ABSTRACT
Asthma and chronic obstructive pulmonary disease (COPD) are heterogeneous diseases with different inflammatory phenotypes. Various inflammatory mediators play a role in these diseases. The aim of this study was to analyze the neutrophilic and eosinophilic airway and systemic inflammation as the phenotypic characterization of patients with asthma and COPD. Twenty-four patients with asthma and 33 patients with COPD were enrolled in the study. All the patients were in mild-to-moderate stage of disease, and none of them were treated with inhaled corticosteroids. Concentrations of IL-6, neutrophil elastase (NE), matrix metalloproteinase 9 (MMP-9), eosinophil cationic protein (ECP), and IL-33 and IL-17 in serum and induced sputum (IS) were measured by enzyme-linked immunosorbent assay (ELISA). The cellular composition of blood and IS was evaluated. Hierarchical clustering of patients was performed for the combination of selected clinical features and mediators. Asthma and COPD can be differentiated based on eosinophilic/neutrophilic systemic or airway inflammation with unsatisfactory efficiency. Hierarchical clustering of patients based on blood eosinophil percentage and clinical data revealed two asthma clusters differing in the number of positive skin prick tests and one COPD cluster with two subclusters characterized by low and high blood eosinophil concentrations. Clustering of patients according to IS measurements and clinical data showed two main clusters: pure asthma characterized by high eosinophil/atopy status and mixed asthma and COPD cluster with low eosinophil/atopy status. The neutrophilic phenotype of COPD was associated with more severe airway obstruction and hyperinflation.
Introduction
Despite a number of important differences in the pathogenesis, course, and prognosis of asthma and chronic obstructive pulmonary disease (COPD), both diseases share some common features. These include airway inflammation and remodeling that lead to airway obstruction. Although, in general, the nature of the airway inflammation in asthma and COPD patients differs considerably Citation(1,2), there are some inflammatory changes that can be observed in both diseases. This particularly refers to the mild stages of asthma and COPD Citation(3) and certain disease phenotypes, e.g., non-allergic Th1-mediated asthma in the elderly, which might have very similar characteristics to COPD Citation(4). Albeit airway eosinophilia and remodeling are hallmarks of asthma Citation(5), in some patients, non-eosinophilic inflammation is present Citation(6,7). Neutrophilic asthma is a distinct asthma phenotype with poor steroid response and evidence of systemic inflammation Citation(8), both of which are common features of COPD. Neutrophils are considered the main inflammatory cells in COPD, but as many as 20–40% of COPD patients may have elevated sputum eosinophil count and airway eosinophilic infiltration Citation(9,10). This, however, does not predict good response to inhaled steroids, even though treatment with systemic steroids may be more effective in patients with eosinophilic COPD than in those with the non-eosinophilic phenotype Citation(11–13). There is no doubt that the ultimate aim of the research undertaken to better and more precisely define the nature of asthma and COPD is the improvement in treatment efficacy. Therefore, determination of the type of airway inflammation in asthma and COPD can be useful not only in terms of differentiation between both entities but also in the prediction of response to treatment Citation(14).
In the aforementioned context, the studies on inflammatory phenotypes, including those evaluating different inflammatory biomarkers in asthma and COPD seem to have potential practical applications.
The neutrophilic airway inflammation is characterized, among others, by increased level of matrix metalloproteinase 9 (MMP-9), neutrophil elastase (NE), interleukin 6 (IL-6), and IL-17 that may not only serve as markers of inflammatory process, airway remodeling, and lung damage Citation(15,16) but also as biomarkers of response to therapy Citation(17). On the other hand, markers of eosinophilic inflammation include eosinophil cationic protein (ECP) and IL-33. ECP reflects eosinophilic airway infiltration and was reported to be more closely related to lung function parameters than eosinophil counts Citation(18). Interleukin 33 is regarded as one of the most important cytokines responsible for Th2 immune deviation Citation(19).
It has been shown that cytokine profile of sputum determined distinct and overlapping asthma and COPD clusters, which supported both the British and Dutch hypotheses on pathogenesis of obstructive airway diseases Citation(20). According to a new interesting concept on therapeutic strategies in obstructive airway diseases, the management of asthma and COPD should be based on clinical, functional, biological, and imaging features of the disease and focus on “treatable traits” reflecting the needs of the individual patient rather than numerical indices such as forced expiratory volume in 1 second (FEV1) Citation(21).
Since, according to numerous earlier studies, the type of airway inflammation is an important factor that determines the clinical features and the course of disease, we undertook a study to characterize asthma and COPD using the cellular and biochemical markers of eosinophilic and neutrophilic inflammation. The specific aims of the study were as follows: 1) to evaluate and compare the cellular composition and concentration of selected inflammatory mediators (IL-6, ECP, NE, IL-17, IL-33, MMP-9) in serum and induced sputum (IS) from patients with mild-to-moderate asthma and COPD; 2) to evaluate the relationship between the level of various cytokines and clinical and laboratory data, with special regard to cellular phenotypes; and 3) to cluster the asthma and COPD based on selected inflammatory phenotype markers and clinical features to differentiate the distinct clusters of the disease.
