Abstract
Supervised exercise training (EXT) as part of pulmonary rehabilitation is arguably the most effective intervention for improving exercise tolerance in patients with chronic obstructive pulmonary disease (COPD). In the current review, we focus on the physiological rationale for EXT and the expected physiological benefits that can be achieved in patients who can be exposed to sufficiently high training stimuli. Thus, after a brief consideration of the mechanisms of exercise limitation and their sensory consequences, we expose the potential beneficial effects of EXT on respiratory mechanical and peripheral muscular adaptations to exercise. The available evidence indicates that changes in exertional ventilation, breathing pattern, operating lung volumes and static respiratory muscle strength after EXT are modest and often inconsistent. Inspiratory muscle training may have a role in patients showing inspiratory weakness pre-rehabilitation. Beneficial changes in peripheral muscles can be seen in those who can tolerate higher training intensity, particularly using combined resistance and dynamic (including interval) exercise. It should be recognised, however, that it might not be feasible to reach meaningful physiological training effects in many frail elderly patients with advanced respiratory mechanical and pulmonary gas exchange derangements with serious co-morbidities (such as cardiac and peripheral vascular disease). These potential shortcomings should not discourage the use of pulmonary rehabilitation as an effective strategy to improve patients’ capacity to tolerate physical activity. Currently, the greatest challenge is to develop effective strategies to ensure that these important gains in functional capacity are translated into sustained increases in daily physical activity for patients with COPD.
Introduction
Pulmonary rehabilitation which incorporates supervised exercise training (EXT) is arguably the most effective intervention for improving exercise tolerance in patients with chronic respiratory diseases, such as those suffering from chronic obstructive pulmonary disease (COPD) (Citation1–3). Indeed, influential meta-analyses have confirmed that in spite of broad variability in the nature and composition of the rehabilitation programs, and considerable diversity in population samples enrolled in various studies, consistent improvements in exercise tolerance (measured by various field tests) are achieved when compared with controls (Citation4–6). It is no surprise, therefore, that participation in pulmonary rehabilitation is strongly recommended as a pivotal intervention in symptomatic COPD, (Citation7) regardless of the severity of resting functional impairment (Citation7).
Exercise limitation in chronic respiratory diseases is characteristically multi-factorial (Citation8). Recognised physiological causes include:
impaired pulmonary gas exchange (Citation9–12),
mechanical abnormalities (airways, lung parenchyma, chest wall) (Citation10,Citation13–16),
respiratory and peripheral muscle abnormalities (Citation17,Citation18) and
cardio-circulatory derangements (Citation19–23).
It is important to recognise, however, that patients are ultimately limited by uncomfortable sensations (“symptoms”) which can only be partially explained by physiological abnormalities (Citation24). Thus, the severity of symptoms such as dyspnea or leg discomfort is subject to the modulatory influence of genetic background, past experience, stoicism, peer influence and psychological status which are highly variable on an individual basis (Citation3). It follows that some degree of reductionism is present in any discussion on the “physiology of rehabilitation”. Indeed, important beneficial effects of pulmonary rehabilitation (e.g. general well-being, improved coping strategies, lower dyspnea-induced anxiety) (Citation25,Citation26) are not necessarily linked to measurable physiological changes (Citation27). It should also be recognised that a variety of other factors commonly contribute to poor exercise performance in COPD, including: body mass index, effects of natural aging, deconditioning, musculoskeletal disorders, anemia, and important co-morbidities such as cardiovascular disease (Citation28). Thus, efforts to improve exercise capacity in pulmonary rehabilitation participants requires a recognition of potentially reversible factors that can be targeted for attention. Increasingly, pulmonary rehabilitation programs are becoming populated by the frail elderly with multi-system disease in whom sustained physiological training effects are difficult to achieve. Moreover, physiological and clinical improvements that follow from a supervised program are often not sustained over time in this population who are prone to exacerbations with progressive and often relentless functional decline (Citation29,Citation30).
In the light of these precautionary considerations, the current review focuses on the physiological rationale for EXT as part of pulmonary rehabilitation and the expected physiological benefits that can be achieved in patients with COPD who can be exposed to sufficiently high training stimuli. Thus, after a succinct consideration of the mechanisms of exercise limitation and their sensory consequences, we discuss the potential beneficial effects of EXT on respiratory mechanical and peripheral muscular adaptations to exercise in these patients.
Mechanisms of exercise intolerance in COPD
The relative importance of different mechanisms of exercise intolerance varies markedly depending on the severity of COPD (Citation31,Citation32). Dominant abnormalities in mild disease include () (Citation13–16):
Figure 1. Metabolic and ventilatory responses to exercise. (a) oxygen uptake (V′O2), (b) minute ventilation (V′E)/carbon dioxide production (V′CO2), (c) V′E, (d) respiratory frequency (fR), (e) tidal volume (VT) and (f) operating lung volumes at different work rates in patients with mild COPD and healthy controls. Triangles represent the VT/V′E inflection. Data are presented as mean ± standard error of the mean, *p<.05. EELV: end-expiratory lung volume; EILV: end-inspiratory lung volume; fR: respiratory frequency; TLC: total lung capacity; V′CO2: carbon dioxide production; V′E: minute ventilation; V′O2: oxygen uptake; VT: tidal volume. Reproduced with permission of the © ERS 2019: European Respiratory Journal 44 (5) 1177–1187; DOI:10.1183/09031936.00034714 Published 31 October 2014.
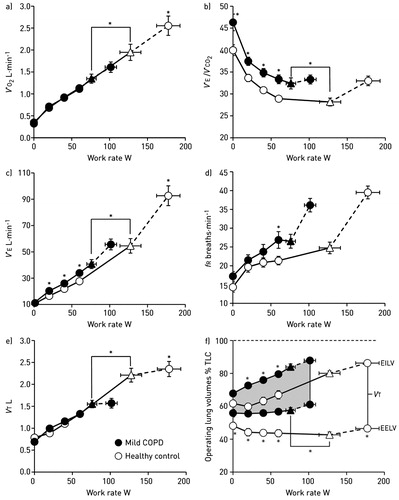
increased chemostimulation of respiratory centers secondary to the effects of high physiological dead space (i.e. inefficient pulmonary gas exchange) and/or low arterial partial pressure for carbon dioxide (PaCO2) set-point, which is reflected by an increased ventilation (V˙E)-CO2 output (V˙CO2) relationship and
increased airways resistance and dynamic hyperinflation due to the combined effects of expiratory flow limitation and increased ventilatory demand.
It follows that a low inspiratory capacity (IC) during exercise [due to increased end-expiratory lung volume (EELV)] (Citation33) associated with higher inspiratory neural drive (secondary to inefficient pulmonary gas exchange and increased mechanical loading) (Citation15) lead to critical mechanical constraints earlier in exercise in mild COPD than age-matched healthy controls (Citation10,Citation13–15,Citation31). In moderate-to-severe COPD (), the progressively increasing intrinsic mechanical loading of the functionally weakened respiratory muscles (due to acute-on-chronic hyperinflation) (Citation34) requires augmented increases in efferent motor drive to achieve a given force generation (Citation10). Additionally, increased reflex stimulation of the central and peripheral chemoreceptors may occur secondary to:
Figure 2. Tidal volume (VT)/dynamic inspiratory capacity (IC) ratio as a function of ventilation during constant work rate exercise in COPD patients showing progressively lower FEV1 values [quartile (Q) 1 to 4] (A). Dyspnea is expressed relative to VT/IC and ventilation (absolute and relative to peak values) in (B), (C) and (D), respectively. Note the clear inflection (plateau) in the VT/ventilation relationship (A), which coincides with a simultaneous inflection in dyspnea (B) across the FEV1 quartiles. FEV1: forced expiratory volume in 1 s; IC: inspiratory capacity; VT: tidal volume. Reprinted from Chest, 141(3), O'Donnell DE, Guenette JA, Maltais F, Webb KA, Decline of resting inspiratory capacity in COPD the impact on breathing pattern, dyspnea, and ventilatory capacity during exercise, page 760, Copyright (2012), with permission from Elsevier.
