ABSTRACT
Impaired wound healing particularly in diabetics creates a significant healthcare burden. The study aimed to evaluate the effect of keratin-butyrate fibers (FKDP +0.1%NaBu) in a full-thickness skin wound model in 30 diabetic rats. Physicochemical examination showed that the obtained dressing possesses a heterogeneous structure and butyrate was slowly released into the wound. Moreover, the obtained dressing is nontoxic and supports cell growth. In vivo results showed that keratin-butyrate dressing accelerated wound healing on days 4 and 7 post-injury (p < .05). Histopathological and immunofluorescence examination revealed that applied dressing stimulated macrophage infiltration, which favors tissue remodeling and regeneration. The dressing was naturally incorporated into regenerating tissue. The highest mRNA expression level of interleukin 1β (IL-1β) was observed during the first 2 weeks in the control wounds compared to FKDP +0.1%NaBu treated wounds, in which IL-1β was significantly decreased. In FKDP +0.1%NaBu dressed wounds, mRNA expression of IL-10 and VEGF increased significantly (p < .05) from day 14. Keratin-butyrate treated wounds enhanced mRNA expression of keratin 16 and 17 and zonula occludens protein-1 and junctional adhesion molecules (p < .05) on days 14, 21, and 28 post-injuries. Our study showed that keratin butyrate dressing is safe and can efficiently accelerate skin wound healing in diabetic rats.
摘要
伤口愈合受损,尤其是糖尿病患者,造成了巨大的医疗负担. 本研究旨在评估角蛋白丁酸纤维 (FKDP +0.1%NaBu) 在30只糖尿病大鼠全层皮肤创伤模型中的作用. 理化检查表明,获得的敷料具有异质结构,丁酸盐缓慢释放到伤口中. 此外,所得敷料无毒,并支持细胞生长。体内实验结果显示,丁酸角蛋白敷料可在损伤后第4天和第7天加速伤口愈合 (p < .05) . 组织病理学和免疫荧光检查显示,敷料刺激巨噬细胞浸润,有利于组织重塑和再生. 敷料自然融入再生组织中. 与FKDP +0.1%NaBu处理的伤口相比,前两周对照伤口的白细胞介素1β (IL-1β) mRNA表达水平最高,其中IL-1β显著降低. 在FKDP +0.1%NaBu包扎伤口中,IL-10和VEGF的mRNA表达从第14天开始显著增加 (p < .05) . 在损伤后14天、21天和28天,丁酸角蛋白治疗的伤口中,角蛋白16和17的mRNA表达增强,小带阻断蛋白-1和连接粘附分子 (p < .05) . 我们的研究表明,丁酸角蛋白敷料安全有效地促进糖尿病大鼠皮肤伤口愈合.
Introduction
Diabetes is a common chronic metabolic disorder that is characterized by hyperglycemia and loss of glucose homeostasis. Patients with diabetes suffer from chronic nonhealing wounds, which remain a global medical problem creating high burden for health care system (Spampinato et al. Citation2020; Wan et al. Citation2021). The main goal of tissue engineering and regenerative medicine is to search for a new type of biomaterials that would accelerate the healing process. Several biomaterials including keratin, gelatin, or collagen were examined as potential bandages, which can promote tissue recovery.
Keratin biomaterials (soluble or insoluble) gain much attention since they are biocompatible, biodegradable and support cell migration and proliferation (Bochynska-Czyz et al. Citation2020; Konop et al. Citation2017; Konop, Rybka, and Drapała Citation2021; Moay et al. Citation2021; Park et al. Citation2015; Tachibana et al. Citation2002; Verma et al. Citation2008). Keratin fibers can release keratin peptides into the wound bed. These peptides activate keratinocytes and stimulate their proliferation to provide epithelialization of the open wound (Feroz et al. Citation2020; Konop, Rybka, and Drapała Citation2021). Cells at the migrating wound front showed up-regulation of genes for keratin 6, keratin 16, and keratin 17 (KRT) in a sequence consistent with keratinocyte activation occurring earlier in the keratin-treated wound and can accelerate skin regeneration (Pechter et al. Citation2012; Zhang, Yin, and Juan Zhang Citation2019). The KRT16-KRT6 keratin pair functions to maintain collective cell migration and integrity by increasing cell–cell and cell–matrix contact, decreasing cell migration and directionality while maintaining mechanical integrity. KRT17 can pair with either KRT6 or KRT5 to drive keratinocyte hyperproliferation by increasing cell size and increasing protein synthesis. KRT17 can also induce Th1/Th17 cytokine production from keratinocytes (Mazzalupo et al. Citation2003; Zhang, Yin, and Juan Zhang Citation2019).
The obtained keratin dressing fulfills criteria of ideal wound dressing proposed by Turner. The main advantage is that this dressing can be compared to allogeneic transplantation, since rat fur was used as a substrate for final dressing production. Moreover, the dressing is biocompatible and biodegradable, and it is naturally incorporated into regenerated tissue without any signs of inflammatory reaction (Konop et al. Citation2017; Konop, Rybka, and Drapała Citation2021). What distinguishes it from other biomaterials (natural or synthetic), it can be used directly as a wound dressing (Lahiri et al. Citation2021; Zhong et al. Citation2021).
Moreover, due to the expanded surface of the keratin biomaterial, it is possible to incorporate substances with analgesic or anti-inflammatory effects into it. In this context, sodium salt of n-butyric acid (Na-Bu) gains attention due to its anti-inflammatory properties. Butyrate is produced by fermentation of dietary fiber by anaerobic bacteria in the colon. Na-Bu induces cell differentiation and is also an inhibitor of histone deacetylase (HDAC). It is a nontoxic, naturally occurring compound that relieves epithelial inflammation (Keshava and Gope Citation2015).
To our knowledge, there are no reports on the biological functions of keratin-butyrate scaffolds in the healing of diabetic skin wounds. In this study, we evaluated keratin scaffolds containing butyrate as a wound dressing in a full-thickness skin wound model in diabetic rats. The results suggest that Na-Bu supports cutaneous wound healing in diabetic rats. Our data also show that FKDP +0.1%NaBu might be crucial for the expression of KRT16, KRT17, tight junction protein – ZO1, JAM-A, and down-regulation of IL-1 during wound healing in diabetic conditions.
Materials and methods
Reagents were described in supplementary materials.
Scanning electron microscopy (SEM) and water absorbance test
Samples were prepared according to the method described previously (Konop et al. Citation2017). The surface structure of the sample was analyzed using a Hitachi S-3400N Scanning Electron Microscope with a variable vacuum using the high vacuum mode (SE secondary electron detector). Fiber diameter was measured for raw fur, FKDP, and FKDP +0.1%NaBu. All technical details are described in supplementary materials.
