Abstract
A concern of antibiotic use in chronic obstructive pulmonary disease (COPD) is the emergence and propagation of antimicrobial resistance (AMR). A systematic review was conducted to determine prevalence, pattern, risk factors and consequences of AMR in COPD. Bibliographic databases were searched from inception to November 2020, with no language restrictions, including studies of any design that included patients with COPD and reported prevalence and pattern of AMR. 2748 unique titles and abstracts were identified, of which 63 articles, comprising 26,387 patients, met inclusion criteria. Forty-four (69.8%) studies were performed during acute exacerbation. The median prevalence of AMR ranged from 0–100% for Pseudomonas aeruginosa, Moraxella catarrhalis, Klebsiella pneumoniae and Acinetobacter baumannii. Median resistance rates of H influenzae and S pneumoniae were lower by comparison, with maximum rates ≤40% and ≤46%, respectively, and higher for Staphylococcus aureus. There was a trend towards higher rates of AMR in patients with poorer lung function and greater incidence of previous antibiotic exposure and hospitalisation. The impact of AMR on mortality was unclear. Data regarding antimicrobial susceptibility testing techniques and the impact of other risk factors or consequences of AMR were variable or not reported. This is the first review to systematically unify data regarding AMR in COPD. AMR is relatively common and strategies to optimise antibiotic use could be valuable to prevent the currently under-investigated potential adverse consequences of AMR.
Supplemental data for this article is available online at https://doi.org/10.1080/15412555.2021.2000957 .
Introduction
Chronic obstructive pulmonary disease (COPD) is characterised by persistent and progressive airflow limitation [Citation1]. COPD is estimated to affect 11.7% of the global population, although these figures may be an underestimate due to rapidly increasing prevalence and underdiagnosis [Citation2–4]. Patients with COPD experience acute exacerbations (AECOPD), recognised clinically by deviation from usual sputum volume, sputum purulence and breathlessness [Citation5]. AECOPD may be infective or non-infective; infective causes comprise bacterial, viral and bacterial-viral coinfection [Citation6]. The airways of patients with COPD are more susceptible to bacterial infection as a result of impaired barrier and innate immune cell function [Citation7]. In a cyclical manner, airway bacteria influence bacterial acquisition or expansion by promoting inflammation and directly impairing host defences [Citation7]. This, combined with excessive mucus production and reduced mucous means that COPD airways provide a fertile environment for bacteria. Accordingly, bacteria are detected in approximately 50% of AECOPD cases, prompting antibiotic use as a management strategy in primary care and COPD patients consequently receive 3 times more antibiotic prescriptions than the general public [Citation8,Citation9].
A primary concern of antibiotic use in COPD, is the emergence and propagation of antimicrobial resistance (AMR). AMR can be defined according to microbiological or clinical resistance, but is considered here to denote any reduction in susceptibility in a bacterial strain compared to the susceptible wildtype [Citation10,Citation11]. AMR is recognised as a global threat and an NHS and UK government priority to reduce [Citation12–14]. Besides frequent antibiotic provision, the COPD lung facilitates the development of AMR by being permissive towards the formation of biofilms, which limit antimicrobial infiltration and induce a phenotypically quiescent bacterial phenotype which persist and develop multidrug resistance [Citation15]. Owing to the extent of antibiotic exposure, long-term prophylactic therapy puts the greatest pressure on AMR development. This is worsened by the relatively long half-life of azithromycin which may lead to prolonged suboptimal concentrations of the antibiotic, potentially below the minimum inhibitory concentration (MIC) [Citation16]. Furthermore, the use of chronic macrolide therapy for chronic respiratory diseases may link to population-level resistance [Citation17]. Understanding AMR in COPD is therefore of importance for both public and individual health interventions.
In order to address the heterogeneous nature of the literature and guide future work, we aimed to systematically collate the evidence concerning the prevalence, pattern, risk factors and consequences of AMR in COPD.
Methods
The protocol for this review was registered with PROSPERO (2020:CRD42020218684) prior to commencing work [Citation18].
Inclusion/exclusion criteria
Study designs eligible were randomised controlled trials (RCTs), cohort studies, case control studies, case series ≥10 cases and systematic reviews. Duplicate publications or publications using the same dataset e.g. sub-group analyses, editorials and non-systematic reviews were excluded. Studies published only in abstract form dated before 2017 were excluded. The population, intervention, comparator, outcome and study design (PICOS) framework was used to define the inclusion criteria. Included patients had a clinical diagnosis of COPD. Studies of chronic bronchitis (CB) or emphysema were also included. In the event of identifying studies with mixed populations, studies were included if data from COPD patients was presented separately. Patients with a primary clinical diagnosis of bronchiectasis were excluded. Included studies may have had intervention with an antibiotic, or could be observational in nature, in order to identify reports of the prevalence and pattern of AMR, irrespective of antibiotic use. In studies using antibiotics as the intervention or reporting on history of antibiotic use, the prevalence of antibiotic resistance in intervention versus control arms was considered, as was the association of previous antibiotic exposure on AMR rates. The comparator was placebo or usual care or none. This allowed inclusion of cohorts with relevant prevalence data.
Outcomes
The primary outcome was the prevalence and pattern of resistant isolates, as measured by rate of resistance of selected microorganisms to selected antibiotic. Microorganisms and antibiotics were selected on the basis of clinical relevance and pilot searches. Resistance rates of the following bacteria to the following antibiotics were recorded:
Bacteria:Haemophilus influenzae; Pseudomonas aeruginosa ; Streptococcus pneumoniae; Staphylococcus aureus; Moraxella catarrhalis ; Klebsiella pneumoniae; Acinetobacter baumannii.
Antibiotics:Penicillin; Ampicillin; Amoxicillin; Co-Amoxiclav; Tetracycline; Doxycycline; Levofloxacin; Ciprofloxacin; Azithromycin; Erythromycin; Clarithromycin; Cefuroxime; Piperacillin/Tazobactam; Colistin.
Secondary outcomes and measures of these are presented in .
Table 1. Antimicrobial resistances rates in non-hospitalised patients.
