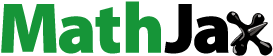
ABSTRACT
In this study, we successfully polymerized natural phenol (gallic acid, ferulic acid) and pyrrole (Py) monomers by oxidative polymerization of horseradish peroxidase (HRP). Then, we successfully colored silk fabrics using these chemicals. We analyzed the polymerization mechanism of polyphenol and polypyrrole complexes in detail using UV-Vis spectroscopy, Fourier transform infrared spectroscopy (FT-IR), and high-performance liquid chromatography/electrospray ionization mass spectrometry (HPLC/ESI-MS). In addition, we investigated the electrochemical activity, thermal properties and anti-UV activity of the colored silk fabrics. We found that the oxidative polymerization of phenol and Py on silk fibers under mild reaction conditions using HRP produced technically conductive silk fabrics that combine the superior chemical and physical properties of silk fabrics with superior levels of electrochemical activity and thermal stability. Upon analyzing the color depth of the fabrics, we found that silk fabrics treated with Py have a darker color than silk fabrics treated in the absence of Py. Additionally, the fabrics had slightly different levels of color fastness, but all were within the acceptable range. This technique expands the range of applications of natural phenols for textile dyeing and functionalization as well as providing new prospects for the enzymatically environmentally friendly production of natural functional fabric.
摘要
在本研究中,我们通过辣根过氧化物酶(HRP)的氧化聚合成功地聚合了天然苯酚(没食子酸、阿魏酸)和吡咯(Py)单体. 然后,我们成功地用这些化学物质给丝绸染色. 我们使用紫外可见光谱、傅立叶变换红外光谱(FT-IR)和高效液相色谱/电喷雾电离质谱(HPLC/ESI-MS)详细分析了多酚和聚吡咯复合物的聚合机理. 此外,我们还研究了彩色真丝织物的电化学活性、热性能和抗紫外线活性. 我们发现,在温和的反应条件下,使用HRP在丝绸纤维上氧化聚合苯酚和吡咯烷酮,生产出技术上导电的丝绸织物,该织物结合了丝绸织物的优异化学和物理性能以及优异的电化学活性和热稳定性. 通过分析织物的颜色深度,我们发现,经PY处理的真丝织物比未经PY的真丝面料颜色更深. 此外,这些织物的色牢度水平略有不同,但都在可接受的范围内. 该技术扩大了天然酚在纺织品染色和功能化中的应用范围,并为天然功能织物的酶促环保生产提供了新的前景.
Introduction
Conventional fibers have no or low conductivity. Therefore, conductive fibers are produced by polymerizing conductive fillers into the fiber structure (either during melting or electrostatic spinning, or through metallic coatings of the conductive polymers, etc.). Polypyrrole (PPy) is one of the most promising conductive polymers for coating textile materials due to its high conductivity, easy synthesis, and environmental stability (Cetiner Citation2014). Therefore, polypyrrole (PPy) is used as a textile coating agent for sensors (Mabrook, Pearson, and Petty Citation2006), actuators (Ramanavicius, Ramanaviciene, and Malinauskas Citation2006), batteries (Jager, Smela, and Inganaes Citation2000), anti-electrostatic coatings (Nystrom et al. Citation2009), and various biomedical devices (Geetha and Trivedi Citation2004). In addition, conductive PPy can be readily polymerized using chemical or electrochemical methods, including in situ polymerization, two-step polymerization, emulsion polymerization, and vapor phase polymerization (Bai et al. Citation2016).
Silk is a natural fibrous polymer produced by silk worm. It is one of the most extensively studied biomaterial because of its good biodegradability, biocompatibility, and oxygen permeability in the wet state (Malhotra, Maity, and Chatterhee Citation2014). Conductive silk could be used in new electronic devices, such as wearable electronics, and it has a wide range of possibilities, ranging from medical or aerospace applications. Due to these demands, natural silk fibers have been applied to the polymerization of pyrrole monomers for the production of conductive fabrics using diverse polymerization methods. Wang et al. (Citation2020) developed a facile method to form a uniform graphene oxide (GO)– polypyrrole (PPy) double coating on porous silk fibroin (SF) scaffold (SF@GO-PPY); this was used to produce scaffolds with the desired electrical conductivity in the nerve tissue engineering field. Zhao et al. (Citation2018) reported the fabrication of PPy-coated SF (PPy/SF) conductive composite scaffolds using 3D bioprinting and electrospinning. They also found that combining 3D bioprinting with electrospinning helped optimize the structure of the composite scaffold to enhance nerve regeneration. In a recent study, Cucchi et al. (Citation2009) reported coating silk fabrics with electrically conductive doped PPy by in situ oxidative polymerization from an aqueous solution of pyrrole (Py) at room temperature using FeCl3 as a catalyst. Polymerization occurred on the fiber surface rather than the bulk; the intrinsic crystal and molecular structure of silk was not affected by polymerization.
