-world data show that different types of environments, such as rough terrain, ditch types, and whether multiple events occur, may be important contributing factors to occupant injury. Though countermeasures addressing front seat occupants have been presented, studies on rear seat occupant retention in situations such as run-off-road events are lacking. The aim of this study was to investigate the seat belt pre-pretensioner effect on rear-seated child-sized anthropomorphic test devices (ATDs) during 2 different types of run-off-road events.
Methods: The study was carried out using 2 test setups: a rig test with a vehicle rear seat mounted on a multi-axial robot simulating a road departure event into a side ditch and an in-vehicle test setup with a Volvo XC60 entering a side ditch with a grass slope, driving inside the ditch, and returning back to the road from the ditch. Potential subsequent rollovers or impacts were not included in the test setups. Three different ATDs were used. The Q6 and Q10 were seated on an integrated booster cushion and the Hybrid III (HIII) 5th percentile female was positioned directly on the seat. The seat belt retractor was equipped with a pre-pretensioner (electrical reversible retractor) with 3 force level settings. In addition, reference tests with the pre-pretensioner inactivated were run. Kinematics and the shoulder belt position were analyzed.
Results: In rig tests, the left-seated ATD was exposed to rapid inboard lateral loads relative to the vehicle. The displacement for each ATD was reduced when the pre-pretensioner was activated compared to tests when it was inactivated. Maximum inboard displacement occurred earlier in the event for all ATDs when the pre-pretensioner was activated. Shoulder belt slip-off occurred for the Q6 and Q10 in tests where the pre-pretensioner was inactivated. During in-vehicle tests, the left-seated ATD was exposed to an inboard movement when entering the road again after driving in the ditch. The maximum inboard head displacement was reduced in tests where the pre-pretensioner was activated compared to tests in which it was inactivated.
Conclusions: During both test setups, the activation of the pre-pretensioner resulted in reduced lateral excursion of the Q6, Q10, and HIII 5th percentile female due to the shoulder belt remaining on the shoulder and supporting the side of the lower torso. The results provide new insights into the potential benefits of using a pre-pretensioner to reduce kinematic responses during complex run-off-road events through supporting the seat belt to remain on the shoulder. This study addresses potential countermeasures to improve real-world protection of rear-seated children, and it provides a broader perspective including the influence of precrash kinematics.
Introduction
Run-off-road events occur frequently and the consequences can be severe. In the United States, single-vehicle roadway departure crashes accounted for about 20% of all police-reported crashes (Wang and Knipling Citation1994). In Germany, 33% of all fatal crashes were run-off-road crashes (Statistisches Bundesamt Citation2011). Single-vehicle car crashes represent the most common type with regard to fatal accidents in Sweden (Hillerdal Citation2011). Several potential injury-causing mechanisms can be observed in the diverse types of run-off-road events. Based on real-world data, Jakobsson et al. (Citation2014) highlighted the different types of environments, such as rough terrain, different types of ditches, and whether multiple events occur, as important contributing factors to occupant injury. In more than 80% of the run-off-road cases studied, no turnovers or rollovers occurred. Injury outcome was influenced by occupant position during an event: head and arms impacting the interior, bent postures reducing the tolerance for spinal injuries, and suboptimal occupant positioning relative to protection systems. Jakobsson et al. (Citation2015) presented countermeasures addressing the safety of front seat occupants through activation of an electrical reversible seat belt pretensioner when run-off-road events were detected, together with a unique energy-absorbing functionality in the front seats.
Worldwide, road traffic was the second leading cause of death among 5- to 14-year-olds in 2002 (World Health Organization Citation2004). In the United States, in 2006, on average 5 children 14 years and younger were killed and 568 were injured each day in motor vehicle crashes (NHTSA Citation2006). The majority of rear seat occupants are less than 13 years old (McCray et al. Citation2006). Furthermore, the head is the most commonly injured body region resulting in severe injuries among children injured in motor vehicle crashes, regardless of restraint system and crash direction (Arbogast et al. Citation2004; Durbin et al. Citation2003; Howard et al. Citation2004). Bohman, Arbogast, and Bostrom (Citation2011) found that precrash maneuvers might contribute to head injuries to restrained children in frontal impacts. An evasive emergency maneuver prior to impact can place the occupant in a suboptimal restraint condition that may affect the injury outcome should a crash occur after the emergency event. Controlled emergency steering events using child volunteers (Bohman, Stockman, et al. Citation2011) and braking events (Stockman, Bohman, Jakobsson, and Brolin Citation2013) showed that an evasive maneuver would be an unstable restraint situation for belted children in the rear seat.
