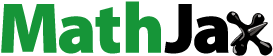
Abstract
Objective
The objective of this study was the quantitative evaluation and comparison of the responses of the Hybrid III 5th percentile female (HIII-05F) and the 5th percentile female Test Device for Human Occupant Restraint (THOR-05F) anthropomorphic test devices (ATDs) subjected to abdominal loading conditions.
Method
The HIII-05F and THOR-05F were subjected to 3 different abdominal loading conditions: fixed-back belt pull (low compression), fixed-back belt pull (high compression), and free-back rigid bar impact at 6 m/s. The stroke of the impact was controlled to represent injurious and noninjurious loading conditions as observed in the experiments with postmortem human subjects (PMHS). Quantitative comparisons were made between the ATD abdominal force and compression responses and biofidelity corridors obtained from matched-pair PMHS tests under identical loading conditions, using the most recent version of the NHTSA Biofidelity Ranking System (BRS).
Results
The overall THOR-05F BRS scores across all tests (BRS score = 1.84) indicated good biofidelity. For the belt loading test conditions, the average BRS scores for both THOR-05F (BRS scores = 1.45 and 1.34) and HIII-05F (BRS scores = 1.42 and 1.01) showed good biofidelity. For the rigid bar loading condition, the THOR-05F (BRS score = 2.74) showed better biofidelity compared to HIII-05F (BRS score = 10.63), with the HIII-05F exhibiting poor performance in this condition. The average pressures recorded by the abdomen pressure twin sensors (APTS) in the current study ranged from 45 to 130 kPa, increasing proportionally with higher stroke and loading rate.
Conclusions
Overall, the THOR-05F BRS scores were better than the HIII-05F BRS scores, which suggests improved biofidelity of the THOR-05F abdomen. The abdominal insert in the HIII-05F did not provide enough room for compression, leading to higher stiffness and occupant motion as observed in the rigid bar tests. Because of practical challenges in measuring abdomen deflection in a soft ATD abdomen component, use of APTS in THOR-05F provides the ability to measure the restraint loading to the abdomen and assess the risk of abdominal injury. With good BRS scores observed in this study for THOR-05F, pressure and other measurements included in the THOR-05F may be used to develop abdominal injury risk functions in the future.
Introduction
Abdominal injuries are among the most common types of Abbreviated Injury Scale 4+ injuries in frontal, farside, and nearside crashes according to a study of the NASS-CDS (Klinich et al. Citation2010). The study found that the abdomen, head, and thorax represented the 3 most frequently and severely injured body regions in motor vehicle crashes.
To better understand injury tolerance or mechanisms of injury, several studies have provided impact and injury responses of the abdomen under various loading conditions, often using postmortem human subjects (PMHS) or animal models. However, the subjects included in many of these studies were predominantly male, with a focus on understanding mid-size male injury response and tolerance. Studies have suggested that females may generally be at greater risk of Abbreviated Injury Scale 2+ and 3+ injuries compared to males in similar crash scenarios (Kahane Citation2013; Parenteau et al. Citation2013; Forman et al. Citation2019). In terms of abdominal injuries, it is unclear whether the suggested higher risk to female occupants is related to a lower injury tolerance, a mechanism of injury due to their preferred seated posture (closer to the steering wheel), or an increased submarining risk due to a combination of smaller stature and poor belt fit (Fong et al. Citation2016). These are important factors to determine whether or not the abdominal response corridors used for anthropomorphic test devices (ATD) representing male and female occupants should be different. Female PMHS- and ATD-specific testing needs to be conducted to better understand the mechanism of abdominal injury.