Enzymatic polymerization has been regarded as an environmentally benign synthesis process, which is generally carried out under relatively mild pH and temperature conditions. This is an attractive method because it has good chemo-, enantio- and regioselectivity (Baek et al. Citation2021). Horseradish peroxidase (HRP, EC1.11.1.7), a plant peroxidase extracted from horseradish root, has a well-known structure and catalytic mechanism (Baek et al. Citation2020). Zhou et al. (Citation2013) demonstrated that HRP/H2O2 incubation is an efficient approach to polymerization of Lignosulfonates (LSs) in an aqueous solution at room temperature. The polymerization was mainly due to the coupling of phenoxyl radicals. In (Liu et al. Citation2015), HRP was used to catalyze the free-radical copolymerization of acrylamide (AM) and lignin on the surface of jute fibers, thus grafting AM onto the jute fiber surface and improving the moisture absorption, wettability, etc. Like this, HRP readily bonds with hydrogen peroxide. The resulting HRP-H2O2 complex can catalyze various hydrogen donors and primary amines.
Bai et al. (Citation2016) reported that PPy was prepared by laccase-mediated in situ polymerization. Additionally, they simultaneously obtained PPy-colored wool fabric with conductive function. In their study, they created two different colors (green and black) through adjusting the dyeing conditions. Conductive fibers are limited in color because black materials such as carbon or graphite are used as conductive materials. Polypyrrole-coated fabrics are also typically black (Boschi et al. Citation2008). In our previous study (Baek et al. Citation2020, Citation2021), we succeeded in polymerizing and coloring various natural phenols on silk fabrics using HRP catalysts. The resulting fabric has high fastness and functionality. It has also been shown that some color adjustment is possible depending on the dyeing conditions. In particular, ferulic acid and gallic acid, which are natural phenolic substances, can dye vivid yellow and brown colors, respectively, using only a small amount.
Although many studies have successfully coated silk fabrics with PPy to obtain conductive silk fabrics, but no studies have been conducted on diversifying the color of the silk fabrics obtained. The demand for conductive fabrics is high and their uses diverse. Therefore, it is necessary to develop naturally colored silk fabrics with conductivity. As mentioned earlier, enzyme-catalyzed polymerization is an eco-friendly oxidative polymerization mechanism that can polymerize the hydroxyl groups of natural phenolic substances and the amine groups of pyrrole monomers. Therefore, the purpose of this experiment is to develop naturally colored conductive silk fabrics through oxidatively polymerizing natural phenol (gallic acid, ferulic acid) and PPy using HRP (Scheme 1).
Materials and methods
Reagents and materials
Horseradish peroxidase (HRP, EC1.11.1.7, enzymatic activity ≥200 U/mg) was obtained from J&K Chemical Co., Ltd. (Shanghai, China) and stored at − 20°C prior to use. Silk fabric (density of 68 g/m2) was provided by Wu-Jiang HMT SILK Textile Co., Ltd. Pyrrole (purity 99.5%) was supplied by Aladdin Reagent Ltd. (Shanghai, China) and stored at 4°C in the dark before use. Gallic acid (3,4,5-Trihydroxybenzoic acid monohydrate) were purchased from Sinopharm Chemical Reagent Co., Ltd. (Shanghai, China). Ferulic acid was obtained from Innochemm Science & Technology Co., Ltd. (Beijing, China). All other reagents were of analytical grade or higher.
Enzymatic oxidative polymerization of phenol and pyrrole
Natural phenol (GA or FA, 6 mM), Pyrrole (4 mM) and HRP (6 U/mL) were dissolved in 50 mL of phosphate buffer (0.1 M, pH 7.0) in a reaction vessel. The incubation process was started by adding H2O2 (10 mM). The reaction was kept at 50°C in a shaker water bath for 2 h. At the same time, the solution was stirred at a speed of 65 rpm (revolutions per minute). Then the solution was centrifuged off and collected via freeze-drying at −20°C.
Dyeing process of silk fabrics
In the case of HRP-catalyzed dyeing of silk fabric, Phenol (6 mM), Pyrrole (4 mM) and HRP (6 U/ml) were prepared in 0.1 M phosphate buffer (pH 7.0). The incubation process was started by adding H2O2 (10 mM). A liquor-to-goods ratio was 100:1 and treated at 50°C for 3 h. At the same time, the solution was stirred at a speed of 65 rpm.
After treatment, the colored silk fabrics were thoroughly washed several times with deionized water in order to eliminate any residual HRP or non-reacted monomer, as well as any unfixed polymer. Then the silk fabric samples were dried in an oven at 40°C.
Characterization of polymers
UV – Vis spectra analysis
The UV – vis spectra of natural phenol polymer (PGA, PFA) and phenol polymer/polypyrrole copolymer (PGA/PPy, PFA/PPy) samples were prepared in distilled water (1:200 v/v) and analyzed from 200 to 700 nm using a UV −1800 spectrophotometer (Shimadzu Corporation, Japan).
FTIR spectroscopy
The spectra of the compounds (PGA, PFA, PGA/PPy, PFA/PPy) were obtained using a Nicolet iS10 FT-IR spectrophotometer (Thermo Fisher Scientific, USA). Background scanning spectra were performed using KBr powder prior to data collection. HRP-treated four copolymers were mixed with a small amount of KBr powder. A minimum of 48 scans were examined to obtain an appropriate signal-to-noise ratio. The Spectra were obtained in the region 450–4000 cm−1 at room temperature with a resolution of 8 cm−1.
HPLC and ESI-MS conditions
HPLC separation of the PGA, PFA polymer and PGA/PPy, PFA/PPy complexes was performed on a Waters Acquity UPLC at room temperature at a flow rate of 0.3 mL/min using gradient elution. The HRP-catalyzed complex was prepared in deionized water and the injection volume was 1.5 ml. HPLC-ESI-MS data were collected in negative ion mode using Waters Maldi Synapt Q-Tof mass software.