Materials and methods
Study design
This was a prospective, cross-sectional study performed between 2012 and 2014, which involved 24 asthmatics and 33 patients with COPD. The patients were recruited from the pulmonary outpatient department of the University Hospital in Warsaw. All patients underwent the following evaluations: medical history, physical examination, spirometry (with flow-volume curve), body plethysmography, bronchial obstruction reversibility test, methacholine bronchial challenge, allergy skin prick tests, the evaluation of the absolute and percentage cell count in peripheral blood and IS. The number of exacerbations in the past year was registered; disease control was assessed by Asthma Control Test (ACT) in asthmatics and by the COPD Assessment Test (CAT) in patients with COPD.
All the participants signed an informed consent. The study project was approved by the institutional review board and registered at ClinicalTrial.gov (NCT02069054).
Definitions and exclusion/inclusion criteria
The diagnosis of asthma and its severity were established in accordance with the Global Initiative for Asthma Citation(22); the diagnosis of COPD was made in accordance with the Global Initiative for Chronic Obstructive Lung Disease Citation(23).
Following criteria had to be met to be included in the asthma study group: 1) signs and symptoms consistent with asthma, 2) spirometric features of airway obstruction with positive bronchial reversibility test, and 3) a positive result of methacholine challenge test (PC20 < 8 mg/mL). Inclusion criteria of the COPD group were as follows: 1) signs and symptoms consistent with COPD with onset after the age of 40 years, 2) a positive smoking history (>10 pack-years), and 3) evidence of bronchial obstruction (FEV1/FVC <5th percentile) in post-bronchodilator spirometry.
Bronchial obstruction reversibility and airway hyperresponsiveness were tested according to the European Respiratory Society (ERS) and American Thoracic Society recommendations, respectively Citation(24). Reversible airflow limitation was defined as post-bronchodilator FEV1/FVC >5th percentile of predicted value, while persistent (“fixed”) airway obstruction as post-bronchodilator FEV1/FVC <5th percentile, regardless of positive (i.e., FEV1 and/or FVC increase of both >200 mL and >12% of predicted) or negative bronchial reversibility test. The severity of airflow limitation was evaluated in accordance with the ERS guidelines Citation(25). Patients with cigarette smoke exposure less than 10 pack-years were considered as nonsmokers, and patients who had smoked in the past but denied smoking in 1 year before the study onset were defined as ex-smokers. Atopy was defined as the presence of at least one positive skin prick test to common allergens, with a diameter of 3 mm or greater than the negative control Citation(26). The atopic status was stratified according to number of positive skin prick test (SPT) result.
Exclusion criteria for all subjects were disease exacerbation or symptoms of respiratory tract infection in the previous 6 weeks. Patients who had received inhaled or oral corticosteroids within 6 weeks prior to enrollment were also excluded.
Sputum induction and processing
Sputum induction was preceded by inhalation of 400 µg of salbutamol and subsequent pulmonary function testing. After the post-bronchodilator spirometry, the patients inhaled sterile hypertonic saline (NaCl) at increasing concentrations (3%, 4%, and 5% solutions) at room temperature via an ultrasonic nebulizer (ULTRA-NEB ™ 2000, DeVilbiss, USA). The duration of each inhalation was 7 minutes, and the induction was stopped after the decrease of FEV1 by at least 20% from baseline (post-bronchodilator) FEV1 value.
The sputum samples were processed and examined within 2 hours after induction, according to the ERS recommendations Citation(27). The volume of the sputum was measured, and the plugs were separated and weighed. A freshly prepared 0.1% solution of dithiothreitol (DTT, Sigma-Aldrich, USA) was added in a volume equal to four times the weight of the sputum, and the mixture was shaken for 15 minutes. Subsequently, a double volume of phosphate-buffered saline (PBS) was added, and the mixture was vortexed briefly. After filtration through two layers of a sterile gauze, the sputum was centrifuged for 10 minutes at 1800 g. The cells were counted, and the percentage of dead cells and epithelial cells was assessed. The supernatants were stored at −70°C for further investigation. The differential cell count (the percentage of eosinophils, neutrophils, macrophages, and lymphocytes) was determined in May-Grünwald-Giemsa-stained smears based on the morphology of 300 cells from various fields. Eosinophilic airway inflammation was defined as IS eosinophil percentage ≥ 3%, while neutrophilic inflammation was defined as IS neutrophil percentage ≥ 60% Citation(28).
Blood cell count evaluation
White blood cell count was measured using Sysmex XN-2000 (Sysmex Corporation, Kobe, Japan). The differential cell count was evaluated manually in May-Grünwald-Giemsa-stained smears.
IL-6, ECP, NE, IL-17, IL-33, MMP-9 concentration measurement
The measurements of cytokine concentrations in serum and IS supernatants were performed with ELISA kits for: IL-6, IL-17, IL-33, MMP-9 (R&D Systems, Minneapolis, USA), ECP, and NE (EIAab, Wuhan, China) according to the manufacturer's instructions. The mean minimal detectable concentration of the tests was: 0.7 pg/mL for IL-6, 15 pg/mL for IL-17, 0.519 pg/mL for IL-33, 0.156 ng/mL for MMP-9, 0.156 ng/mL for ECP, and 78 pg/mL for NE. In the preliminary study, the evaluation of any possible effects of DTT on the cytokines during sputum processing was performed according our previous protocol Citation(29). The level of evaluated protein standards reconstituted in PBS-DTT dilution buffer did not differ from those solutes in recommended diluents; the final protein measurements were made without DTT addition.
