ABSTRACT
Polyoxometalate-based composite materials have recently attracted extensive attention due to their unique structural characteristics and physicochemical properties. In the present work, a flexible anti-UV blocker compatible with cotton fabric was prepared by simple covalent bond self-assembly of carboxyl group (−COOH) and phosphomolybdate. The resultant cotton fabrics were investigated and confirmed by FT-IR, PXRD and SEM-EDS, demonstrating the successful preparation of polyoxometalate-based composite fabric. The UV spectrum of as-designed composite fabric imparts excellent UV absorption capacity and the UPF value reaches 397.19, which is far superior to original cotton (7.09). Furthermore, the blocker was tested on the photosensitive material in direct UV irradiation, which proved its outstanding performance as a flexible, wearable, and visualized UV blocker. In addition, the experimental results confirmed that the prepared composite material exhibited an excellent and stable UV resistant performance (UPF: 100+) after 5 days UV aging irradiation and five times washing. The proposed fabrication strategy could promisingly endow polyoxometalate-based fiber materials with extensive applicability potential.
摘要
多金属氧酸盐基复合材料由于其独特的结构特点和物理化学性质,近年来受到广泛关注. 本文通过羧基(-COOH)和磷钼酸盐的简单共价键自组装,制备了一种与棉织物相容的柔性抗紫外线阻滞剂. 通过FT-IR、PXRD和SEM-EDS对所得棉织物进行了研究,证明了多金属氧酸盐基复合织物的成功制备. 设计的复合织物的紫外光谱赋予其优异的紫外线吸收能力,UPF值达到397.19,远远优于原棉(7.09). 此外,该阻滞剂在光敏材料上进行了直接紫外线照射试验,证明其作为柔韧、耐磨和可视化的紫外线阻滞剂具有优异的性能. 此外,实验结果证实,所制备的复合材料经过5天的紫外线老化辐照和5次洗涤后,表现出优异且稳定的抗紫外线性能(UPF:100+). 提出的制造策略有望赋予多金属氧酸盐基纤维材料广泛的应用潜力.
Introduction
Ultraviolet (UV) ray is the wavelength from sunlight ranging from 400 nm to 10 nm. According to the wavelength ranges, UV ray is comprised of UVA (400–320 nm), UVB (320–290 nm) and UVC (290–10 nm) (Zhang, Pi, and Guo Citation2011). Long-time exposure of the human skin to UV light usually leads to severe skin diseases and even cancer (Zou et al. Citation2020; Li et al. Citation2017; Xu et al. Citation2018). Generally speaking, UVA passing through the atmosphere directly could cause skin damage and aging, while UVB and UVC could be the main ringleader causing sunburn, skin tumors and even immunosuppression, which usually hard to arrive at the earth owing to the intercept by the ozone layer. However, with the wastage of the ozone layer and the increment of the ozone hole caused by the release of large amounts of nitrogen oxides, hydrochlorofluorocarbons and others, more and more UVB and UVC could reach to the ground (Magnaldo and Sarasin Citation2004; Matsumi and Kawasaki Citation2003). Therefore, UV shielding of all UVA, UVB and UVC become equally important. One economical and environment-friendly strategy could be the UV-resistant finishing of textiles.
Cotton fiber has the characteristics of excellent air permeability, soft feel, excellent hygroscopicity and biodegradability, so it is widely used in textiles and has become the fiber material with the largest consumption. However, the UV resistance of the conventional cotton fabric is inferior, resulting in unsuccessful protection of the human skin. At present, nanoparticles (such as TiO2 and ZnO) (Hu et al. Citation2022; Rashid et al. Citation2022; Salla, Pandey, and Srinivas Citation2012; Xu et al. Citation2018; Wang et al. Citation2018) and organic UV modifiers (Erdem, Cireli, and Erdogan Citation2009; Xu et al. Citation2018) are mainly used for the modification of textiles. In general, studies have shown that these provide UV protection via an absorption, reflection, refraction and scattering mechanism. However, these finishing agents possess poor adhesion, aggregation and unevenly distributed for the nanoparticles (Pospíšil and Nešpurek Citation2000), while for the organic compound, such as derivatives of benzophenone, benzotriazoles, and esters, are toxicity and lose efficiency, reducing the shielding effect and mechanical property (Qi et al. Citation2014). Additionally, the preparation of these protectors often involves many steps and even hazardous chemicals. Therefore, simple and efficient strategies to improve the UV resistance of cotton fiber are urgently needed.