Advancements in restraint systems have primarily been in the front seat, including the development of airbag and seat belt technologies, such as pretensioners and load limiters. In order to advance rear seat safety for complex events such as run-off-road or crashes with a preceding evasive maneuver, potential precrash kinematics of rear-seated child occupants and means of protection need to be further explored. Pre-pretensioners (electrical reversible seat belt pretensioners) have shown effectiveness in reducing forward displacements for front seat occupants in evasive braking maneuvers (Òlafsdòttir et al. Citation2013; Östh et al. Citation2013) and lateral displacement in oblique and lateral low-speed sled tests with child and adult volunteers (Arbogast et al. Citation2012). Arbogast et al. (Citation2012) concluded that the benefit of pretensioning on reduced displacement was achieved through early engagement of the torso by the shoulder belt. Front seat occupant protection in run-off-road events is addressed in production vehicles by using run-off-road detection for triggering an electrical reversible retractor early in the events that together with the seat design help keep the occupant in a controlled position (Jakobsson et al. Citation2015). However, studies on rear seat occupant retention in situations such as run-off-road events are lacking. Hence, the aim of the present study was to investigate the seat belt pre-pretensioner effect on rear-seated child-sized anthropomorphic test devices (ATDs) during 2 different types of run-off-road events.
Method
The study was carried out applying 2 test setups to simulate 2 different types of run-off-road events: a rig test with a complete rear seat of a Volvo XC60 passenger car mounted on a multi-axial robot simulating a sudden and intense road departure into a side ditch and a test setup including a Volvo XC60 passenger car driving in and out of a side ditch on a test track. Potential subsequent rollovers or other types of impacts were not included in the test setups.
Three different ATDs were used: 2 child ATDs, the Q6 and Q10, and a Hybrid III (HIII) 5th percentile female. The HIII 5th percentile female has a similar sitting height and shoulder width as an average 12-year-old (Pheasant and Haslegrave Citation2006). The ATDs were positioned in the left outboard rear seat of a Volvo XC60. With respect to seat belt geometries, the seat belts in the in-vehicle tests were standard Volvo XC60 seat belts, whereas in the rig test setup they were somewhat modified. The shoulder belt outlet in the rig test setup contained a prototype device with a spring element, which enabled a small lateral inboard movement of the shoulder belt when loaded. The ATDs were centralized in an upright position and aligned with the centerline of the seat and the lower back in contact with the vehicle seat back, for each test. The Q6 was seated on the upper position of the 2-stage integrated booster cushion (Jakobsson et al. Citation2007) and the Q10 was positioned on the lower position of the integrated booster cushion. The HIII 5th percentile female was positioned directly on the vehicle seat. The lap belt was placed across the lower part of the pelvis and upper thighs, and the shoulder belt was placed across the clavicle at a mid-shoulder position with the seat belt directly routed to the belt outlet. Any seat belt slack was removed.
The seat belt retractor was equipped with an electrical reversible retractor (pre-pretensioner) with 3 force level settings: low, medium, and high. In addition, reference tests without activation of the pre-pretensioner were run.
Rig test
A robot-controlled test setup was used for the rig tests, simulating occupant kinematics during a road departure sequence into a side ditch. The rear seat of a Volvo XC60 was mounted on a multi-axial robot (ABB S4 C+ auto M2000A; IRB-7600; Figure A1a, see online supplement). The robot was programmed in a repeatable manner to re-create a part of a motion sequence of a complete vehicle test launched at 80 km/h and drifting at an angle of 15° into a ditch (Figure A1b, see online supplement). The ditch was 0.80 m deep with an approximately 30% slope from the road surface. The test method used for front seat occupant retention tests was previously described in Jakobsson et al. (Citation2014, Citation2015). The road departure sequence, recorded by 3-axial rotational angles and 3-axial displacements in the complete vehicle crash test, was translated into a global coordinate system and programmed into the robot.