Search strategy
Bibliographic databases were searched from inception to 02 November 2020 with no language restrictions. The Ovid interface was used to search MEDLINE and EMBASE. The Cochrane Central Register of Controlled Trials (CENTRAL) and ClinicalTrials.gov were used to search for ongoing trials. The EBSCO interface was used to search CINAHL. Reference lists (e.g. from reviews) were searched manually to identify additional studies. The search strategy included terms relating to or describing COPD and AMR, including relevant synonyms and can be found in the supplementary material. Search limits were applied to include human studies only. Non-English texts were translated using a combination of online language translation services with input from native speakers when required.
Study selection, quality assessment and data extraction
Standard systematic review methodology aimed at minimising bias was employed, in accordance with guidance from the Cochrane Handbook of Systematic Reviews [Citation19]. Following searches, duplicates were electronically identified and removed. Titles and/or abstracts were screened by two independent review authors with a similar process at full text (DS, AG and LH). Any disagreement over the eligibility of particular studies at both title/abstract and full text review stage was resolved by discussion with a third reviewer, AT. Any missing or additional required data was requested from the studies’ corresponding authors.
During the full text review, DS, AG and LH independently assessed the risk of bias in the included studies. The risk of bias in included RCTs was assessed using the Cochrane Collaboration’s Risk of Bias tool (RoB 2.0 tool) [Citation20]. The risk of bias in included prevalence studies was assessed using the Joanna Briggs Institute Critical Appraisal Checklist for Prevalence Studies [Citation21]. This tool was also used for non-RCT studies where extraction of AMR prevalence was our primary interest.
A standardised form was created and used by DS to extract data from included studies for evidence synthesis and assessment of study quality. Full details of fields for data extraction are in the supplement.
Data synthesis
A narrative synthesis was produced together with summary statistics of resistance rates of selected bacteria to selected antibiotics in each major setting. Studies contributing antibiotic susceptibility testing in ≥10 samples per antibiotic per microorganism were used to calculate summary statistics (median and range of resistance rates (%)).
Results
Search results
A total of 3506 records were identified. After duplicate removal 2748 titles and abstracts were screened for inclusion. 231 articles were reviewed in full and 63 articles were judged to meet the inclusion criteria (), including 26,387 COPD patients. 58 studies were published primary research, 3 articles were published only in abstract or poster form and 2 articles were ongoing clinical trials. A summary of characteristics of studies is in . Many studies failed to report data for lung function and other baseline demographic information. Types of sample used for testing and general methods for assessment of AMR varied and are shown in . Five studies reported data on AMR genes only; this data is reported in the supplement since it is unclear whether it fully relates to resistance in clinical practice.
Figure 2. Visual representation of distribution of: (A) origin of sample for antimicrobial susceptibility testing, (B) antibiotic susceptibility testing technique and (C) breakpoint antimicrobial susceptibility guidelines used, within included studies. CLSI, Clinical Laboratory Standards Institute; NCCLS, National Committee on Clinical Laboratory Standards; EUCAST, European Committee on Antimicrobial Susceptibility Testing.
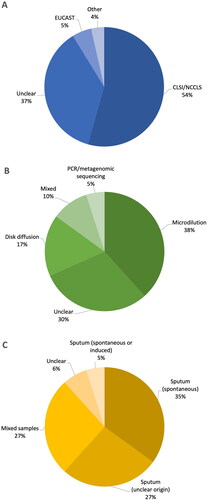
Table 2. Risk of bias in included RCTs.
Prevalence of AMR
Forty-one (65.1%) studies included results of antibiotic susceptibility testing. Studies of hospitalised AECOPD comprised the majority of studies within the review (n = 26, ), followed by non-hospitalised setting (n = 17, ), mixed/unclear settings (n = 14, supplementary material Table 4) and ICU (n = 6, supplementary material Table 5). Across all settings, resistance of H influenzae to amoxicillin was ≤40%, and lower to co-amoxiclav, as expected. Ten out of 19 (53%) of the median resistance rates calculated for P. aeruginosa across all settings were >50%, of which 7 were 100%. Lower median rates of AMR were observed for P. aeruginosa towards colistin and piperacillin/tazobactam, as expected. Variable patterns of resistance were seen for S pneumoniae ranging from 0% to 46%, greater for macrolide and tetracycline antibiotics than other antibiotic classes, and higher in hospitalised compared to non-hospitalised patients. AMR rates were generally low for M catarrhalis (≤26%), with the exception of penicillin, amoxicillin and ampicillin, as expected. Antimicrobial susceptibility rates were available for S. aureus isolates from hospitalised and ICU settings only, with AMR generally being higher in ICU cases. A. baumannii was also studied in hospitalised and ICU settings, while K. pneumoniae was only studied in the hospitalised setting.
Table 3. Risk of bias in included non-randomised studies.
Risk factors for AMR
Eight studies reported on the relationship between antibiotic exposure and AMR rates. Three studies identified higher rates of previous antimicrobial prescriptions in patients with multidrug resistant (MDR) microorganisms detected in sputum when compared to patients with susceptible isolates [Citation22–24]. Higher rates of antibiotic prescriptions in the previous 3 months were seen in hospitalised patients with P. aeruginosa-resistant compared to P. aeruginosa-sensitive isolates (77% vs. 33%, p = 0.01) [Citation25]. Brill et al. analysed 243 isolates from 69 non-hospitalised COPD patients whom underwent antimicrobial susceptibility testing at baseline and following treatment with either moxifloxacin, azithromycin, doxycycline or placebo [Citation26]. At 13 weeks, each antibiotic was associated with a ≥3-fold increase in MIC compared to baseline with a parallel increase in clinical resistance in patients assigned to those antibiotics compared with placebo (p = 0.01 for all). Similarly, 54.4% of pneumococcal isolates from patients exposed to macrolides in the previous 3 months exhibited resistance to erythromycin versus 18.7% of isolates from non-exposed patients (p < 0.05) [Citation27]. Higher frequencies of β-lactamase-negative ampicillin-resistant strains have also been reported in cultures isolated from patients with a history of repeated antibiotic prescriptions [Citation28].