Measuring the risk of abdominal injuries with ATDs is a challenge from both construction and instrumentation standpoints. It is difficult to accurately represent the viscoelastic, heterogeneous nature of the human abdomen and organ mobility in response to loading. It is also a challenge to develop viable instrumentation to measure any penetration in such a soft component. Additionally, a lack of a biofidelic response may lead to inaccurate conclusions while evaluating motor vehicle safety systems. To evaluate and improve upon the biofidelity of an ATD abdomen, it is desirable to compare data from ATD tests to published response corridors using PMHS under identical test conditions. Additionally, an ATD should be capable of measuring parameters that are relevant to prediction of injury when subjected to crash loads.
The standard Hybrid III 5th percentile female (HIII-05F) ATD includes an abdominal insert that is made of urethane foam covered with a vinyl skin and fills the space in the pelvis. It is somewhat elliptical in shape and has no abdominal measurement capabilities. Because of such practical challenges in measuring abdomen deflection in a soft ATD abdomen component, abdomen pressure twin sensors (APTS) were developed as part of the Enabling Protection for Older Children project for the Q‐series child ATDs to measure restraint loading to the abdomen (Beillas et al. Citation2012; Lemmen et al. Citation2013). These APTS in the abdomen are included in the design of the 5th percentile small female Test Device for Human Occupant Restraint (THOR-05F), allowing pressure measurement during loading, which in previous studies has shown to correlate with abdominal injuries (Kremer et al. Citation2011; Ramachandra et al. Citation2016; Suntay et al. Citation2021).
Due to limited sex- and size-specific data, both HIII-05F and THOR-05F ATDs have often been evaluated against response data that have been scaled from 50th percentile male tests. However, these scaled reference corridors use simplified scaling techniques (Eppinger et al. Citation1984; Mertz et al. Citation2001) that do not account for anatomical differences between males and females. For instance, the average male pelvis is reported to be generally taller and narrower than the female pelvis (Wang et al. Citation2004). In fact, a study by Hwang et al. (Citation2020) using finite element human body models suggested that accounting for size and shape effects is necessary to predict human responses accurately. Furthermore, the simplified scaling techniques were not explicitly developed for use in predicting abdomen response.
Recently, small female PMHS abdomens were subjected to seat belt and bar loading conditions and biofidelity response corridors were generated (Ramachandra et al. Citation2022). A total of nine unembalmed PMHS, with an average mass and stature of 51 ± 8 kg and 163 ± 9 cm, respectively, were subjected to one of two mid-abdominal loading scenarios: fixed-back belt pull at 4 m/s and free-back rigid bar impact at 6 m/s. Abdomen biofidelity response corridors for fixed-back belt pull and free-back rigid bar loading were created to evaluate and improve the biofidelity of small female ATDs. In two of the nine tests, ruptured kidneys and tears in the jejunum, duodenum, and splenic capsule were present. The other seven tests resulted in no organ injury. The organ injuries observed therein were similar to those in comparable tests (Hardy et al. Citation2001; Lamielle et al. Citation2008) with mid-sized male subjects. However, the belt loading response showed higher stiffness in small female subjects compared to the mid-sized male subjects. This phenomenon may be attributed to engagement of lower ribs or pelvis in small subjects with less gap between the inferior aspect of rib cage and anterior–superior iliac spine.
The objective of this study was to evaluate and compare the abdominal response biofidelity of HIII-05F and THOR-05F ATDs in the same seat belt and bar loading conditions presented in Ramachandra et al. (Citation2022). A biofidelic abdomen would allow for realistic belt interaction and submarining behavior and the development of injury risk functions using measurement capabilities of the THOR-05F.
Methods
Both a HIII-05F and a THOR-05F were subjected to two fixed-back seat belt loading conditions (low and high compressions) and one free-back rigid bar loading condition. Three repeat tests were conducted in each condition for each ATD, as shown in the test matrix (Table A1, see online supplement). These conditions were identical to the PMHS tests described in Ramachandra et al. (Citation2022), allowing the ATD results from the current study to be compared to the biomechanical corridors generated therein. The mass and seated abdominal depths of both ATDs were very close to the PMHS average dimensions (Table A2, see online supplement).