Characterization of silk fabrics
Cyclic voltammetry (CV) test
Cyclic voltammetry measurements of the treated silk samples were performed on a CHI 660D Electrochemical Workstation (CH Instruments Inc., Austin, USA). A glassy-carbon electrode was used as the working electrode, whereas platinum and Ag/AgCl electrodes were used as the counter and the reference electrodes, respectively. Prior to the test, a small piece of silk sample was fixed onto the glassy-carbon electrode using dried Nafion emulsion (1.5 wt%) (Karamyshev et al. Citation2003).
Thermogravimetric analysis (TGA)
The HRP-catalyzed polymeric products were analyzed for TGA using a TGA/SDTA 851e Thermogravimetric Analyzer (Mettler Toledo, Switzerland), in which approximately 5 mg of each sample was used. The samples were heated from 50 to 600°C at a heating rate of 20°C/min under nitrogen atmosphere.
Anti-UV properties measurements
The anti-UV ability of silk fabrics was determined by a UV transmittance analyzer YG(B)912E (Darong, China) (Cao and Wang Citation2017). The detective wavelength ranged from 280 to 400 nm. Each sample was measured five times and the results were averaged.
Evaluation of color depth
The colorimetric properties of each silk fabric were measured at 400 nm using a Color-Eye 7000 A Spectrophotometer (Gretag Macbeth, USA). In addition, the average value was calculated by measuring 10 times under standard lighting D65. The color depth (K/S value) was calculated based on the Kubelka-Munk equation, as shown in Equationequation (1)(1)
(1) below:
Where K is the absorbance coefficient, S is the scattering coefficient, and R is the reflectance.
Color fastness tests
The washing colorfastness and sunlight fastness were tested based on ISO 105-C06:2010 and AATCC-16.3:2014, respectively. The dry rubbing fastness and wet rubbing fastness were tested based on AATCC-8:2016 by mounting the silk fabrics to a panel and applying 10 strokes on both the dry and wet fabrics, respectively.
Results and discussion
UV-Vis spectra of natural phenol and PPy complexes in the presence of HRP
GA and FA can be formed into dimeric, oligomeric, and polymeric products using HRP. We studied representative structures of gallic acid dimers in our previous work (Baek et al. Citation2020). Sun, Xing, and Tang (Citation2015) observed that FA was polymerized using the laccase enzyme. As shown in , without HRP, GA and FA are colorless and transparent solutions, as shown in A-a B-a. Additionally, their absorbance curves are smooth. However, in the presence of HRP, new peaks for GA and FA appeared at 328 nm and 430 nm, respectively. Also, the colorless solution turned brown and yellow in color, respectively ().
Figure 1. UV-Vis absorption data obtained for natural phenol and the PPy complexes: A) GA; B) FA; a) untreated, b) treated with HRP, c) treated with HRP in the presence of PPy.
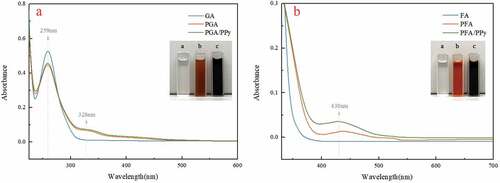
shows the oxidative polymerization process of GA using HRP. The aromatic ring of GA is a chromophore with three absorbance peaks at 184, 204 (not discussed), and 259 nm. The hydroxyl group (−OH) of GA has an auxochromic effect at the peak observed at 256 nm, displacing these peaks to a longer wavelength region due to the bathochromic effect (Cotero et al. Citation2017). As the reaction started, a new peak appeared at 328 nm. This is the absorption peak of the hydroxyl group caused by π →π* excitation. This indicates that GA was esterified to low molecular weight compounds via a dehydration step (Valdez et al. Citation2017). Thus, free radicals are formed and undergo condensation reactions with each other. This new peak (328 nm) of PGA and PGA/PPy was about 2 times higher after reacting with HRP. In addition, the color of the mixed solution of GA and Py changes to a black color during the enzymatic oxidation process, which corresponds to the formation of the conjugate structure. The results also showed a high activity of HRP on the polymerization of Py and GA.
shows the UV-Vis spectrum of the FA solution during the enzymatic reaction. The aromatic ring of FA is a chromophore with an absorbance peak at 290–330 nm; a new peak at 430 nm appeared after the reaction started. FA monomers were esterified to low molecular weight compounds through a dehydration step, resulting in them undergoing condensation reactions with each other and forming free radicals. As a result, the PFA solution’s color changes to yellow and shows a specific absorption band at 430 nm, indicating that enzymatic oxidation has occurred. This new peak (430 nm) was about two times higher when reacted with Py. Also, like GA, the color of the mixed solution of FA and Py changed to dark brown during enzymatic oxidation.
FT-IR spectroscopy
The FT-IR spectra obtained for GA, FA, Py and the PGA/PPy, PFA/PPy complex are shown in . GA and FA, polyphenolic compounds, had hydroxyl groups that could be utilized as potential sites for chemical modification (Singh et al. Citation2010).
In the Py spectra, large descending baselines appear between 1700 and 2200 cm−1 indicating free-electron conduction. We attribute the peak at 3400 cm−1 in the Py spectra to N – H bonds; the band at 1535 cm−1 corresponds to the pyrrole ring (Noroozian and Rahimi Citation2015).