Statistical analysis
Sample size estimation
Estimation of the sample size was based on data from an earlier study by Grubek-Jaworska et al. Citation(29). We assumed that the difference between sputum IL-6 level in asthma and COPD, which should be detected, would be at least twofold lower than that found by Grubek-Jaworska et al. Citation(29). To detect the difference with a power of 80% and a significance level of 5%, the sample size was estimated as 47 patients (19 asthmatics and 28 COPD patients).
Data presentation and statistical analysis
Statistical analyses were performed using Statistica 12.0 software (StatSoft Inc., Tulsa, USA). Data are presented as median and interquartile range (IQR). Differences between continuous variables were tested using nonparametric Mann–Whitney U test. Fisher's exact test was used to test the differences between nominal variables. Correlations between variables were analyzed with Spearman's rank test. Differences were considered statistically significant at p < 0.05. Missing values were imputed with predictive mean matching method implemented in “mice” package Citation(30) with data set including all variables as starting matrix. Receiver operating characteristic (ROC) curves were constructed, and the area under ROC curve (AUC) was calculated as a measure of diagnostic accuracy of different biomarkers. To assess combined predictive potential of measured markers, a logistic regression (implemented in “stats” package) was used. Prediction from logistic regression was used to plot ROC. Hierarchical clustering of asthma and COPD patients was performed on scaled data, using the complete method with Euclidean distance as the dissimilarity measure. Heatmaps and dendrograms were generated with “stats” package Citation(31). A single set of clinical and laboratory features (including differential cell count, as well as, cytokine concentration in blood/serum and IS samples) was evaluated in each patient. In figures presenting hierarchical clustering, each column corresponds to one patient. Computations were performed in R environment Citation(31).
Results
Patient characteristics
Comparative characteristics of patients with asthma and COPD are presented in . Mild persistent asthma was diagnosed in 19 (79%) asthmatics and moderate asthma in the remaining 5 (21%) asthma patients. Mild and moderate airflow limitation (consistent with GOLD 1 and GOLD 2 stage) was diagnosed in 10 (30%) and 23 (70%) COPD patients, respectively. The median score in ACT was 20 points (14–23.5), and in the CAT, it was 14 points Citation(7–18), respectively.
Table 1. Clinical characteristics of patients with asthma and chronic obstructive pulmonary disease participating in the study.
Cells and cytokine concentration in blood/serum and IS
The only difference observed in serum cytokine levels between the investigated groups was higher IL-6 level in COPD patients compared to asthmatics ().
Table 2. Mediator concentrations and cell numbers in serum/blood and induced sputum from asthma and COPD patients.
More differences were found for the biomarker concentrations in IS (). IS levels of IL-6, MMP-9, and NE were higher in COPD patients than those in asthmatics. No significant differences between asthma and COPD patients in terms of IS IL-17, IL-33, and ECP concentration were observed.
Asthma patients had increased eosinophil number and percentage in blood compared to COPD patients. Sputum eosinophils were significantly higher in asthmatics than in COPD patients. Sputum neutrophils were significantly higher in COPD patients compared to those in asthmatics (). Correlations between blood and IS eosinophils and blood and IS neutrophils in asthmatics and COPD patients are demonstrated in .
Figure 1. Correlations between blood and sputum eosinophils (red triangles) and blood and sputum neutrophils (black circles) in asthma and COPD patients.
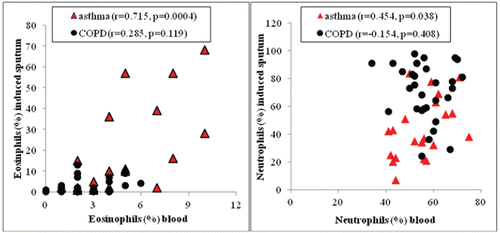
Subanalysis of asthma and COPD patients with and without IS eosinophilia showed higher blood eosinophil percentage in asthma: 2.5% (IQR, 2–3.5), n = 11 vs. 5% (IQR, 4–8), n = 13, p = 0.03 for non-eosinophilic and eosinophilic, respectively, and in COPD 2% (IQR, 1–3), n = 23 vs. 4% (IQR, 2–5), n = 10, p = 0.027 for non-eosinophilic and eosinophilic, respectively.
ROC analysis of the discriminative value of serum and sputum cytokine levels and neutrophil and eosinophil concentrations in differentiation between asthma and COPD
All evaluated biomarkers showed moderate or low sensitivity and specificity in discrimination between asthma and COPD (). The highest diagnostic accuracy for differentiation between asthma and COPD was demonstrated for IS neutrophil percentage, IS eosinophil percentage, and serum IL-6 (AUC = 0.82, 0.79, and 0.75, respectively). The combined ROC curve (labeled “model”) had the best discriminative value for both serum/blood (AUC = 0.766) and IS (AUC = 0.878) evaluation. The optimal cutoff points for model curve analysis were: 0.596 for serum/blood (sensitivity 83%, specificity 66%) and 0.867 for IS (sensitivity 74%, specificity 80%).
