Abstract
There is a dearth of chemical inhibitors of connexin-mediated intercellular communication. The advent of short “designer” connexin mimetic peptides has provided new tools to inhibit connexin channels quickly and reversibly. This perspective describes the development of mimetic peptides, especially Gap 26 and 27 that are the most popular and correspond to specific sequences in the extracellular loops of connexins 37, 40 and 43. Initially they were used to inhibit gap-junctional coupling in a wide range of mammalian cells and tissues. Currently, they are also being examined as therapeutic agents that accelerate wound healing and in the early treatment of spinal cord injury. The mimetic peptides bind to connexin hemichannels, influencing channel properties as shown by lowering of electrical conductivity and potently blocking the entry of small reporter dyes and the release of ATP by cells. A mechanism is proposed to help explain the dual action of connexin mimetic peptides on connexin hemichannels and gap-junctional coupling.
Abbreviations | ||
Cx | = | connexin |
Hc | = | hemichannel |
CxHc | = | connexin hemichannel(s) |
Intercellular communication via gap junctions has proven a difficult function to manipulate especially with reagents that confine their primary action to binding to the cell's outer surface. Experimental manipulation of communication across gap junctions, despite its fundamental importance in tissue/organ homeostasis as well as its implication in several diseases (Fleishman et al., Citation2006), has been largely avoided, especially by pharmacologists possibly because of its directness and poor accessibility of the gap junction from the outside. Thus, there is a paucity of chemical tools available to execute a specific impairment of connexin-mediated communication. A short list of compounds used to inhibit experimentally gap-junctional communication includes halothane, octanol, 18α -glycyrrhetinic acid, carbenoxolone, and flufenamic acid (Evans and Boitano, Citation2001). The former two induce radical changes in membrane fluidity that impact on most if not all channels. The remainder generate intracellular effects that propagate into the cell's metabolic/signaling cascades. All display poor specificity towards connexin (Cx) channels and discerned effects of these reagents on gap-junctional communication are thus likely to be secondary and indirect. Many other compounds, all with limited or questionable specificity, have been used (Evans et al., Citation2006; Spray et al., Citation2002). Glucosaccharide probes are also advocated as reversible high-affinity inhibitors of Cx channels (Locke et al., Citation2003). Genetic ablation of several connexins using knock-out mice (Willecke et al., Citation2002) or by using connexin-specific antisense oligodeoxynucleotides are finding increasing application (Law et al., Citation2006). Other procedures, handicapped by limited general application, include low-pH media, especially in Xenopus oocytes, to close Cx channels (Hirst-Jensen et al., Citation2007).
The pharmacologist's prayer for tools that specifically target Cx channels by a rapid and easily reversible mode of action has been partially answered by the advent of short peptides that correspond to or “mimic” selected amino acid sequences in each of the two well conserved extracellular loops of connexins, the protein subunits of hemichannels and gap junctions. Recently, these “designer” peptides have been extended in range to include short peptides corresponding to amino acid regions in the highly variable intracellular loop domains (De Vuyst et al., Citation2006).