The O – H stretching vibration peak was observed in the FTIR spectrum of GA at 3392 and 3290 cm−1. The O – H vibrations of the carboxylic acid groups appeared at 2657 cm−1. The peak observed at 1713 cm−1 corresponds to the C=O vibrations of GA (Baek et al. Citation2020). After we used HRP to catalyze the polymerization of GA, the peaks observed at 1362 and 1617 cm−1 could be attributed to the C – O and C=O vibrations, respectively, the absorbance bands observed at 1150 and 1043 cm−1 corresponded to the ether bond (C – O–C) vibrations. The differences in the two spectra (GA vs PGA) indicate that GA was polymerized in the presence of HRP and H2O2.
In the FTIR spectrum of FA, we observed a narrow and intensive O – H stretching of the phenolic ring at 3452 cm−1. The spectrum shows the propenoic acid C – H stretching mode at 3020 cm−1, in-plane bending at 1200 cm−1, and alkene C=C stretching at 1620 cm−1 (Sun, Xing, and Tang Citation2015). The peaks within 1380–1465 cm−1 and at 2968 cm−1 are due to CH3 vibrations. After enzymatic polymerization (PFA), the – OH band at 3452 cm−1 was changed to a broad band at 3408 and 3195 cm−1. Additionally, the C – H and C=C bands were weakened.
After polymerization with Py, two stretching vibration peaks (PGA: 3400, 3212 cm−1; PFA: 3408, 3195 cm−1) that were attributed to the O – H vibrations of the existing polyphenols became one broad peak. These peaks (PGA/PPy: 3408 cm−1, PFA/PPy: 3404 cm−1) are attributed to the N – H bond or O – H bond between PPy and polyphenol. The peaks we observed from 1693 to 1623 cm−1 for PGA/PPy and PFA/PPy are related to the C – N=C bond. In the spectra of the PFA/PPy complex, we observed a narrow peak attributed to the C – H vibrations at 2965 cm−1. In addition, the spectra show a characteristic band for C – N at 1030 cm−1. The difference in the spectrum that appears, depending on the presence of Py, indicated that Py and phenol were polymerized in the presence of HRP and H2O2.
HPLC-ESI-MS analysis
To analyze the mechanism of oxidative polymerization initiated by HRP, we investigated solutions of polymers (PGA, PFA, PGA/PPy, PFA/PPy) in the presence of HRP using HPLC ESI-MS in anion mode. The ESI-MS spectra observed for the polymers are shown in and . We observed that the major retention time for the polymerized products was 0.14 min.
Table 1. Possible mass-to-charge (m/z) assignments for PGA and PGA/PPy.
Table 2. Possible mass-to-charge (m/z) assignments for PFA and PFA/PPy.
Also, we observed ESI-MS fragmentation patterns of GA monomers at 169 ([M – H]–), 125([M – COOH]–), 170([M – H2O]); we studied these results in our previous experiments (Baek et al. Citation2020, Citation2021). Additionally, we observed the fragmentation pattern of FA monomer at 194([M]), 193([M – H]–), 195([M+H+]), 176 ([M – H2O]) and 150([M – COOH]–) (Zhu et al. Citation2016; Kumar and Pruthi Citation2015; Santos and Grace Citation2006). We found the fragmentation patterns of Py monomers at 66([M – H]–), 67([M]), and 68([M+H+]) (Athar, Hacaloglu, and Toppare Citation2004; Sinha et al. Citation2009). Therefore, the fragmentation patterns were used as the base peak for GA, FA, and Py in the ESI-MS spectrum.
The MS spectra recorded during the analysis of the PGA polymer are shown in and . The mass-to-charge (m/z) ratios observed were attributed to GA monomer (125, 169 Da), dimer (249 Da), trimer (327, 395 Da), tetramer (463, 541, 667 Da), and pentamer (826 Da). Our previous work (Baek et al. Citation2020, Citation2021) demonstrated that dimers, trimers, tetramers and pentamers of GA may have been formed via phenyl-phenyl (Ph – Ph) and oxygen-phenyl (O – Ph) linkages. The MS data obtained on the PGA/PPy complex are shown in and . shows that the molecular weight distribution was between 100 and 2000 Da. The polymeric structures observed in the MS spectra can be attributed to the different degrees of polymerization. These polymers were found to be GA dimers, trimers, and tetramers with molecular weights of 249, 327, and 463 Da, respectively. Meanwhile, in the MS spectrum, the peaks due to Py dimer, trimer, tetramer, and pentamer appeared at 130, 173, 249 and 317 Da, respectively. In addition, new peaks that appear to be co-polymers of PGA and PPy were observed at 327, 463, 531, 599, 669 and 689 Da, respectively. These can be attributed to the various combinations of PGA and PPy (MG +3 MP, 2 MG +2 MP, 2 MG +3 MP, 2 MG +4 MP, 2 MG +6 MP, 3 MG +2 MP, 3 MG +4 MP). Considering the FTIR results () we obtained previously, the copolymers between PGA and PPy were probably formed via Ph – Ph, O – Ph, and amino – phenyl (NH-Ph) linkages. shows that the PGA/PPy complex and silk fabric were formed through hydrogen bonding.