Preparation of keratin butyrate dressing and in vitro drug release from the wound dressing
The preparation of the keratin-derived wound dressing from rat fur was performed as published previously (Konop, Rybka, and Drapała Citation2021) and supplemented with 0.1% sodium butyrate (NaBu). The release of butyrate from keratin dressing (FKDP +0.1%NaBu) was examined according to the method described previously (Onyszkiewicz et al. Citation2019). All technical details are described in supplementary materials.
Evaluation of the antibacterial activity of the dressing
The obtained dressing was tested for antibacterial properties utilizing the agar well diffusion minimal inhibitory concentration method. Technical details are described in supplementary materials.
Microwave plasma – atomic emission spectrometer measurements
The mineralization process was performed with the use of the microwave mineralization system Magnum II, Ertec. Technical details are described in supplementary materials.
Hemolysis assay
Compound-induced hemolysis was measured following a modified Mazzarino procedure (Mazzarino et al. Citation2015) with a modification described in supplementary materials.
Cells viability assay
Cell viability was determined with Cell Proliferation Kit I MTT (Sigma Aldrich, St. Louis, MO, USA). All experiments were performed according to the manufacturer’s protocol and described in the supplementary materials.
Animals
In this study 13–15-week-old male (N = 30), Sprague Dawley rats were obtained from the Central Laboratory for Experimental Animals, Medical University of Warsaw (Poland). Procedures adhered to guidelines published in European Directive 2010/63/EU on the protection of animals used for scientific purposes and the study was approved by the National Ethical Committee for Animal Experiments in Warsaw, Poland (Certificate of approval no. 13/2021).
Iatrogenically induced diabetes
Diabetes in rats was induced with an intraperitoneal injection of streptozotocin (STZ) at a dose of 65 mg/kg. Rats were considered diabetic if three consecutive measurements of a blood glucose level taken from the lateral saphenous vein were higher than 250 mg/dL. Technical details are described in supplementary materials.
Surgical procedure
Skin wounds were performed according to the protocol described previously (Konop et al. Citation2020). Skin biopsies were taken from control and FKPDP +0.1%NaBu treated wounds at the relevant time points for histological and molecular biology examination. Technical details are described in supplementary materials.
Histopathological and immunofluorescence examination
Preparation of histological specimens was identical for all examined tissue samples according to the standard procedure at Histology Laboratory at the Department of Dermatology, Warsaw, Poland, as described previously (Konop et al. Citation2018, Citation2020). Immunofluorescence staining for macrophages, Nuclear Factor kappa B (NF-κβ; subunits: p65 and p50), Tumor protein P53, VEGF, Flk-1 receptors, pancytokeratin, and neutrophils were performed with the appropriate primary and secondary antibodies. Results were examined using an Optiphot-2 Nikon fluorescent microscope (Nicon Instruments Inc., Toki, Japan) equipped with the appropriate filters and recorded with a Model DS-L1 Nikon camera. All technical details are described in supplementary materials.
Protein extraction and immunoblotting analysis
For the protein analysis, the total protein extract was prepared from the control and FKDP +0.1%NaBu treated wound area (central part). Technical details are described in supplementary materials.
RNA isolation and RT-qPCR
Total cellular RNA was extracted from the wound area (central part), (approx. 15 mg of wet tissue) using Trizol reagent (Invitrogen, Carlsbad, USA) according to the manufacturer’s protocol. Technical details are described in supplementary materials.
Statistical analyses
All the data are presented as means ± SEM. Determination of statistical significance was made using either t-tests (two-tailed), one-way ANOVA (with Bonferroni post hoc test), or two-way ANOVA (with Bonferroni multiple comparisons), depending on the data structure. The minimal level of significance was P ≤ .05. All comparisons were calculated using GraphPad Prism 5.0 software for Windows (GraphPad Software, San Diego, CA, USA).
Results and discussion
In the present study, we investigated a novel therapeutic approach to wound healing – a wound dressing based on keratin-derived powder loaded with butyrate. A novel finding of our study is that such dressing supported skin wound healing, increased macrophage infiltrate into treated wounds, decreased inflammatory reaction by reduction of mRNA expression of IL-1β, enhanced level of IL-10 and bicellular tight junction protein (ZO1 and JAM-A).
SEM analysis showed that the treatment of rat fur with sodium hydroxide and pepsin generated a distinct decrease in hair shaft thickness, by partial degradation of the hair cuticle and cortical cells. Physicochemical examination showed that obtained keratin-butyrate scaffolds possess heterogeneous structure ( a–), and butyrate was slowly released into the wound during the following 5 days (, Fig S1 in supplementary materials).
Figure 1. SEM images of untreated rat fur (a), FKDP before grinding (b), and FKDP +0.1%NaBu (c), (d) fiber diameter e) water absorption test. The data were only tested by the t-test for dependent samples (mean ± standard error of the mean). Original magnification of SEM images: 300 × .
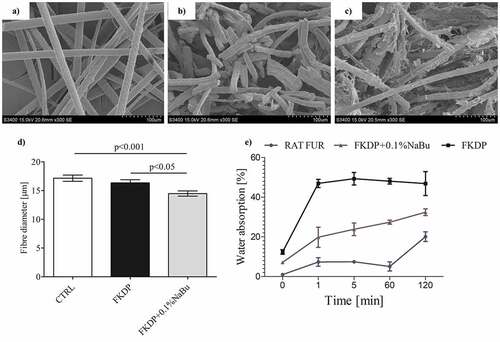
Table 1. Semi-quantitative results of the LC-MS study: detected concentration of butyrate released from keratin dressing into PBS measured during 120 h.
Our earlier studies showed that the heterogeneous structure of keratin allows the slow release of compounds with anti-inflammatory or antibacterial properties. The obtained dressing possesses weak antimicrobial properties (Fig. S2, Table S1 in supplementary materials). Moreover, the obtained dressing possesses the ability for fluid absorption () and can create a moist environment, which fits the characteristics of an ideal dressing according to Turner’s criteria (Konop et al. Citation2017, Citation2020; Konop, Rybka, and Drapała Citation2021; Ma et al. Citation2012).
In vitro experiment showed that FKDP +0.1%NaBu scaffolds were nontoxic and increased cell viability compared with cells treated with FKDP alone. In our earlier studies, we showed that three main fractions of fur keratin protein: 0.05–0.15 mm; 0.15–0.5 mm; 0.5–1.0 mm in length, and an average diameter of 50 µm can be obtained (Bochynska-Czyz et al. Citation2020).
Hemolytic activity of tested dressings was evaluated according to Mazzarino et al. (Citation2015). It was observed that up to the concentration of 1% (v/w), FKDP and FKDP-0.1%NaBu dressings do not induce hemolytic process () and could be safely used as a potential wound dressing.
Figure 2. Effect of FKDP and FKDP +0.1%NaBu on: a) rat red blood cells hemolysis, b) cell viability. The data are statistically significant when p < .05, *- p < .05, *** p < .001, 2-way ANOVA followed by Bonferroni post hoc tests, mean ± standard error of the mean.