Six studies reported on the isolation of AMR microorganisms following long-term azithromycin therapy. Albert et al. observed no difference in the overall prevalence of macrolide resistance in patients receiving azithromycin vs. placebo (52% vs. 57%; p = ns) [Citation29]. However, patients in the azithromycin group who were not colonised at baseline were more likely to become colonised during the trial with macrolide resistant microorganisms (81% vs. 41%; p < 0.001) [Citation29]. Similarly a retrospective study of cyclical azithromycin was observed to increase the rate of detection of macrolide resistant organisms compared to baseline (9/18 vs. 1/52) [Citation30]. Conversely, Uzun et al. observed that the detection of macrolide resistant bacteria was significantly lower in patients receiving azithromycin compared to placebo (6% vs. 25%, respectively, p = 0.036) [Citation31]. In another study, the number of patients receiving prophylactic macrolide therapy did not differ significantly between patients with sensitive versus resistant pseudomonas isolates [Citation25]. Pettigrew et al. observed a four-fold increase in macrolide MIC in 19% of H. influenzae strains, which persisted following exposure to macrolides [Citation32]. Brill et al. noted increases in MIC and clinical resistance in patients treated for 13 weeks with azithromycin [Citation26].
Four studies reported a relationship between lung function and AMR; in general more severe COPD was associated with higher AMR rates. Three studies reported a relationship between frequent hospitalisation and higher rates of AMR, and studies of ICU care also supported a role for intubation and length of stay with worsening AMR rates. Smoking and demographic features exhibited inconsistent patterns of association with AMR. These results are shown in more detail in the supplement.
Impact of AMR
Five studies reported on the effect of AMR on mortality rate [Citation23–25,Citation33,Citation34]. There were no clear trends identified across the included studies. Three studies reported on the impact of AMR on duration of inpatient admission, two showing no difference [Citation23,Citation35] and one suggesting stay was longer by 3 days [Citation36]. There were no differences observed in the rate of ICU admission, invasive or non-invasive mechanical ventilation between patients with microorganisms resistant to conventional antibiotic treatment compared to patients with susceptible and negative isolates [Citation23]. AMR appeared to have no effect on future AECOPD frequency [Citation23]. No data was available on the relationship between the incidence of AMR and chronic bacterial colonisation, quality of life or disease progression.
Study quality and bias
Variability in sample description and testing methods contributed to bias across studies in general (). Most studies displayed moderate risks of bias. These are summarised in and .
Discussion
This review has demonstrated that AMR is relatively common in COPD, in particular during AECOPD. However, study of AMR has been driven by acute antibiotic studies, such that the impact of AMR at population level remains poorly described and may need to be a focus for future work. Putative risk factors for AMR such as long term or repeated acute antibiotic use (see also introduction) were confirmed by the data in included studies.
It is well recognised that H influenzae, S pneumoniae and M catarrhalis are the most commonly isolated PPMs in both the stable and exacerbated COPD state. The higher levels of resistance of H influenzae and M catarrhalis to beta-lactam antibiotics in likely reflects the increasing global prevalence of beta-lactamase producing microorganisms, especially M. catarrhalis, of which 95% of global clinical isolates were observed to be beta-lactamase produces [Citation37–39]. In the UK, the recommended first line antibiotics for AECOPD are amoxicillin, doxycycline and clarithromycin [Citation40]. However, we found that at least one of H. influenzae, S. pneumoniae and M. catarrhalis to show high levels of resistance to at least one of these antibiotics, which likely contributes to treatment failure and facilitates emergence of resistance. Accordingly, this represents a substantial barrier to the effectiveness of empirical antibiotic prescribing.
S. aureus was observed to have very high median rates of resistance in this review, particularly in the ICU setting, with all median resistance rates ≥90%. While the lack of susceptibility testing results for S. aureus in the non-hospitalised setting does not imply its absence in this setting, previous evidence has demonstrated low prevalence in the community [Citation41]. Furthermore, S. aureus is not typically considered to be a pathogen of major importance in COPD, unlike CF, in which S. aureus, particularly resistant strains, have been shown to associate with disease progression [Citation42–46]. Therefore, while the high rates of resistance of S. aureus in this study should be viewed with caution, the clinical implication may be less than for other pathogens. Susceptibility rates of K. pneumoniae and A. baumannii were infrequently reported, making it hard to compare resistance rates between study settings. Of particular note, high median resistance rates were observed for A. baumannii in the ICU setting. While previous evidence implicates colistin as the most effective antimicrobial for A. baumannii eradication, we were only able to corroborate this finding in the hospitalised setting, owing to lack of susceptibility data in other settings [Citation47]. In fact, across all bacteria, there was a tendency for greater rates of susceptibility to colistin and piperacillin/tazobactam, supporting the roles for these antibiotics in severe AECOPD cases, especially those with P. aeruginosa infection [Citation40,Citation48]. Perhaps reflecting the severity of AECOPD encountered in non-hospitalised setting, we observed little evidence of colistin and piperacillin/tazobactam susceptibility testing in this setting, but surprisingly found no evidence of susceptibility testing in the ICU, where these antibiotics may be most required. Susceptibly to quinolones was generally high, with the exception of S. aureus in the ICU setting, in agreement with previous studies [Citation49,Citation50].
Our review has confirmed that use of antibiotics appears to drive AMR in COPD, at least when looking at data for Pseudomonas and for various species with regard to macrolides. Despite the lack of clear or high-quality evidence [Citation51], antibiotics are used very frequently in COPD, with approximately 50% of AECOPD treated with antibiotics in primary care [Citation8]. This use of antibiotics is not without consequence. Aside from resistance, adverse effects include diarrhoea and Clostridium difficile infection thus guidelines emphasise the use of factors such as biomarkers or clinical signs to optimise antibiotic use in COPD [Citation1,Citation52,Citation53].
We had hypothesised that AMR would be associated with poorer outcomes for patients, but were not able to prove this in the published works. Nevertheless hints that length of stay may be longer, and antibiotic choices more limited in primary care are a concern. Our review supports the fact that AMR is an increasing problem, such that stewardship is important if we are to reduce impact of this on clinical care—already where quinolone resistance rates are high, for example, treatment with intravenous agents may mandate admission for treatment in many healthcare systems.