Polyoxometalates (POMs) are a unique family of well-defined metal-oxo clusters composed of early transition metal ions in their highest oxidation state fused in the corner-, edge- or face-sharing fashions (Liu et al. Citation2016; Zheng and Yang Citation2012). Excellent electronic properties, redox performance, and unique nucleophilicity are used in coordination compounds, having a broad range of applications for catalysis, photoelectricity, and other fields (Cheng et al. Citation2022; Ong et al. Citation2022; Pérez-Álvarez et al. Citation2019; Stamate et al. Citation2020; Walsh et al. Citation2016; Wang and Yang, Citation2015). Up to now, the research of POMs has mainly focused on its intrinsic characteristics. Particularly, the developments of POMs and textile are limited. In addition, carboxylic acid ligands are easily covalently linked to POM units via p-block elements (mainly N or O atoms), enriching the structural diversities and many new properties (Ma et al. Citation2016). Hence, carboxyl groups can act as a “bridge” for POM and cotton fiber binding. Considering these aspects, we report the facile incorporation of the POM into cotton fiber in this present work by in-situ self-assembly method. The resulting fabric not only presents excellent UV blocking property in the whole UV region but also exhibits outstanding durability and stability. Meanwhile, the fabric has the advantages of flexibility, recyclability, as well as wearable. To the best of our knowledge, we report on the first fabric with UV blocking properties through the incorporation of POM-based nanomaterials. This research will open a new way to develop more effective and stable UV-protective textiles.
Materials and methods
Materials
The bleached cotton (plain cloth, 32S, 120 g/m2) was collected from Nantong Tianyi Textiles Printing and Dyeing Co., Ltd (Nantong, China). Ethanol, citric acid, sodium hypophosphate hydrate, (NH4)6Mo7O24·4 H2O and phosphoric acid were of analytical grade and purchased from Aladdin Reagent Co., Ltd.
Pretreatment of bleached cotton fabric (CF)
The bleached cotton fabric was trimmed into 5 cm × 5 cm squares without the edge burr. The fiber was transferred to the beaker containing ethanol and water using continuous ultrasonication for 30 min to remove the fine impurities attached to the fabric surface. After the treatment, the sample was dried at 60°C and sealed for use.
Preparation of carboxyl modified cotton fabric (CMCF)
The CMCF was prepared by a modified method according to the reported literature (Li, Dong, and Li Citation2015). Ten grams of citric acid and 5 g of sodium hypophosphate hydrate were dissolved in 85 mL of deionized water. The treated cotton fabric was added to the above solution and was soaked for 30 min and rolled at room temperature for 2 times, then dried in an oven at 80 ℃ for 3 min, and thermally treated at 180 ℃ for about 90 s, and cooled for later use.
Preparation of composite cotton fabrics (CCF)
1.06 g of (NH4)6Mo7O24·4 H2O and 80 mg of phosphoric acid was dissolved in 30 mL of deionized water. The pretreated cotton cloth (CMCF) was immersed in this aqueous solution and stirred at 70°C for 4 h. After the bath, the reaction mixture was allowed to stand for 24 h. Then, the cotton cloth was washed with deionized water and dried at 60°C for 10 min ().
Characterizations and instruments
The detection of functional groups on the surface of CF, CMCF, and CCF was performed using Fourier-transform infrared spectroscopy (FT-IR, Nicolet IS 10) in the range of 500–4000 cm‒1 for testing. The surface morphology of CF and CCF was observed by Scanning Electron Microscopy (SEM, using a Gemini 300, Germany). The composition of elements on the surface of the CCF sample was obtained by Energy Dispersive Spectrometer (EDS). The change of the UV absorption value of the CF, CMCF, and CCF was evaluated by the UV-Vis Spectrophotometer (UV-2600, SHIMADZU, Japan). PXRD was recorded on a D8 Advance diffractometer in the range of 5–45° with Cu Kα radiation (λ = 1.5405 Å) as the X-ray source and a scanning rate of 5°/min.
UV-Vis resistance performance test and evaluation
The CCF sample was put into a metal device to perform the UV resistance tests recorded on a testing machine (YG(B)912E, China). The wavelength of irradiation light was in the range of 200–400 nm. To investigate the durability and stability of UV resistant material, the fibers were put into a UV-accelerated aging box to perform the UV photoaging test (YG(B)982X), where the light intensity and environmental temperature, as well as irradiation distance, were set as 20 W, 25 ℃, 365 nm and 40 cm, respectively. The samples were taken out from the UV-accelerated aging box every 24 h in succession for 5 days. Finally, UV-resistant tester evaluated the anti-UV properties of various cotton fibers after different UV-accelerated aging times, respectively. In addition, the CCF sample was washed by using water five times, and the temperature was set to 40 ℃ for 30 min. After every washing, the anti-UV property was measured. Furthermore, we used UV light with a wavelength of 365 nm as external irradiation and photosensitive materials as the color agent to test the blocking performance of the composite fabric in this work.