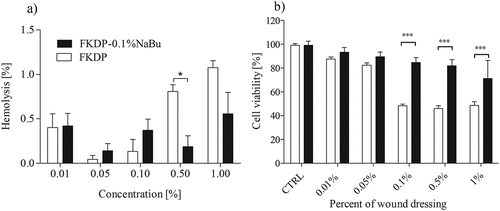
Fibroblasts exposed to FKDP and FKDP +0.1%NaBu at concentrations of 0.1%, 0.5%, and 1% presented decreased cell viability after 24 h incubation period (). At those concentrations, FKDP +0.1%NaBu caused a significantly lower decrease in cell viability when compared to cells exposed to FKDP alone. It is well known that Na-Bu can maintain the stability of the gut environment by regulating various cellular functions, including proliferation, differentiation, apoptosis, and intestinal epithelial permeability (Qiu et al. Citation2017; Yang et al. Citation2021).
Histological evaluation showed that the healing process of the wounded tissue in FKDP +0.1%NaBu treated wound was significantly faster (p < .05) compared with untreated side on days 4 and 7 post-injury (). From day 14 onwards, the rate of wound healing was similar, but statistical significance was lost. On the 28th day of observation, the regenerated skin in the butyrate keratin-treated wound showed full-thickness epidermis and condensed collagen (Fig. S4 in supplementary materials) fibrils in the dermis with a scant keratin remnants present in the macrophages with appearing hair follicle epithelia. These processes were delayed at the untreated wounds for several days. Moreover, it was observed that the number of microhemorrhages (extravasated erythrocytes) significantly decreased in keratin-butyrate treated wounds during the recovery period compared with untreated side (). Similar results were observed in our earlier studies (Konop et al. Citation2018, Citation2020).
Figure 3. The effect of FKDP-0.1%nabu dressing on: a) changes in the number of microhemorrhages, b) on skin wound healing. Rat numbers at Day 4, N = 30; Day 7, N = 24; Day 14, N = 18; Day 21, N = 12; Day 28, N = 6. c) Hematoxylin-eosin staining of harvested tissues from control and FKDP +0.1%NaBu treated wounds, magnification 100x. The data were only tested by the t-test for dependent samples (for each post-wounding day separately).
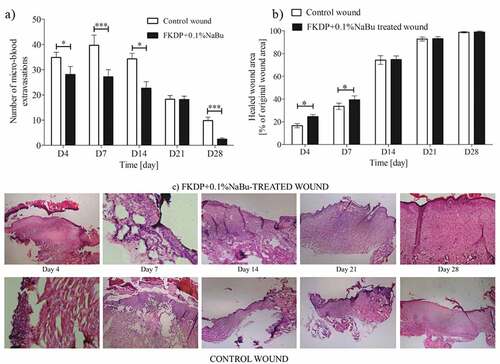
It can be concluded that the addition of 0.1% sodium butyrate as a surface modifier of keratin particles significantly accelerated the healing process in diabetic rats in the early stage of healing. This is most likely an example of the synergistic effect of both FKDP and sodium butyrate on wound healing process. Keshava and Gope (Citation2015) examined the effect of topical application of sodium butyrate (Na-Bu), EGF, and PDGF-BB on the acute cutaneous wound healing in a streptozotocin-induced diabetic mouse model. They showed that the topical application of a combination of EGF plus PDGF-BB along with a differentiation-inducing agent as Na-Bu may eventually be considered for clinical treatment and management of various human wounds and burns. Butyrate can modulate cell growth, and the effect is concentration- and cell line type-dependent (Dyson, Daniel, and Surrey Citation1992; Guilloteau et al. Citation2010).
In the next step, we evaluated the impact of Na-Bu on mRNA expression of pro- and anti-inflammatory cytokines, growth factors, cytokeratins, and bicellular tight junction proteins (Lee et al. Citation2017; Liu et al. Citation2018; Mowat and Agace Citation2014; Park et al. Citation2007). Butyrate treatment diminished mRNA expression of the proinflammatory interleukin-1β in the keratin-butyrate treated wound during the first 2 weeks after injury, which suggested the promotion of wound healing by butyrate (). Moreover, immunofluorescence studies showed that strong labeling for macrophages was observed in keratin-butyrate treated wounds, whereas neutrophils predominated in the control wounds (, Fig S4 in supplementary materials). This confirmed the anti-inflammatory properties of butyrate (Chen et al. Citation2018) released from FKDP +0.1%NaBu dressing. On the other hand, some studies showed that butyrate enhances interleukin-1β production by activating caspase-1 (Ohira et al. Citation2012).
Figure 4. The expression of IL-1β, IL-10, TNF-α, VEGF, KRT1, KRT10, KRT16, and KRT17 mRNA levels in skin biopsies. The data were only tested by the t-test for dependent samples (for each post-wounding day separately, mean ± standard error of the mean) and were statistically significant when p-value <.05, were: * - p < .05,**-p < .01, *** - p < 0,001.
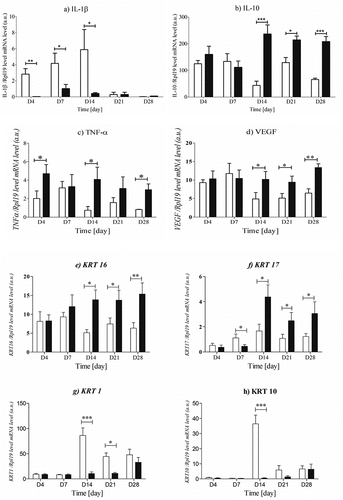
Figure 5. Tissue biopsy taken from FKDP +0.1%NaBu (upper panels) treated wound and control wound (lower panel) immunolabeled for a) macrophages (red), p53 (green), and cell nuclei (blue), b) Flk-1 (red), pancytokeratin (green), and cell nuclei (blue). Note the changes in immunoreactive cell numbers during the healing course.
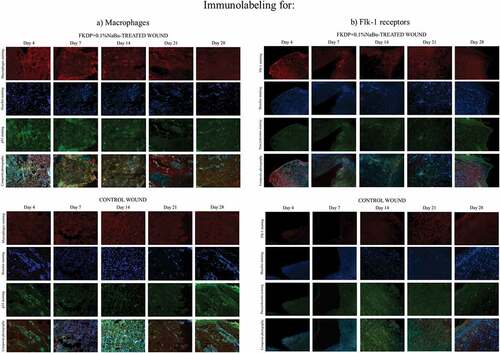
In keratin-butyrate treated wounds, we observed enhanced mRNA expression of the IL-10 on the days 14, 21, and 28 post-injury, which proves the anti-inflammatory properties of the tested dressing (). There is deficient information about the impact of butyrate on skin wound healing; however, it is well known that butyrate stimulates anti-inflammatory mechanisms that may also promote the restoration of mucosal barrier function through decreased inflammatory cytokines production (Chang et al. Citation2014; Plöger et al. Citation2012; Zheng et al. Citation2017). Moreover, enhanced expression of IL-10 can be associated with the presence of macrophages, which are known to be major producers of the anti-inflammatory cytokine IL-10 (e.g. in the intestine) (Morhardt et al. Citation2019). Studies in which the inflammatory process was monitored by measuring the mRNA and protein level of IL-10 in serum show that an increased IL-10 mRNA level corresponds to an increased protein level of IL-10 (Amoras et al. Citation2020; Ndishimye et al. Citation2015; Sapan et al. Citation2017).