The data was highly variable but use of median resistance rates assisted the interpretation of results. Variability of data may relate to a number of factors, not least the variations in definitions of COPD and AECOPD, disease severity, geographical location of the studies, year of publication, sample size and antimicrobial susceptibility testing (AST) technique. We noted substantial variation in AST technique, origin of sample and breakpoint guidelines used. The lack of reporting and standardisation between AST techniques and breakpoint guidelines hinders ability of researchers, including ourselves, to compare results across settings and disease state. Future studies should strive to employ standardised techniques and methodology; the advent of application of metagenomic approaches to AMR may play a role in this regard, because of their ability to screen the full microbiome.
A key strength of our study is that by including a broad range of search terms, reviewing references of included works, and employing few restrictions other than human studies we are confident our searches should have retrieved all relevant data. We also included a comprehensive range of outcomes, thus aiding identification of future areas of priority for research. As with all systematic reviews we were limited by the quality and indexing of relevant studies, and it was primarily the variable quality and type of reporting in included works which limited the conclusions we could draw. Furthermore, by only reporting PPMs, we are unable to determine the extent of AMR in non-PPMs. It has recently been demonstrated than COPD microbiome acts as a reservoir for AMR genes, particularly macrolide resistant genes harboured by Streptococcus and Actinomyces gena, independent of antibiotic exposure [Citation54]. Although clinical utility remains low at present, it is likely that metagenomic sequencing approaches may become the optimum method for investigating the airway microbiome, dispersion of AMR genes and inter-species interactions which may play a key role in AMR. Such methods may also overcome some measurement inconsistencies encountered by this review [Citation54]. We suggest that the framework we used here to present data by organism and drug class may be a starting point for such a consensus to be drawn up on AMR reporting.
Conclusion
AMR is common in COPD, driven by antibiotic use, and may be associated with adverse clinical consequences for patients. Standardised reporting of AMR rates in all future antibiotic studies in COPD could help to quantify the problem fully. The framework we used here to present data by organism and drug class may be a starting point for such a consensus to be drawn up.
Disclosure statement
The authors report no conflict of interest.
Additional information
Funding
References
- Global Initiative for Chronic Obstructive Pulmonary Disease. Global strategy for the diagnosis, management, and prevention of chronic obstructive pulmonary disease (2021 report). 2020.
- British Lung Foundation. Chronic Obstructive Pulmonary Disease (COPD) statistics [cited 2020 Nov 7]. Available from: https://statistics.blf.org.uk/copd.
- Adeloye D, Chua S, Lee C, Global Health Epidemiology Reference Group (GHERG), et al. Global and regional estimates of COPD prevalence: Systematic review and meta-analysis. J Glob Health 2015;5(2):020415. DOI:https://doi.org/10.7189/jogh.05-020415
- Bednarek M, Maciejewski J, Wozniak M, et al. Prevalence, severity and underdiagnosis of COPD in the primary care setting. Thorax 2008;63(5):402–407. DOI:https://doi.org/10.1136/thx.2007.085456
- Anthonisen NR, Manfreda J, Warren CP, et al. Antibiotic therapy in exacerbations of chronic obstructive pulmonary disease. Ann Intern Med. 1987; 201106(2):196–204. DOI:https://doi.org/10.7326/0003-4819-106-2-196
- Papi A, Bellettato CM, Braccioni F, et al. Infections and airway inflammation in chronic obstructive pulmonary disease severe exacerbations. Am J Respir Crit Care Med. 2006;173(10):1114–1121. DOI:https://doi.org/10.1164/rccm.200506-859OC
- Sethi S, Murphy TF. Bacterial infection in chronic obstructive pulmonary disease in 2000: a state-of-the-art review. Clin Microbiol Rev. 2001;14(2):336–363. DOI:https://doi.org/10.1128/CMR.14.2.336-363.2001
- Roede BM, Bindels PJ, Brouwer HJ, et al. Antibiotics and steroids for exacerbations of COPD in primary care: Compliance with Dutch guidelines. Br J Gen Pract. 2006;56(530):662–665.
- Shallcross L, Beckley N, Rait G, et al. Antibiotic prescribing frequency amongst patients in primary care: a cohort study using electronic health records. J Antimicrob Chemother. 2017;72(6):1818–1824. DOI:https://doi.org/10.1093/jac/dkx048
- Davison HC, Low JC, Woolhouse ME. What is antibiotic resistance and how can we measure it? Trends Microbiol. 2000;8(12):554–559. DOI:https://doi.org/10.1016/S0966-842X(00)01873-4
- Kraemer SA, Ramachandran A, Perron GG. Antibiotic pollution in the environment: from microbial ecology to public policy. Microorganisms 2019;7(6): 180.DOI:https://doi.org/10.3390/microorganisms7060:
- World Health Organisation. Global action plan on antimicrobial resistance [2021 Mar 17]. Available at: https://www.who.int/publications/i/item/9789241509763. Updated 2016. .
- NHS England. Fighting Antimicrobial Resistance [2021 Mar4]. Available at: https://www.england.nhs.uk/patient-safety/fighting-antimicrobial-resistance/.
- HM Government. Department of Health and Social Care. Contained and controlled: The UK’s 20-year vision for antimicrobial resistance [2021 Mar 3]. Available at: https://www.gov.uk/government/publications/uk-20-year-vision-for-antimicrobial-resistance.
- Kyd JM, McGrath J, Krishnamurthy A. Mechanisms of bacterial resistance to antibiotics in infections of COPD patients. Curr Drug Targets 2011;12(4):521–530. DOI:https://doi.org/10.2174/138945011794751519
- Nightingale CH. Pharmacokinetics and pharmacodynamics of newer macrolides. Pediatr Infect Dis J. 1997;16(4):438–443. DOI:https://doi.org/10.1097/00006454-199704000-00027
- Serisier DJ. Risks of population antimicrobial resistance associated with chronic macrolide use for inflammatory airway diseases. Lancet Respir Med. 2013;1(3):262–274. DOI:https://doi.org/10.1016/S2213-2600(13)70038-9
- PROSPERO. The prevalence, pattern and impact of antibiotic resistance in COPD: A systematic review 2020 (CRD42020218684). [2021 Mar 10]. Available at: https://www.crd.york.ac.uk/prospero/display_record.php?ID=CRD42020218684.