The Discovery and Exploitation of Cx-Mimetic Peptides
Following the genetic cloning of Cx32 and 43, it became possible to synthesize chemically to high-purity short peptides corresponding to specific sequences in the proteins. For example, several peptides corresponding to intracellular loop regions were used to generate antibodies to gap junctions. When microinjected, these anti-peptide antibodies proved to be useful tools to block gap-junctional communication during embryonic development (Becker et al., Citation1995) and in the intercellular propagation of Ca signaling waves (Boitano et al., Citation1998; Boitano and Evans, Citation2000) although the main purpose of development of anti-peptide antibodies was to generate immunological reagents for connexin/gap junction research by Western blotting, immunofluorescence and/or gold electron microscopy (Rahman and Evans, Citation1991; Zimmer et al., Citation1987). Subsequently, it was appreciated that these short peptides could become valuable tools to inhibit physiological processes dependent on gap-junctional communication overcoming the large size of immunoglobulins, which had been perceived as an experimental disadvantage. In one experimental model, a series of short peptides covering most of the two extracellular loops of Cx32, the range enhanced by synthesis of selected intracellular peptides, was used to determine their efficacy in inhibiting the achievement of synchronized contraction by aggregates of chick myocytes, a process requiring gap-junctional communication (Warner et al., Citation1995). This novel approach allowed identification of specific peptides that were most effective in blocking by external application gap-junctional coupling in a wide range of situations. Dahl et al. (Citation1994) used a similar synthetic peptide approach to inhibit cell–cell channel formation in paired Xenopus oocytes. The general applicability of mimetic peptides was advanced when it became clear that Cx43 was a widely expressed gap junction protein (Willecke et al., Citation2002) and this Cx rapidly became a primary candidate in the development of inhibitors. Consequently, two short peptides were designed called Gap26 and Gap27, corresponding to sequences in the first and second extracellular loops, respectively, of Cx43 (named as such because they were the 26th and 27th of a series of peptides synthesised for gap-junction projects by the peptide facility at the Medical Research Council (MRC) National Institute for Medical Research, London). Gap 26 and 27 have since been used extensively to evaluate and later confirm the importance of gap-junctional communication in smooth muscle–endothelial cooperation underpinning contraction in rabbit arteries (Chaytor et al., Citation1997, Citation1999; Figueroa et al., Citation2006; Griffith, Citation2004). The utility of Gap26 and Gap27, as well as other short peptides with sequences derived from other regions of the extracellular loop, also benefited from the early work described above () and have been extensively used to inhibit gap junctions constructed of Cx43 mainly, but also Cx32, 40 and 37 in a wide range of cells and tissues. The Cx-mimetic peptides have found wide application in studies of the immune system where Cx43 and Cx40 are expressed (Oviedo-Orta and Evans, Citation2002). Recent examples include studies showing Gap27 inhibiting intercellular communication and antigen cross presentation in dendritic cells (Matsue et al., Citation2006; Neijssen et al., Citation2005), T-cell activation (Bopp et al., Citation2007), and production of immunoglobulins and cytokines in mixed lymphocyte cultures (Oviedo-Orta et al., Citation2001). In the vascular system, mimetic peptides have found application as specific inhibitors of Cx-mediated communication (Isakson and Duling, Citation2005; Isakson et al., Citation2007; Martin et al., Citation2005; Mather et al., Citation2005), including studies in atherosclerosis (Wong et al., Citation2006), cardiac protection (Przyklenk et al., Citation2005) and reperfusion injury following ischemia (Shintani-Ishida et al., Citation2007). The peptides have also been applied as inhibitory tools of cell communication in studies of osteoclastic differentiation (Ilvesaro et al., Citation2001) in epidermis (Kandyba et al., Citation2007) and in dissecting the effects of laminin on Ca signaling in tracheal epithelial cells (Isakson et al., Citation2006) and lipopolysaccharide (LPS) as well as fibroblast growth factor on gap junctions relative to connexin hemichannels (CxHc) in HeLa and glioma cells expressing Cx43 (De Vuyst et al., Citation2007). Other Cx-mimetic peptides mimicking extracellular loop sequences have also found use as inhibitors in dendritic cells (Mendoza-Naranjo et al., Citation2007), hepatocytes (Eugenin et al., Citation1998) and aortic smooth muscle of cell coupling cells (Kwak and Jongsma, Citation1999).
Table 1 Cx-mimetic peptides: structures and properties
Gap 26 and 27 cover highly conserved ectodomain consensus sequences and have been used as “pan” reagents to inhibit connexin-facilitated communication, especially where a number of connexins are expressed (e.g., skin and brain). A derivative of Gap 26 specific to Cx37 and Cx40 has proved especially useful in studies of vascular systems (Chaytor et al., Citation1999) indicating that the peptides are tools that are likely to interact at a common Cx ectodomain communication locus. The peptides have been used thus far mainly with rodent and human cells, but they are effective in other species reflecting the high conservation of amino and sequence in the two extracellular loops. The mimetic peptides do not block junctional communication completely in all instances but they have the advantage of being non-toxic to cells, in contrast to 18α -glycyrrhetinic acid that is highly toxic, for example, to lymphocyte T-cells.
Cx-Mimetic Peptides as Inhibitors of Hemichannels
Gap junctions and CxHc are Cx dodecameric/hexameric oligomers respectively exhibiting different structural and functional features (Evans et al., Citation2006; Goodenough and Paul, Citation2003; Saez et al., Citation2005). A prime difference between the channels is that gap junctions allow cells to communicate with each other whereas CxHc permit cells to “sense” the immediate environment. CxHc are biogenetic precursors of gap-junction channels, a process occurring when those present in closely aligned cells dock head-to-head to form the basic “communication units” that accrete into gap-junction plaques; these can be formed out of up to 200,000 units.