This study confirmed our previously published data that increased expression of IL-10 can correlate with enhanced infiltration with macrophages during healing, which was also confirmed by immunofluorescence examination (Konop et al. Citation2021; Konop, Rybka, and Drapała Citation2021). The effect of sodium butyrate on piroxicam-induced gastric ulceration in cholestatic rats was evaluated, demonstrating that Na-Bu effectively protected gastric and hepatic tissues from cholestasis-induced damage and increased production of IL-10 (Elnozahi et al. Citation2020).
Tumor necrosis factor (TNF)-α is a quickly released cytokine that initiates inflammation in wounds. We showed that keratin-butyrate treatment enhanced mRNA expression of the TNF-α in the dressed part at the beginning and slowly decreased during recovery (). Its precise role in wound healing is not yet fully understood, however, TNF-α is closely related to the very early process of skin wound healing with upregulation of TNF-α synthesis within a few hours after injury, and then successive decrease (Ashcroft et al. Citation2013; Ritsu et al. Citation2017; Rodero and Khosrotehrani Citation2010).
The main angiogenic factor in the wound is Vascular Endothelial Growth Factor (VEGF) and its reduced expression results in impaired wound healing. The increased production of VEGF in the wound is described in migratory keratinocytes and macrophages infiltrating the granulation tissue (Brown et al. Citation1992; Frank et al. Citation1995; Rodero and Khosrotehrani Citation2010). Our study showed that keratin-butyrate treated wounds increased mRNA expression of VEGF was detected. These findings were also confirmed with immunofluorescence staining for VEGF, Flk-1 receptors, and macrophages (, Fig. S6 in supplementary materials). Such findings suggest a beneficial effect of examined dressing in angiogenic activity and are in agreement with the previous reports (Kim and Chuang Citation2014), which documented the up-regulation of VEGF expression in brain tissues following Na-Bu treatment. It was previously demonstrated that local treatment with a low dose (0.2 mM) of Na-Bu induced angiogenesis, matrix remodeling, and maturation during granulation tissue formation in an experimental model of sponge-induced subcutaneous fibrovascular tissue in mice (Castro et al. Citation2021).
Furthermore, we examined the impact of keratin butyrate scaffolds on the expression of selected cytokeratins (KRT1, KRT10, KRT16, and KRT17) and tight junction proteins at the mRNA level. All of them are involved in tissue regeneration and wound healing divided as KRT16/KRT17 and KRT10/KRT1 (Hobbs, Lessard, and Coulombe Citation2012; Konop et al. Citation2017; Konop, Rybka, and Drapała Citation2021). Keratin-butyrate treatment enhanced mRNA expression of KRT16 and KRT17 starting on the day 14 until the end of the experiment ( e–). This suggests that the tested dressing promoted wound healing. Epidermal injury leads to KRT16 and KRT17 induction, which occurs at the expense of KRT1/10 (Pauline and Coulombe Citation2003). Studies have shown that KRT17 affects protein growth and synthesis (Seyun, Wong, and Coulombe Citation2006). The epithelial cells at the edge of the wound rapidly induce KRT17, which is mediated at least in part via the modulation of tumor necrosis factor-α secretion (Pechter et al. Citation2012; Seyun, Wong, and Coulombe Citation2006). Expression of KRT16 and KRT17 persists through the epithelial remodeling phases until the barrier function is restored, suggesting that these keratins play an important physiological role during repair (Zhang, Yin, and Juan Zhang Citation2019). KRT1 is co-expressed with KRT10 in the suprabasal layer of the epidermis, and elevated expression of the KRT1/KRT10 complex indicates increased terminal differentiation of keratinocytes (Haake and Cooklis Citation1997). In our study, we did not observe increased expression of KRT1/KRT10, which may suggest that the process of keratinocyte differentiation was not fully activated. However, there are reports that KRT10 is up-regulated at post-wounding phase (Gangnuss et al. Citation2004). Since KRT10 is a marker for keratin differentiation, it suggests that wound re-epithelialization in both groups of our study had not been yet completed (Adamskaya et al. Citation2011).
Tight junction proteins (TJs) are intercellular connections composed of various transmembrane proteins including occludins, claudins, and junctional adhesion molecules (JAMs), as well as intracellular scaffolding proteins such as zonula occludens (ZOs) (Leonardo et al. Citation2020; Shi et al. Citation2018), which are important for the formation of an intercellular part of the skin (Volksdorf et al. Citation2017). Sodium butyrate was found to protect the intestinal barrier by regulating the expression of tight junction proteins (Ma et al. Citation2012; Wang and Wang Citation2012); however, there is a gap in the impact of butyrate on tight junction proteins during skin wound healing. Our study showed that wounds treated with keratin-butyrate dressing enhanced mRNA expression of Tjp1 (ZO1) and F11 r (JAM-A) proteins, which may suggest that promotion of wound healing and neovascularization may occur through the released butyrate from the applied dressing. These findings suggest that ZO proteins appear to participate in the regulation of cell growth and proliferation and support wound healing (). It was shown that butyrate treatment enhanced mRNA expression of the intestinal mucosal tight junction proteins occludin and zonula occludin protein-1 (P < .05), suggesting the promotion of wound healing by butyrate (Ma et al. Citation2012).
Figure 6. The expression of Tjp1 (a), and F11 r (b) mRNA levels in skin biopsies. c) the protein expression levels of AKT, p- AKT, RPS6, and mTOR were examined by Western blot analysis. The protein expression levels are displayed as the mean ± sem (n = 5). The data were only tested by the t-test for dependent samples (p-value <.05 was considered significant; *-p < .05, **-p < .01, ***-p < .001, mean ± standard error of the mean).