- Higgins J, Thomas J, Chandler J, et al. Cochrane handbook for systematic reviews of interventions version 6.2. [2021 Mar 10]. Available at: www.training.cochrane.org/handbook.
- Sterne JAC, Savović J, Page MJ, et al. RoB 2: a revised tool for assessing risk of bias in randomised trials. BMJ. 2019;366:l4898. DOI:https://doi.org/10.1136/bmj.l4898
- Munn Z, Moola S, Lisy K, et al. Methodological guidance for systematic reviews of observational epidemiological studies reporting prevalence and cumulative incidence data. Int J Evid Based Healthcare 2015;13(3):147–153. DOI:https://doi.org/10.1097/XEB.0000000000000054
- Guo Z, Chang C, Chen Y, et al. [bacteriology in acute exacerbation in patients hospitalized frequently for acute exacerbation of chronic obstructive pulmonary disease. Zhonghua Yi Xue Za Zhi 2014;94(10):729–732 (in Chinese).
- Estirado C, Ceccato A, Guerrero M, et al. Microorganisms resistant to conventional antimicrobials in acute exacerbations of chronic obstructive pulmonary disease. Respir Res. 2018;19(1):119. DOI:https://doi.org/10.1186/s12931-018-0820-1
- Nseir S, Di Pompeo C, Cavestri B, et al. Multiple-drug-resistant bacteria in patients with severe acute exacerbation of chronic obstructive pulmonary disease: Prevalence, risk factors, and outcome. Crit Care Med. 2006;34(12):2959–2966. DOI:https://doi.org/10.1097/01.CCM.0000245666.28867.C6
- Rodrigo-Troyano A, Suarez-Cuartin G, Peiró M, et al. Pseudomonas aeruginosa resistance patterns and clinical outcomes in hospitalized exacerbations of COPD. Respirology. 2016;21(7):1235–1242. DOI:https://doi.org/10.1111/resp.12825
- Brill SE, Law M, El-Emir E, et al. Effects of different antibiotic classes on airway bacteria in stable COPD using culture and molecular techniques: a randomised controlled trial. Thorax. 2015;70(10):930–938. DOI:https://doi.org/10.1136/thoraxjnl-2015-207194
- Desai H, Richter S, Doern G, et al. Antibiotic resistance in sputum isolates of Streptococcus pneumoniae in chronic obstructive pulmonary disease is related to antibiotic exposure. COPD. 2010;7(5):337–344. DOI:https://doi.org/10.3109/15412555.2010.510162
- Inoue S, Watanuki Y, Miyazawa N, et al. High frequency of β-lactamase-negative, ampicillin-resistant strains of Haemophilus influenzae in patients with chronic bronchitis in Japan. J Infect Chemother. 2010;16(1):72–75. DOI:https://doi.org/10.1007/s10156-009-0020-5
- Albert RK, Connett J, Bailey WC, et al. Azithromycin for prevention of exacerbations of COPD [published correction appears in N Engl J med. 2012;366(14):1356]. N Engl J Med. 2011;365(8):689–698. DOI:https://doi.org/10.1056/NEJMoa1104623
- Pomares X, Montón C, Bullich M, et al. Clinical and safety outcomes of long-term azithromycin therapy in severe COPD beyond the first year of treatment. Chest 2018;153(5):1125–1133. DOI:https://doi.org/10.1016/j.chest.2018.01.044
- Uzun S, Djamin RS, Kluytmans JA, et al. Azithromycin maintenance treatment in patients with frequent exacerbations of chronic obstructive pulmonary disease (COLUMBUS): a randomised, double-blind, placebo-controlled trial. Lancet Respir Med. 2014;2(5):361–368. DOI:https://doi.org/10.1016/S2213-2600(14)70019-0
- Pettigrew MM, Tsuji BT, Gent JF, et al. Effect of fluoroquinolones and macrolides on eradication and resistance of Haemophilus influenzae in chronic obstructive pulmonary disease. Antimicrob Agents Chemother. 2016;60(7):4151–4158. DOI:https://doi.org/10.1128/AAC.00301-16
- Montero M, Domínguez M, Orozco-Levi M, et al. Mortality of COPD patients infected with multi-resistant pseudomonas aeruginosa: a case and control study. Infection 2009;37(1):16–19. DOI:https://doi.org/10.1007/s15010-008-8125-9
- Pan W, Yu J, Zhang L, et al. Predictors of in-hospital mortality in patients with acute exacerbation of COPD requiring ventilation: a retrospective study. Int J Clin Exp Med. 2016;9(11):22093–22101.