CxHc, when in an open configuration, compromise plasma membrane permeability. This is observed especially under conditions regarded as stressful to cells. Studies have shown that cells release ATP, prostaglandins, glutamate, and glutathione via CxHc (Evans et al., Citation2006). ATP release across CxHc has been studied extensively since its release initiates paracrine purinergic intercellular signaling pathways involving P2X and P2Y receptors on adjacent cells (Burnstock, Citation2006). Although gap junctions and CxHc allow molecules/ions up to 1000 Da to pass across, they have been shown to exhibit different gating characteristics with gap junctions closing at 500–2000 nM and CxHc opening at 500 nM (DeVuyst et al., Citation2006). Thus, intracellular Ca levels that open CxHc close gap junctions, although allowances should be made for local variations in Ca levels observed beneath various specialized domains comprising the plasma membrane.
CxHc were first shown to open at low extracellular Ca levels (Ebihara, Citation2003). However, it is now evident that channel gating is also susceptible to other environmental contingencies, including metabolic inhibition (Contreras et al., Citation2003; Contreras et al., Citation2002; Contreras et al., Citation2004), osmotic changes (Quist et al., Citation2000), and oxidative (Ramachandran et al., Citation2007; Retamal et al., Citation2007) and ischemic stress (Shintani-Ishida et al., Citation2007).
Although most studies on CxHc function have been carried out using cultured cells, in vivo studies using folliculogenesis as a model have recently emphasized that they function independently of intercellular gap junctions (Tong et al., Citation2007). It has been shown that Gap 26 and 27 attenuated the release of ATP by an endothelial cell line (Braet et al., Citation2003a, Citation2003b), thereby pointing a finger at a contribution by CxHc to its release. Similar inhibition of ATP release by Cx mimetics has been demonstrated in a range of mammalian cells, including neural cells (Pearson et al., Citation2005), taste cells (Romanov et al., Citation2007), corneal epithelial cells (D'Hondt et al., Citation2007; Gomes et al., Citation2006), and neutrophils (Eltzschig et al., Citation2006). It soon became evident that attenuation of CxHc permeability by Gap26 may occur independently of gap-junctional coupling, providing, for example, an alternative mechanism explaining the roles of Cx in ischemia and reperfusion injury in the heart and brain (Contreras et al., Citation2002; Shintani-Ishida et al., Citation2007); unpublished work by Clarke, Williams, Martin, and Evans (2007). In many studies specificity in the context of the precise amino acid sequence mimicked was controlled either by using “scrambled” peptides of the same length and amino acid composition or with similar length peptides often corresponding to intracellular Cx sequences.
How Do Cx-Mimetic Peptides Inhibit Cell Communication?
Since Cx-mimetic peptides were first shown to inhibit gap-junctional communication, proposed mechanisms focused on their direct action in interference with the docking of CxHc to form gap junctions. (Berthoud et al., Citation2000) and argued that higher affinity of the peptide for its counterpart sequence on the extracellular loop region would compete with and thus impede the docking of two CxHc causing inhibition of gap junction coupling. Although gap junction plaques, once formed, are internalized by cells as complete units, there may also be interference by bound peptide to a steady-state balance between docking and undocking, especially at the peripheral regions of the plaques, leading to a lowering of “communication capacity” and/or the number of gap junctions. Arguing against such a mechanism is the demonstration by Berman et al. (Citation2002) using HeLa cells expressing Cx43-GFP that despite Gap 27 and Gap26 inhibiting Lucifer yellow dye transfer, there was no diminution in the overall number of autofluorescent gap-junctional plaques during the treatment, suggesting that the peptides had minimal effect on gap junction biogenesis and turnover. On the other hand, an overcapacity in overall communication through gap junctions in tissues (e.g., heart and liver) and questions arising concerning whether all channels compressed into plaques are in an open configuration, indicate that caution should be exercised in reaching definitive conclusions.