Table 1. Average BRS scores (BRS) and phase shift values (DPS) for all tests.
Fixed-back belt loading test setup
The seat belt loading device, similar to the one presented in Lamielle et al. (Citation2008), was used to simulate a belted occupant in a frontal crash. The device used a pneumatic piston to pull a seat belt into the abdomen of a PMHS or ATD in a controlled, dynamic scenario. The test fixture utilized pressurized nitrogen to propel a piston with a 4 inch. bore and 10 inch. stroke rearward. This piston was connected to a T-bar mechanism, which also featured attachment points for a standard seat belt. As the piston pulled the T-bar rearward, the seat belt was driven into the abdomen of each PMHS. The T-bar had a mass of 9.1 kg positioned in front of a load cell and the seat belt, with standard 46 mm webbing, was attached to the T-bar using adjustable clamps to account for various abdomen widths. The T-bar was oriented left to right and positioned behind the specimen in an “out” starting position. It was guided backwards by Teflon bushings in steel tubing when fired. Both sides of the seat belt were kept parallel to each other at a distance equal to the seated abdomen width of the ATD during the event.
Each ATD was seated on a table with the back upright against support plates used to arrest spinal motion. The seat belt was wrapped around the anterior and lateral aspects of the abdomen at the mid-abdomen level corresponding approximately to the umbilicus of a PMHS. Although such loading may not be completely representative of lap belt loading in a motor vehicle crash environment, the location was chosen to maximize the interaction of the belt with the abdomen to characterize the response of the abdomen without interference from the rib cage or pelvis. Initial belt tension was adjusted so that each seat belt load cell measured 15 to 20 N to ensure a repeatable initial position of the belt. Prior to impact, the arms were lifted to shoulder level to ensure that they would not interfere with the movement of the ATD. The legs were splayed slightly outward in a natural seated position. Pretest positioning in the belt pull test apparatus is shown in and the schematics of the setup are provided in Figure B1 (see online supplement).
Like the PMHS study, the fixed-back belt pull tests were divided into low-compression (LC) and high-compression (HC) conditions. This was done by limiting the intended compression of the abdomen to around 27% of the seated abdomen depth for the LC tests and 50% for the HC tests.
Free-back rigid bar loading test setup
In the test series with a rigid bar, each ATD was seated in a free-back upright configuration with the impact aimed at the mid-abdomen at the level of the umbilicus. Pretest positioning of the ATDs in the rigid bar impact test apparatus is shown in . The impactor loading device included a 2.5 cm diameter, 30 cm wide rigid bar attached to the ram shaft by means of a six-axis load cell to measure impact force. Total mass of the impactor was 23 kg, scaled down from 46 kg used in Hardy et al. (Citation2001) to accommodate small subjects. The impact speed of the bar was approximately 6 m/s.
The ATDs were seated on a Teflon skid. The feet of the THOR-05F had to be placed higher than the table due to fixture constraints. However, this did not affect the desired positioning of the thoracic spine, h-point, or thigh angle. Additional Teflon and boot covers were introduced to eliminate any friction effect under the feet. Whereas the arms of the HIII-05F were able to stay up for the event, support structures were used to keep the THOR-05F’s arms away from the impact path without influencing the overall kinematics. Strips of conductive tape were placed on both the ATD jacket and the impactor face. When contact between conductive tapes occurred, the data acquisition system was triggered and time zero was defined. A total of three tests per ATD were conducted in this configuration. These tests were designed to not induce injuries by restricting the ram stroke to a length of approximately 27% of the ATD’s seated abdominal depth.
Instrumentation and data processing
Data were acquired at a sampling frequency of 20,000 Hz using TDAS G5 or SLICE PRO, and all signals were filtered according to standard SAE J211 (2014 version).