- Vitacca M, Marino S, Comini L, et al. Bacterial colonization in COPD patients admitted to a rehabilitation respiratory unit and impact on length of stay: a real-life study. COPD. 2018;15(6):581–587. DOI:https://doi.org/10.1080/15412555.2019.1572731
- Liu H, Zheng D, Lin Y, et al. Association of sputum microbiome with clinical outcome of initial antibiotic treatment in hospitalized patients with acute exacerbations of COPD. Pharmacol Res. 2020;160:105095 DOI:https://doi.org/10.1016/j.phrs.2020.105095
- Bae S, Lee J, Lee J, et al. Antimicrobial resistance in haemophilus influenzae respiratory tract isolates in korea: Results of a nationwide acute respiratory infections surveillance. Antimicrob Agents Chemother. 2010;54(1):65–71. DOI:https://doi.org/10.1128/AAC.00966-09
- Hasegawa K, Chiba N, Kobayashi R, et al. Rapidly increasing prevalence of beta-lactamase-nonproducing, ampicillin-resistant Haemophilus influenzae type b in patients with meningitis. Antimicrob Agents Chemother. 2004;48(5):1509–1514. DOI:https://doi.org/10.1128/aac.48.5.1509-1514.2004
- Khan MA, Northwood JB, Levy F, et al. Bro {beta}-lactamase and antibiotic resistances in a global cross-sectional study of Moraxella catarrhalis from children and adults. J Antimicrob Chemother. 2010;65(1):91–97. DOI:https://doi.org/10.1093/jac/dkp401
- National Institute for Health and Care Excellence. Chronic obstructive pulmonary disease (acute exacerbation): antimicrobial prescribing NICE guideline [NG114]. [internet]. London: National Institute for Health and Care Excellence; 2018. [cited 2021 mar 19]. Available from: www.nice.org.uk/guidance/ng114
- Shimizu K, Yoshii Y, Morozumi M, et al. Pathogens in COPD exacerbations identified by comprehensive real-time PCR plus older methods. Int J Chron Obstruct Pulmon Dis. 2015;10:2009–2016. DOI:https://doi.org/10.2147/COPD.S82752
- Siddiqi A, Sethi S. Optimizing antibiotic selection in treating COPD exacerbations. Int J Chron Obstruct Pulmon Dis. 2008;3(1):31–44. DOI:https://doi.org/10.2147/copd.s1089
- Sethi S. Bacteria in exacerbations of chronic obstructive pulmonary disease: phenomenon or epiphenomenon? Proc Am Thorac Soc. 2004;1(2):109–114. DOI:https://doi.org/10.1513/pats.2306029
- Sawicki GS, Rasouliyan L, Pasta DJ, et al. The impact of incident methicillin resistant S. aureus detection on pulmonary function in cystic fibrosis. Pediatr Pulmonol. 2008;43(11):1117–1123. DOI:https://doi.org/10.1002/ppul.20914
- Goss CH, Muhlebach MS. Review: S. aureus and MRSA in cystic fibrosis. J Cyst Fibros. 2011;10(5):298–306. DOI:https://doi.org/10.1016/j.jcf.2011.06.002
- Cogen J, Emerson J, Sanders DB, et al., for the EPIC Study Group. Risk factors for lung function decline in a large cohort of young cystic fibrosis patients. Pediatr Pulmonol. 2015;50(8):763–770. DOI:https://doi.org/10.1002/ppul.23217
- Sieniawski K, Kaczka K, Rucińska M, et al. Acinetobacter baumannii nosocomial infections. Pol Przegl Chir. 2013;85(9):483–490. DOI:https://doi.org/10.2478/pjs-2013-0075
- Montón C, Prina E, Pomares X, et al. Nebulized colistin and continuous cyclic azithromycin in severe COPD patients with chronic bronchial infection due to Pseudomonas aeruginosa: a retrospective cohort study. Int J Chron Obstruct Pulmon Dis. 2019;14:2365–2373. DOI:https://doi.org/10.2147/COPD.S209513
- Iffat W, Shoaib MH, Muhammad IN, et al. Antimicrobial susceptibility testing of newer quinolones against gram positive and gram negative clinical isolates. Pak J Pharm Sci. 2010;23(3):245–249.
- Kim ES, Hooper DC. Clinical importance and epidemiology of quinolone resistance. Infect Chemother. 2014;46(4):226–238. DOI:https://doi.org/10.3947/ic.2014.46.4.226
- Vollenweider DJ, Frei A, Steurer-Stey C, et al. Antibiotics for exacerbations of chronic obstructive pulmonary disease. Cochrane Database Syst Rev. 2018;10(10):CD010257. DOI:https://doi.org/10.1002/14651858.CD010257.pub2
- National Institute for Health and Care Excellence. Chronic obstructive pulmonary disease in over 16s: Diagnosis and management. NICE guidelines NG115. [internet]. London: National Institute for Health and Care Excellence; 2018. [cited 2021 mar 16]. Available from: www.nice.org.uk/guidance/ng115.
- Zhang H, Tan M, Qiu A, et al. Antibiotics for treatment of acute exacerbation of chronic obstructive pulmonary disease: a network Meta-analysis. BMC Pulm Med. 2017;17(1) DOI:https://doi.org/10.1186/s12890-017-0541-0
- Mac Aogáin M, Lau KJX, Cai Z, et al. Metagenomics reveals a core macrolide resistome related to microbiota in chronic respiratory Disease. Am J Respir Crit Care Med. 2020;202(3):433–447. DOI:https://doi.org/10.1164/rccm.201911-2202OC
- Maddi S, Kolsum U, Jackson S, et al. Ampicillin resistance in haemophilus influenzae from COPD patients in the UK. Int J COPD. 2017;12:1507–1518. DOI:https://doi.org/10.2147/COPD.S135338
- Pettigrew MM, Gent JF, Revai K, Patel JA, Chonmaitree T. Microbial interactions during upper respiratory tract infections. Emerg Infect Dis. 2008;14(10):1584–1591. DOI:https://doi.org/10.3201/eid1410.080119
- Pfaller MA, Ehrhardt AF, Jones RN. Frequency of pathogen occurrence and antimicrobial susceptibility among community-acquired respiratory tract infections in the respiratory surveillance program study: Microbiology from the medical office practice environment. Am J Med. 2001;111:4S–38S.