The inhibition of ATP release by Cx-mimetic peptides has prompted a major re-examination of closure mechanisms featuring their interaction with CxHc. Two complementary lines of evidence show that there is direct action by Gap 26 on CxHc, resulting in lowering of their conductivity. A substantial lowering of outward currents in hemichannels likely to be constructed of Cx occurred after addition of Gap26 to taste cells (Romanov et al., Citation2007). Also, a five-fold lowering of conductivity within around 10 min of application of Gap 26 to HeLa cells expressing Cx43 with cell coupling being impeded at later time has been recorded (unpublished work by Desplantez, Verma, Evans and Weingard). It is also a general observation that Gap 26 was more effective than Gap 27 when applied at the same concentration. Furthermore, atomic force microscopy of the extracellular loop region of Cx43-Hc demonstrated direct binding by Gap 26 (Liu et al., Citation2006).
shows a possible mechanism of action of Cx-mimetic peptides. It takes into account the early inhibition of connexin hemichannels followed by later closure of gap junctions as the hemichannels with bound peptide dock and accrete into gap junctions. The half-life of Cx43 is around two to three hours but half-lives of other connexins may vary (Laird, Citation2006). Therefore, the faster the turnover, the more rapid the blocking effects of the connexin mimetics will be on gap junctions, thereby explaining different kinetics of inhibition of gap junctions in various cell types. The model also indicates that short peptides are likely to permeate into intercellular spaces surrounding gap junctions where they also bind to Cx extracellular loops, inducing direct closure of gap junctions. Since Cx-mimetic peptide interaction with connexin hemichannels disturbs intracellular Ca2 + oscillations that underpin cell signaling, they may also have indirect effects on gap-junctional coupling. Further clarification of how the Cx-mimetic peptides work will be aided by higher resolution models of gap junctions and connexin hemichannels (Sosinsky and Nicholson, Citation2005). Also, the precise nature of the peptide–protein interaction needs exploration by, for example, yeast hybrid screening using the mimetic peptides as bait, which will help to fill gaps in knowledge of the presumed targets.
Figure 1 A proposed mechanism of action by which Cx-mimetic peptides inhibit CxHc and gap junctions. Hemichannels in unapposed regions of the cell's plasma membrane open and release ATP. Peptides bind to channel external domains causing closure, thus limiting ATP release. CxHc: peptide complexes move laterally in the plasma membrane, dock and accrete into gap junctions that become closed thus impairing intercellular coupling/communication. Peptides may also diffuse into intercellular spaces, bind to CxHc and gap junctions also inducing their closure. Connexin channel gating may also be regulated indirectly by changes in sub-plasma membrane Ca levels caused by binding of the peptides to CxHc and influencing, for example, Ca entry into cells. Reproduced with permission of the Biochemical Journal.
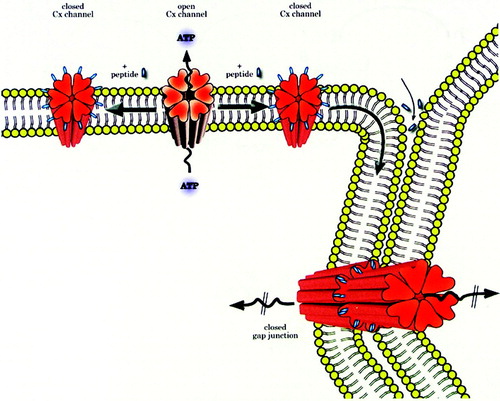
Questions Regarding Specificity of Action
Given the physiological and pharmacological profiles of Gap26 and Gap27, additional studies will be required to further refine their mechanism of action at the peptide–protein level using techniques such as nuclear magnetic resonance (NMR) and others. Modifications to improve their efficacy and, especially when used in a whole animal setting, are needed to decelerate breakdown by proteases. The use of peptides synthesized using D–amino acids is one possibility and could add further support for their action as strict “mimetics”, as well as allowing specificity to be investigated further. Nevertheless, the literature now reviewed shows that mimetic peptides have become an important addition to the range of tools available to inhibit Cx-dependent cell communication. Doubtless, their higher specificity compared to other inhibitors is enhanced by confinement of their action (at least in the short term) to action at the plasma membrane's exterior. Hemichannels are often described as large-diameter anion channels, and it is becoming clear that other channels exist in the plasma membrane and that effects of binding to one channel type may be transmitted to others. For example, a large anion channel permeable to ATP has been described (Dutta et al., Citation2004) adding to the several mechanisms by which ATP “escapes” from cells. Recently, interest has been aroused concerning the roles of pannexin hemichannels in cell communication. Pannexins, of which three subtypes have been described, are unrelated to connexins, and their oligomerization is restricted to forming hemichannels in the plasma membrane. In other words, they oligomerize to form hemichannels but they are less likely to form gap junctions. Interestingly, they allow ATP release by cells but their calcium sensitivity is different from that of connexins (Locovei et al., Citation2006). Evidence has been presented claiming that Gap 26 and 27 interact with pannexin hemichannels, inducing closure, and that their action on connexin hemichannels is mainly steric and not of a strictly “mimetic” nature (Wang et al., Citation2007). These studies, carried out in Xenopus oocytes, await corroboration and support from other model systems, especially mammalian cells expressing Cx43. For example, Gap 27 did not inhibit pannexin1 channels in mouse or human macrophages (Pelegrin and Surprenant, Citation2006).