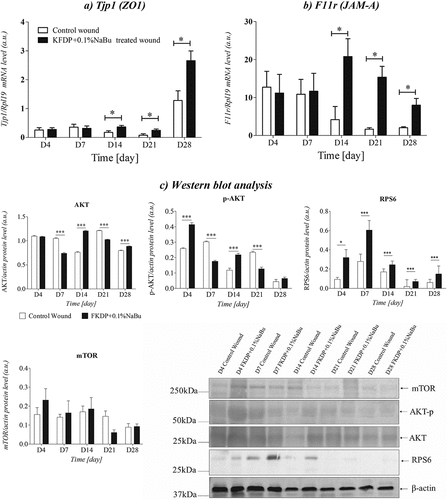
In addition, JAM-A up-regulation promoted both mesenchymal stem cells (MSC) homing to full-thickness skin wounds and wound healing-related cytokine secretion by MSCs (Feng et al. Citation2018). These effects promote angiogenesis at the wound surface and accelerated epithelialization, as represented by the increased thickness of the neoepidermis after wound healing and the formation of more skin appendage-like structures, thus improving the quality of wound healing. Moreover, JAM-A participates in angiogenesis by mediating the movement and migration of CD34+ hematopoietic progenitor cells to damaged vascular walls (Peddibhotla et al. Citation2013).
Western blot analysis was performed to detect the levels of phosphorylated transcription factors (p-AKT) and protein kinases (mTOR, AKT, RPS6) involved in intracellular signaling pathways in the wounded tissue to identify the signaling pathway involved in sodium butyrate action. In general, it has been reported that activation of the AKT/mTOR pathway can promote cell proliferation and migration, collagen synthesis, and angiogenesis. Butyrate might increase the levels of tight junction proteins by involving the activation of AKT/mTOR-mediated protein synthesis (Hui and Ajuwon Citation2017). In our study in keratin-butyrate treated wounds, ZO1 and JAM-A expression were increased. This finding provided solid evidence that AKT phosphorylation is essential for the sodium butyrate-mediated up-regulation of ZO1 and JAM-A expression in the wounded skin. It was shown that sodium butyrate promotes tight junction protein expression e.g. ZO1 in colon and Caco-2 cells in a GPR109A-dependent manner (Feng et al. Citation2018). A subsequent study showed that AKT/mTOR signaling pathway is activated in the wounded skin of both nondiabetic and diabetic rats, but in the wounded skin of diabetic rats, the activation is remarkably attenuated (Huang et al. Citation2015).
Ribosomal protein S6 (RPS6) is a key component of the translational machinery in eukaryotic cells and is essential for ribosome biogenesis. It plays an important role in ribosome biogenesis, protein translation, cell proliferation, cell growth, DNA repair, apoptosis, and cell differentiation (Ruvinsky and Meyuhas Citation2006; Salmond et al. Citation2009). In this study, we showed that in keratin-butyrate treated wounds RPS6 expression was elevated during recovery. RPS6 regulates pro-survival ribosomal mTORC2 signaling, which serves as a positive feedback loop in AKT activation (Yano et al. Citation2014). It is well known that RPS6 is rapidly phosphorylated in cells stimulated for growing or division. Furthermore, numerous studies have documented a strong correlation between increased S6 phosphorylation and protein synthesis, leading to the idea that S6 phosphorylation is involved in translation up-regulation. Therefore, it seems that RPS6 phosphorylation is a critical effector of mTOR activation of cell growth regulation (Baffi et al. Citation2021; Yang et al. Citation2015; Yano et al. Citation2014).
Conclusion
In summary, our study demonstrates that keratin butyrate dressing is safe and efficient in skin wound healing promotion in diabetic rat model. We have proven that butyrate was gradually released from the dressing and presented anti-inflammatory properties. RT-qPCR analysis showed that keratin-butyrate treated wounds decreased mRNA expression of inflammatory IL-1 and increased mRNA expression of VEGF, TNF-α, and anti-inflammatory IL-10. Moreover, we found that keratin-butyrate treated wounds increased mRNA expression of KRT16, KRT17, which are involved in tissue regeneration. Additionally, keratin-butyrate wound dressing increased the levels of tight junction proteins: ZO1 and JAM-A through the activation of AKT/mTOR-mediated protein synthesis. Furthermore, applied dressing stimulated macrophage infiltration, which favors tissue remodeling and regeneration without signs of aggravated inflammatory response. However, future studies with insoluble keratin scaffolds are needed to explore the molecular mechanism of the AKT/mTOR pathway involved in skin regeneration induced by keratin-based dressings.
Author contributions
MK – investigation, data curation, formal analysis, funding acquisition, writing – original draft, and writing – review & editing, JC, AKL, MS, ŁM, MR, DS, BC, ES, JF, PK, AW – investigation, formal analysis, writing – original draft, ZR, AW, JC – formal analysis, writing – original draft, RAS – supervision, writing – original draft, JC, MK, ZR, RAS – supervision, formal analysis, writing – original draft, and writing – review & editing.
Ethical approval
We confirm that all the research meets ethical guidelines and adheres to the legal requirements of the study country. The experiments were conducted only on rats, and National Ethics Committee approval was obtained for the experiment. Certificate of approval no.13/2021
Supplemental Material
Download MS Word (10 MB)Acknowledgments
We thank Agnieszka Bujanowska of the Department of Dermatology, Medical University of Warsaw, Poland, for her assistance in the preparation of the histological slides.
Disclosure statement
No potential conflict of interest was reported by the author(s).
Data availability statement
The data that support the findings of this study are available on request from the corresponding author (MK).
Supplementary material
Supplemental data for this article can be accessed online at https://doi.org/10.1080/15440478.2022.2136325.
Additional information
Funding
References
- Adamskaya, N., P. Dungel, R. Mittermayr, J. Hartinger, G. Feichtinger, K. Wassermann, H. Redl, and M. van Griensven. 2011. Light therapy by blue LED improves wound healing in an excision model in rats. Injury 42 (9):917–16. doi:10.1016/j.injury.2010.03.023.
- Amoras, E. D. S. G., S. T. Monteiro Gomes, M. A. Freitas Queiroz, M. S. M. de Araújo, M. T. F. de Araújo, S. R. S. da Silva Conde, R. Ishak, and A. C. R. Vallinoto. 2020. Intrahepatic interleukin 10 expression modulates fibrinogenesis during chronic HCV infection. PLoS ONE 15 (10):e0241199. doi:10.1371/journal.pone.0241199.
- Ashcroft, G. S., Jeong, M. J., Ashworth, J. J., Hardman, M., Jin, W., Moutsopoulos, N., Wild, T., McCartney-Francis, N., Sim, D., McGrady, G., Song, X. Y., & Wahl, S. M. 2013. TNFα is a therapeutic target for impaired cutaneous wound healing. Wound Repair and Regeneration : Official Publication of the Wound Healing Society [And] the European Tissue Repair Society 20 (1):38–49. doi:10.1111/j.1524-475X.2011.00748.x.
- Baffi, T. R., G. Lordén, J. M. Wozniak, A. Feichtner, W. Yeung, A. P. Kornev, C. C. King, J. C. Del Rio, A. J. Limaye, J. Bogomolovas, et al. 2021. mTORC2 controls the activity of PKC and Akt by phosphorylating a conserved TOR interaction motif. Science Signaling 14 (678):eabe4509. doi:10.1126/scisignal.abe4509.