The MS data obtained for the PFA polymer are shown in and . The major retention time for the PFA polymerized product was observed at 0.14 min. The mass-to-charge (m/z) ratios recoded at 341, 533, 681, 919 and 1067 Da were attributed to the dimer (2 MF), trimer (3 MF), tetramer (4 MF), pentamer (5 MF), and hexamer (6 MF) of FA, respectively. The major products and possible structures of FA polymerization by lignin peroxidase (Sun, Xing, and Tang Citation2015; Ward et al. Citation2001) and hydrogen peroxide/peroxidase (Oosterveld et al. Citation1997) have already been reported. and show that the data on the complexes to which the PPy was added indicate a complex with a new molecular weight. These polymers were found to be MF+MP, MF +2 MP, MF +3 MP, 2 MF +2 MP, 2 MF +3 MP, and 3 MF +3 MP complexes with molecular weights of 238, 282, 341, 462, 612, and 793 Da, respectively.
Compared to PGA and PFA polymers, the molecular weight of the PGA/PPy and PFA/PPy composites was relatively low. This is thought to be because the Ph – Ph bonding and the NH – Ph bonding proceeded together due to the addition of the Py monomer. In addition, smaller peaks were observed in the regions of higher m/z than those of the protonated species in each peak group. These peaks may arise due to additives such as sodium or potassium.
Electroactivity of colored silk fabric
Electrochemical tests, used to further evaluate the electron transport capability of the fabrics, are shown in . The voltammograms of the two materials were closed circular curves, indicating that the surface of the composites had electron transfer movement during the measurement time. The larger the area of the circular curve, the greater the electrochemical properties and the conductivity of the material are (Wang et al. Citation2020).
The silk fabrics treated with the PGA/PPy and PFA/PPy complexes in 0.1 M phosphate buffer solution (pH 7.0) were electrochemically active, as can be seen from . We observed two oxidation peaks in the silk fabric treated with PGA/PPy in the anodic sweep at 298 and 761 mV. Additionally, we observed a reduction peak at 201 mV versus Ag/AgCl. Likewise, we observed two oxidation peaks for PFA/PPy-colored silk fabrics with anodic sweeps of 334 and 682 mV as well as reduction peaks of 168 mV versus Ag/AgCl. Silk fabrics treated only with PGA or PFA phenol also had a degree of electrochemical activity. But, after treatment with PPy, this electrochemical activity became more pronounced. Fabrics treated with PFA/PPy did not show a dramatic difference in the change in electrochemically active with the addition of Py, but a small peak at 682 mV was found, which was not observed in fabrics treated with PFA alone. Therefore, the PFA/PPy-treated fabrics showed no significant change in electrochemical activity compared to the PGA/PPy-treated fabrics, but showed a slight improvement with the addition of Py. Silk fabrics treated with phenol and PPy are well defined and reversible compared to the curves of silk fabrics without PPy. This confirms the high electrochemical activity of colored silk fabrics, indicating the possibility of using these types of polymers in functional textiles.
Thermal properties of treated silk fabrics
The thermal stabilities of treated silk fabrics were investigated by non-isothermal thermogravimetric analysis (). We see from the TG curve () that the overall weight loss is attributable to thermal degradation. Weight loss below 100°C was mainly due to volatilization of moisture in the sample. The weight remained almost constant between 100°C and 250°C (Wang et al. Citation2020). When the temperature was raised from 300°C to 400°C, the weight loss of all silk samples increased dramatically, which could be attributed to silk fibroin degradation of the protein backbone and side chains (Darshan et al. Citation2017).
The major thermal degradation temperature of PGA, PFA, PGA/PPy and PFA/PPy was 345, 344, 339 and 345°C, respectively. This indicated that the major thermal degradation temperature was similar in all four samples treated with and without PPy. Thermogravimetric analyses for PGA and PGA/PPy are shown in . Up to 600°C, about 22% of the mass of PGA colored silk fabric remained, but 31.7% of the mass of PGA/PPy treated silk fabric remained. For silk fabrics treated with PFA and PFA/PPy (), 32% of the mass of the colored silk fabrics with the PFA/PPy remained and 25% of the mass of the PFA treated silk fabric remained up to 600°C.
These results suggest that silk fabrics treated with PPy retain more mass than silk fabrics colored only with natural phenol. Therefore, the silk fabrics treated with PGA/PPy and PFA/PPy had enhanced thermal stability compared to those colored with PGA and PFA.
Anti-UV activity of silk fabrics
Anti-UV ability was measured by examining the UPF (ultraviolet protection factor) and transmittance of UVA and UVB radiation in all the silk samples. As shown in , the UPF values of silk fabrics treated with only PGA or PFA were 34.8 and 34.3, respectively. These were higher compared to untreated silk fabrics. It appears that the influence of the aromatic structure of GA or FA coating led to these results; the aromatic structure absorbs high-energy UV rays, effectively reducing the transmittance of UVA and UVB radiation.
Table 3. Anti-UV activity of the treated silk fabrics.
However, silk fabrics treated with PGA/PPy and PFA/PPy showed UPF values of 21.8 and 26.7, respectively. This is probably related to the degree of polymerization of phenol. In the MS analysis mentioned above (3.3), we confirmed that the degree of polymerization decreased compared to the PGA and PFA polymers after adding PPy. Therefore, we found that the UV protection of silk fabrics treated with phenol and PPy was lower than that of silk fabrics treated with phenol only.