Cluster analysis
Clustering yielded the most promising results for the combination of clinical features and mediators as presented below.
Clustering of patients according to peripheral blood eosinophil percentage, FEV1%, FEV1/VC, RV%, pack-years, and atopy revealed three main clusters (A: patients 9–11 mainly asthma, B: patients 24–16 asthma, C: patients 46–36 COPD) (). Clusters A and B contained all asthma patients and only one COPD (patient 26), and these asthma clusters differed in the number of positive SPT (higher number in cluster A). The most evident difference between asthma and COPD referred to pack-years, FEV1%, and FEV1/VC. Cluster C, with COPD patients, could be further divided into cluster with high (patients 27–55) and low (patients 38–36) eosinophil blood percentages.
Figure 3. Hierarchical clustering of asthma and COPD patients. Single set of clinical and laboratory data per one patient was used for clustering. (a) Patients clustered according to peripheral blood eosinophil percentage, FEV1%, FEV1/VC, RV%, pack-years, and atopy. (b) Patients clustered according to data from sputum samples (eosinophil and neutrophil percentages, IL-6, NE and MMP-9 concentrations) and clinical data (FEV1/VC, FEV1%, RV%, pack-years, and atopy status). Patient IDs are given at the bottom of the plot. The color scale codes the value of a variable with the brightest shade corresponding to the highest value.
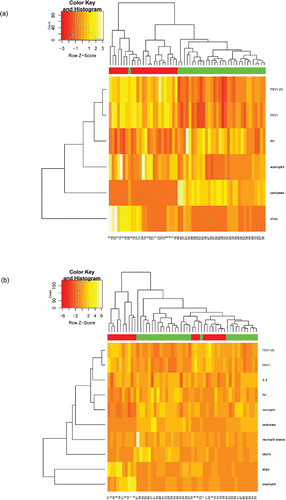
Clustering of patients that was based on data from IS analysis (eosinophil and neutrophil percentages, IL-6, NE and MMP-9 concentrations), as well as clinical data (FEV1/VC, FEV1%, RV%, pack-years, and atopy status), showed two main groups: A—patients 17–3, containing only asthmatics, and B—patients 51–30, mixed asthma and COPD group. Group B could be further divided into five subgroups. Groups B1 (patients 51–49) and B2 (patients 37–42) contained only COPD patients, group 3 (patients 32–23) comprised both asthma and COPD patients, group B4 (patients 7–18) was formed of asthma patients only, while B5 (patients 40–30) was formed of COPD patients only (). The pure asthma clusters: A and B4 subgroup differed predominantly with atopy and eosinophils. The clusters that contained COPD patients only: B1, B2, and B5 were characterized by high MMP-9 concentration (B1), low FEV1%, FEV1/VC, high RV%, and high number of pack-years (B2); the group B5 had higher FEV1%, FEV1/VC, lower RV%, and lower number of pack-years than B2 subcluster.
Discussion
This study, which included a complex analysis of eosinophilic/neutrophilic inflammation in asthma and COPD patients, showed that cytological characteristics may be an important “treatable trait” of these heterogeneous airway diseases. We demonstrated that asthma and COPD can be poorly differentiated based on eosinophilic/neutrophilic systemic or airway inflammation only. There was a significant positive correlation between the percentage of eosinophils, as well as neutrophils in blood and IS in asthmatics, but not in COPD patients. Our study also showed a significant overlap in cytokine concentration in IS and serum of patients with mild-to-moderate asthma and COPD. In cluster analysis, we confirmed that eosinophils and neutrophils are important determinants of phenotypes in asthma and COPD. Hierarchical clustering of asthma and COPD patients according to peripheral blood eosinophil percentage and clinical data revealed two asthma clusters differing in the number of positive SPT and one COPD cluster with two subclusters characterized by low and high blood eosinophil concentrations. Clustering of asthma and COPD patients based on data from IS analysis and clinical data showed two main clusters: pure asthma characterized by high eosinophil/atopy status and mixed asthma and COPD cluster with low eosinophil/atopy status. It should be underlined that our study involved only patients with well-defined mild-to-moderate stages of both diseases and, what seems particularly important, none of the patients were treated with anti-inflammatory agent, inhaled corticosteroids (ICS) that could suppress airway inflammation. Thus, we believe we studied airway inflammation in the natural or near-natural course of both diseases.