![Figure 2. Tidal volume (VT)/dynamic inspiratory capacity (IC) ratio as a function of ventilation during constant work rate exercise in COPD patients showing progressively lower FEV1 values [quartile (Q) 1 to 4] (A). Dyspnea is expressed relative to VT/IC and ventilation (absolute and relative to peak values) in (B), (C) and (D), respectively. Note the clear inflection (plateau) in the VT/ventilation relationship (A), which coincides with a simultaneous inflection in dyspnea (B) across the FEV1 quartiles. FEV1: forced expiratory volume in 1 s; IC: inspiratory capacity; VT: tidal volume. Reprinted from Chest, 141(3), O'Donnell DE, Guenette JA, Maltais F, Webb KA, Decline of resting inspiratory capacity in COPD the impact on breathing pattern, dyspnea, and ventilatory capacity during exercise, page 760, Copyright (2012), with permission from Elsevier.](/cms/asset/76be2350-c2da-4d9d-9974-360cf4caa10a/icop_a_1606189_f0002_b.jpg)
increased areas of high alveolar ventilation (V˙A)/capillary perfusion (Qc) reflected as poorer ventilatory efficiency and larger physiological dead space compared to patients with milder disease (Citation9,Citation32,Citation35),
low V˙A/Qc units which, in association with reduced systemic mixed venous oxygen (O2), may lead to critical arterial O2 desaturation (Citation36,Citation37) and
increased acid–base disturbances (e.g. early metabolic acidosis due to peripheral muscle abnormalities or impaired cardiac function) (Citation38,Citation39).
In advanced COPD, alveolar hypoventilation may occur during exercise, reflecting severe mechanical limitation and respiratory muscle dysfunction at low work rates (Citation40), particularly in the setting of a large dead space and restricted tidal volume expansion (Citation40,Citation41). The negative haemodynamic consequences of hyperinflation may dynamically impair cardiac output and O2 delivery to peripheral muscles (Citation42–44) with consequent worsening of metabolic acidosis and further stimulation of ventilation (Citation38).
Abnormalities in peripheral muscle structure and function are frequently seen in patients with COPD, particularly those with more advanced disease (Citation17). Appendicular muscle atrophy (i.e. increased protein breakdown relative to synthesis) (Citation45) may occur secondary to years of physical inactivity (Citation17,Citation46), systemic inflammation (Citation47), oxidative stress (Citation48) and chronic hypoxemia (Citation49). Many patients present with a shift in muscle fiber type distribution toward a more glycolytic profile, i.e. reduction in type I and increase in type II fibers (Citation17). Lower mitochondrial density (Citation50) and decreased oxidative enzyme activity (Citation51) are also described. Consequently, there might be a down-regulation of Krebs cycle or β-oxidation pathways in favor of glycolytic metabolism with impaired efficiency of oxidative phosphorylation (Citation52–54). In fact, a sizeable fraction of patients with COPD develop contractile fatigue after exercise (ranging from ∼50 to 100% according to disease severity, exercise intensity and duration and severity of hypoxemia) (Citation34,Citation55–57). Of note, perceived fatigability is frequently reported by patients with COPD undergoing pulmonary rehabilitation (Citation58). At least part of the post-exercise neuromuscular fatigue seen in COPD is secondary to hypoxemia and poor O2 delivery; for instance, Amann et al. (Citation58) showed that approximately a third of fatigue induced by exercise was attenuated with supplemental O2 in COPD patients with moderate hypoxemia. The remaining fatigue has been attributed to intrinsic muscle abnormalities; in fact, electrically evoked muscle contractions led to greater muscle deoxygenation and lower torque even in non-hypoxemic patients with COPD (Citation130).
Mechanisms of activity-related dyspnea in COPD
Fundamentally, exertional dyspnea occurs when the central respiratory drive from bulbo-pontine and cortical motor centers in the brain to the respiratory muscles is excessively increased relative to maximum (Citation59–65). Unpleasant respiratory sensations that result from increased chemoreflex activation are known to be perceived directly (likely via central corollary discharge to the somatosensory cortex) (Citation66–68). Thus, there is experimental evidence that perceived breathing difficulty (“air hunger”) can be provoked by chemo-stimulation alone in the absence of attendant respiratory muscle activity (Citation69). However, in spontaneously breathing humans, the experience of dyspnea is profoundly influenced by simultaneous afferent feedback from of a multitude of sensory receptors throughout the respiratory system (Citation70–75).
There is evidence in COPD (using surrogate markers of central drive, muscular and mechanical responses) that dyspnea occurs in the context of demand–capacity imbalance of the respiratory system (Citation76). In neurophysiological terms this is referred to as: neuro-muscular, neuro-mechanical or efferent-afferent dissociation of the respiratory system. Collectively these terms point to the disharmony, disparity or mismatch between the excessive drive to breathe and the ability of the respiratory system to appropriately respond (Citation59–62,Citation64). Thus, dyspnea occurs when, with each breath, very little air enters the lung in the face of vigorous inspiratory effort. Crude indirect measures of increased central drive include:
Figure 3. Shallower slopes of exertional breathlessness (A) and leg discomfort (B) over time during constant-load cycle exercise after exercise training (EXT) or control intervention in patients with COPD. Endurance time also increased significantly after EXT. Slopes of carbon dioxide output (VCO2) (C) ventilation (VE) (D) and breathing frequency (F) also fell significantly after EXT with no significant changes in tidal volume (VT) (E), *p<.05. EXT: exercise training; F: respiratory frequency; V′CO2: carbon dioxide production; V′E: minute ventilation; VT: tidal volume. Reprinted with permission of the American Thoracic Society. Copyright © 2019 American Thoracic Society. O'Donnell DE, McGuire M, Samis L, Webb KA. General exercise training improves ventilatory and peripheral muscle strength and endurance in chronic airflow limitation. Am J Respir Crit Care Med. 1998;157(5):1489–1497. The American Journal of Respiratory and Critical Care Medicine is an official journal of the American Thoracic Society.
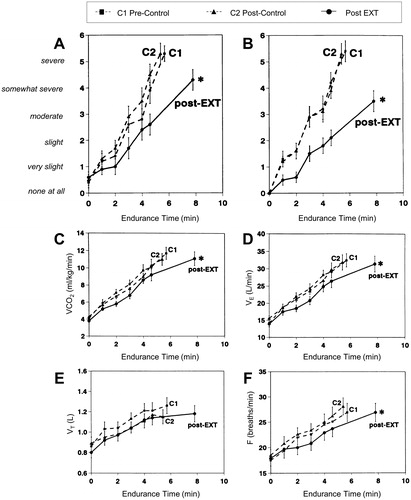
overall ventilatory output (V˙E relative to maximal ventilatory capacity) (Citation77,Citation78),
magnitude of forces generated by the muscles of respiration during tidal breathing (esophageal pressure relative to maximum) (Citation79,Citation80) and
inspiratory neural drive to the diaphragm [diaphragm activation relative to maximum (EMGdi/EMGdimax)] (Citation81,Citation82).
Each of these physiological ratios correlate directly with the rise in intensity of dyspnea during exercise (Citation9,Citation10,Citation13–16). Conversely, therapeutic interventions that improve (reduce) these ratios are consistently associated with improved dyspnea and exercise tolerance (Citation83–86).
Mechanisms of improvement in activity-related dyspnea with EXT
The mechanisms of improvement of dyspnea and exercise tolerance following EXT in pulmonary rehabilitation have not been fully elucidated and remain poorly studied. Available data have been generated from a few small studies, often without suitable control arms, with wide variation in the baseline clinical and pathophysiological characteristics of the study participants.
Among the most common physiological benefits of EXT identified in patients with COPD is reduced ventilatory demand at standardised work rates during exercise () (Citation36,Citation87–89). This, at least in part, is attributed to improved oxidative capacity of the trained peripheral muscles and consequent delayed metabolic (lactic) acidosis (Citation36,Citation87,Citation90–93). Improved metabolic milieu in the locomotor muscles means less hydrogen ion generation and CO2 output and therefore reduced chemo-stimulation of central medullary and bulbo-pontine control centers. The consequent reduced central corollary discharge from these centers to the somatosensory cortex is postulated to result in reduced perceived respiratory discomfort (Citation94). Additionally, reduced mechano- and ergo-receptor stimulation, as a result of improved acid–base status within the active muscles and resultant alterations in ascending afferent inputs to respiratory control centers, also potentially reduces inspiratory neural drive to breathe and associated dyspnea (Citation91).