The road departure motion sequence programmed into the robot represented a lateral and vertical motion sequence with rotation around the longitudinal axis. Figure A2 (see online supplement) shows the motion of the robot arm with example images of the rotation of the seat. Position (a) illustrates the starting position of the event. The initial vertical motion is to build up the vertical velocity of the robot, in order to re-create the motion sequence of the complete vehicle test. Position (b) corresponds to the start of the road departure in the vehicle test (approximately 0.5 s after the start of the robot event). Position (c) is the time of pre-pretensioner activation, position (d) is the maximum rotation of the seat (26°), and position (e) is the end of the event.
A 3-axial gyro and a 3-axial linear accelerometer were mounted on the test rig to track the rig motion. Examples of lateral and vertical accelerations as well as angular velocity around the longitudinal axis are shown in Figures A3–A5, respectively (see online supplement). Upper shoulder belt force was measured at shoulder level. The pre-pretensioner was triggered by a timer, based on information from the complete vehicle test. The trig time corresponds to a certain acceleration/rotation threshold.
Eight test configurations were run, including a variation of ATD sizes, 2 different pre-pretension forces (medium and high), and no pre-pretension (inactivated). One test configuration, the HIII 5th percentile female with inactivated pre-pretensioner, was repeated 3 times, in order to investigate the repeatability of the test setup. In total, 10 tests were conducted.
Three high-speed cameras were used, providing a front and side view of the complete test rig and a top view of the belt retractor and shoulder of the ATD. The recording rate was 250 frames per second. The front-view camera was used to quantify the lateral motion of the head and upper sternum of the ATDs relative to the starting position centralized in the seat. The relevant frames were imported into TEMA v3.5-012 (Image Systems Citation2017) in order to track the forehead and upper sternum targets to determine maximum lateral head motion during the event. In addition, the kinematic responses and the shoulder belt position on the shoulder and upper body were analyzed. Pictures of the ATDs in maximum lateral position were selected for comparison between the force levels of the pre-pretensioner. Time of maximum lateral displacement and time of potential shoulder belt slip-off were noted.
In-vehicle test
A series of tests was conducted at a closed test track to study a vehicle driving 20 km/h into a side ditch with a grass slope, driving in the ditch, and driving up from the ditch. The driving sequence is illustrated in . The Volvo XC60 was equipped with a robot driving device (Anthony Best Dynamic Ltd., Wiltshire, UK). The test track sequence was programmed into the steering robot by driving the car manually while recording the Global Positioning System positions of the car.
A photocell mounted on the outside of rear left fender of the vehicle was used to provide the seat belt retractor with a trig signal, used to activate the pre-pretensioner. The seat belt retractor was activated when the vehicle started to drive off the road and down into the side ditch. This occurred between images (a) and (b) in . Nine tests were run, including a variation of ATD sizes, 2 different pre-pretension forces (low and medium), and no pre-pretension.
Figure 2. From left to right: Maximum inboard position of the Q6, Q10, and HIII 5th percentile female with the pre-pretensioner inactivated (top) and pre-pretensioner activation of medium force (middle) and high force (bottom). The Q6 was not tested with the high pre-pretensioner force (bottom left).
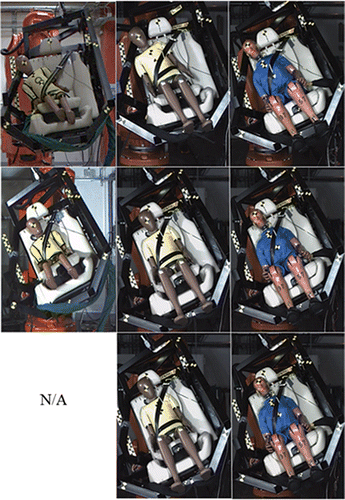
Figure 3. Maximum inboard lateral motion of head for the Q6, Q10, and HIII 5th percentile female (left to right) with the pre-pretensioner inactivated (top) and with low (middle) and medium (bottom) pre-pretension activated.