Dying depth of treated silk fabrics
The K/S values of the silk fabrics treated with PGA and PFA as well as the PGA/PPy and PFA/PPy complexes are shown in and . The difference in the dyeing depth and color of the silk fabrics was measured using the K/S (color depth), L*, a*, b* and h* values. L* refers to the degree of lightness, a* refers to degree of redness and greenness, and b* refers to the degree of yellowness and blueness, and h* refers to hue angle values (Liu et al. Citation2013).
Table 4. Cielab values obtained for the dyed silk samples.
The K/S value of silk fabrics treated with PGA/PPy (K/S = 16.5) was slightly higher than that of silk fabrics colored only with PGA (K/S = 15.7), but there was only a small difference. However, the L*, a*, and b* value of the silk fabric treated with the PGA/PPy complex was significantly lower, indicating that it was colored a darker color. Similarly, the K/S value of silk fabrics treated with PFA/PPy (K/S = 18.7) was also slightly higher than that of silk fabrics colored with PFA only (K/S = 17.3). There was no significant difference in the K/S values of the two colored fabrics, but there was a significant difference in the color of the fabrics. Fabrics colored with PFA/PPy had much lower a* values than fabrics colored only with PFA; they were colored yellowish brown when viewed with the naked eye.
Compared to the untreated silk fabrics, the sample treated with Phenol (GA, FA) or Phenol/PPy had colors characteristic of the formation of the colored compounds. In this study, the colored compounds were PGA, PFA, PGA/PPy, and PFA/PPy catalyzed by HRP in solution. These colorimetric parameters presented an intuitive expression of the best coloration of a dyed silk fabric treated with Phenol, Py, and HRP.
Colorfastness
Eco-dyed fabrics using natural materials have lower fastness than that of chemical dyeing. In particular, silk fabric is easily discolored by the surrounding environment, so its durability is weak compared to the durability of other fabrics. Therefore, the washing, rubbing, and light fastness properties were studied for colored silk fabrics ().
Table 5. The color fastness of the dyed silk fabric.
The washing fastness test was used to measure the degree of discoloration and transfer to other fabrics (cotton and silk) during washing. shows that the wash fastness decreases after adding PPy. In particular, the transfer to silk fabrics occurred during washing; this phenomenon was more pronounced in FA-treated fabrics. PGA and PFA compounds showed better washing fastness compared to PGA/PPy and PFA/PPy compounds, which we believe is due to the lower degree of polymerization of polyphenol and PPy than that of phenol polymer. The rubbing fastness of both PGA and PGA/PPy fabrics were grades 4–5 or higher. However, the dry rubbing fastness of the PGA/PPy fabrics was lower than that of the PGA-treated fabrics. Silk samples treated with PFA and PFA/PPy showed an average of 3–4 grades, and in wet rubbing fastness, fabrics colored with PFA/PPy showed worse fastness than fabrics treated with PFA. The light fastness of silk fabrics colored with PGA and PGA/PPy were grades 4–5 and 5, respectively. Also, the fabrics colored with PFA and PFA/PPy were grades 2–3 and 3, respectively. This suggests that fabrics treated with PPy have higher lightfastness than fabrics treated only with phenol. Therefore, silk fabrics treated only with phenol exhibited higher fastness to washing and rubbing compared to silk fabrics treated with PPy. However, silk samples treated with PPy had higher grades of light fastness.
Conclusions
In this study, natural phenols (GA, FA) and pyrrole monomer were successfully polymerized by the oxidative polymerization of HRP. They were successfully used to color silk fabrics with their characteristic colors.
UV-Vis spectroscopy, FTIR spectroscopy, and HPLC/ESI-MS were used to analyze the possible structures and bonding modes of polyphenols (PGA, PFA) and polypyrrole (PPy) complexes. Copolymers were likely formed between PGA, PFA, and PPy via Ph-Ph, O-Ph and amino-phenyl (NH-Ph) bonds.
In addition, we analyzed the chemical and physical properties of the colored silk fabric. Silk fabrics treated with PPy displayed higher electrochemical activity and improved thermal stability compared to silk fabrics treated without PPy. However, the UV protection of PGA/PPy and PFA/PPy treated silk fabrics was lower than that of silk fabrics treated with only natural phenol. As a result of color depth analysis, the K/S values of silk fabrics colored with PGA/PPy and PFA/PPy were slightly higher than those of silk fabrics colored with natural phenol only. In the case of the silk fabrics treated with PPy, the L*, a*, b*, C*, and h* values all decreased. Therefore, it was treated with a less saturated color than fabrics colored only with natural phenols. As a result of analyzing the color fastness of the silk fabric obtained, the silk fabrics colored only with phenol showed higher washing fastness and rubbing fastness than the silk fabric treated in the presence of PPy. However, the silk samples stained with PGA/PPy and PFA/PPy demonstrated higher light fastness.
Silk fabrics treated with PGA and PGA/PPy were colored brown and dark brown, respectively. Fabrics treated with PFA and PFA/PPy were colored yellow and reddish brown, respectively. Previously studied pyrrole-treated silk has usually been dark green or black; they did not vary in color. However, in this experiment, we confirmed that each unique color was formed due to the polymerization of natural phenol and pyrrole. This is meaningful because of the demand for color diversification in natural dyeing and the production of functional silk fabrics using pyrrole.