In the HIII-05F, an accelerometer was attached to the lumbar spine and aligned with the laboratory x-axis, which was parallel to the line of motion of the impactor. For the THOR-05F, data from the T12 6DX block and APTS were collected. Data from the 6DX motion blocks were transformed to the laboratory coordinate system. The x-axis accelerations (αx) from the lumbar accelerometer for HIII-05F and T12 accelerometer for THOR-05F were double integrated to obtain the displacement of the ATD to measure the compression of the abdomen.
Time zero for the tests was set when a relevant force measurement, which differed depending on the test condition, reached 50 N. Force applied to the abdomen in the belt pull tests was calculated as the sum of two tension seat belt load cell forces. In the rigid bar tests, the x-axis impact force (the force parallel to the line of motion of the impactor) was measured using a six-axis load cell installed between the ram shaft and the impactor face. The impact force data recorded were compensated to account for the mass in front of the load cell. Abdomen penetration (δAbd) was defined as the deflection of the abdomen versus time, where deflection is measured at the point anterior to the abdomen at the sagittal midline. In the belt loading tests, abdomen penetration was equal to belt penetration (δBelt) measured by an anterior string potentiometer (EquationEq. (1)(1)
(1) ). For the rigid bar tests, abdomen penetration was calculated by subtracting the displacement of the lumbar spine at the level of the impact from the displacement of the ram (δRam) measured via a linear potentiometer upon contact (EquationEq. (2)
(2)
(2) ). Abdomen compression was calculated by dividing abdominal penetration by seated abdominal depth (225 mm for both HIII-05F and THOR-05F).
(1)
(1)
(2)
(2)
Biofidelity assessment
To quantitatively assess the similarity of ATD responses to the PMHS-based corridors (mean ±1 SD), objective BioRank scores were calculated using the recently updated NHTSA Biofidelity Ranking System (BRS; Hagedorn et al. Citation2022). Each ATD response curve was assessed for shape and magnitude biofidelity.
First, the dummy phase shift (DPS), defined as the amount of time shift required to optimally align the phase of the ATD response curve with that of the mean PMHS response curve, was calculated. For this, the dummy cumulative absolute difference (DCAD) and the cadaver cumulative standard deviation (CCSD) were first calculated. To calculate the CCSD, the 1 SD values from the PMHS mean curve were summed. DCAD was the sum of the absolute differences between the mean PMHS curve and the ATD response curve. In this study, the ATD curve was shifted in time repeatedly until the minimum DCAD was found.
With the ATD curve now aligned to the mean PMHS curve, the BRS score was calculated (BRS score = DCAD/CCSD). Because the ATD tests in this study were quite similar to the represented PMHS tests, the time over which the BRS scores were calculated included relevant data in an event. For instance, in the LC belt pull tests and the rigid bar tests, the time over which the BRS scores were calculated included data from time 0 ms to the time at which 27% compression was achieved. In addition to reporting the BRS scores, the DPSs required to align the data meaningfully are reported as additional information.
Results
BRS scores and the DPS for each test are reported in the corresponding plots and the averages for each loading condition are reported in . Additionally, average BRS score and average DPS for force and compression responses from each test condition along with PMHS phase shift values are reported in (see online supplement).
Any BRS score over two indicates that the ATD response varied from the mean PMHS by more than two standard deviations (SD) on average. As a general guideline, scores of two or less may be considered good biofidelity. The BRS calculation range included time 0 to peak value of the corresponding PMHS response because this was considered the meaningful part of the event. The ATD force and compression responses were shifted using a minimum DCAD alignment method, because this resulted in the best alignment of the ATD response phase to that of the mean PMHS response. The time histories as displayed in are the result of shifting the individual time histories by the calculated DPS.
The overall THOR-05F BRS scores across all tests (BRS score = 1.84) indicated good biofidelity (). For the LC belt loading test conditions, the average BRS scores for both THOR-05F (BRS score = 1.45) and HIII-05F (BRS score = 1.42) showed good biofidelity. Similarly, in the HC belt loading conditions, good biofidelity was observed for both THOR-05F (BRS score = 1.34) and HIII-05F (BRS score = 1.01). The amount of shift required to best align the signals for BRS calculations was less than 7 ms. For the rigid bar loading condition, the THOR-05F (BRS score = 2.74) showed better biofidelity compared to the HIII-05F (BRS score = 10.63), with the HIII-05F exhibiting poor performance in this condition.