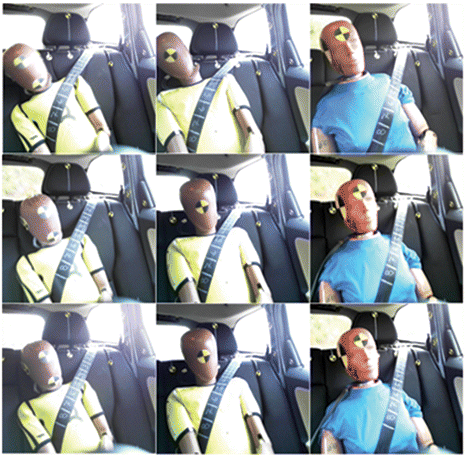
A camera was mounted inside the vehicle facing the left outboard rear seat, where the ATDs were placed. With the objective to evaluate the shoulder belt position relative to the ATD shoulder during the inboard movement at the end of the event, the video was analyzed to determine maximum inboard lateral motion of the head of the ATD. No effort was made to quantify the exactz positions.
Results
Rig test
In all tests, the ATD moved inboard due to the combined rotational, lateral, and vertical motion as shown in Figure A2. The amount of ATD head lateral movement in relation to the seat varied depending on ATD size and activation level of the pre-pretensioner. In each test, locking of the seat belt occurred during the initial vertical motion. In tests with pre-pretensioner activation, the shoulder belt was retracted up to 100 mm and moved up to 50 mm inboard at the outlet. Relative to the shoulder, the inboard motion of the shoulder belt was minor due to the start of motion of the ATD. The activation of the pre-pretensioner occurred at the start of road departure and corresponded to the start of lateral inboard motion of the ATD. In the test with the pre-pretensioner inactivated, the shoulder belt moved with the shoulder of the ATD, resulting in up to 30 mm pull-out of the belt due to the film spool effect.
The maximum lateral head and sternum displacements relative to the seat are shown in . When comparing displacement for each ATD it was found to be substantially reduced when the pre-pretensioner was activated compared to when it was inactivated. The displacements for the Q10 and HIII 5th percentile female were reduced as the force level of the pre-pretensioner increased; however, the differences between the medium and high force levels were small in comparison to when inactivated. Maximum inboard displacement occurred earlier for all ATDs when the pre-pretensioner was activated.
Table 1. Maximum lateral displacement (mm) relative to the seat for head and sternum and corresponding time (s).
shows maximum lateral inboard head position comparing tests with the pre-pretensioner inactivated and the 2 force levels of the pre-pretensioner (medium and high). Overall, the lateral displacement of the ATD was smaller for tests with the pre-pretensioner, and the pre-pretensioner helped retain the shoulder belt on the shoulder. As can be seen in , the shoulder belt slipped off the Q6 and Q10 when the pre-pretensioner was inactivated, whereas it stayed on the shoulder when activated. Shoulder belt slip-off occurred for the Q6 and Q10 at 1.85 and 1.94 s, respectively. For the HIII 5th percentile female, the shoulder belt did not slip off the shoulder in any of the tested configurations although it settled somewhat further out on the shoulder when the pre-pretensioner was inactivated. The HIII 5th percentile female was tested 3 times in the same test setup. The results for lateral head displacement were 210, 240, and 210 mm for tests 1–3, respectively. and show the results from test 3.
The peak shoulder belt force ranged from 0.23 to 0.27 kN with medium force level of the pre-pretensioner and 0.45 to 0.48 kN in the tests with high pre-pretensioner activation. With medium pre-pretensioner activation the shoulder belt force increased with increased ATD size: from 0.23 kN for the Q6, 0.25 kN for the Q10, and 0.27 kN for the HIII 5th percentile female. When there was no activation, the maximum shoulder belt force was less than 0.04 kN.
Figure A6 (see online supplement) shows the initial seat belt geometry for the 3 ATDs from a side view. A greater amount of the shoulder belt was in contact with the lower torso for the Q10 and HIII 5th percentile female compared to the Q6. Furthermore, the head and the shoulders of the HIII 5th percentile female is further forward than for the Q6 and Q10, resulting in a different “grabbing effect” of the shoulder belt.