- Pfaller MA, Jones RN. Gatifloxacin phase IV surveillance trial (TeqCES study) utilizing 5000 primary care physician practices: Report of pathogens isolated and susceptibility patterns in community-acquired respiratory tract infections. Diag Microbiol Infect Dis. 2002;44(1):77–84. DOI:https://doi.org/10.1016/s0732-8893(02)00446-7
- Querol-Ribelles J, Molina J, Naberan K, Esteban E, Herreras A, Garcia-de-Lomas J. Discrepancy between antibiotics administered in acute exacerbations of chronic bronchitis and susceptibility of isolated pathogens in respiratory samples: Multicentre study in the primary care setting. Int J Antimicrob Agents. 2006;28(5):472–476. DOI:https://doi.org/10.1016/j.ijantimicag.2006.05.034
- Gallego M, Pomares X, Espasa M, et al. Pseudomonas aeruginosa isolates in severe chronic obstructive pulmonary disease: Characterization and risk factors. BMC Pulm Med. 2014;14(1):103. DOI:https://doi.org/10.1186/1471-2466-14-103
- DeAbate CA, Bettis R, Munk ZM, et al. Effectiveness of short-course therapy (5 days) with grepafloxacin in the treatment of acute bacterial exacerbations of chronic bronchitis. Clin Ther. 1999;21(1):172–188. DOI:https://doi.org/10.1016/S0149-2918(00)88277-6
- Grossman RF, Beaupre A, LaForge J, Lampron N, Hanna K. A prospective randomised parallel single-blind comparison of oral ciprofloxacin with oral cotrimoxazole in the treatment of respiratory tract infections in patients with chronic obstructive lung disease. Drug Invest. 1994;8(2):110–117. DOI:https://doi.org/10.1007/BF03257434
- Kahn JB, Khashab M, Ambruzs M. Study entry microbiology in patients with acute bacterial exacerbation of chronic bronchitis in a clinical trial stratifying by disease severity. Curr Med Res Opin. 2007;23(1):1–7. DOI:https://doi.org/10.1185/030079907X159515
- Agmy G, Mohamed S, Gad Y, Farghally E, Mohammedin H, Rashed H. Bacterial profile, antibiotic sensitivity and resistance of lower respiratory tract infections in upper Egypt. Mediterranean J Hematol Infect Dis. 2013;5(1). DOI:https://doi.org/10.4084/mjhid.2013.056
- Aogain MM, Zhao C, Purbojati RW, et al. The airway ‘resistome’ in chronic respiratory disease: A metagenomics approach. European Respiratory Journal. Conference: 29th International Congress of the European Respiratory Society, ERS, Spain. 2019;54.
- Blasi F, Farrell DJ, Dubreuil L. Antibacterial activity of telithromycin and comparators against pathogens isolated from patients with community-acquired respiratory tract infections: The prospective resistant organism tracking and epidemiology for the ketolide telithromycin study year 5 (2003–2004). Diagn Microbiol Infect Dis. 2009;63(3):302–308. DOI:https://doi.org/10.1016/j.diagmicrobio.2008.11.012
- Butt HL, Cripps AW, Clancy RL. In vitro susceptibility patterns of nonserotypable haemophilus influenzae from patients with chronic bronchitis. Pathology. 1997;29(1):72–75. DOI:https://doi.org/10.1080/00313029700169584
- Casellas JM, Gilardoni M, Tome G, et al. Comparative in-vitro activity of levofloxacin against isolates of bacteria from adult patients with community-acquired lower respiratory tract infections. J Antimicrob Chemother. 1999;43:37–42. DOI:https://doi.org/10.1093/jac/43.suppl_3.37
- Chen L, Xie Z, Yang Y. Composition of pathogenic bacteria of chronic obstructive pulmonary disease patients and drug resistance of gram-negative bacilli for various antibiotics. Biomed Res. 2018;29(4) DOI:https://doi.org/10.4066/biomedicalresearch.29-17-3313
- Ding YL, Yao WZ, Zhou QT, Chen YH, Ning YZ. Effect of mechanical ventilation beginning time after admission and ventilation duration on pathogens and antibiotic susceptibility of lower respiratory tract infection in chronic obstructive pulmonary disease patients [Chinese]. Chin J Antibiot. 2011;36(2):154–159
- Domenech A, Puig C, Martí S, et al. Infectious etiology of acute exacerbations in severe COPD patients. J Infect. 2013;67(6):516–523. DOI:https://doi.org/10.1016/j.jinf.2013.09.003
- Domenech A, Ardanuy C, Calatayud L, et al. Serotypes and genotypes of streptococcus pneumoniae causing pneumonia and acute exacerbations in patients with chronic obstructive pulmonary disease. J Antimicrob Chemother. 2011;66(3):487–493. DOI:https://doi.org/10.1093/jac/dkq480
- Domenech A, Ardanuy C, Pallares R, et al. Some pneumococcal serotypes are more frequently associated with relapses of acute exacerbations in COPD patients. PLoS One. 2013;8(3):e59027 DOI:https://doi.org/10.1371/journal.pone.0059027
- Domenech A, Ardanuy C, Tercero A, Garcia-Somoza D, Santos S, Linares J. Dynamics of the pneumococcal population causing acute exacerbations in COPD patients in a Barcelona hospital (2009–12): Comparison with 2001-04 and 2005-08 periods. J Antimicrob Chemother. 2014;69(4):932–939. DOI:https://doi.org/10.1093/jac/dkt476
- Engler K, Muhlemann K, Garzoni C, Pfahler H, Geiser T, Von Garnier C. Colonisation with pseudomonas aeruginosa and antibiotic resistance patterns in COPD patients. Swiss Med Wkly. 2011;142. DOI:https://doi.org/10.4414/smw.2012.13509
- Erkan L, Uzun O, Findik S, Katar D, Sanic A, Atici AG. Role of bacteria in acute exacerbations of chronic obstructive pulmonary disease. Int J COPD. 2008;3(3):463–467
- Ewig S, Soler N, Gonzalez J, Celis R, El-Ebiary M, Torres A. Evaluation of antimicrobial treatment in mechanically ventilated patients with severe chronic obstructive pulmonary disease exacerbations. Crit Care Med. 2000;28(3):692–697
- Firdaus H, Khan NA, Fatima N, Shameem M, Bhargava R, Ahmad Z. Acute exacerbation of chronic obstructive pulmonary disease: Phenotypic screening and sensitivity of microbiological profile. J Clin Diagn Res. 2020; 14(6):LC15–LC20.