- Bochynska-Czyz, M., P. Redkiewicz, H. Kozlowska, J. Matalinska, M. Konop, and P. Kosson. 2020. Can keratin scaffolds be used for creating three-dimensional cell cultures? Open Medicine 15 (1):249–53. doi:10.1515/med-2020-0031.
- Brown, L. F., K. T. Yeo, B. Berse, T. K. Yeo, D. R. Senger, H. F. Dvorak, and L. van de Water. 1992. Expression of vascular permeability factor (vascular endothelial growth factor) by epidermal keratinocytes during wound healing. The Journal of Experimental Medicine 176 (5):1375–79. doi:10.1084/jem.176.5.1375.
- Castro, P. R., L. F. F. Bittencourt, S. Larochelle, S. P. Andrade, C. R. Mackay, M. Slevin, V. J. Moulin, and L. S. Barcelos. 2021. GPR43 regulates sodium butyrate-induced angiogenesis and matrix remodeling. American Journal of Physiology - Heart and Circulatory Physiology 320 (3):H1066–79. doi:10.1152/ajpheart.00515.2019.
- Chang, P. V., L. Hao, S. Offermanns, and R. Medzhitov. 2014. The microbial metabolite butyrate regulates intestinal macrophage function via histone deacetylase inhibition. Proceedings of the National Academy of Sciences of the United States of America 111 (6):2247–52. doi:10.1073/pnas.1322269111.
- Chen, G., X. Ran, B. Li, Y. Li, D. He, B. Huang, S. Fu, J. Liu, and W. Wang. 2018. Sodium butyrate inhibits inflammation and maintains epithelium barrier integrity in a TNBS-induced inflammatory bowel disease mice model. eBioMedicine 30:317–25. doi:10.1016/j.ebiom.2018.03.030.
- Dyson, J. E. D., J. Daniel, and C. R. Surrey. 1992. The effect of sodium butyrate on the growth characteristics of human cervix tumour cells. British Journal of Cancer 65 (6):803–08. doi:10.1038/bjc.1992.172.
- Elnozahi, N. A., E. A. Said, A. E. Bistawroos, and R. G. Aly. 2020. Effect of sodium butyrate on gastric ulcer aggravation and hepatic injury inflicted by bile duct ligation in rats. Saudi Pharmaceutical Journal 28 (6):675–82. doi:10.1016/j.jsps.2020.04.008.
- Feng, W., Y. Wu, G. Chen, S. Fu, B. Li, B. Huang, D. Wang, W. Wang, and J. Liu. 2018. Sodium butyrate attenuates diarrhea in weaned piglets and promotes tight junction protein expression in colon in a GPR109A-dependent manner. Cellular Physiology and Biochemistry 47 (4):1617–29. doi:10.1159/000490981.
- Feroz, S., N. Muhammad, J. Ranayake, and G. Dias. 2020. Keratin - based materials for biomedical applications. Bioactive Materials 5 (3):496–509. doi:10.1016/j.bioactmat.2020.04.007.
- Frank, S., G. Hübner, G. Breier, M. T. Longaker, D. G. Greenhalgh, and S. Werner. 1995. Regulation of vascular endothelial growth factor expression in cultured keratinocytes. Implications for normal and impaired wound healing. The Journal of Biological Chemistry 270 (21):12607–13. doi:10.1074/jbc.270.21.12607.
- Gangnuss, S., A. J. Cowin, I. S. Daehn, N. Hatzirodos, J. A. Rothnagel, A. Varelias, and T. E. Rayner. 2004. Regulation of MAPK activation, AP-1 transcription factor expression and keratinocyte differentiation in wounded fetal skin. The Journal of Investigative Dermatology 122 (3):791–804. doi:10.1111/j.0022-202X.2004.22319.x.
- Guilloteau, P., G. Savary, Y. Jaguelin-Peyrault, V. Romé, L. Le Normand, and R. Zabielski. 2010. Dietary sodium butyrate supplementation increases digestibility and pancreatic secretion in young milk-fed calves. Journal of Dairy Science 93 (12):5842–50. doi:10.3168/jds.2009-2751.
- Haake, A. R., and M. Cooklis. 1997. Incomplete differentiation of fetal keratinocytes in the skin equivalent leads to the default pathway of apoptosis. Experimental Cell Research 231 (1):83–85. doi:10.1006/excr.1996.3441.
- Hobbs, R. P., J. C. Lessard, and P. A. Coulombe. 2012. Keratin intermediate filament proteins - novel regulators of inflammation and immunity in skin. Journal of Cell Science 125 (22):5257–58. doi:10.1242/jcs.122929.
- Huang, H., W. Cui, W. Qiu, M. Zhu, R. Zhao, D. Zeng, C. Dong, X. Wang, W. Guo, W. Xing, et al. 2015. Impaired wound healing results from the dysfunction of the Akt/MTOR pathway in diabetic rats. Journal of Dermatological Science 79 (3):241–51. doi:10.1016/j.jdermsci.2015.06.002.
- Hui, Y., and K. M. Ajuwon. 2017. Butyrate modifies intestinal barrier function in IPEC-J2 cells through a selective upregulation of tight junction proteins and activation of the Akt signaling pathway. PLoS ONE 12 (6):20179586. doi:10.1371/journal.pone.0179586.
- Keshava, R., and R. Gope. 2015. Sodium butyrate plus EGF and PDGF-BB aids cutaneous wound healing in diabetic mice. Advances in Biology 2015:1–10. ID 527231. doi:10.1155/2015/527231.
- Kim, H. J., and D. M. Chuang. 2014. HDAC inhibitors mitigate ischemia-induced oligodendrocyte damage: Potential roles of oligodendrogenesis, VEGF, and anti-inflammation. American Journal of Translational Research 6 (3):206–23.
- Konop, M., J. Czuwara, E. Kłodzińska, A. K. Laskowska, D. Sulejczak, T. Damps, U. Zielenkiewicz, I. Brzozowska, A. Sureda, T. Kowalkowski, et al. 2020. Evaluation of keratin biomaterial containing silver nanoparticles as a potential wound dressing in full-thickness skin wound model in diabetic mice. Journal of Tissue Engineering and Regenerative Medicine 14 (2):334–46. doi:10.1002/term.2998.
- Konop, M., J. Czuwara, E. Kłodzińska, A. K. Laskowska, U. Zielenkiewicz, I. Brzozowska, S. M. Nabavi, and L. Rudnicka. 2018. Development of a novel keratin dressing which accelerates full-thickness skin wound healing in diabetic mice: In vitro and in vivo studies. Journal of Biomaterials Applications 33 (4):527–40. doi:10.1177/0885328218801114.