In-vehicle test
Maximum inboard lateral head displacement occurred at the end of the motion sequence when the vehicle was driving up from the ditch and the vehicle rolled to the right, as shown in .
shows the ATD in maximum inboard lateral motion for all the tests. In tests with the pre-pretensioner inactivated, the lateral displacements were more pronounced, whereas when the pre-pretensioner was activated, lateral displacements were reduced. As can be seen in , shoulder belt slip-off did not occur for the Q6 and Q10. In this test series, shoulder belt slip-off was only seen for the HIII 5th percentile female while the pre-pretensioner was inactivated.
Discussion
Complex events such as run-off-road situations account for many injuries in real-world traffic. Research suggests that evasive maneuvers and complex events occurring prior to an impact can place children in a nonoptimal position in relation to the seat belt, which likely will affect the protection of the child during a crash (Bohman, Arbogast, and Bostrom Citation2011). This study provides data on the kinematics of child-sized ATDs during 2 types of run-off-road events and evaluates the influence of a potential countermeasure. Specifically, the effect of activation of a seat belt pre-pretensioner is shown for child-sized ATDs in the outboard rear seat of a modern passenger car. In the simulated run-off-road tests (rig tests), the activation of a pre-pretensioner resulted in substantial reduced lateral excursion for all of the tested ATD sizes due to the shoulder belt remaining on the shoulder, retaining the torso. The same principles were seen in the run-off-road driving in side ditch test setup (in-vehicle tests).
Prior studies in the area of evasive maneuvers for rear seat occupants have included evasive braking and steering events (Bohman, Stockman, et al. Citation2011; Stockman, Bohman, Jakobsson, and Brolin Citation2013). These events are frequent (Stockman Citation2016) and thus important. The child occupant kinematics in the steering events showed similar kinematics as in the present study with lateral inboard motion and shoulder belt slip-off (Bohman, Stockman, et al. Citation2011). The present study provides a complement to the steering maneuvers. Specifically, 2 different types of run-off-road situations were selected: a sudden and intense road departure at 80 km/h, exposing the left-positioned ATD to rapid inboard lateral loads, and a run-off-road at 20 km/h driving in a side ditch, exposing the left-seated ATD to an inboard movement when entering the road again after driving in the ditch. The combination of these 2 different situations will increase knowledge on different contexts of evasive maneuvers, adding variances in kinematics and time durations, which are important aspects when designing countermeasures.
In the rig tests, the activation of a pre-pretensioner at medium force level resulted in reduced head lateral excursion by 240, 170, and 90 mm of the Q6, Q10, and HIII 5th percentile female, respectively, compared to the inactive pre-pretensioner. This was due to the shoulder belt remaining on the shoulder, retaining the torso. Occupant retention was a combination of several factors. During the pre-pretensioning, the shoulder belt was retracted up to 100 mm. This was likely the most dominant factor. Another factor in the rig test setup was that the modified shoulder belt outlet enabled a lateral inboard movement. This could potentially also have influenced the occupant retention and contributed to the positive results of the pre-pretensioner in the study. However, the effect of shoulder belt outlet inboard movement on occupant retention was neutralized because the activation of the pre-pretensioner corresponded to the start of lateral inboard motion of the ATD. In addition, at that point in time, the ATD had the shoulder belt on the shoulder and had not moved laterally out of the protective restraint zone. In the in-vehicle test setup, when the shoulder belt outlet was fixed in position, the importance of the seat belt retraction was confirmed. In addition, the pre-pretensioner was activated before driving into the side ditch and hence before the motion of the ATDs started. Further exploration of seat belt design factors, including trig time of the pre-pretensioner, is encouraged.