- Glogovska PT, Ivanova ZI, Popova TP, et al. Sputum isolates from ambulatory patients with chronic obstructive pulmonary disease—frequent pathogens and antibiotic resistance. J IMAB - Ann Proceed. 2015;21(2):770–774. DOI:https://doi.org/10.5272/jimab.2015212.770
- Grochowalska A, Koziol-Montewka M, Sobieszczanska A. Analysis of acinetobacter baumannii resistance patterns in patients with chronic obstructive pulmonary disease (COPD) in terms of choice of effective empiric antibiotic therapy. Ann Agric Environ Med. 2017;24(2):307–311. DOI:https://doi.org/10.26444/aaem/74710
- Hui DS, Ip M, Ling T, et al. A multicentre surveillance study on the characteristics, bacterial aetiologies and in vitro antibiotic susceptibilities in patients with acute exacerbations of chronic bronchitis. Respirology. 2011;16(3):532–539 DOI:https://doi.org/10.1111/j.1440-1843.2011.01943.x
- Kennedy M, Ramsheh MY, Williams CML, et al. Face mask sampling reveals antimicrobial resistance genes in exhaled aerosols from patients with chronic obstructive pulmonary disease and healthy volunteers. BMJ Open Resp Res. 2018;5(1):e000321. DOI:https://doi.org/10.1136/bmjresp-2018-000321
- Khilnani GC, Dubey D, Hadda V, et al. Predictors and microbiology of ventilator-associated pneumonia among patients with exacerbation of chronic obstructive pulmonary disease. Lung India. 2019;36(6):506–511. DOI:https://doi.org/10.4103/lungindia.lungindia_13_19
- Kuwal A, Joshi V, Dutt N, Singh S, Agarwal KC, Purohit G. A prospective study of bacteriological etiology in hospitalized acute exacerbation of COPD patients: Relationship with lung function and respiratory failure. Turk Toraks Dergisi/Turkish Thoracic J. 2018;19(1):19–27. DOI:https://doi.org/10.5152/TurkThoracJ.2017.17035
- Larsen MV, Janner JH, Nielsen SD, Friis-Møller A, Ringbaek T, Lange P. Bacteriology in acute exacerbation of chronic obstructive pulmonary disease in patients admitted to hospital. Scand J Infect Dis. 2009;41(1):26–32. DOI:https://doi.org/10.1080/00365540802484828
- Ma X, Cui J, Wang J, et al. Multicentre investigation of pathogenic bacteria and antibiotic resistance genes in Chinese patients with acute exacerbation of chronic obstructive pulmonary disease. J Int Med Res. 2015;43(5):699–710. DOI:https://doi.org/10.1177/0300060515587577
- Nian L, Liangyi X, Gang J, Wei L, Yongliang J. Analysis on constituents and drug resistance of pathogenic bacteria causing chronic obstructive pulmonary disease. Biomed Res (India). 2017;28(20):8939–8942.
- Perez-Trallero E, Marimon JM, Larruskain J, Alonso M, Ercibengoa M. Antimicrobial susceptibilities and serotypes of streptococcus pneumoniae isolates from elderly patients with pneumonia and acute exacerbation of chronic obstructive pulmonary disease. Antimicrob Agent Chemother. 2011;55(6):2729–2734. DOI:https://doi.org/10.1128/AAC.01546-10
- Rodrigo-Troyano A, Melo V, Marcos PJ, et al. Pseudomonas aeruginosa in chronic obstructive pulmonary disease patients with frequent hospitalized exacerbations: a prospective multicentre study. Respiration. 2018;96(5):417–424. DOI:https://doi.org/10.1159/000490190
- Sharma P, Narula S, Sharma K, Lohchab K, Kumar N. Sputum bacteriology and antibiotic sensitivity pattern in COPD exacerbation in india. Egypt J Chest Dis Tubercul. 66(4):593–597. DOI:https://doi.org/10.1016/j.ejcdt.2017.08.003
- Shoji H, Vazquez-Sanchez D, Gonzalez-Diaz A, et al. Overview of pneumococcal serotypes and genotypes causing diseases in patients with chronic obstructive pulmonary disease in a Spanish hospital between 2013 and 2016. Infect Drug Resist. 2018;11:1387–1400. DOI:https://doi.org/10.2147/IDR.S165093
- Shrestha R, Shrestha B, Shakya Shrestha S, Pant A, Prajapati B, Karmacharya BM. Study of pre-disposing factors of acute exacerbation of chronic obstructive pulmonary disease and antibiotic prescribing pattern with reference to antibiotic sensitivity test. Kathmandu Univ Med J. 13(51):259–264. DOI:https://doi.org/10.3126/kumj.v13i3.16817
- Srinivasan SV, Ahmed JS. Gram negative organisms as a cause of acute exacerbation of ‘COPD’ and assess the multi drug resistant in the gram-negative isolates. Int J Res Ayurveda Pharm. 2017;8(2):91–94. DOI:https://doi.org/10.7897/2277-4343.08272
- Vandevelde NM, Tulkens PM, et al. Characterisation of a collection of streptococcus pneumoniae isolates from patients suffering from acute exacerbations of chronic bronchitis: In vitro susceptibility to antibiotics and biofilm formation in relation to antibiotic efflux and serotypes/serogroups. Int J Antimicrob Agent. 2014;44(3):209–217. DOI:https://doi.org/10.1016/j.ijantimicag.2014.05.016
- Varon F, Torres-Caro C, Herrera-Diaz C, et al. Microbiological characterization of severe exacerbations in chronic obstructive pulmonary disease (COPD) in patients admitted to the ICU with or without associated pneumonia: A retrospective cross-sectional study. Infectio. 2019;23(4):307–312. DOI:https://doi.org/10.22354/in.v23i4.803
- Xu P, Song W, Liu Y, Pi J, Li Y, Yang H. Analysis of bacterial pathogens isolated from lower respiratory tract in patients with acute exacerbation of chronic obstructive pulmonary diseases [Chinese]. Chinese J Infect Chemother. 10(2):108–111.
- Ye F, He LX, Cai BQ, et al. Spectrum and antimicrobial resistance of common pathogenic bacteria isolated from patients with acute exacerbation of chronic obstructive pulmonary disease in mainland of china. Chin Med J. 2013;126(12):2207–2214.
- Zhao JN, Zhang XX, He XC, et al. Multidrug-resistant tuberculosis in patients with chronic obstructive pulmonary disease in china. PLoS One. 2015;10(8):e0135205. DOI:https://doi.org/10.1371/journal.pone.0135205