The abdominal compression and belt forces were calculated from the LC fixed-back belt pull tests for both ATDs ( and ). Neither the HIII-05F nor the THOR-05F abdomens compressed as much as the PMHS for the same applied stroke. However, the THOR-05F responses were closer to the lower boundary of the PMHS target than the HIII-05F, leading to better BRS scores. The HIII-05F peak force values were higher than the PMHS mean force, whereas the THOR-05F peak values were much lower.
Figure 2. Abdominal compression–time histories from LC belt loading tests; H3-5F in blue and T5F in red.
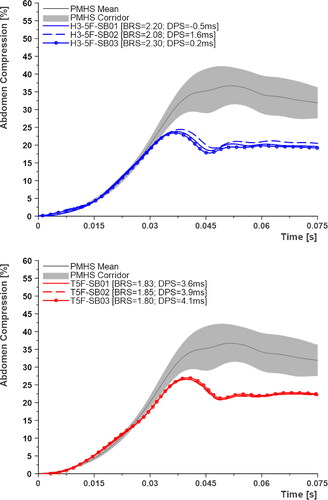
Abdominal compression and belt forces were reviewed for the HC tests ( and , respectively). Both the HIII-05F and THOR-05F followed the PMHS compression closely; however, the THOR-05F had a greater peak compared to both the HIII-05F and PMHS. Unlike the gradual increase of belt force observed in the PMHS, both ATDs displayed low initial forces followed by a rapid rise, reaching peak values higher than the PMHS. However, the HIII-05F did marginally better in staying within the corridors, giving it a slightly better BRS score (1.2 vs. 1.5).
Figure 4. Abdominal compression–time histories from HC belt loading tests; H3-5F in blue and T5F in red.
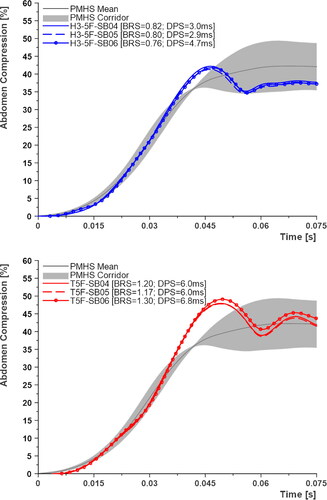
The abdominal compression and force responses were also compared for the rigid bar loading tests ( and ). Although both ATDs closely followed the PMHS corridors, neither ATD achieved the same maximum compression as the PMHS corridor. It must be noted here that in the PMHS tests, the ram displaced beyond the stopper set at 27% of the abdomen depth due to the damping mechanism (70 durometer Sorbothane) used to stop the impactor. The final ram stop was a combination of the Sorbothane and the stiffness of the abdomen. Because the overshoot occurred later in the event after the maximum force was recorded and did not cause any injuries, the data were considered usable. A somewhat similar phenomenon was seen in the test with THOR-05F where there was some overshoot beyond the intended compression.
Figure 6. Abdominal compression–time histories from rigid bar loading tests; H3-5F in blue and T5F in red.
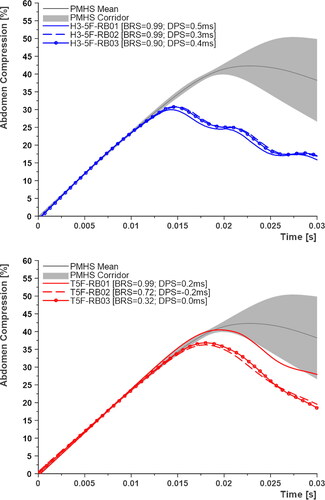
The peak average pressures (Pmax) measured in the APTS of the THOR-05F are reported in Table C2 (see online supplement). Additionally, calculated peak rates of pressure change, Ṗmax, which has been shown to correlate well with abdominal injuries (Kremer et al. Citation2011; Ramachandra et al. Citation2016), are reported in Table C2.