As a result, the oxidative polymerization of phenol and Py on silk fibers under mild reaction conditions using HRP allowed us to produce technically conductive silk fabrics that combine the superior chemical and physical properties of silk fabrics with superior levels of electrochemical activity and thermal stability. These results are important because they address the possible applications of conductive silk fabrics, from the design of apparel to technical uses, including the most advanced field of knowledge-based multifunctional materials.
Highlights
Natural phenols (Gallic acid, Ferulicacid) and pyrrole monomer were successfully polymerized by the oxidative polymerization of HRP, and, successfully colored silk fabrics with characteristic colors.
Silk fabrics dyed with PPy displayed higher electrochemical activity and improved thermal stability compared to silk fabrics dyed without PPy.
As a result of color depth analysis, the K/S values of silk fabrics dyed with PGA/PPy and PFA/PPy were slightly higher than those of silk fabrics dyed with natural phenol only.
As a result of analyzing the color fastness of the silk fabric obtained, the silk fabrics dyed only with phenol showed higher washing fastness and rubbing fastness than the silk fabric dyed in the presence of PPy.
Disclosure statement
No potential conflict of interest was reported by the author(s).
Additional information
Funding
References
- Athar, I., J. Hacaloglu, and L. Toppare. 2004. Characterization of doped polypyrrole–poly(methylthienyl methacrylate) films via pyrolysis mass spectrometry. Polymer International 53 (7):926–15. doi:10.1002/pi.1467.
- Baek, N. W., X. R. Fan, J. G. Yuan, and Q. Wang. 2020. Polymerization and dyeing properties of gallic acid on silk fabric catalyzed by horseradish peroxidase. Fibers and Polymers 22 (8):2145–55. doi:10.1007/s12221-021-0673-Yv.
- Baek, N. W., X. R. Fan, X. Zhang, D. Wang, and J. G. Yuan. 2021. Horseradish peroxidase-catalyzed “template” polymerization of gallic acid for the functionalization of silk fabrics. Journal of Natural Fibers. Advance online publication. 1–14. doi:10.1080/15440478.2021.1982836.
- Bai, R. B., Y. Y. Yu, Q. Wang, J. G. Yuan, and X. R. Fan. 2016. Laccase-mediated in situ polymerization of pyrrole for simultaneous coloration and conduction of wool fabric. Textile Research Journal 88 (1):27–35. doi:10.1177/0040517516673336.
- Boschi, A., C. Arosio, I. Cucchi, F. Bertini, M. Catellani, and G. Freddi. 2008. Properties and performance of polypyrrole (PPy)-coated silk fibers. Fibers and Polymers 9 (6):698–707. doi:10.1007/s12221-008-0110-5.
- Cao, J. L., and C. X. Wang. 2017. Multifunctional surface modification of silk fabric via graphene oxide repeatedly coating and chemical reduction method. Applied Surface Science 405:380–88. doi:10.1016/j.apsusc.2017.02.017.
- Cetiner, S. 2014. Dielectric and morphological studies of nanostructured polypyrrole-coated cotton fabrics. Textile Research Journal 84 (14):1463–75. doi:10.1177/0040517514523180.
- Cotero, D. A., A. V. Carvajal, R. M. G. Espinoza, O. D. Garcia, and A. D. Lopez. 2017. Radical scavenging activity of an inulin-gallic acid graft and its prebiotic effect on lactobacillus acidophilus in vitro growth. Journal of Functional Foods 29:135. doi:10.1016/j.jff.2016.12.014.
- Cucchi, I., A. Boschi, C. Arosio, F. Bertini, G. Freddi, and M. Catellani. 2009. Bio-based conductive composites: Preparation and properties of polypyrrole (PPy)-coated silk fabrics. Synthetic Metals 159 (3–4):246–53. doi:10.1016/j.synthmet.2008.09.012.
- Darshan, G. H., D. Kong, J. Gautrot, and S. K. Vootal. 2017. Fabrication and characterization of conductive conjugated polymer-coated antheraea mylitta silk fibroin fibers for biomedical applications. Macromolecular Bioscience 17 (7):1600443. doi:10.1002/mabi.201600443.
- Geetha, S., and D. C. Trivedi. 2004. Studies on polypyrrole film in room temperature melt. Materials Chemistry and Physics 88 (2–3):388–97. doi:10.1016/j.matchemphys.2004.08.006.
- Jager, E. W. H., E. Smela, and O. Inganaes. 2000. Microfabricating conjugated polymer actuators. Science 290 (5496):1540–45. doi:10.1126/science.290.5496.1540.
- Karamyshev, A. V., S. V. Shleev, O. V. Koroleva, A. I. Yaropolov, and I. Y. Sakharov. 2003. Laccase-catalyzed synthesis of conducting polyaniline. Enzyme and Microbial Technology 33 (5):556–64. doi:10.1016/S0141-0229(03)00163-7.
- Kumar, N., and V. Pruthi. 2015. Structural elucidation and molecular docking of ferulic acid from parthenium hysterophorus possessing COX-2 inhibition activity. 3 Biotech 5 (4):541–51. doi:10.1007/s13205-014-0253-6.