Peptides as Therapeutic Tools
Changes in connexin channel operation evidenced by modified protein expression levels or genetic mutations have prompted the adoption of therapeutic approaches that exploit peptides that can regulate Cx-dependent intercellular communication. Rotigaptide, a short hexapeptide containing D–amino acids, and modified from its parent analogue, AAP10, is currently in Phase II clinical trails as a drug to reduce atrial fibrillation. Rotigaptide augmented gap-junctional conductance and improved cell coupling (Shiroshita-Takeshita et al., Citation2007). Rotigaptide also increased coupling in cells expressing Cx43, but not those expressing Cx32 and Cx26 (Clarke et al., Citation2006).
Studies with Cx-mimetic peptides to determine their therapeutic value are at a more preliminary stage, but evidence points to value in accelerating wound healing when applied topically in gels. Recently, O'Carroll et al (in press) demonstrated that Cx-mimetic peptides, including Gap27, may be useful in reducing damage after spinal cord injury. Such observations raise further exciting possibilities for the use of Cx-mimetic peptides in the treatment of ischaemia in heart and brain (Evans et al., Citation2006).
REFERENCES
- Becker D L, Evans W H, Green C R, Warner A. Functional analysis of amino-acid-sequences in connexin43 involved in intercellular communication through gap-junctions. J Cell Sci 1995; 108: 1455–1467
- Berman R S, Martin P EM, Evans W H, Griffith T M. Relative contributions of NO and gap-junctional communication to endothelium-dependent relaxations of rabbit resistance arteries vary with vessel size. Microvasc Res 2002; 63: 115–128
- Berthoud V M, Beyer E C, Seul K H. Peptide inhibitors of intercellular communication. Am J Physiol Lung Cell Mol Physiol 2000; 279: L619–L622
- Boitano S, Dirksen E R, Evans W H. Sequence specific antibodies to connexins block intercellular calcium signaling through gap junctions. Cell Calcium 1998; 23: 1–9
- Boitano S, Evans W H. Connexin mimetic peptides reversibly inhibit Ca2 + signaling through gap junctions in airway cells. Am J Physiol Lung Cell Mol Physiol 2000; 279: L623–L630
- Bopp T, Becker C, Klein M, Klein-Hessling S, Palmetshofer A, Serfling E, Heib V, Becker M, Kubach J, Schmitt S, et al. Cyclic adenosine monophosphate is a key component of regulatory T cell-mediated suppression. J Exp Med 2007; 204: 1303–1310
- Braet K, Aspeslagh S, Vandamme W, Willecke K, Martin P EM, Evans W H, Leybaert L. Pharmacological sensitivity of ATP release triggered by photoliberation of inositol-1,4,5-trisphosphate and zero extracellular calcium in brain endothelial cells. J Cell Physiol 2003b; 197: 205–213
- Braet K, Vandamme W, Martin P EM, Evans W H, Leybaert L. Photoliberating inositol-1,4,5-trisphosphate triggers ATP release that is blocked by the connexin mimetic peptide gap 26. Cell Calcium 2003a; 33: 37–48
- Burnstock G. Historical review: ATP as a neurotransmitter. Trends Pharmacol Sci 2006; 27: 166–176
- Chaytor A T, Evans W H, Griffith T M. Peptides homologous to extracellular loop motifs of connexin 43 reversibly abolish rhythmic contractile activity in rabbit arteries. J Physiol (London) 1997; 503: 99–110
- Chaytor A T, Martin P EM, Evans W H, Randall M D, Griffith T M. The endothelial component of cannabinoid-induced relaxation in rabbit mesenteric artery depends on gap-junctional communication. J Physiol (London) 1999; 520: 539–550
- Clarke T C, Thomas D, Petersen J S, Evans W H, Martin P EM. The antiarrhythmic peptide rotigaptide (ZP123) increases gap junction intercellular communication in cardiac myocytes and HeLa cells expressing connexin 43. Br J Pharmacol 2006; 147: 486–495