Figure 4. Changes in inspiratory capacity (IC) as a function of time (A) and breathing frequency (B) during constant-load exercise prior to and following an exercise training program in a representative patient with COPD. The dashed arrows connect isotime values. COPD: chronic obstructive pulmonary disease; f: respiratory frequency; IC: inspiratory capacity. Reprinted from Chest, 128(4), Porszasz J, Emtner M, Goto S, Somfay A, Whipp BJ, Casaburi R, Exercise training decreases ventilatory requirements and exercise induced hyperinflation at submaximal intensities in patients with COPD, page 2030, Copyright (2005), with permission from Elsevier.
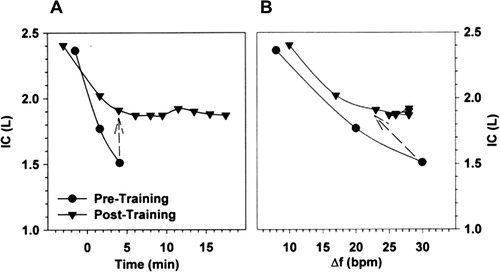
Reduced ventilation following EXT is mainly reflected as reduced breathing frequency, usually in the order of 10–15% for the pre-training frequency at standardised work rates (Citation95). Increases in tidal volume are generally more modest, particularly in advanced COPD where critical constrictive mechanics while breathing close to total lung capacity markedly diminish any volume expansion capability (Citation31,Citation96). Reduced breathing frequency has potentially favorable physiological consequences:
reduced velocity of shortening of the inspiratory muscles may improve their dynamic strength and endurance and
by increasing expiratory time, dynamic lung hyperinflation (gas trapping) is reduced at a given level of expiratory flow limitation.
In fact, under conditions of expiratory flow limitation and delayed mechanical time constants for emptying within heterogeneously distributed alveolar units, dynamic EELV (and, reciprocally, IC) during exercise is strongly influenced by the prevailing breathing pattern (Citation13,Citation16). Simply put, slower breathing frequency facilitates more effective lung emptying with each breath, reduced EELV and increased IC (). Increases in dynamic IC at standardised exercise times after EXT averaged 0.1–0.4 L/min in some studies (Citation88,Citation89,Citation97,Citation98), but not all studies (Citation95). Reduced dynamic lung hyperinflation is beneficial in that it is associated with reduction of the elastic and inspiratory threshold load on the overburdened inspiratory muscles and increases their functional strength. In this situation, the magnitude of inspiratory neural drive from motor command centers should diminish with partial reversal of neuromechanical dissociation and some alleviation of exertional dyspnea (Citation88,Citation89).
Figure 5. Change in resting maximal inspiratory and expiratory pressure (MIP and MEP, respectively) and inspiratory muscle endurance time in COPD patients who completed a general exercise training program compared to control, *p<.05 compared to pre-intervention baseline. COPD: chronic obstructive pulmonary disease; MIP: maximum inspiratory pressure; MEP: maximum expiratory pressure. Reprinted with permission of the American Thoracic Society. Copyright © 2019 American Thoracic Society. O'Donnell DE, McGuire M, Samis L, Webb KA. General exercise training improves ventilatory and peripheral muscle strength and endurance in chronic airflow limitation. Am J Respir Crit Care Med. 1998;157(5):1489–1497. The American Journal of Respiratory and Critical Care Medicine is an official journal of the American Thoracic Society.
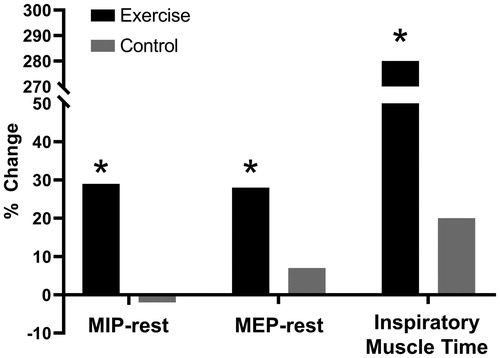
Improved IC following EXT may also reflect increased static inspiratory muscle strength (Citation95). This is to be expected when patients with COPD are required to undertake sustained physical activity over time in EXT regimens which demand relatively high ventilatory output on a regular basis during multi-modality training. Thus, the respiratory muscles, faced with significant intrinsic mechanical loads, adapt by increasing their strength and endurance (). Accordingly, the cortical motor command output required to generate force and sustain ventilation to the trained respiratory muscles falls and with it sense of breathing effort diminishes. In these patients, effort intensity diminishes for a given ventilation as well as for a given metabolic demand (Citation85). In a small physiological study, specific inspiratory muscle training (IMT) using a flow-tapered device improved inspiratory muscle strength and endurance in mechanically compromised patients with COPD showing inspiratory muscle weakness. Of note, the attendant reduction in EMGdi/EMGdimax helped explain the decrease in perceived respiratory discomfort despite sustained high ventilation and intrinsic mechanical loading over a longer exercise duration () (Citation85). These beneficial effects of IMT for patients with inspiratory muscle weakness were recently confirmed in a large double-blind, multicentre randomised controlled trial which detected additional gains (relative to standard pulmonary rehabilitation) in endurance time and exertional dyspnea (Citation99).
Figure 6. Lower dyspnea intensity (A and D), diaphragm electromyography measured during tidal inspiration/largest value during a maximum inspiratory maneuver (EMGdi/EMGdimax) (B and E), and ventilation (C and F) during constant work rate exercise before and after inspiratory muscle training (IMT) (A–C) and the control intervention (D–F) in patients with COPD showing inspiratory muscle weakness at baseline. Values are mean ± standard error. *p<.05, post- versus pre-intervention at isotime. COPD: chronic obstructive pulmonary disease; IMT: inspiratory muscle training; EMGdi/EMGdimax: diaphragm electromyography measured during tidal inspiration/largest value during a maximum inspiratory maneuver. Adapted from: Langer D, Ciavaglia C, Faisal A, Webb KA, Neder JA, Gosselink R, Dacha S, Topalovic M, Ivanova A, O’Donnell DE. Inspiratory muscle training reduces diaphragm activation and dyspnea during exercise in COPD. J Appl Physiol. 2018;125(2):381–92.
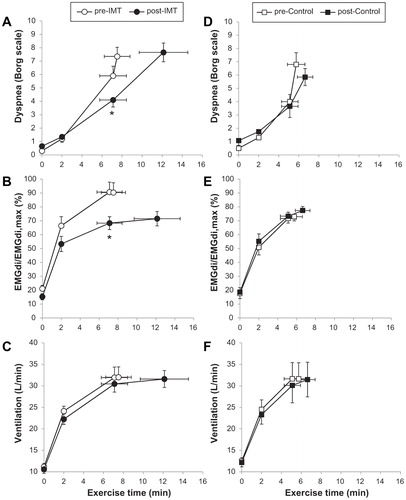
It should be recognised, however, that such physiological improvements are not obligatory for reduction of exertional dyspnea in all circumstances. Negative affective responses such as fear, anxiety, distress, panic and sense of impending suffocation are integral to the experience of respiratory discomfort in COPD. This is believed to be physiologically linked to over-activation of limbic and para-limbic centers of the brain such as the insula, anterior cingulate gyrus and amygdala (Citation63,Citation100–104). Moreover, attendant over-activation of the sympathetic nervous system has major negative cardio-circulatory and respiratory consequences which ultimately further amplify breathing difficulty (Citation28,Citation105). In a clinical physiological study involving 48 patients randomised to either 8 weeks of pulmonary rehabilitation or usual care, chronic activity-related dyspnea, anxiety, depression and self-efficacy scores all consistently improved in the former group () (Citation27). These desirable patient-centered outcomes occurred in the absence of physiological training effects (reduced ventilation, breathing frequency and lung hyperinflation) (). Thus, significant and clinically meaningful improvements were found in the affective and impact domains of dyspnea and likely reflect major psychosocial gains and behavioral modification that are still incompletely understood (Citation27). The preeminent importance of addressing the affective domain of dyspnea has been elegantly corroborated by a neurophysiological study, which demonstrated that pulmonary rehabilitation in COPD consistently altered brain activity in stimulus valuation networks measured by functional magnetic resonance imaging (Citation106).