In the rig tests, the maximum inboard displacement for the 3 ATDs when the pre-pretensioner was activated ranged from 60 to 120 mm for the head and 40 to 90 mm for the sternum. In the tests where the pre-pretensioner was inactivated, the variation was greater (maximum head displacement range: 210–340 mm), probably due to the different designs of the ATDs and the influence of how the ATDs were restrained by the seat belt. In addition to the ATD seated height, the depth of the torso and the distance between the back of the head and the head restraint, as well as the interaction between the shoulder belt and the torso of the ATDs, influenced the results. Visual analysis of the views from 3 directions highlighted the importance of the shoulder belt supporting the lower torso/side of the abdomen for reduced lateral displacement.
A pre-pretensioner is designed to be active during the whole emergency event. The greatest slack reduction normally takes place during the initial activation of the pre-pretensioner, reducing the initial slack in the belt system. The pre-pretensioner also removes the film spool effect of the retractor once it is activated. In the tests with inactivated pre-pretensioner, the slack due to the film spool effect was seen during the initial lateral inboard motion, contributing to the less optimal restraining of the upper torso. Because the pre-pretensioner continuously tightens the belt after it has been activated, any additional slack occurring during the event will be removed. A continuous retraction provides benefits to the occupant in complex events.
Based on the initial purpose of the robot used in the rig tests (industrial assembly application), with a precision requirement of repeatability in millimeter positioning, re-creation of seat movements was essentially identical in all of the tests. The variability in the results was mainly influenced by the precision in ATD positioning, seat belt positioning, as well as the accuracy of the tracking analysis. For the configuration with 3 repetitions (HIII 5th percentile female with pre-pretensioner inactivated), the results ranged from 210 to 240 mm when comparing the maximum lateral head movement. This variability (30 mm) is deemed acceptable for the purpose of this study, comparing the overall difference of the pre-pretensioner capacity of supporting the seat belt to remain on the shoulder during the event while activated and inactivated.
The repeatability of the in-vehicle test has not been evaluated in detail. There are several influencing factors in the test setup that will affect vehicle kinematics. For instance, occupant kinematics may vary somewhat due to the tests being conducted on a gravel road and in a grass-covered ditch whereby inherent variations may cause the surface to move slightly. Furthermore, due to the lack of muscular response in the ATDs and their inability to return to the initial position after being exposed to loads, there was a small variation in seated posture and position of the seat belt at the time when the vehicle returned from the ditch resulting in inboard motion of the ATDs. For this reason, this substudy was qualitatively analyzed only comparing maximum lateral displacement and shoulder belt position on the shoulder by visual inspection of the videos. Nevertheless, the effect of the pre-pretensioner on reduced lateral inboard motion of the ATDs was apparent and it is obvious that the activation of the pre-pretensioner has a potential to help keep the child ATD in a more upright position by assisting the shoulder belt staying on the shoulder during the whole event.
This study used one rear seat environment only. Several aspects are likely to affect the kinematics of the ATD. In the in-vehicle test setup, the rear seat was covered in fabric. It is possible that leather would reduce the friction between the seat and the ATD, possibly influencing the lateral motion of the ATD. In addition, the influence of backrest shape and initial seat belt geometry, including type and shape of the booster, might influence the lateral motion of the ATD but was not included in this study. Hence, the conclusions in this study were drawn based on the limitations in the test setup.
The occupant tools used in this study were ATDs developed for crash testing, which poses limitations when used in situations as in this study. Although not developed for evasive maneuvers, the analysis in this study stays within the capacity of the ATDs as shown by Stockman (Citation2016) due to the design of the test setups and the method of analysis. To some extent, depending on severity, a human would be able to maintain position by activing the muscles. However, Bohman, Stockman, et al. (Citation2011) found that younger children could not compensate for a lateral steering maneuver even though they tried to. Taking evasive maneuvers into account when designing protective systems creates new requirements for available physical and numerical test tools. The challenges include occupant kinematics to be predicted for a longer period of time and the influence of muscle activation in nonimpact situations. Human body models could be such a tool, if developed to incorporate the capacity of recreating occupant kinematics in evasive maneuvers and complex events, in addition to the injury prediction capacity in crashes. Future studies are encouraged to incorporate the use of such models to further evaluate the benefits of a seat belt pretensioner and its capacity to help achieve good occupant retention through different types of events, in addition to understanding the influence of other measures such as seat structure, booster types, and belt geometries. The present study illustrates the importance of regarding the protection of children in the rear seat from a wider perspective, including the influence of the precrash event.