- Contreras J E, Saez J C, Bukauskas F F, Bennett M VL. Gating and regulation of connexin 43 (U43) hemichannels. Proc Natl Acad Sci U S A 2003; 100: 11388–11393
- Contreras J E, Sanchez H A, Eugenin E A, Speidel D, Theis M, Willecke K, Bukauskas F F, Bennett M VL, Saez J C. Metabolic inhibition induces opening of unapposed connexin 43 gap junction hemichannels and reduces gap-junctional communication in cortical astrocytes in culture. Proc Natl Acad Sci USA 2002; 99: 495–500
- Contreras J E, Sanchez H A, Veliz L P, Bukauskas F F, Bennett M VL, Saez J C. Role of connexin-based gap junction channels and hemichannels in ischemia-induced cell death in nervous tissue. Brain Res Rev 2004; 47: 290–303
- D'Hondt C, Ponsaerts R, Srinivas S P, Vereecke J, Himpens B. Thrombin inhibits intercellular calcium wave propagation in corneal endothelial cells by modulation of hemichannels and gap junctions. Invest Ophthalmol Vis Sci 2007; 48: 120–133
- Dahl G, Nonner W, Werner R. Attempts to define functional domains of gap junction proteins with synthetic peptides. Biophys J 1994; 67: 1816–1822
- De Vuyst E, Decrock E, Cabooter L, Dubyak G R, Naus C C, Evans W H, Leybaert L. Intracellular calcium changes trigger connexin 32 hemichannel opening. EMBO J 2006; 25: 34–44
- De Vuyst E, Decrock E, De Bock M, Yamasaki H, Naus C C, Evans W H, Leybaert L. Connexin hemichannels and gap junction channels are differentially influenced by lipopolysaccharide and basic fibroblast growth factor. Mol Biol Cell 2007; 18: 34–46
- Dutta A K, Sabirov R Z, Uramoto H, Okada Y. Role of ATP-conductive anion channel in ATP release from neonatal rat cardiomyocytes in ischaemic or hypoxic conditions. J Physiol (London) 2004; 559: 799–812
- Ebihara L. New roles for connexons. News Physiol Sci 2003; 18: 100–103
- Eltzschig H K, Eckle T, Mager A, Kuper N, Karcher C, Weissmuller T, Boengler K, Schulz R, Robson S C, Colgan S P. ATP release from activated neutrophils occurs via connexin 43 and modulates adenosine-dependent endothelial cell function. Circ Res 2006; 99: 1100–1108
- Eugenin E A, Gonzalez H, Saez C G, Saez J C. Gap-junctional communication coordinates vasopressin-induced glycogenolysis in rat hepatocytes. Am J Physiol 1998; 274: G1109–G1116
- Evans W H, Boitano S. Connexin mimetic peptides: specific inhibitors of gap-junctional intercellular communication. Biochem Soc Trans 2001; 29: 606–612
- Evans W H, De Vuyst E, Leybaert L. The gap junction cellular internet: connexin hemichannels enter the signalling limelight. Biochem J 2006; 397: 1–14
- Figueroa X F, Isakson B E, Duling B R. Vascular gap junctions in hypertension. Hypertension 2006; 48: 804–811
- Fleishman S J, Sabag A D, Ophir E, Avraham K B, Ben-Tal N. The structural context of disease-causing mutations in gap junctions. J Biol Chem 2006; 281: 28958–28963
- Gomes P, Srinivas S P, Vereecke J, Himpens B. Gap-junctional intercellular communication in bovine corneal endothelial cells. Exp Eye Res 2006; 83: 1225–1237
- Goodenough D A, Paul D L. Beyond the gap: Functions of unpaired connexon channels. Nat Rev Mol Cell Biol 2003; 4: 285–294
- Griffith T M. Endothelium-dependent smooth muscle hyperpolarization: do gap junctions provide a unifying hypothesis?. Br J Pharmacol 2004; 141: 881–903
- Hirst-Jensen B J, Sahoo P, Kieken F, Delmar M, Sorgen P L. Characterization of the pH-dependent interaction between the gap junction protein connexin43 carboxyl terminus and cytoplasmic loop domains. J Biol Chem 2007; 282: 5801–5813