Figure 7. Impact of exercise training as part of pulmonary rehabilitation or control intervention on dyspnea (A), anxiety (B), depression (C) and self-efficacy (D) in patients with COPD. Lack of change in O2 uptake (V′O2) (E and F) and ventilation (V′E) (G and H) during constant-load exercise pre- and post-exercise training as part of pulmonary rehabilitation (EXT) or control intervention (C) in patients with COPD. Error bars represent standard error of the mean, *p<.05. COPD: chronic obstructive pulmonary disease; EXT: pulmonary rehabilitation; HADS: Hospital Anxiety and Depression Scale; TDI: Translational Dyspnea Index; V′O2: oxygen consumption. Adapted from: Wadell K, Webb KA, Preston ME, Amornputtisathaporn N, Samis L, Patelli J, Guenette JA, O’Donnell DE. Impact of pulmonary rehabilitation on the major dimensions of dyspnea in COPD. COPD. 2013;10(4):425–35.
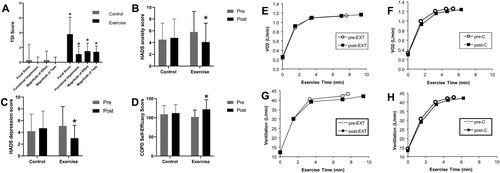
Mechanisms of improvement in peripheral muscle abnormalities with EXT
There is mounting evidence that EXT might improve limb muscle function and structure in a sub-set of patients with COPD (Citation107,Citation108). The magnitude of these responses, however, is highly variable; in fact, some patients may show little or no measurable physiological benefit. In those who respond, quadriceps muscle strength (Citation108), endurance (Citation109–111) and fatigability (Citation56) have been shown to increase. Some studies (Citation112–114), but not all (Citation115), showed increases in midthigh muscle cross-sectional area and fat-free mass. Endurance training may increase the cross-sectional area of all fiber types within the quadriceps femoris and decrease the proportion of type IIx fibers (Citation17). From a bioenergetic standpoint, the following can be observed in some patients:
increase in capillary-to-fiber ratio (Citation116–118) and the activity of oxidative enzymes (Citation92,Citation119,Citation120]), i.e. improved oxidative metabolism,
decrease in exercise-induced lactic acidosis (Citation87) and
attenuation of the decline in the phosphocreatine/inorganic phosphorus ratio during exercise (Citation119).
Strategies to foster anabolism might be relevant for depleted patients with low fat-free mass, particularly considering the increased energy expenditure during exercise training. Combining nutritional supplementation (high-protein oral nutritional supplements) during in-patient pulmonary rehabilitation of depleted patients has shown improvements in weight, fat-free mass, exercise capacity, respiratory and peripheral muscle strength (Citation2,Citation3). A multimodal intervention approach, including nutritional supplements, anabolic steroids and exercise training might prove useful in improving body weight and fat free mass with beneficial consequences to exercise tolerance (Citation45,Citation121).
As expected, longer pulmonary rehabilitation programs are associated with greater training effects, with most experts recommending a minimum of 8 weeks to achieve a substantial physiological effect (Citation2,Citation3). The optimal exercise intensity remains debatable. In the seminal study of Casaburi et al. (Citation87), high intensity exercise (>60% peak work rate) for 20–60 min per session was more efficient in inducing physiological responses than less intense exercise (<40% peak work rate). However, many patients are unable to train at that a level of stress sufficient to improve peripheral muscle function (Citation3): patients who develop less quadriceps contractile fatigue during the training sessions (i.e. those who trained at lower intensities) showed lower training effects in terms of functional exercise capacity (Citation122). For these subjects, symptom-targeted exercise intensity and/or localised, passive training (e.g. neuromuscular electrical stimulation) (Citation123) might be the only feasible alternatives. Interval exercise training is also a valid option (Citation117,Citation124,Citation125): several smaller bouts (typically lasting 30–180 s) of high-intensity exercise (80–120% peak capacity) are interspersed with periods of rest or unloaded exercise (lasting 30–60 s) or by lower-intensity exercise bouts (40–80%). The former approach is particularly useful to delay the attainment of critical mechanical and ventilatory constraints, thereby prolonging exercise duration with lower sensations of dyspnea and leg discomfort () (Citation126). Interestingly, high-intensity interval training seems particularly efficient to reverse type I-to-II muscle fiber shift in these patients (Citation107,Citation116).
Figure 8. Metabolic (A), ventilatory, (B and C), mechanical (D) and sensory (E) responses to 30s:60s (left) and 60s:120s (right) interval protocols in COPD patients who tolerated or not off-loaded protocols for 30 min (“higher-” and “lower-tolerance”, respectively). The latter group repeated the most demanding protocol (60s:120s) with no load in the recovery phases (off-unloaded). Shaded areas indicate physiological limits typically associated with diminished exercise tolerance in COPD. Values are mean and standard error. *p<.05, between-groups, intra-protocol comparisons; †60s:120 s off-unloaded versus off-loaded in the “lower-tolerance” group. EILV: end inspiratory lung volume; MVV: maximal voluntary ventilation; V′CO2: carbon dioxide output; V′E: minute ventilation; TLC: total lung capacity. Reprinted from Respiratory Physiology & Neurobiology, 250, Bravo DM, Gimenes AC, Amorim BC, Alencar MC, Berton DC, O'Donnell DE, Nery LE, Neder JA, Excess ventilation in COPD implications for dyspnoea and tolerance to interval exercise, page 11, Copyright (2018), with permission from Elsevier.
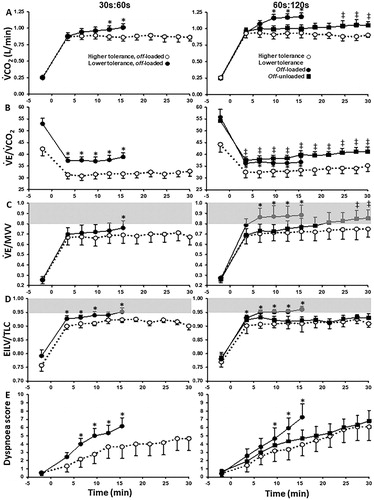
Resistance training (typically 12 weeks duration, two to three sessions weekly, two to four sets of 8 to 12 repetitions of loads ranging from ∼30–90% of one repetition maximum) has also been shown to increase muscle strength and mass in patients with COPD (Citation108,Citation127). The combination of resistance with endurance training has revealed a greater improvement in quadriceps muscle strength and thigh muscle cross-sectional area compared with endurance training alone (Citation112,Citation128,Citation129). Thus, the prevailing view is that patients with stable COPD should perform a combination of endurance and resistance training to maximise improvement in both limb muscle function and whole-body exercise capacity (Citation128,Citation129).
Conclusion
Exertional dyspnea in COPD is linked to high inspiratory neural drive to the respiratory muscles. EXT, by improving dynamic respiratory mechanics and muscle function, and by delaying metabolic acidosis can reduce IND and attendant dyspnea. In reality, however, such physiological training effects are difficult to achieve in more advanced COPD. Importantly, pulmonary rehabilitation in general, favorably alters the affective component of dyspnea in the majority of participants. Beneficial changes in peripheral (leg) muscles can be seen in those who can tolerate higher training intensity, particularly using combined resistance and dynamic (including interval) exercise. However, it is now broadly acknowledged that in many frail elderly patients with advanced respiratory mechanical and pulmonary gas exchange derangements with serious co-morbidities (such as cardiac and peripheral vascular disease) and intolerable dyspnea, meaningful physiological training effects might not be achievable. These potential shortcomings should not discourage the use of pulmonary rehabilitation as an effective strategy to improve patients’ capacity to tolerate physical activity. In the modern era, our greatest challenge is to develop effective strategies to ensure that these important gains in functional capacity are translated into sustained increases in daily physical activity for patients with COPD.