- Konop, M., A. K. Laskowska, M. Rybka, E. Kłodzińska, D. Sulejczak, R. A. Schwartz, and J. Czuwara. 2021. Keratin scaffolds containing casomorphin stimulate macrophage infiltration and accelerate full-thickness cutaneous wound healing in diabetic mice. Molecules 26 (9):2554. doi:10.3390/molecules26092554.
- Konop, M., M. Rybka, and A. Drapała. 2021. Keratin biomaterials in skin wound healing, an old player in modern medicine: A mini review. Pharmaceutics 13 (12):2029. doi:10.3390/pharmaceutics13122029.
- Konop, M., D. Sulejczak, J. Czuwara, P. Kosson, A. Misicka, A. W. Lipkowski, and L. Rudnicka. 2017. The role of allogenic keratin-derived dressing in wound healing in a mouse model. Wound Repair and Regeneration 25 (1):62–74. doi:10.1111/wrr.12500.
- Lahiri, D., M. Nag, B. Dutta, A. Dey, T. Sarkar, S. Pati, H. A. Edinur, Z. Abdul Kari, N. H. Mohd Noor, and R. R. Ray. 2021. Bacterial cellulose: Production, characterization, and application as antimicrobial agent. International Journal of Molecular Sciences 22 (23):12984. doi:10.3390/ijms222312984.
- Lee, C., B. G. Kim, J. H. Kim, J. Chun, J. P. Im, and J. S. Kim. 2017. Sodium butyrate inhibits the NF-Kappa B signaling pathway and histone deacetylation, and attenuates experimental colitis in an IL-10 independent manner. International Immunopharmacology 51:47–56. doi:10.1016/j.intimp.2017.07.023.
- Leonardo, T. R., J. Shi, D. Chen, H. M. Trivedi, and L. Chen. 2020. Differential expression and function of bicellular tight junctions in skin and oral wound healing. International Journal of Molecular Sciences 21 (8):2966. doi:10.3390/ijms21082966.
- Liu, H., J. Wang, T. He, S. Becker, G. Zhang, D. Li, and X. Ma. 2018. Butyrate: A double-edged sword for health? Advances in Nutrition 9 (1):21–29. doi:10.1093/advances/nmx009.
- Ma, X., P. X. Fan, L. S. Li, S. Y. Qiao, G. L. Zhang, and D. F. Li. 2012. Butyrate promotes the recovering of intestinal wound healing through its positive effect on the tight junctions. Journal of Animal Science 90 (Suppl 4):266–68. doi:10.2527/jas.50965.
- Mazzalupo, S., P. Wong, P. Martin, and P. A. Coulombe. 2003. Role for keratins 6 and 17 during wound closure in embryonic mouse skin. Developmental Dynamics 226 (2):356–65. doi:10.1002/dvdy.10245.
- Mazzarino, L., G. Loch-Neckel, L. dos Santos Bubniak, F. Ourique, I. Otsuka, S. Halila, R. Curi Pedrosa, M. C. Santos-Silva, E. Lemos-Senna, E. Curti Muniz, et al. 2015. Nanoparticles made from xyloglucan-block-polycaprolactone copolymers: Safety assessment for drug delivery. Toxicological Sciences 147 (1):104–15. doi:10.1093/toxsci/kfv114.
- Moay, Z. K., L. T. H. Nguyen, P. Hartrianti, D. P. Lunny, D. Leavesley, Y. O. Kok, S. J. Chong, A. W. C. Chua, S. I. Tee, and K. W. Ng. 2021. Keratin-alginate sponges support healing of partial-thickness burns. International Journal of Molecular Sciences 22 (16):8594. doi:10.3390/ijms22168594.
- Morhardt, T. L., A. Hayashi, T. Ochi, M. Quirós, S. Kitamoto, H. Nagao-Kitamoto, P. Kuffa, K. Atarashi, K. Honda, J. Y. Kao, et al. 2019. IL-10 produced by macrophages regulates epithelial integrity in the small intestine. Scientific Reports 9 (1):1223. doi:10.1038/s41598-018-38125-x.
- Mowat, A. M., and W. W. Agace. 2014. Regional specialization within the intestinal immune system. Nature Reviews Immunology 14 (10):667–85. doi:10.1038/nri3738.
- Ndishimye, P., F. Seghrouchni, B. Domokos, O. Soritau, A. Sadak, D. Homorodean, R. E. Aouada, and C. M. Pop. 2015. Evaluation of interleukin-10 levels in the plasma of patients with various stages of tuberculosis. Medicine and Pharmacy Reports 88 (2):164–67. doi:10.15386/cjmed-459.
- Ohira, H., Y. Fujioka, C. Katagiri, M. Yano, R. Mamoto, M. Aoyama, M. Usami, and M. Ikeda. 2012. Butyrate enhancement of inteleukin-1β production via activation of oxidative stress pathways in lipopolysaccharide-stimulated THP-1 cells. Journal of Clinical Biochemistry and Nutrition 50 (1):59–66. doi:10.3164/jcbn.11-22.
- Onyszkiewicz, M., M. Gawrys-Kopczynska, P. Konopelski, M. Aleksandrowicz, A. Sawicka, E. Koźniewska, E. Samborowska, and M. Ufnal. 2019. Butyric acid, a gut bacteria metabolite, lowers arterial blood pressure via colon-vagus nerve signaling and GPR41/43 receptors. Pflügers Archiv - European Journal of Physiology 471 (11–12):1441–53. doi:10.1007/s00424-019-02322-y.
- Park, J.-S., E.-J. Lee, J.-C. Lee, W.-K. Kim, and H.-S. Kim. 2007. Anti-inflammatory effects of short chain fatty acids in IFN-γ-stimulated RAW 264.7 murine macrophage cells: Involvement of NF-ΚB and ERK signaling pathways. International Immunopharmacology 7 (1):70–77. doi:10.1016/j.intimp.2006.08.015.
- Park, M., H. K. Shin, B. S. Kim, M. J. Kim, I. S. Kim, B. Y. Park, and H. Y. Kim. 2015. Effect of discarded keratin-based biocomposite hydrogels on the wound healing process in vivo. Materials Science & Engineering: C, Materials for Biological Applications 55:88–94. doi:10.1016/j.msec.2015.03.033.
- Pauline, W., and P. A. Coulombe. 2003. Loss of Keratin 6 (K6) proteins reveals a function for intermediate filaments during wound repair. The Journal of Cell Biology 163 (2):327–37. doi:10.1083/jcb.200305032.
- Pechter, P. M., J. Gil, J. Valdes, M. Tomic-Canic, I. Pastar, O. Stojadinovic, R. S. Kirsner, and S. C. Davis. 2012. Keratin dressings speed epithelialization of deep partial-thickness wounds. Wound Repair and Regeneration 20 (2):236–42. doi:10.1111/j.1524-475X.2012.00768.x.