Discussion
This study evaluated the biofidelity of small female ATDs, specifically the HIII-05F and THOR-05F. For the purposes of biofidelity evaluation, ATD tests were conducted and matched to PMHS exposure conditions described in Ramachandra et al. (Citation2022). This study is unique in that rather than comparing these small female ATD responses to scaled data from 50th percentile male to 5th percentile female, the responses were compared with small-sized PMHS response corridors developed using the same conditions and apparatus.
In the fixed-back belt pull tests, the belt was wrapped around the abdomen at the mid-abdominal level. Because the HIII-05F does not include a continuous suit like the THOR-05F, the lower part of the belt was not in contact with the jacket that ends approximately at the mid-abdominal level. The abdominal insert for the HIII-05F, made of urethane foam covered with vinyl skin, simply fills the space in the pelvis and does not encompass the upper abdomen. As the belt was thrusted into the ATD, rather than compressing the abdomen, the top of the abdomen insert was pinched by the lower part of the belt. Though this resulted in good biofidelity in this study, it highlights a limitation of the HIII-05F design in that it may not be ideal in identifying abdominal injuries due to submarining because the belt may travel into the gap between the rib cage and the abdomen. The design and construction of the THOR-05F, however, allowed for the belt to remain on the anterior surface of the abdominal insert and not result in unintended penetration.
Unlike the HIII-05F and THOR-05F, in the LC belt pull tests with the PMHS, the softer abdomen allowed the desired penetration to be achieved easily and exceeded due to inertial effects. The response differences may have resulted from both energy dissipated by the piston assembly and stiffness of the abdomen. Further testing is planned where the belt and specimen are replaced with a nondeformable mass in pull tests to exclude the abdominal characteristic as a variable.
The force–compression responses from the belt pull tests are shown in . The stiffness, calculated as a ratio of peak force and corresponding abdominal penetration, of the HIII-05F was observed to be higher when compared to the PMHS in the LC tests, whereas the stiffness of the THOR-05F was lower than that of the PMHS. In the HC tests, the overall stiffness was higher for both ATDs, although they exhibited a softer initial response compared to the PMHS. Upon reaching approximately 30% compression, there was a sudden increase in stiffness until reaching the peak force. Even though a preload of 15 to 20 N was applied on the belt to remove any slack, there was little to no initial belt penetration due to the preload. The delayed onset of loading in the THOR-05F () might include effects due to any gap between the jacket and abdomen anteriorly or between the abdomen and spine structure posteriorly.
Figure 8. Comparison of force–compression responses between HIII-05F, THOR-05F, and PMHS corridors: LC belt loading tests (top), HC belt loading tests (middle), and rigid bar loading tests (bottom).
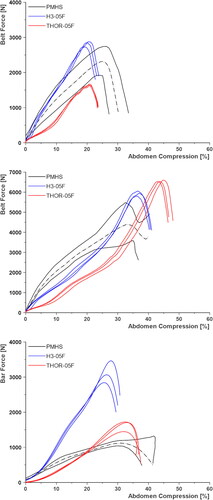
The force–compression responses from the rigid bar tests are also shown in . Unlike the belt pull tests, the back was not constrained in the rigid bar impact tests. The HIII-05F experienced much higher loads and exhibited a stiffer response compared to the PMHS and the THOR-05F. After an initial compression of approximately 20%, the HIII-05F moved backwards, which may be due to the combination of abdominal stiffness and a frictionless surface. The THOR-05F exhibited a softer initial response than the PMHS similar to what was observed in the belt pull tests. However, there was an increase in force from 20% to 30% compression, possibly due to mass recruitment as the foam compressed against the posterior structure. The postimpact behavior of the THOR-05F was similar to that of the PMHS, where after the event the torso underwent extension rather than the whole ATD sliding backwards.