Disclosure statement
D.E.O’D. has received research funding via Queen’s University from AstraZeneca, Boehringer Ingelheim, Canadian Institutes of Health Research and Canadian Respiratory Research Network and has served on speaker bureaus, consultation panels, and advisory boards for AstraZeneca, Boehringer Ingelheim and Novartis. J.A.N., M.M., A.C.B., M.D.J., K.M.M. and D.E.O’D. report no conflict of interest.
References
- Spruit MA, Pitta F, McAuley E, et al. Pulmonary rehabilitation and physical activity in patients with chronic obstructive pulmonary disease. Am J Respir Crit Care Med. 2015;192(8):924–933. doi: 10.1164/rccm.201505-0929CI.
- Spruit MA, Singh SJ, Garvey C, et al. An official American Thoracic Society/European Respiratory Society statement: key concepts and advances in pulmonary rehabilitation. Am J Respir Crit Care Med. 2013;188(8):e13–e64.
- Troosters T, Casaburi R, Gosselink R, Decramer M. Pulmonary rehabilitation in chronic obstructive pulmonary disease. Am J Respir Crit Care Med. 2005;172(1):19–38. doi: 10.1164/rccm.200408-1109SO.
- Lacasse Y, Brosseau L, Milne S, et al. Pulmonary rehabilitation for chronic obstructive pulmonary disease. Cochrane Database Syst Rev. 2002;3:CD003793.
- Dowman L, Hill CJ, Holland AE. Pulmonary rehabilitation for interstitial lung disease. Cochrane Database Syst Rev. 2014 (10):Cd006322.
- McCarthy B, Casey D, Devane D, et al. Pulmonary rehabilitation for chronic obstructive pulmonary disease. Cochrane Database Syst Rev. 2015 (2):CD003793.
- GOLD COPD. Global Strategy for the Diagnosis, Management, and Prevention of Chronic Obstructive Pulmonary Disease 2019 Report; 2019.
- O’Donnell DE, Elbehairy AF, Berton DC, et al. Advances in the evaluation of respiratory pathophysiology during exercise in chronic lung diseases. Front Physiol. 2017;8:82.
- Elbehairy AF, Ciavaglia CE, Webb KA, et al. Pulmonary gas exchange abnormalities in mild chronic obstructive pulmonary disease. Implications for dyspnea and exercise intolerance. Am J Respir Crit Care Med. 2015;191(12):1384–1394. doi: 10.1164/rccm.201501-0157OC.
- Faisal A, Alghamdi BJ, Ciavaglia CE, et al. Common mechanisms of dyspnea in chronic interstitial and obstructive lung disorders. Am J Respir Crit Care Med. 2016;193(3):299–309. doi: 10.1164/rccm.201504-0841OC.
- Barbera JA, Roca J, Ramirez J, et al. Gas exchange during exercise in mild chronic obstructive pulmonary disease. Correlation with lung structure. Am Rev Respir Dis. 1991;144(3 Pt 1):520–525. doi: 10.1164/ajrccm/144.3_Pt_1.520.
- Rodriguez-Roisin R, Drakulovic M, Rodriguez DA, et al. Ventilation-perfusion imbalance and chronic obstructive pulmonary disease staging severity. J Appl Physiol. 2009;106(6):1902–1908. doi: 10.1152/japplphysiol.00085.2009.
- Chin RC, Guenette JA, Cheng S, et al. Does the respiratory system limit exercise in mild chronic obstructive pulmonary disease? Am J Respir Crit Care Med. 2013;187(12):1315–1323. doi: 10.1164/rccm.201211-1970OC.
- Elbehairy AF, Guenette JA, Faisal A, et al. Mechanisms of exertional dyspnoea in symptomatic smokers without COPD. Eur Respir J. 2016;48(3):694–705. doi: 10.1183/13993003.00077-2016.
- Guenette JA, Chin RC, Cheng S, et al. Mechanisms of exercise intolerance in global initiative for chronic obstructive lung disease grade 1 COPD. Eur Respir J. 2014;44(5):1177–1187. doi: 10.1183/09031936.00034714.
- Ofir D, Laveneziana P, Webb KA, et al. Mechanisms of dyspnea during cycle exercise in symptomatic patients with GOLD stage I chronic obstructive pulmonary disease. Am J Respir Crit Care Med. 2008;177(6):622–629. doi: 10.1164/rccm.200707-1064OC.
- Maltais F, Decramer M, Casaburi R, et al. An official American Thoracic Society/European Respiratory Society statement: update on limb muscle dysfunction in chronic obstructive pulmonary disease. Am J Respir Crit Care Med. 2014;189(9):e15–e62. doi: 10.1164/rccm.201402-0373ST.
- Gea J, Casadevall C, Pascual S, et al. Respiratory diseases and muscle dysfunction. Exp Rev Respir Med. 2012;6(1):75–90. doi: 10.1586/ers.11.81.
- Aliverti A, Macklem PT. How and why exercise is impaired in COPD. Respiration. 2001;68(3):229–239. doi: 10.1159/000050502.
- Light RW, Mintz HM, Linden GS, Brown SE. Hemodynamics of patients with severe chronic obstructive pulmonary disease during progressive upright exercise. Am Rev Respir Dis. 1984;130(3):391–395. doi: 10.1164/arrd.1984.130.3.391.
- Mahler DA, Brent BN, Loke J, et al. Right ventricular performance and central circulatory hemodynamics during upright exercise in patients with chronic obstructive pulmonary disease. Am Rev Respir Dis. 1984;130(5):722–729. doi: 10.1164/arrd.1984.130.5.722.
- Magee F, Wright JL, Wiggs BR, et al. Pulmonary vascular structure and function in chronic obstructive pulmonary disease. Thorax. 1988;43(3):183–189.
- Oswald-Mammosser M, Apprill M, Bachez P, et al. Pulmonary hemodynamics in chronic obstructive pulmonary disease of the emphysematous type. Respiration. 1991;58(5–6):304–310. doi: 10.1159/000195950.
- Killian KJ, Jones NL. The use of exercise testing and other methods in the investigation of dyspnea. Clin Chest Med. 1984;5(1):99–108.
- Normandin EA, McCusker C, Connors M, et al. An evaluation of two approaches to exercise conditioning in pulmonary rehabilitation. Chest. 2002;121(4):1085–1091.
- Puente-Maestu L, Sanz ML, Sanz P, et al. Comparison of effects of supervised versus self-monitored training programmes in patients with chronic obstructive pulmonary disease. Eur Respir J. 2000;15(3):517–525. doi: 10.1034/j.1399-3003.2000.15.15.x.
- Wadell K, Webb KA, Preston ME, et al. Impact of pulmonary rehabilitation on the major dimensions of dyspnea in COPD. COPD. 2013;10(4):425–435. doi: 10.3109/15412555.2012.758696.
- Neder JA, Rocha A, Alencar MCN, et al. Current challenges in managing comorbid heart failure and COPD. Exp Rev Cardiovasc Ther. 2018;16(9):653–673. doi: 10.1080/14779072.2018.1510319.
- Reardon JZ, Lareau SC, ZuWallack R. Functional status and quality of life in chronic obstructive pulmonary disease. Am J Med. 2006;119(10):32–37. doi: 10.1016/j.amjmed.2006.08.005.
- Raskin J, Spiegler P, McCusker C, et al. The effect of pulmonary rehabilitation on healthcare utilization in chronic obstructive pulmonary disease: the Northeast Pulmonary Rehabilitation Consortium. J Cardiopulm Rehabil. 2006;26(4):231–236.
- O’Donnell DE, Guenette JA, Maltais F, Webb KA. Decline of resting inspiratory capacity in COPD: the impact on breathing pattern, dyspnea, and ventilatory capacity during exercise. Chest. 2012;141(3):753–762. doi: 10.1378/chest.11-0787.