- Liu, R. R., A. X. Dong, X. R. Fan, Q. Wang, Y. Y. Yu, and A. C. Paulo. 2015. HRP-mediated polyacrylamide graft modification of raw jute fabric. Journal of Molecular Catalysis B, Enzymatic 116:29–38. doi:10.1016/j.molcatb.2015.03.004.
- Liu, J., J. F. Lu, J. Kan, and C. H. Jin. 2013. Synthesis of chitosan-gallic acid conjugate: Structure characterization and in vitro anti-diabetic potential. International Journal of Biological Macromolecules 62:321. doi:10.1016/j.ijbiomac.2013.09.032.
- Mabrook, M. F., C. Pearson, and M. C. Petty. 2006. Inkjet-printed polypyrrole thin films for vapour sensing. Sensors & Actuators, B: Chemical 115 (1):547–51. doi:10.1016/j.snb.2005.10.019.
- Malhotra, U., S. Maity, and A. Chatterhee. 2014. Polypyrrole-silk electro-conductive composite fabric by in situ chemical polymerization. Applied Polymer 41336:1–10. doi:10.1002/APP.41336.
- Noroozian, E., and M. Rahimi. 2015. Application of poly(Pyrrole-co-phenol) electrosynthesized for stir-bar sorptive extraction of polycyclic aromatic hydrocarbons and liquid chromatographic analysis. Journal of Liquid Chromatography & Related Technologies 38 (2):172–81. doi:10.1080/10826076.2014.896815.
- Nystrom, G., A. Razaq, M. Strmme, L. Nyholm, and A. Mihranyan. 2009. Ultrafast all polymer paper-based batteries. Nano Letters 9 (10):3635–39. doi:10.1021/nl901852h.
- Oosterveld, A., J. H. Grabber, G. Beldman, J. Ralph, and A. G. J. Voragen. 1997. Formation of ferulic acid dehydrodimers through oxidative cross-linking of sugar beet pectin. Carbohydrate research 300 (2):179. doi:10.1016/S0008-6215(97)00041-4.
- Ramanavicius, A., A. Ramanaviciene, and A. Malinauskas. 2006. Electrochemical sensors based on conducting polymer—polypyrrole. Electrochimica Acta 51 (27):6025–37. doi:10.1016/j.electacta.2005.11.052.
- Santos, S., and J. Graca. 2006. Glycerol-ω-hydroxyacid-ferulic acid oligomers in cork suberin structure. Holzforschung 60 (2):171–77. doi:10.1515/HF.2006.028.
- Singh, R. K., D. Rai, D. Yadav, A. Bhargava, J. Balzarini, and E. D. Clercq. 2010. Synthesis antibacterial and antiviral properties of curcumin bioconjugates bearing dipeptide, fatty acids and folic acid. European Journal of Medicinal Chemistry 45 (3):1078–86. doi:10.1016/j.ejmech.2009.12.002.
- Sinha, V., T. G. Custer, T. Kluepfel, and J. Williams. 2009. The effect of relative humidity on the detection of pyrrole by PTR-MS for OH reactivity measurements. International Journal of Mass Spectrometry 282 (3):108–11. doi:10.1016/j.ijms.2009.02.019.
- Sun, S. S., T. L. Xing, and R. C. Tang. 2015. Enzymatic dyeing and functional finishing of textile fibers with ferulic acid. Indian Journal of Fiber & Textile Research 40:62–69.
- Valdez, J. G. L., R. R. B. Quintana, J. A. A. Barrios, G. R. C. Montfort, A. M. M. Wilson, J. N. M. Ruiz, T. J. M. Santana, A. R. Chu, and G. C. Qulica. 2017. Structural and physicochemical characterization of nanoparticles synthesized from an aqueous extract of wheat bran by a cold-set gelation/desolvation approach. Food Hydrocolloids 62 (1):165–73. doi:10.1016/j.foodhyd.2016.07.034.
- Wang, Y. Q., H. Yu, H. F. Liu, and Y. B. Fan. 2020. Double coating of graphene oxide polypyrrole on silk fibroin scaffolds for neural tissue engineering. Journal of Bioactive and Compatible Polymers 35 (3):088391152091390. doi:10.1177/0883911520913905.
- Ward, G., Y. Hadar, I. Bilkis, L. Konstantinovsky, and C. G. Dosoretz. 2001. Initial steps of ferulic acid polymerization by lignin peroxidase. The Journal of Biological Chemistry 276 (22):18734. doi:10.1074/jbc.M009785200.
- Zhao, Y. H., C. M. Niu, J. Q. Shi, Y. Y. Wang, Y. M. Yang, and H. B. Wang. 2018. Novel conductive polypyrrole/silk fibroin scaffold for neural tissue repair. Neural Regeneration Research 13 (8):1455–64. doi:10.4103/1673-5374.235303.
- Zhou, H. F., D. J. Yang, X. Q. Qiu, X. L. Wu, and Y. Li. 2013. A novel and efficient polymerization of lignosulfonates by horseradish peroxidase/h2o2 incubation. Applied Microbiology and Biotechnology 97 (24):10309–20. doi:10.1007/s00253-013-5267-1.
- Zhu, J. F., Y. X. Heng, D. X. Zhang, Y. Wen, H. Li, and G. H. Zhao. 2016. Synthesis and characterization of enzymatically cross-linked feruloyl amylopectin for curcumin encapsulation. Journal of Biological Macromolecules 85:126–32. doi:10.1016/j.ijbiomac.2015.12.073.