Asthma and COPD are complex and multicomponent inflammatory diseases with a wide spectrum of clinical and laboratory features (disease phenotypes). There is evidence that some features of asthma and COPD can overlap in the same patient. This may hinder proper diagnosis. In our study, none of the single biomarkers was found to sufficiently discriminate between asthma and COPD. A combined analysis that included all markers characterizing eosinophilic/neutrophilic inflammation gave slightly better results, but still, they were not specific enough, particularly in terms of IS analysis. We believe these findings reflect the complexity of asthma and COPD. Various clusters of patients with obstructive airway diseases have already been reported in previous studies. For example, Ghebre et al. Citation(20) presented three clusters of patients, distinguished by detailed analysis of sputum cytokines: 1) predominantly asthma (95%) characterized by eosinophil inflammation and high Th2 cytokine concentrations; 2) combined asthma and COPD group with neutrophilic inflammation caused by bacterial colonization, elevated IL-1β level; and 3) preferably COPD group with mixed granulocytic sputum and high IL-6 and CCL-13 levels. The results of our study seem to confirm that single binary differentiation between asthma and COPD carries the risk of missing the most efficient treatment for the patient. The existence of similar phenotypes in asthma and COPD undermines the relevance of the classic distinction between asthma and CODP and suggests a more personalized treatment of obstructive lung diseases based on a variety of their clinical features.
The analysis of blood/serum and IS biomarkers in mild-to-moderate asthma and COPD showed a poor correlation between various features of systemic and local inflammation. The relationship between local and systemic inflammation seems to be a relevant issue that can make an interesting point in analysis of our results. As sputum induction is a time-consuming procedure, the possibility to follow the inflammatory phenotype based on blood testing is an attractive alternative. Our study confirmed a strong positive correlation between the percentage of IS as well as peripheral blood eosinophils and neutrophils in patients with asthma. A similar relationship between sputum and blood eosinophils in asthma was also reported in a recent study by Wagener et al. Citation(32) (r = 0.59; p < 0.001). Likewise, the associations between blood and sputum eosinophils as well as neutrophils in asthma patients were reported by Hastie et al. Citation(33). These authors demonstrated a significant predictive value of blood neutrophils for sputum neutrophilia. Such a relationship was not found for eosinophils. On the other hand, only a weak correlation between neutrophil percentage in sputum and blood from asthma patients was demonstrated in the study by Schleich et al. Citation(7) (r = 0.19, p = 0.0015).
Contrary to asthma, we did not demonstrate a positive correlation between blood and sputum neutrophils in COPD. This is consistent with the results reported by the other authors Citation(7,34). These findings may suggest that the degree of systemic inflammation in COPD is independent of the level of airway neutrophilia. Similar to the lack of relationship between sputum and blood neutrophils in COPD, the same observation was made for eosinophils (in this study group). This was an unexpected finding, as some other studies showed that in COPD blood, eosinophilia can serve as the predictor of sputum eosinophilia and eosinophilic airway inflammation Citation(10,35). The possible explanation for the lack of correlation between blood and sputum eosinophilia observed in our study could be that we investigated stable and mild stages of the diseases. The majority of literature data on the relationship between systemic and airway eosinophilia in COPD were based on studies that involved patients with moderate and severe COPD or patients with COPD exacerbations. On the other hand, the clustering of asthma and COPD patients, which took into account the differential cell count of peripheral blood, showed that the most relevant cellular component differentiating COPD patients was eosinophil percentage. What seems even more interesting is that blood eosinophilic percentage did not differentiate the asthma clusters. The role of eosinophils in the context of COPD course and response to ICS treatment has been well recognized. High blood eosinophilia was shown to be associated with COPD exacerbations Citation(36). The number of blood eosinophils was reported as an informative predictor of positive response to ICS measured as reduction in exacerbation rate Citation(11,37). In our study, the cluster of COPD patients with higher blood eosinophil percentage was characterized by a higher number of pack-years, and lower spirometric test results were compared to the cluster with lower blood eosinophilia. These results may confirm that blood eosinophilia may predict worse prognosis of COPD. Further evaluation of these patients is necessary to confirm this hypothesis.
A principal component analysis of airway inflammation in COPD performed by Roy et al. Citation(38) identified four main components representative for the different pathophysiological processes associated with the disease. The two major components were neutrophilic and eosinopilic airway inflammation; it seems evident that the type of airway inflammation is an important factor in COPD phenotyping. It should be mentioned that then absolute count (and percentage) of sputum neutrophils and eosinophils was the major determinant of the type of airway inflammation. In our study, the percentages of asthma and COPD patients with eosinophilic airway inflammation were 54% and 30%, respectively. Neutrophilic airway inflammation was found in 21% of asthmatics and 63% of COPD patients. It should be noted that the percentage of asthma patients with airway neutrophilia was similar to that previously reported. In a large cohort of asthmatics (n = 508) studied by Schleich et al. Citation(7), 42% of patients presented with airway eosinophilia, while only 16% had airway neutrophilia. In the study by Simpson et al. Citation(39), 41% and 20% of asthmatics were classified as having eosinophilic and neutrophilic airway inflammation, respectively. It is noteworthy that in our study, the percentage of eosinophils was slightly higher than that reported in the aforementioned papers. The differences between the results of our study and the above-cited studies may be related to various factors, including different cutoff values for eosinophilic and neutrophilic inflammation, different patient characteristics (untreated or treated with ICS), and others. Contrary to asthmatics, the distribution of eosinophilic and neutrophilic airway inflammation in our COPD patients was similar to that found in other studies. For example, in the study by Leigh et al. Citation(40), sputum eosinophilia was present in 38% of mild-to-moderate COPD patients (n = 40).