- Neder JA, Arbex FF, Alencar MC, et al. Exercise ventilatory inefficiency in mild to end-stage COPD. Eur Respir J. 2015;45(2):377–387. doi: 10.1183/09031936.00135514.
- O’Donnell DE, Revill SM, Webb KA. Dynamic hyperinflation and exercise intolerance in chronic obstructive pulmonary disease. Am J Respir Crit Care Med. 2001;164(5):770–777. doi: 10.1164/ajrccm.164.5.2012122.
- Mador MJ, Kufel TJ, Pineda LA, Sharma GK. Diaphragmatic fatigue and high-intensity exercise in patients with chronic obstructive pulmonary disease. Am J Respir Crit Care Med. 2000;161(1):118–123. doi: 10.1164/ajrccm.161.1.9903010.
- Buist AS. Early detection of airways obstruction by the closing volume technique. Chest. 1973;64(4):495–499.
- Andrianopoulos V, Franssen FM, Peeters JP, et al. Exercise-induced oxygen desaturation in COPD patients without resting hypoxemia. Respir Physiol Neurobiol. 2014;190:40–46. doi: 10.1016/j.resp.2013.10.002.
- Moreira MA, Medeiros GA, Boeno FP, et al. Oxygen desaturation during the six-minute walk test in COPD patients. J Bras Pneumol. 2014;40(3):222–228.
- Patessio A, Casaburi R, Carone M, et al. Comparison of gas exchange, lactate, and lactic acidosis thresholds in patients with chronic obstructive pulmonary disease. Am Rev Respir Dis 1993;148(3):622–626. doi: 10.1164/ajrccm/148.3.622.
- Pleguezuelos E, Esquinas C, Moreno E, et al. Muscular dysfunction in COPD: Systemic effect or deconditioning? Lung. 2016;194(2):249–257. doi: 10.1007/s00408-015-9838-z.
- O’Donnell DE, D’Arsigny C, Fitzpatrick M, Webb KA. Exercise hypercapnia in advanced chronic obstructive pulmonary disease: the role of lung hyperinflation. Am J Respir Crit Care Med. 2002;166(5):663–668. doi: 10.1164/rccm.2201003.
- O’Donnell DE, Maltais F, Porszasz J, et al. The continuum of physiological impairment during treadmill walking in patients with mild-to-moderate COPD: patient characterization phase of a randomized clinical trial. PLoS One. 2014;9(5):e96574. doi: 10.1371/journal.pone.0096574.
- Chiappa GR, Borghi-Silva A, Ferreira LF, et al. Kinetics of muscle deoxygenation are accelerated at the onset of heavy-intensity exercise in patients with COPD: relationship to central cardiovascular dynamics. J Appl Physiol. 2008;104(5):1341–1350. doi: 10.1152/japplphysiol.01364.2007.
- Laveneziana P, Palange P, Ora J, et al. Bronchodilator effect on ventilatory, pulmonary gas exchange, and heart rate kinetics during high-intensity exercise in COPD. Eur J Appl Physiol. 2009;107(6):633–643. doi: 10.1007/s00421-009-1169-4.
- Laveneziana P, Valli G, Onorati P, et al. Effect of heliox on heart rate kinetics and dynamic hyperinflation during high-intensity exercise in COPD. Eur J Appl Physiol. 2011;111(2):225–234. doi: 10.1007/s00421-010-1643-z.
- Simoes DCM, Vogiatzis I. Can muscle protein metabolism be specifically targeted by exercise training in COPD? J Thorac Dis. 2018;10(Suppl. 12):S1367–S1376. doi: 10.21037/jtd.2018.02.67.
- Jaitovich A, Barreiro E. Skeletal muscle dysfunction in chronic obstructive pulmonary disease. What we know and can do for our patients. Am J Respir Crit Care Med. 2018;198(2):175–186. doi: 10.1164/rccm.201710-2140CI.
- Remels AH, Gosker HR, van der Velden J, et al. Systemic inflammation and skeletal muscle dysfunction in chronic obstructive pulmonary disease: state of the art and novel insights in regulation of muscle plasticity. Clin Chest Med. 2007;28(3):537–552. doi: 10.1016/j.ccm.2007.06.003.
- Moylan JS, Reid MB. Oxidative stress, chronic disease, and muscle wasting. Muscle Nerve. 2007;35(4):411–429. doi: 10.1002/mus.20743.
- Favier FB, Costes F, Defour A, et al. Downregulation of Akt/mammalian target of rapamycin pathway in skeletal muscle is associated with increased REDD1 expression in response to chronic hypoxia. Am J Physiol Regul Integr Comp Physiol. 2010;298(6):R1659–R1666. doi: 10.1152/ajpregu.00550.2009.
- Gosker HR, Hesselink MK, Duimel H, et al. Reduced mitochondrial density in the vastus lateralis muscle of patients with COPD. Eur Respir J. 2007;30(1):73–79. doi: 10.1183/09031936.00146906.
- Allaire J, Maltais F, Doyon JF, et al. Peripheral muscle endurance and the oxidative profile of the quadriceps in patients with COPD. Thorax. 2004;59(8):673–678. doi: 10.1136/thx.2003.020636.
- Saey D, Lemire BB, Gagnon P, et al. Quadriceps metabolism during constant workrate cycling exercise in chronic obstructive pulmonary disease. J Appl Physiol. 2011;110(1):116–124. doi: 10.1152/japplphysiol.00153.2010.
- Puente-Maestu L, Perez-Parra J, Godoy R, et al. Abnormal mitochondrial function in locomotor and respiratory muscles of COPD patients. Eur Respir J. 2009;33(5):1045–1052. doi: 10.1183/09031936.00112408.
- Taivassalo T, Hussain SN. Contribution of the mitochondria to locomotor muscle dysfunction in patients with COPD. Chest. 2016;149(5):1302–1312. doi: 10.1016/j.chest.2015.11.021.
- Mador MJ, Bozkanat E, Kufel TJ. Quadriceps fatigue after cycle exercise in patients with COPD compared with healthy control subjects. Chest. 2003;123(4):1104–1111.
- Mador MJ, Kufel TJ, Pineda LA, et al. Effect of pulmonary rehabilitation on quadriceps fatiguability during exercise. Am J Respir Crit Care Med. 2001;163(4):930–935. doi: 10.1164/ajrccm.163.4.2006125.
- Saey D, Debigare R, LeBlanc P, et al. Contractile leg fatigue after cycle exercise: a factor limiting exercise in patients with chronic obstructive pulmonary disease. Am J Respir Crit Care Med. 2003;168(4):425–430. doi: 10.1164/rccm.200208-856OC.
- Amann M, Regan MS, Kobitary M, et al. Impact of pulmonary system limitations on locomotor muscle fatigue in patients with COPD. Am J Physiol Regul Integr Comp Physiol. 2010;299(1):R314–R324. doi: 10.1152/ajpregu.00183.2010.
- Wright GW, Branscomb BV. The origin of the sensations of dyspnea. Trans Am Clin Climatol Assoc. 1955;66:116–125.
- Schwartzstein RM, Simon PM, Weiss JW, et al. Breathlessness induced by dissociation between ventilation and chemical drive. Am Rev Respir Dis. 1989;139(5):1231–1237. doi: 10.1164/ajrccm/139.5.1231.
- Harty HR, Corfield DR, Schwartzstein RM, Adams L. External thoracic restriction, respiratory sensation, and ventilation during exercise in men. J Appl Physiol. 1999;86(4):1142–1150. doi: 10.1152/jappl.1999.86.4.1142.
- Manning HL, Shea SA, Schwartzstein RM, et al. Reduced tidal volume increases 'air hunger' at fixed PCO2 in ventilated quadriplegics. Respir Physiol. 1992;90(1):19–30. doi: 10.1016/0034-5687(92)90131-F.
- Evans KC, Banzett RB, Adams L, et al. BOLD fMRI identifies limbic, paralimbic, and cerebellar activation during air hunger. J Neurophysiol. 2002;88(3):1500–1511. doi: 10.1152/jn.2002.88.3.1500.