Studies have shown that children's self-selected seated postures when riding in cars are affected by several factors (Andersson et al. Citation2010; Jakobsson et al. Citation2011; Osvalder et al. Citation2013). Substantial inboard or forward tilting often occurred periodically as the child was reaching for something, interacting with the parent in the driver's seat, or looking out the window (Jakobsson et al. Citation2011; Osvalder et al. Citation2013). The present study did not take into account less optimal belt geometries or self-selected seated postures resulting in the shoulder belt settling far out on the shoulder or not on the shoulder at all. Although not investigated here, it may be assumed that the capacity of the pre-pretensioner to reduce the lateral motion is less when the initial shoulder belt geometry is poor due to reduced retaining of the torso by the seat belt.
This study provides new insights into the potential benefits of a pre-pretensioner to retain rear seat occupants in complex emergency events. The real effect is influenced by several aspects such as initial seat belt geometry and shoulder belt position throughout the event, seat geometry, and body size of the occupant. Douglas et al. (Citation2007) exposed adult volunteers and ATDs to a lateral force of 1 g to characterize factors influencing occupant-to–seat belt interaction in a far-side impact. They observed that the likelihood of the shoulder engaging the seat belt was increased when the D-ring was moved rearward and the pretension of the seat belt was increased. Their results also highlighted that the occupant-to-seat belt interaction is dependent on occupant anthropometry and that how well the torso is restrained by the belt is highly influenced by torso shape and shoulder depth. For optimal protection it is important to restrain the clavicle throughout the precrash phase as well as in the crash.
In the simulated run-off-road tests, the activation of a pre-pretensioner resulted in reduced lateral head excursion of the Q6, Q10, and HIII 5th percentile female. This was due to the shoulder belt remaining on the shoulder, which was a combination of tightening the belt and the lower torso being supported by the shoulder belt. The results of this study show the potential of countermeasures to improve real-world protection of children in cars by pretensioning the seat belt, helping to keep the child in a good position during complex events.
gcpi_a_1312000_sm7707.pdf
Download PDF (1,010.6 KB)Acknowledgments
The authors thank Henrik Nilsson and Maria Wimmerstedt at Volvo Cars and Mats Svensson at Chalmers University of Technology.
Funding
This study was carried out at SAFER–Vehicle and Traffic Safety Centre at Chalmers, Sweden, and partly financed by FFI (Strategic Vehicle Research and Innovation) by VINNOVA, the Swedish Transport Administration, the Swedish Energy Agency, and the industrial partners, Sweden.
References
- Andersson M, Bohman K, Osvalder A-L. Effect of booster seat design on children's choice of seating positions during naturalistic riding. Ann Adv Automot Med. 2010;54:171–180.
- Arbogast K, Chen I, Durbin D, Winston F. Injury risks for children in child restraint systems in side impact crashes. Paper presented at: IRCOBI Conference; September 22–24, 2004; Graz, Austria.
- Arbogast KB, Mathews EA, Seacrist T, et al. The effect of pretensioning and age on torso rollout in restrained human volunteers in far-side lateral and oblique loading. Stapp Car Crash J. 2012;56:443–467.
- Bohman K, Arbogast K, Bostrom O. Head injury causation scenarios for belted, rear-seated children in frontal impact. Traffic Inj Prev. 2011;12:62–70.
- Bohman K, Stockman I, Jakobsson L, Osvalder AL, Bostrom O, Arbogast KB. Kinematics and shoulder belt position of child rear seat passengers during vehicle maneuvers. Ann Adv Automot Med. 2011;55:15–26.
- Douglas C, Fildes B, Gibson T, Boström O, Pintar F. Factors influencing occupant-to–seat belt interaction in far-side crashes. Annu Adv Automot Med. 2007;51:319–339.
- Durbin D, Elliott M, Winston F. Belt-positioning booster seats and reduction in risk of injury among children in vehicle crashes. JAMA. 2003;289:2835–2840.