In the LC belt loading tests, the HIII-05F abdomen resulted in a higher magnitude of force, whereas the THOR-05F was lower in comparison with the PMHS. The combination of lower force and higher compression in the THOR-05F indicates a softer front part of the abdomen compared to the HIII-05F. The stiffer nature of the HIII-05F abdomen was also evident in the rigid bar tests where the impactor was brought to a complete halt at the desired compression level in the tests with HIII-05F, resulting in a higher magnitude of force compared to the PMHS and THOR-05F.
The overall stiffness of the THOR-05F abdomen was greater in belt pull tests compared to rigid bar tests, as was found for the PMHS. This difference is likely because the back was constrained in the belt pull tests, and the belt distributed the load on the abdomen anterolaterally with little to no mobility of the ATD. Whereas in the rigid bar tests, in addition to no posterior constraint, the impact area on the abdomen was smaller due to the shape of the impactor, allowing the abdomen to expand laterally. However, this distinction could not be made in the HIII-05F tests (i.e., similar stiffness in all test conditions) for reasons described previously.
The APTS in the THOR-05F provide an opportunity to measure restraint loading to the abdomen and potentially assess the risk of abdomen injury. Recently, Suntay et al. (Citation2021) used internal APTS pressure and external penetration responses along with pediatric biomechanical data to generate a pressure-based abdominal injury risk function for the Large Omni-Directional Child (LODC) ATD. With THOR-05F demonstrating good biofidelity in the current study, using data from injurious and noninjurious matched-pair tests between the PMHS and THOR-05F, a pressure-based injury risk function may be constructed for small female abdominal injury prediction in motor vehicle crashes.
In summary, the biofidelity of the HIII-05F and THOR‐05F small female ATDs was evaluated under 3 abdominal loading conditions in this study. Using the most recent version of NHTSA’s BRS, the THOR-05F displayed good biofidelity (BRS score < 2) in most channels across all test conditions. The average BRS scores were considered good for both the HIII-05F and THOR-05F in both belt loading conditions. In the rigid bar loading condition, the THOR-05F performed better (BRS score = 2.74) than the HIII-05F (BRS score = 10.63). There were a few areas where the THOR-05F differed from the PMHS corridors, such as initial stiffness of the response (softer compared to PMHS) in all loading conditions and rapid increase in force when compressed beyond 30% of abdomen depth. Despite some of these differences, the good BRS scores observed in this study for the THOR-05F suggest that pressure and other measurements may be used to develop abdominal injury risk functions in the future.
Supplemental Material
Download Zip (1.7 MB)Additional information
Funding
References
- Beillas P, Alonzo F, Chevalier M-C, Lesire P, Leopold F, Trosseille X, Johannsen H. 2012. Abdominal twin pressure sensors for the assessment of abdominal injuries in Q dummies: in-dummy evaluation and performance in accident reconstructions. Stapp Car Crash J. 56:387–410. doi:10.4271/2012-22-0010.
- Eppinger RH, Marcus JH, Morgan RM. 1984. Development of Dummy and Injury Index for NHTSA’s Thoracic Side Impact Protection Research Program. SAE Trans. 93:359–387.
- Fong CK, Keay L, Coxon K, Clarke E, Brown J. 2016. Seat belt use and fit among drivers aged 75 years and older in their own vehicles. Traffic Inj Prev. 17(2):142–150. doi:10.1080/15389588.2015.1052420.
- Forman J, Poplin GS, Shaw CG, McMurry TL, Schmidt K, Ash J, Sunnevang C. 2019. Automobile injury trends in the contemporary fleet: belted occupants in frontal collisions. Traffic Inj Prev. 20(6):607–612. doi:10.1080/15389588.2019.1630825.