- O’Donnell DE, Hong HH, Webb KA. Respiratory sensation during chest wall restriction and dead space loading in exercising men. J Appl Physiol. 2000;88(5):1859–1869. doi: 10.1152/jappl.2000.88.5.1859.
- Banzett RB, Pedersen SH, Schwartzstein RM, Lansing RW. The affective dimension of laboratory dyspnea: air hunger is more unpleasant than work/effort. Am J Respir Crit Care Med. 2008;177(12):1384–1390. doi: 10.1164/rccm.200711-1675OC.
- Chen Z, Eldridge FL, Wagner PG. Respiratory-associated thalamic activity is related to level of respiratory drive. Respir Physiol. 1992;90(1):99–113.
- Chen Z, Eldridge FL, Wagner PG. Respiratory-associated rhythmic firing of midbrain neurones in cats: relation to level of respiratory drive. J Physiol. 1991;437:305–325.
- Eldridge FL, Millhorn DE, Waldrop TG. Exercise hyperpnea and locomotion: parallel activation from the hypothalamus. Science. 1981;211(4484):844–846. doi: 10.1126/science.7466362.
- Gandevia SC, Killian K, McKenzie DK, et al. Respiratory sensations, cardiovascular control, kinaesthesia and transcranial stimulation during paralysis in humans. J Physiol. 1993;470:85–107.
- Banzett RB, Lansing RW, Brown R, et al. 'Air hunger' from increased PCO2 persists after complete neuromuscular block in humans. Respir Physiol. 1990;81(1):1–17.
- Banzett RB, Lansing RW, Reid MB, et al. 'Air hunger' arising from increased PCO2 in mechanically ventilated quadriplegics. Respir Physiol. 1989;76(1):53–67.
- Banzett RB, Mulnier HE, Murphy K, et al. Breathlessness in humans activates insular cortex. Neuroreport. 2000;11(10):2117–2120.
- Fowler WS. Breaking point of breath-holding. J Appl Physiol. 1954;6:539–545. doi: 10.1152/jappl.1954.6.9.539.
- Harty HR, Mummery CJ, Adams L, et al. Ventilatory relief of the sensation of the urge to breathe in humans: are pulmonary receptors important? J Physiol. 1996;490(3):805–815. doi: 10.1113/jphysiol.1996.sp021188.
- Bloch-Salisbury E, Spengler CM, Brown R, Banzett RB. Self-control and external control of mechanical ventilation give equal air hunger relief. Am J Respir Crit Care Med. 1998;157(2):415–420. doi: 10.1164/ajrccm.157.2.9701024.
- Jones NL. The ins and outs of breathing: how we learnt about the body’s most vital function. Bloomington, IN: iUniverse; 2011.
- Means JH. Dyspnea. Medical Monograph. Vol. 5. Baltimore (MD): Williams & Wilkins; 1924.
- Cournand A, Richards DW. Pulmonary insufficiency, Part IL Discussion of a physiological classification and presentation of clinical tests. Am Rev Tuberc. 1941;44:26–41.
- Killian KJ, Gandevia SC, Summers E, Campbell EJ. Effect of increased lung volume on perception of breathlessness, effort, and tension. J Appl Physiol Respir Environ Exerc Physiol. 1984;57(3):686–691. doi: 10.1152/jappl.1984.57.3.686.
- Bradley TD, Chartrand DA, Fitting JW, et al. The relation of inspiratory effort sensation to fatiguing patterns of the diaphragm. Am Rev Respir Dis. 1986;134(6):1119–1124. doi: 10.1164/arrd.1986.134.5.1119.
- Jolley CJ, Luo YM, Steier J, et al. Neural respiratory drive in healthy subjects and in COPD. Eur Respir J. 2009;33(2):289–297. doi: 10.1183/09031936.00093408.
- Jolley CJ, Luo YM, Steier J, et al. Neural respiratory drive and breathlessness in COPD. Eur Respir J. 2015;45(2):355–364. doi: 10.1183/09031936.00063014.
- O’Donnell DE, D’Arsigny C, Webb KA. Effects of hyperoxia on ventilatory limitation during exercise in advanced chronic obstructive pulmonary disease. Am J Respir Crit Care Med. 2001;163(4):892–898. doi: 10.1164/ajrccm.163.4.2007026.
- O’Donnell DE, Bain DJ, Webb KA. Factors contributing to relief of exertional breathlessness during hyperoxia in chronic airflow limitation. Am J Respir Crit Care Med. 1997;155(2):530–535. doi: 10.1164/ajrccm.155.2.9032190.
- Langer D, Ciavaglia CE, Faisal A, et al. Inspiratory muscle training reduces diaphragm activation and dyspnea during exercise in COPD. J Appl Physiol. 2018;125(2):381–392. doi: 10.1152/japplphysiol.01078.2017.
- Peters MM, Webb KA, O’Donnell DE. Combined physiological effects of bronchodilators and hyperoxia on exertional dyspnoea in normoxic COPD. Thorax. 2006;61(7):559–567. doi: 10.1136/thx.2005.053470.
- Casaburi R, Patessio A, Ioli F, et al. Reductions in exercise lactic acidosis and ventilation as a result of exercise training in patients with obstructive lung disease. Am Rev Respir Dis. 1991;143(1):9–18. doi: 10.1164/ajrccm/143.1.9.
- Porszasz J, Emtner M, Goto S, et al. Exercise training decreases ventilatory requirements and exercise-induced hyperinflation at submaximal intensities in patients with COPD. Chest. 2005;128(4):2025–2034. doi: 10.1378/chest.128.4.2025.
- Puente-Maestu L, Abad YM, Pedraza F, et al. A controlled trial of the effects of leg training on breathing pattern and dynamic hyperinflation in severe COPD. Lung 2006;184(3):159–167. doi: 10.1007/s00408-005-2576-x.
- Maltais F, Jobin J, Sullivan MJ, et al. Metabolic and hemodynamic responses of lower limb during exercise in patients with COPD. J Appl Physiol. 1998;84(5):1573–1580. doi: 10.1152/jappl.1998.84.5.1573.
- Maltais F, LeBlanc P, Jobin J, et al. Intensity of training and physiologic adaptation in patients with chronic obstructive pulmonary disease. Am J Respir Crit Care Med. 1997;155(2):555–561. doi: 10.1164/ajrccm.155.2.9032194.
- Maltais F, Leblanc P, Simard C, et al. Skeletal muscle adaptation to endurance training in patients with chronic obstructive pulmonary disease. Am J Respir Crit Care Med. 1996;154(2):442–447. doi: 10.1164/ajrccm.154.2.8756820.
- Maltais F, Simard AA, Simard C, et al. Oxidative capacity of the skeletal muscle and lactic acid kinetics during exercise in normal subjects and in patients with COPD. Am J Respir Crit Care Med. 1996;153(1):288–293. doi: 10.1164/ajrccm.153.1.8542131.
- Mahler DA, O’Donnell DE. Recent advances in dyspnea. Chest. 2015;147(1):232–241. doi: 10.1378/chest.14-0800.
- O’Donnell DE, McGuire M, Samis L, Webb KA. General exercise training improves ventilatory and peripheral muscle strength and endurance in chronic airflow limitation. Am J Respir Crit Care Med. 1998;157(5 Pt 1):1489–1497. doi: 10.1164/ajrccm.157.5.9708010.
- O’Donnell DE, Laveneziana P, Webb K, Neder JA. Chronic obstructive pulmonary disease: clinical integrative physiology. Clin Chest Med. 2014;35(1):51–69. doi: 10.1016/j.ccm.2013.09.008.
- Gigliotti F, Coli C, Bianchi R, et al. Exercise training improves exertional dyspnea in patients with COPD: evidence of the role of mechanical factors. Chest. 2003;123(6):1794–1802.
- Georgiadou O, Vogiatzis I, Stratakos G, et al. Effects of rehabilitation on chest wall volume regulation during exercise in COPD patients. Eur Respir J. 2007;29(2):284–291. doi: 10.1183/09031936.00121006.