In our study, IS eosinophilia was the variable that showed the strongest influence on asthma and COPD clusterization. This was a major factor that allowed identification of the two main clusters: highly eosinophilic pure asthma group and low eosinophilic mixed asthma and COPD groups. Asthma patients with airway eosinophilic inflammation were characterized by a larger number of positive SPT results. Neutrophilic airway inflammation was a weaker discriminative factor in cluster analysis than sputum eosinophilia. However, airway neutrophilia was higher in subclusters that included only COPD patients than that in other subclusters. In B2 COPD subcluster, higher neutrophil percentage was associated with more severe airway obstruction and hyperinflation. This was opposite to B5 subcluster. Neutrophils are known to be involved in the pathogenesis of COPD and emphysema, through different mechanisms including releasing of proteases (e.g., MMP-9, NE). In the study by Singh et al. Citation(34), sputum neutrophilia was associated with lower FEV1 and worse health status (measured by St. George's Respiratory Questionnaire). In a different study, the massive presence of neutrophil extracellular traps, web-like extracellular structures of decondensed chromatin associated with histones and enzymes (NE and myeloperoxidase), in the sputa of COPD patients correlated with the severity of airflow limitation Citation(41). The results of our analysis suggest that airway neutrophilia is associated with lung function impairment in some subgroups of COPD patients only. Ghebre et al. Citation(20) found that airway neutrophilia was a feature of two clusters. In the first cluster (asthma and COPD overlap group), it was associated with (and was probably a consequence of) bacterial colonization, while in the second cluster (COPD predominant group), it coexisted with eosinophils (mixed granulocytic phenotype) and was classified as a primary abnormality of the disease. Since in our study microbiological analysis of the sputum was not performed, we cannot confront our results with those of Ghebre et al. Citation(20). We believe that bacterial colonization may be an important driving mechanism in different clusters and should be more specifically evaluated in the future.
B1 subcluster was distinguished from others by high MMP-9 level with simultaneous moderate airflow limitation. MMPs are thought to be important factors causing cigarette smoke–induced emphysema. The major sources of MMP-9 are neutrophilic gelatinase and azurophilic granules Citation(42). Its concentration and activation are associated with airway neutrophil count. Sputum MMP-9 level correlated with the extent of emphysema (measured by CT) and lung diffusion capacity (DLco) Citation(43). Other studies showed that MMP-9 concentration correlated with disease severity Citation(44) and increased during COPD exacerbations Citation(45). In fact, MMP-9 was the only mediator highlighted in our cluster analysis and associated with one COPD subcluster, suggesting the importance of this proteolytic enzyme in the pathophysiology of some COPD phenotypes. The selected B1 subcluster contains too small number of patients for more detailed analysis, and further evaluation of this issue might be an interesting field in future studies.
Our study has some limitations. The major flaws are relatively small groups of patients with only two severity stages (mild and moderate) of both diseases. The analysis of larger subgroups with different disease phenotypes and different disease stages could shed more light on the role of various biomarkers in pathogenesis and phenotyping of asthma and COPD. Secondly, we distinguished only two types of airway inflammation—eosinophilic and neutrophilic, instead of four categories (also including pauci- and mixed granulocyte types). This was because the comparative analysis of the smaller subgroups would have resulted in even smaller statistical power. Thirdly, the spectrum of evaluated biomarkers was quite small and focused on markers of eosinophilic/neutrophilic airway infiltration. These mediators could not fully reflect the complexity of airway disease. Thus, more advanced approaches using more comprehensive assessment of inflammatory networks in the airway-like transcriptomics would be more valid. Fourthly, we realize that the use of the number of positive SPTs to quantify atopy status may raise significant doubts. Nevertheless, the result of SPT is easily available and relatively objective feature describing the atopy. It must be remembered, however, that there is no simple relationship between the number of positive SPTs and the severity of atopy.
Conclusions
Our study showed significant overlaps in different cytokine concentrations in IS and serum of patients with mild-to-moderate asthma and COPD. Thus, the accuracy of differentiation between asthma and COPD based on the features of airway inflammation (eosinophilic or neutrophilic) is highly questionable.
Our study provides new evidence on similarities between the mechanisms of airway inflammation, at least in mild-to-moderate stages of asthma and COPD. Hierarchical clustering of patients according to peripheral blood eosinophil percentage and clinical data revealed two asthma clusters and one COPD cluster that could be further subclassified into two subclusters characterized by low and high eosinophil percentages in peripheral blood. Clustering of patients that was based on IS data and clinical features showed two main clusters: pure asthma cluster characterized by high eosinophil percentage and a large number of positive SPTs as well as mixed asthma and CODP cluster with low eosinophil percentage and a small number of positive SPTs. Our study shows that phenotyping of asthma and COPD based on cellular or physiological features may further identify the subgroups of patients with particularly beneficial response to specific and individualized treatment.
Acknowledgments
The authors want to thank Dr. Marta Maskey-Warzęchowska for helping with manuscript preparation.
Funding
The project received financial support from the National Science Centre, Poland (N N402 124940).
Declaration of interest
The authors report no conflicts of interest. The authors alone are responsible for the content and writing of the paper.