- Peddibhotla, S. S. D., B. F. Brinkmann, D. Kummer, H. Tuncay, M. Nakayama, R. H. Adams, V. Gerke, and K. Ebnet. 2013. Tetraspanin CD9 links junctional adhesion molecule-A to αvβ3 integrin to mediate basic fibroblast growth factor–specific angiogenic signaling. Molecular Biology of the Cell 24 (7):933–44. doi:10.1091/mbc.e12-06-0481.
- Plöger, S., F. Stumpff, G. B. Penner, J.-D. Schulzke, G. Gäbel, H. Martens, Z. Shen, D. Günzel, and J. R. Aschenbach. 2012. Microbial butyrate and its role for barrier function in the gastrointestinal tract. Annals of the New York Academy of Sciences 1258 (1):52–59. doi:10.1111/j.1749-6632.2012.06553.x.
- Qiu, Y., X. Ma, X. Yang, L. Wang, and Z. Jiang. 2017. Effect of sodium butyrate on cell proliferation and cell cycle in porcine intestinal epithelial (IPEC-J2) cells. Vitro Cellular and Developmental Biology - Animal 53 (4):304–11. doi:10.1007/s11626-016-0119-9.
- Ritsu, M., Kawakami K, Kanno E, Tanno H, Ishii K, Imai Y, Maruyama R, Tachi M. 2017. Critical role of tumor necrosis factor-α in the early process of wound healing in skin. Journal of Dermatology & Dermatologic Surgery 21 (1)14–19.
- Rodero, M. P., and K. Khosrotehrani. 2010. Skin wound healing modulation by macrophages. International Journal of Clinical and Experimental Pathology 3 (7):643–53.
- Ruvinsky, I., and O. Meyuhas. 2006. Ribosomal protein S6 phosphorylation: From protein synthesis to cell size. Trends in Biochemical Sciences 31 (6):342–48. doi:10.1016/j.tibs.2006.04.003.
- Salmond, R. J., J. Emery, K. Okkenhaug, and R. Zamoyska. 2009. MAPK, phosphatidylinositol 3-Kinase, and mammalian target of rapamycin pathways converge at the level of ribosomal protein S6 phosphorylation to control metabolic signaling in CD8 T cells. The Journal of Immunology 183 (11):7388–97. doi:10.4049/jimmunol.0902294.
- Sapan, H. B., I. Paturusi, A. A. Islam, I. Yusuf, I. Patellongi, M. N. Massi, A. D. Pusponegoro, S. K. Arief, I. Labeda, L. Rendy, et al. 2017. Interleukin-6 and interleukin-10 plasma levels and MRNA expression in polytrauma patients. Chinese Journal of Traumatology 20 (6):318–22. doi:10.1016/j.cjtee.2017.05.003.
- Seyun, K., P. Wong, and P. A. Coulombe. 2006. A keratin cytoskeletal protein regulates protein synthesis and epithelial cell growth. Nature 441 (7091):362–65. doi:10.1038/nature04659.
- Shi, J., M. Barakat, D. Chen, and L. Chen. 2018. Bicellular tight junctions and wound healing. International Journal of Molecular Sciences 19 (12):3862. doi:10.3390/ijms19123862.
- Spampinato, S. F., G. I. Caruso, R. De Pasquale, M. A. Sortino, and S. Merlo. 2020. The treatment of impaired wound healing in diabetes: Looking among old drugs. Pharmaceuticals 13 (4):60. doi:10.3390/ph13040060.
- Tachibana, A., Y. Furuta, H. Takeshima, T. Tanabe, and K. Yamauchi. 2002. Fabrication of wool keratin sponge scaffolds for long-term cell cultivation. Journal of Biotechnology 93 (2):165–70. doi:10.1016/S0168-1656(01)00395-9.
- Verma, V., P. Verma, P. Ray, and A. R. Ray. 2008. Preparation of scaffolds from human hair proteins for tissue-engineering applications. Biomedical Matererials 3 (2):025007. doi:10.1088/1748-6041/3/2/025007.
- Volksdorf, T., Heilmann J, Eming SA, Schawjinski K, Zorn-Kruppa M, Ueck C, Vidal-y-Sy S, Windhorst S, Jücker M, Moll I, et al. 2017. Tight junction proteins claudin-1 and occludin are important for cutaneous wound healing. The American Journal of Pathology 187 (6):1301–12. doi:10.1016/j.ajpath.2017.02.006.
- Wang, P.-Y., and P.-Y. Wang. 2012. Tu1343 butyrate enhances intestinal epithelial barrier function via up-regulation of tight junction protein claudin-1 transcription. Gastroenterology 142 (5): S-807. doi:10.1016/S0016-5085(12)63137-0.
- Wan, R., J. P. Weissman, K. Grundman, L. Lang, D. J. Grybowski, and R. D. Galiano. 2021. Diabetic wound healing: The impact of diabetes on myofibroblast activity and its potential therapeutic treatments. Wound Repair and Regeneration 29 (4):573–81. doi:10.1111/wrr.12954.
- Yang, X., Z. Jing, W. Wei, Z. Chun, Q. Quan, Z. Qing, X. Jiamin, and H. Shuwen. 2021. Inhibitory effect of sodium butyrate on colorectal cancer cells and construction of the related molecular network. BMC Cancer 21 (1):1. doi:10.1186/s12885-021-07845-1.
- Yang, G., D. S. Murashige, S. J. Humphrey, and D. E. James. 2015. A positive feedback loop between Akt and mTORC2 via SIN1 phosphorylation. Cell Reports 12 (6):937–43. doi:10.1016/j.celrep.2015.07.016.
- Yano, T., M. Ferlito, A. Aponte, A. Kuno, T. Miura, E. Murphy, and C. Steenbergen. 2014. Pivotal role of MTORC2 and involvement of ribosomal protein S6 in cardioprotective signaling. Circulation Research 114 (8):1268–80. doi:10.1161/CIRCRESAHA.114.303562.
- Zhang, X., M. Yin, and L. Juan Zhang. 2019. Keratin 6, 16 and 17-critical barrier alarmin molecules in skin wounds and psoriasis. Cells 8 (8):807. doi:10.3390/cells8080807.
- Zheng, L., C. J. Kelly, K. D. Battista, R. Schaefer, J. M. Lanis, E. E. Alexeev, R. X. Wang, J. C. Onyiah, D. J. Kominsky, and S. P. Colgan. 2017. Microbial-derived butyrate promotes epithelial barrier function through IL-10 receptor–dependent repression of Claudin-2. The Journal of Immunology 199 (8):2976–84. doi:10.4049/jimmunol.1700105.
- Zhong, Y., F. Seidi, C. Li, Z. Wan, Y. Jin, J. Song, and H. Xiao. 2021. Antimicrobial/Biocompatible hydrogels dual-reinforced by cellulose as ultrastretchable and rapid self-healing wound dressing. Biomacromolecules 22 (4):1654–63. doi:10.1021/acs.biomac.1c00086.