- Charususin N, Gosselink R, Decramer M, et al. Randomised controlled trial of adjunctive inspiratory muscle training for patients with COPD. Thorax. 2018;73(10):942–950. doi: 10.1136/thoraxjnl-2017-211417.
- von Leupoldt A, Sommer T, Kegat S, et al. The unpleasantness of perceived dyspnea is processed in the anterior insula and amygdala. Am J Respir Crit Care Med. 2008;177(9):1026–1032. doi: 10.1164/rccm.200712-1821OC.
- von Leupoldt A, Sommer T, Kegat S, et al. Dyspnea and pain share emotion-related brain network. Neuroimage. 2009;48(1):200–206. doi: 10.1016/j.neuroimage.2009.06.015.
- Esser RW, Stoeckel MC, Kirsten A, et al. Brain activation during perception and anticipation of dyspnea in chronic obstructive pulmonary disease. Front Physiol. 2017;8:617.
- Stoeckel MC, Esser RW, Gamer M, et al. Brain responses during the anticipation of dyspnea. Neural plasticity. 2016;2016:6434987. doi: 10.1155/2016/6434987.
- Herigstad M, Hayen A, Evans E, et al. Dyspnea-related cues engage the prefrontal cortex: evidence from functional brain imaging in COPD. Chest. 2015;148(4):953–961. doi: 10.1378/chest.15-0416.
- Rocha A, Arbex FF, Sperandio PA, et al. Excess ventilation in chronic obstructive pulmonary disease-heart failure overlap. Implications for dyspnea and exercise intolerance. Am J Respir Crit Care Med. 2017;196(10):1264. doi: 10.1164/rccm.201704-0675OC.
- Herigstad M, Faull OK, Hayen A, et al. Treating breathlessness via the brain: changes in brain activity over a course of pulmonary rehabilitation. Eur Respir J. 2017;50(3):1701029. doi: 10.1183/13993003.01029-2017.
- De Brandt J, Spruit MA, Derave W, et al. Changes in structural and metabolic muscle characteristics following exercise-based interventions in patients with COPD: a systematic review. Expert Rev Respir Med. 2016;10(5):521–545. doi: 10.1586/17476348.2016.1157472.
- De Brandt J, Spruit MA, Hansen D, et al. Changes in lower limb muscle function and muscle mass following exercise-based interventions in patients with chronic obstructive pulmonary disease: a review of the English-language literature. Chron Respirat Dis. 2018;15(2):182–219. doi: 10.1177/1479972317709642.
- Covey MK, Collins EG, Reynertson SI, et al. Resistance training as a preconditioning strategy for enhancing aerobic exercise training outcomes in COPD. Respir Med. 2014;108(8):1141–1152. doi: 10.1016/j.rmed.2014.06.001.
- Nyberg A, Lindstrom B, Rickenlund A, Wadell K. Low-load/high-repetition elastic band resistance training in patients with COPD: a randomized, controlled, multicenter trial. Clin Respir J. 2015;9(3):278–288. doi: 10.1111/crj.12141.
- Vivodtzev I, Minet C, Wuyam B, et al. Significant improvement in arterial stiffness after endurance training in patients with COPD. Chest. 2010;137(3):585–592. doi: 10.1378/chest.09-1437.
- Bernard S, Whittom F, Leblanc P, et al. Aerobic and strength training in patients with chronic obstructive pulmonary disease. Am J Respir Crit Care Med. 1999;159(3):896–901. doi: 10.1164/ajrccm.159.3.9807034.
- Farias CC, Resqueti V, Dias FA, et al. Costs and benefits of pulmonary rehabilitation in chronic obstructive pulmonary disease: a randomized controlled trial. Braz J Phys Ther. 2014;18(2):165–173. doi: 10.1590/S1413-35552012005000151.
- Menon MK, Houchen L, Harrison S, et al. Ultrasound assessment of lower limb muscle mass in response to resistance training in COPD. Respir Res. 2012;13:119. doi: 10.1186/1465-9921-13-119.
- Bronstad E, Rognmo O, Tjonna AE, et al. High-intensity knee extensor training restores skeletal muscle function in COPD patients. Eur Respir J. 2012;40(5):1130–1136. doi: 10.1183/09031936.00193411.
- Vogiatzis I, Simoes DC, Stratakos G, et al. Effect of pulmonary rehabilitation on muscle remodelling in cachectic patients with COPD. Eur Respir J. 2010;36(2):301–310. doi: 10.1183/09031936.00112909.
- Vogiatzis I, Terzis G, Nanas S, et al. Skeletal muscle adaptations to interval training in patients with advanced COPD. Chest. 2005;128(6):3838–3845. doi: 10.1378/chest.128.6.3838.
- Costes F, Gosker H, Feasson L, et al. Impaired exercise training-induced muscle fiber hypertrophy and Akt/mTOR pathway activation in hypoxemic patients with COPD. J Appl Physiol. 2015;118(8):1040–1049. doi: 10.1152/japplphysiol.00557.2014.
- Puente-Maestu L, Tena T, Trascasa C, et al. Training improves muscle oxidative capacity and oxygenation recovery kinetics in patients with chronic obstructive pulmonary disease. Eur J Appl Physiol. 2003;88(6):580–587. doi: 10.1007/s00421-002-0743-9.
- Gosker HR, Schrauwen P, Broekhuizen R, et al. Exercise training restores uncoupling protein-3 content in limb muscles of patients with chronic obstructive pulmonary disease. Am J Physiol Endocrinol Metab. 2006;290(5):E976–E981. doi: 10.1152/ajpendo.00336.2005.
- Lewis MI, Fournier M, Storer TW, et al. Skeletal muscle adaptations to testosterone and resistance training in men with COPD. J Appl Physiol. 2007;103(4):1299–1310. doi: 10.1152/japplphysiol.00150.2007.
- Burtin C, Saey D, Saglam M, et al. Effectiveness of exercise training in patients with COPD: the role of muscle fatigue. Eur Respir J. 2012;40(2):338–344. doi: 10.1183/09031936.00111811.
- Neder JA, Sword D, Ward SA, et al. Home based neuromuscular electrical stimulation as a new rehabilitative strategy for severely disabled patients with chronic obstructive pulmonary disease (COPD). Thorax. 2002;57(4):333–337. doi: 10.1136/thorax.57.4.333.
- Beauchamp MK, Nonoyama M, Goldstein RS, et al. Interval versus continuous training in individuals with chronic obstructive pulmonary disease–a systematic review. Thorax. 2010;65(2):157–164. doi: 10.1136/thx.2009.123000.
- Vogiatzis I, Nanas S, Roussos C. Interval training as an alternative modality to continuous exercise in patients with COPD. Eur Respir J. 2002;20(1):12–19.
- Bravo DM, Gimenes AC, Amorim BC, et al. Excess ventilation in COPD: implications for dyspnoea and tolerance to interval exercise. Respir Physiol Neurobiol. 2018;250:7–13. doi: 10.1016/j.resp.2018.01.013.
- O’Shea SD, Taylor NF, Paratz JD. Progressive resistance exercise improves muscle strength and may improve elements of performance of daily activities for people with COPD: a systematic review. Chest. 2009;136(5):1269–1283. doi: 10.1378/chest.09-0029.
- Ortega F, Toral J, Cejudo P, et al. Comparison of effects of strength and endurance training in patients with chronic obstructive pulmonary disease. Am J Respir Crit Care Med. 2002;166(5):669–674. doi: 10.1164/rccm.2107081.
- Vonbank K, Strasser B, Mondrzyk J, et al. Strength training increases maximum working capacity in patients with COPD–randomized clinical trial comparing three training modalities. Respir Med. 2012;106(4):557–563. doi: 10.1016/j.rmed.2011.11.005.
- Medeiros WM, Fernandes MCT, Azevedo DP, de Freitas FFM, Amorim BC, Chiavegato LD, Hirai DM, O'Donnell DE, Neder JA. Oxygen delivery-utilization mismatch in contracting locomotor muscle in COPD: peripheral factors. Am J Physiol Regul Integr Comp Physiol. 2015;308:R105-R111.