- Hagedorn A, Stammen J, Ramachandra R, Rhule H, Thomas C, Suntay B, Kang Y-S, Kwon HJ, Moorhouse K, Bolte Iv JH, et al. 2022. Biofidelity evaluation of THOR-50M in rear-facing seating configurations using an updated Biofidelity Ranking System. SAE Int J Trans Safety. 10(2):291–375. 10.4271/09-10-02-0013
- Hardy WN, Schneider LW, Rouhana SW. 2001. Abdominal impact response to rigid-bar, seatbelt, and airbag loading. Stapp Car Crash J. 45:1–32. doi:10.4271/2001-22-0001.
- Hwang E, Hu J, Reed MP. 2020. Validating diverse human body models against side impact tests with post-mortem human subjects. J Biomech. 98:109444. doi:10.1016/j.jbiomech.2019.109444.
- Kahane CJ. 2013. Injury vulnerability and effectiveness of occupant protection technologies for older occupants and women. Report No. DOT HS 811 766. Washington (DC): National Highway Traffic Safety Administration.
- Klinich KD, Flannagan CA, Nicholson K, Schneider LW, Rupp JD. 2010. Factors associated with abdominal injury in frontal, farside, and nearside crashes. Stapp Car Crash J. 54:73–91. doi:10.4271/2010-22-0005.
- Kremer MA, Gustafson HM, Bolte JH, 4th, Stammen J, Donnelly B, Herriott R. 2011. Pressure-based abdominal injury criteria using isolated liver and full-body post-mortem human subject impact tests. Stapp Car Crash J. 55:317–350. doi:10.4271/2011-22-0012.
- Lamielle S, Vezin P, Verriest JP, Petit P, Trosseille X, Vallancien G. 2008. 3D deformation and dynamics of the human cadaver abdomen under seatbelt loading. Stapp Car Crash J. 52:267–294. doi:10.4271/2008-22-0011.
- Lemmen PP, Waagmeester K, Burleigh M, Lakshminarayana A, Korschdon K, Visvikis C, Carroll J, Hynd D, Pitcher MJ. 2013. Development of the Q10 10 year-old child crash test dummy. 23rd International Technical Conference on the Enhanced Safety of Vehicles (ESV), Seoul, South Korea.
- Mertz HJ, Jarrett K, Moss S, Salloum M, Zhao Y. 2001. The hybrid III 10-year-old dummy. Stapp Car Crash J. 45:319–328. doi:10.4271/2001-22-0014.
- Parenteau CS, Zuby D, Brolin K, Svensson MY, Palmertz C, Wang SC. 2013. Restrained male and female occupants in frontal crashes: are we different?. 2013 IRCOBI Conference, Gothenburg, Sweden.
- Ramachandra R, Kang Y-S, Bolte JH, Hagedorn A, Herriott R, Stammen JA, Moorhouse K, 4th. 2016. Biomechanical responses of PMHS subjected to abdominal seatbelt loading. Stapp Car Crash J. 60:59–87. doi:10.4271/2016-22-0004.
- Ramachandra R, Kang YS, Stammen J, Lee E, Moorhouse K, Bolte J, IV. 2022. Biomechanical responses of small female PMHS subjected to abdominal loading. 2022 IRCOBI Conference, Porto, Portugal.
- Suntay B, Carlson M, Stammen J, Ramachandra R. 2021. Abdominal and thoracic injury risk functions for the large omni-directional child (LODC) ATD. 2021 IRCOBI Conference, Held virtually.
- Wang SC, Brede C, Lange D, Poster CS, Lange AW, Kohoyda-Inglis C, Sochor MR, Ipaktchi K, Rowe SA, Patel S, et al. 2004. Gender differences in hip anatomy: possible implications for injury tolerance in frontal collisions. Annu Proc Assoc Adv Automot Med. 48:287–301.