- Ilvesaro J, Tavi P, Tuukkanen J. Connexin-mimetic peptide Gap 27 decreases osteoclastic activity. BMC Musculoskelet Disord 2001; 2: 10
- Isakson B E, Duling B R. Heterocellular contact at the myoendothelial junction influences gap junction organization. Circ Res 2005; 97: 44–51
- Isakson B E, Olsen C E, Boitano S. Laminin-332 alters connexin profile, dye coupling and intercellular Ca2 + waves in ciliated tracheal epithelial cells. Respir Res 2006; 7: 105
- Isakson B E, Ramos S I, Duling B R. Ca2 + and inositol 1,4,5-trisphosphate-mediated signaling across the myoendothelial junction. Circ Res 2007; 100: 246–254
- Kandyba E E, Hodgins M B, Martin P E. A murine living skin equivalent amenable to live-cell imaging: analysis of the roles of connexins in the epidermis. J Invest Dermatol 2007
- Kwak B R, Jongsma H J. Selective inhibition of gap junction channel activity by synthetic peptides. J Physiol 1999; 516: 679–685, (Pt 3)
- Laird D W. Life cycle of connexins in health and disease. Biochem J 2006; 394: 527–543
- Law L Y, Zhang W V, Stott N S, Becker D L, Green C R. In vitro optimization of antisense oligodeoxynucleotide design: an example using the connexin gene family. J Biomol Tech 2006; 17: 270–282
- Liu F, Arce F T, Ramachandran S, Lal R. Nanomechanics of hemichannel conformations: connexin flexibility underlying channel opening and closing. J Biol Chem 2006; 281: 23207–23217
- Locke D, Wang L X, Bevans C G, Lee Y C, Harris A L. Open pore block of connexin26 and connexin32 hemichannels by neutral, acidic and basic glycoconjugates. Cell Commun Adhes 2003; 10: 239–244
- Locovei S, Wang J J, Dahl G. Activation of pannexin 1 channels by ATP through P2Y receptors and by cytoplasmic calcium. FEBS Lett 2006; 580: 239–244
- Martin P EM, Wall C, Griffith T M. Effects of connexin-mimetic peptides on gap junction functionality and connexin expression in cultured vascular cells. Br J Pharmacol 2005; 144: 617–627
- Mather S, Dora K A, Sandow S L, Winter P, Garland C J. Rapid endothelial cell-selective loading of connexin 40 antibody blocks endothelium-derived hyperpolarizing factor dilation in rat small mesenteric arteries. Circ Res 2005; 97: 399–407
- Matsue H, Yao J, Matsue K, Nagasaka A, Sugiyama H, Aoki R, Kitamura M, Shimada S. Gap junction-mediated intercellular communication between dendritic cells (DCs) is required for effective activation of DCs. J Immunol 2006; 176: 181–190
- Mendoza-Naranjo A, Saez P J, Johansson C C, Ramirez M, Mandakovic D, Pereda C, Lopez M N, Kiessling R, Saez J C, Salazar-Onfray F. Functional gap junctions facilitate melanoma antigen transfer and cross-presentation between human dendritic cells. J Immunol 2007; 178: 6949–6957
- Neijssen J, Herberts C, Drijfhout J W, Reits E, Janssen L, Neefjes J. Cross-presentation by intercellular peptide transfer through gap junctions. Nature 2005; 434: 83–88
- Oviedo-Orta E, Evans W H. Gap junctions and connexins: potential contributors to the immunological synapse. J Leukoc Biol 2002; 72: 636–642
- Oviedo-Orta E, Gasque P, Evans W H. Immunoglobulin and cytokine expression in mixed lymphocyte cultures is reduced by disruption of gap junction intercellular communication. FASEB J 2001; 15: 768–774
- Pearson R A, Dale N, Llaudet E, Mobbs P. ATP released via gap junction hemichannels from the pigment epithelium regulates neural retinal progenitor proliferation. Neuron 2005; 46: 731–744
- Pelegrin P, Surprenant A. Pannexin-1 mediates large pore formation and interleukin 1beta release by the ATP-gated P2X7 receptor. EMBO Journal 2006; 25: 5071–5082