- Hillerdal I. Passenger Cars in Fatal Single Vehicle Accidents on Swedish Roads. Uppsala, Sweden: Uppsala University; 2011. UPTEC STS11011.
- Howard A, Rothman L, McKeag A, et al. Children in side-impact motor vehicle crashes—seating positions and injury mechanisms. J Trauma. 2004;56:1276–1285.
- ImageSystemsAB. Available at: http://www.imagesystems.se/index.php/tema/. Accessed April 18, 2017.
- Jakobsson L, Axelson A, Björklund M, Nilsson P, Victor T. Run Off Road Safety. Paper presented at: 24th International Technical Conference on the Enhanced Safety of Vehicles (ESV); June 8–11, 2015; Gothenburg, Sweden. ESV Paper No. 15-0424.
- Jakobsson L, Bohman K, Stockman I, Andersson M, Osvalder A-L. Older children's sitting postures when riding in the rear seat. Paper presented at: IRCOBI Conference; September 14–16, 2011; Krakow, Poland.
- Jakobsson L, Lindman M, Axelson A, Lokensgard B, et al. Addressing run off road safety. SAE International Journal of Passenger Cars - Mechanical Systems. 2014;7(1):132–144.
- Jakobsson L, Wiberg H, Isaksson-Hellman I, Gustafsson J. Rear Seat Safety for the Growing Child—A New 2-Stage Integrated Booster Cushion. Paper presented at: 20th International Technical Conference on the Enhanced Safety of Vehicles (ESV); June 18–21, 2007; Lyon, France. ESV Paper No. 07-0322.
- McCray L, Brewer J, Paciulan K. Protection of children in the rear seat in real world crashes. Paper presented at: NHTSA Meeting; May 10, 2006.
- NHTSA. Traffic Safety Facts 2006, Data, Children. Washington, DC, Author;: 2006. DOT HS 810 803.
- Olafsdóttir JM, Östh J, Davidsson J, Brolin K. Passenger kinematics and muscle responses in autonomous braking events with standard and reversible pre-tensioned restraints. Paper presented at: IRCOBI Conference; September 11–13, 2013; Gothenburg, Sweden.
- Östh J, Olafsdóttir JM, Davidsson J, Brolin K. Driver kinematic and muscle responses in braking events with standard and reversible pre-tensioned restraints: validation data for human models. Stapp Car Crash J. 2013;57:1–41.
- Osvalder AL, Hansson I, Stockman I, Carlsson A, Bohman K, Jakobsson L. Older children's sitting postures, behavior and comfort experience during ride—a comparison between an integrated booster cushion and a high-back booster. Paper presented at: IRCOBI Conference; September 11–13, 2013; Gothenburg, Sweden.
- Pheasant S, Haslegrave C. Bodyspace: Anthropometry, Ergonomics and the Design of Work. 3rd ed. New York: Taylor & Francis; 2006.
- Statistisches Bundesamt. Fachserie 8 Reihe 7, Verkehrsunfälle. 2011. Available at: https://www.destatis.de/GPStatistik/servlets/MCRFileNodeServlet/DEHeft_derivate_00011362/2080700117004.pdf. Available at: http://www.who.int/violence_injury_prevention/publications/road_traffic/world_report/summary_en_rev.pdf Accessed April 18, 2017.
- Stockman I. Safety for Children in Cars—Focus on Three Point Seatbelts in Emergency Events [doctoral thesis]. Gothenburg, Sweden: Chalmers University of Technology; 2016.
- Stockman I, Bohman K, Jakobsson L. Kinematics and shoulder belt position of child anthropomorphic test devices during steering manoeuvres. Traffic Inj Prev. 2013;14:797–806.
- Wang JS, Knipling RR. Single Vehicle Roadway Departure Crashes: Problem Size Assessment and Statistical Description. 1994. DOT HS 808113. Available at: https://ntl.bts.gov/lib/jpodocs/repts_te/1720.pdf. Accessed April 18, 2017.
- World Health Organization. World Report on Road Traffic Injury Prevention: Summary. 2004. Available at: http://www.who.int/violence_injury_prevention/publications/road_traffic/world_report/summary_en_rev.pdf Accessed April 18, 2017.