ORCID
Magdalena Paplińska-Goryca http://orcid.org/0000-0003-0576-7289
Krzysztof Goryca http://orcid.org/0000-0003-0576-7289
References
- Culpitt SV, Rogers DF, Traves SL, Barnes PJ, Donnelly LE. Sputum matrix metalloproteases: comparison between chronic obstructive pulmonary disease and asthma. Respir Med 2005; 99(6):703–710.
- Fabbri LM, Romagnoli M, Corbetta L, Casoni G, Busljetic K, Turato G, et al. Differences in airway inflammation in patients with fixed airflow obstruction due to asthma or chronic obstructive pulmonary disease. Am J Respir Crit Care Med 2003; 167(3):418–424.
- Górska K, Maskey-Warzechowska M, Krenke R. Airway inflammation in chronic obstructive pulmonary disease. Curr Opin Pulm Med 2010; 16(2):89–96.
- Rufo J, Taborda-Barata L, Lourenço O. Serum biomarkers in elderly asthma. J Asthma 2013; 50(10):1011–1019.
- Holgate ST, Davies DE, Lackie PM, Wilson SJ, Puddicombe SM, Lordan JL. Epithelial-mesenchymal interactions in the pathogenesis of asthma. J Allergy Clin Immunol 2000; 105(2 Pt 1):193–204.
- Simpson JL, McElduff P, Gibson PG. Assessment and reproducibility of non-eosinophilic asthma using induced sputum. Respiration 2010; 79(2):147–151.
- Schleich FN, Manise M, Sele J, Henket M, Seidel L, Louis R. Distribution of sputum cellular phenotype in a large asthma cohort: predicting factors for eosinophilic vs neutrophilic inflammation. BMC Pulm Med 2013; 13:11.
- Wood LG, Baines KJ, Fu J, Scott HA, Gibson PG. The neutrophilic inflammatory phenotype is associated with systemic inflammation in asthma. Chest 2012; 142(1):86–93.
- Saha S, Brightling CE. Eosinophilic airway inflammation in COPD. Int J Chron Obstruct Pulm Dis 2006; 1(1):39–47.
- Eltboli O, Mistry V, Barker B, Brightling CE. Relationship between blood and bronchial submucosal eosinophilia and reticular basement membrane thickening in chronic obstructive pulmonary disease. Respirology 2015; 20(4):667–670.
- Pavord ID, Lettis S, Locantore N, Pascoe S, Jones PW, Wedzicha JA, et al. Blood eosinophils and inhaled corticosteroid/long-acting β-2 agonist efficacy in COPD. Thorax 2016; 71(2):118–125.
- Siddiqui SH, Guasconi A, Vestbo J, Jones P, Agusti A, Paggiaro P, et al. Blood eosinophils: a biomarker of response to extrafine beclomethasone/formoterol in chronic obstructive pulmonary disease. Am J Respir Crit Care Med 2015; 192(4):523–525.
- Pascoe S, Locantore N, Dransfield MT, Barnes NC, Pavord ID. Blood eosinophil counts, exacerbations, and response to the addition of inhaled fluticasone furoate to vilanterol in patients with chronic obstructive pulmonary disease: a secondary analysis of data from two parallel randomised controlled trials. Lancet Respir Med 2015; 3(6):435–442.
- Bafadhel M, McKenna S, Terry S, Mistry V, Pancholi M, Venge P, et al. Blood eosinophils to direct corticosteroid treatment of exacerbations of chronic obstructive pulmonary disease: a randomized placebo-controlled trial. Am J Respir Crit Care Med 2012; 186(1):48–55.
- Rovina N, Koutsoukou A, Koulouris NG. Inflammation and immune response in COPD: where do we stand? Mediators Inflamm 2013; 2013:413735.
- Churg A, Zhou S, Wright JL. Series “matrix metalloproteinases in lung health and disease”: matrix metalloproteinases in COPD. Eur Respir J 2012; 39(1):197–209.
- Lee T-M, Lin M-S, Chang N-C. Usefulness of C-reactive protein and interleukin-6 as predictors of outcomes in patients with chronic obstructive pulmonary disease receiving pravastatin. Am J Cardiol 2008; 101(4):530–535.
- Cianchetti S, Bacci E, Ruocco L, Pavia T, Bartoli ML, Cardini C, et al. Are sputum eosinophil cationic protein and eosinophils differently associated with clinical and functional findings of asthma? Clin Exp Allergy J Br Soc Allergy Clin Immunol 2014; 44(5):673–680.
- Chu DK, Llop-Guevara A, Walker TD, Flader K, Goncharova S, Boudreau JE, et al. IL-33, but not thymic stromal lymphopoietin or IL-25, is central to mite and peanut allergic sensitization. J Allergy Clin Immunol 2013; 131(1):187–200.
- Ghebre MA, Bafadhel M, Desai D, Cohen SE, Newbold P, Rapley L, et al. Biological clustering supports both “Dutch” and “British” hypotheses of asthma and chronic obstructive pulmonary disease. J Allergy Clin Immunol 2015; 135(1):63–72.
- Agusti A, Bel E, Thomas M, Vogelmeier C, Brusselle G, Holgate S, et al. Treatable traits: toward precision medicine of chronic airway diseases. Eur Respir J 2016; 47(2):410–419.