- Przyklenk K, Maynard M, Darling C E, Whittaker P. Pretreatment with D-myo-inositol trisphosphate reduces infarct size in rabbit hearts: Role of inositol trisphosphate receptors and gap junctions in triggering protection. J Pharmacol Exp Ther 2005; 314: 1386–1392
- Quist A P, Rhee S K, Lin H, Lal R. Physiological role of gap-junctional hemichannels: extracellular calcium-dependent isosmotic volume regulation. J Cell Biol 2000; 148: 1063–1074
- Rahman S, Evans W H. Topography of connexin32 in rat-liver gap-junctions. Evidence for an intramolecular disulfide linkage connecting the 2 extracellular peptide loops. J Cell Sci 1991; 100: 567–578
- Ramachandran S, Xie L H, John S A, Subramaniam S, Lal R. A novel role for connexin hemichannel in oxidative stress and smoking-induced cell injury. PLoS ONE 2007; 2: e712
- Retamal M A, Schalper K A, Shoji K F, Bennett M VL, Saez J C. Opening of connexin 43 hemichannels is increased by lowering intracellular redox potential. Proc Natl Acad Sci U S A 2007; 104: 8322–8327
- Romanov R A, Rogachevskaja O A, Bystrova M F, Jiang P H, Margolskee R F, Kolesnikov S S. Afferent neurotransmission mediated by hemichannels in mammalian taste cells. EMBO J 2007; 26: 657–667
- Saez J C, Retamal M A, Basilio D, Bukauskas F F, Bennett M VL. Connexin-based gap junction hemichannels: Gating mechanisms. Biochim Biophys Acta 2005; 1711: 215–224
- Seki A, Duffy H S, Coombs W, Spray D C, Taffet S M, Delmar M. Modifications in the biophysical properties of connexin43 channels by a peptide of the cytoplasmic loop region. Circ Res 2004; 95: e22–e28
- Shintani-Ishida K, Uemura K, Yoshida K. Hemichannels in cardiomyocytes open transiently during ischemia and contribute to reperfusion injury following brief ischemia. Am J Physiol Heart Circ Physiol 2007; 293: H1714–H1720
- Shiroshita-Takeshita A, Sakabe M, Haugan K, Hennan J K, Nattel S. Model-dependent effects of the gap junction conduction-enhancing antiarrhythmic peptide rotigaptide (ZP123) on experimental atrial fibrillation in dogs. Circulation 2007; 115: 310–318
- Sosinsky G E, Nicholson B J. Structural organization of gap junction channels. Biochim Biophys Acta 2005; 1711: 99–125
- Spray D C, Rozental R, Srinivas M. Prospects for rational development of pharmacological gap junction channel blockers. Curr Drug Targets 2002; 3: 455–464
- Tong D, Li T Y, Naus K E, Bai D, Kidder G M. In vivo analysis of undocked connexin43 gap junction hemichannels in ovarian granulosa cells. J Cell Sci 2007; 120: 4016–4024
- Wang J, Ma M, Locovei S, Keane R W, Dahl G. Modulation of membrane channel currents by gap junction protein mimetic peptides: size matters. Am J Physiol Cell Physiol 2007; 293: C1112–C1119
- Warner A, Clements D K, Parikh S, Evans W H, De Haan R L. Specific motifs in the external loops of connexin proteins can determine gap junction formation between chick heart myocytes. J Physiol 1995; 488: 721–728, (Pt 3)
- Willecke K, Eiberger J, Degen J, Eckardt D, Romualdi A, Guldenagel M, Deutsch U, Sohl G. Structural and functional diversity of connexin genes in the mouse and human genome. Biol Chem 2002; 383: 725–737
- Wong C W, Christen T, Roth I, Chadjichristos C E, Derouette J P, Foglia B F, Chanson M, Goodenough D A, Kwak B R. Connexin37 protects against atherosclerosis by regulating monocyte adhesion. Nat Med 2006; 12: 950–954
- Zimmer D B, Green C R, Evans W H, Gilula N B. Topological analysis of the major protein in isolated intact rat liver gap junctions and gap junction-derived single membrane structures. J Biol Chem 1987; 262: 7751–7763