- GINA_Report_2010_1.pdf. February 12, 2016. Available from: http://www.ginasthma.org/local/uploads/files/GINA_Report_2010_1.pdf
- GOLDReport_April112011.pdf. February 12, 2016. Available from: http://www.goldcopd.org/uploads/users/files/GOLDReport_April-112011.pdf
- Miller MR, Hankinson J, Brusasco V, Burgos F, Casaburi R, Coates A, et al. Standardisation of spirometry. Eur Respir J 2005; 26(2):319–338.
- Pellegrino R, Viegi G, Brusasco V, Crapo RO, Burgos F, Casaburi R, et al. Interpretative strategies for lung function tests. Eur Respir J 2005; 26(5):948–968.
- Position paper. Allergen standardization and skin tests. The European Academy of Allergology and Clinical Immunology. Allergy 1993; 48(14 Suppl):48–82.
- Vignola AM, Rennar SI, Hargreave FE, Fah JV, Bonsignore MR, Djukanović R, et al. Standardised methodology of sputum induction and processing. Future directions. Eur Respir J Suppl 2002; 37:51s–55s.
- Berry M, Morgan A, Shaw DE, Parker D, Green R, Brightling C, et al. Pathological features and inhaled corticosteroid response of eosinophilic and non-eosinophilic asthma. Thorax 2007; 62(12):1043–1049.
- Grubek-Jaworska H, Paplińska M, Hermanowicz-Salamon J, Białek-Gosk K, Dąbrowska M, Grabczak E, et al. IL-6 and IL-13 in induced sputum of COPD and asthma patients: correlation with respiratory tests. Respiration 2012; 84(2):101–107.
- van Buuren S, Groothuis-Oudshoorn K. mice: Multivariate imputation by chained equations in R. J Stat Software 2011; 45(3):1–67.
- The R Core Team. R. A Language and Environment for Statistical Computing. 2014. Available from: http://www.R-project.org/
- Wagener AH, de Nijs SB, Lutter R, Sousa AR, Weersink EJM, Bel EH, et al. External validation of blood eosinophils, FE(NO) and serum periostin as surrogates for sputum eosinophils in asthma. Thorax 2015; 70(2):115–120.
- Hastie AT, Moore WC, Li H, Rector BM, Ortega VE, Pascual RM, et al. Biomarker surrogates do not accurately predict sputum eosinophil and neutrophil percentages in asthmatic subjects. J Allergy Clin Immunol 2013; 132(1):72–80.
- Singh D, Edwards L, Tal-Singer R, Rennard S. Sputum neutrophils as a biomarker in COPD: findings from the ECLIPSE study. Respir Res 2010; 11:77.
- Eltboli O, Brightling CE. Eosinophils as diagnostic tools in chronic lung disease. Expert Rev Respir Med 2013; 7(1):33–42.
- Bafadhel M, McKenna S, Terry S, Mistry V, Reid C, Haldar P, et al. Acute exacerbations of chronic obstructive pulmonary disease: identification of biologic clusters and their biomarkers. Am J Respir Crit Care Med 2011; 184(6):662–671.
- Saltürk C, Karakurt Z, Adiguzel N, Kargin F, Sari R, Celik ME, et al. Does eosinophilic COPD exacerbation have a better patient outcome than non-eosinophilic in the intensive care unit? Int J Chron Obstruct Pulmon Dis 2015; 10:1837–1846.
- Roy K, Smith J, Kolsum U, Borrill Z, Vestbo J, Singh D. COPD phenotype description using principal components analysis. Respir Res 2009; 10:41.
- Simpson JL, Scott R, Boyle MJ, Gibson PG. Inflammatory subtypes in asthma: assessment and identification using induced sputum. Respirology 2006; 11(1):54–61.
- Leigh R, Pizzichini MMM, Morris MM, Maltais F, Hargreave FE, Pizzichini E. Stable COPD: predicting benefit from high-dose inhaled corticosteroid treatment. Eur Respir J 2006; 27(5):964–971.
- Grabcanovic-Musija F, Obermayer A, Stoiber W, Krautgartner W-D, Steinbacher P, Winterberg N, et al. Neutrophil extracellular trap (NET) formation characterises stable and exacerbated COPD and correlates with airflow limitation. Respir Res 2015; 16:59.
- Faurschou M, Borregaard N. Neutrophil granules and secretory vesicles in inflammation. Microbes Infect Inst Pasteur 2003; 5(14):1317–1327.
- Chaudhuri R, McSharry C, Spears M, Brady J, Grierson C, Messow CM, et al. Sputum matrix metalloproteinase-9 is associated with the degree of emphysema on computed tomography in COPD. Transl Respir Med 2013; 1(1):11.
- Vlahos R, Wark PAB, Anderson GP, Bozinovski S. Glucocorticosteroids differentially regulate MMP-9 and neutrophil elastase in COPD. PloS One 2012; 7(3):e33277.
- Mercer PF, Shute JK, Bhowmik A, Donaldson GC, Wedzicha JA, Warner JA. MMP-9, TIMP-1 and inflammatory cells in sputum from COPD patients during exacerbation. Respir Res 2005; 6:151.