Results and discussion
Optical and SEM images analysis
The field emission scanning electron microscopy (FE-SEM) equipped with energy-dispersive X-ray spectroscopy (EDS) was performed to reveal the surface morphology features and elementary compositions of various fabrics (). It can be seen that the CF is relatively clean and free of impurities due to the cleaning process performed in advance (). When it reacted with (NH4)6Mo7O24·4 H2O and H3PO4, the surface of CCF was no longer smooth, and there were some wrinkles on its surface locally. Besides, the surface of cotton fiber was covered with particles (Bao et al. Citation2020). This phenomenon was due to the various forces between fibers and components, covalent bond. Correspondingly, the optical photos of CF, CMCF, and CCF are presented in the . It was evident that there was not much difference in their appearance. This result showed that this preparation method does not change the external properties, such as the color of the original materials, which is essential for the further processing of fabrics. The layered deposits on the cotton fibers are barely visible to the naked eye, indicating the existence of coordination bond. In addition, EDS elemental mapping showed that Mo, P and O were uniformly distributed on the surface of CCF (), indicating that phosphomolybdate was successfully treated on the surface of the material.
Preparation test of composite fabric
The functional groups of various fabrics were recorded by the Fourier transform infrared (FT-IR) spectroscopy shown in (Figure S1, Table S1). For comparison, the FT-IR spectra of (NH4)6Mo7O24·4 H2O (), CF (), the CMCF () and the CCF () were measured. Compared with the FT-IR spectra of CF (Chung, Lee, and Choe Citation2004), two pronounced carbonyl peaks at 1748 and 1564 cm−1 appeared on that of CMCF, caused by the treatment with citric acid (Marković et al. Citation2018; Yan, Dong, and Bian Citation2022). When it comes to CCF, some new absorption peaks appeared. The peaks in the range of 560–724 cm−1 were attributed to ν(O-Mo-O), and the peaks at 947, 918 cm−1 were attributed to the stretching vibrations of ν(Mo=Ot) (Liang et al. Citation2015). In addition, there were two absorption peaks at 1019 and 1034 cm‒1, which were attributed to the stretching vibration of the P-O bond, indicating that the heteroatom P was coordinated with polyoxomolybdates. Meanwhile, compared with the (NH4)6Mo7O24·4 H2O (913 cm‒1, 716 cm‒1, 606 cm‒1 and 566 cm‒1), the appearance of new FT-IR peaks can confirm that the new polyoxomolybdates were successfully supported on cotton fabric . The FT-IR of CCF in fingerprint region was similar to carboxylate-functionalized {PMo6O27}-based heteropolymolybdates (Yang et al. Citation2013). Hence, we supposed that {PMo6O27} was formed by a self-assemble in-situ method and stabilized by citric acid on the cotton fiber. In order to study the crystallinity of particles deposited on the cotton samples, PXRD analysis was performed (Figure S2). Compared with the PXRD patterns of CF, typical peaks for the cellulose I crystalline form (Li et al. Citation2017) were observed at 2θ = 14.7°, 16.4°, 22.6°, and 34.3° in the PXRD patterns of CMCF and CCF fabrics suggesting that the cotton fibers have not been significantly damaged after the heating process for the coordination of POM and cotton fiber (Figure S2). In addition, some small peaks were assigned to POMs hiding in the pores of cotton fabric, although a washing process was applied several times.
Anti-UV property of the composite fabric
As presents, the prepared CCF was based on CMCF, and then impregnated with an aqueous solution of (NH4)6Mo7O24·4 H2O, stirred at 70°C for 4 h for further self-assembly. The CF is a natural cellulose fiber and its structure contains a large number of hydroxyl groups. Once modified by citric acid, the fabric possesses abundant carboxyl groups. Subsequently, the polyoxoanion {PMo6O27} formed in-situ associates with the carboxyl group owing to the strong coordination ability (Figure S3). In other words, the CMCF underwent coordination with {PMo6O27} generated by the self-assembly of (NH4)6Mo7O24·4 H2O and H3PO4. The results of above-mentioned EDS proved the existence of molybdate and phosphorus on the CCF and showed their homogeneous distribution. To verify the anti-UV properties of different cotton fibers, the optical absorption abilities of them in UV and visible light regions were determined by UV–Vis spectroscopy. The CF and CMCF exhibit tiny and weak absorption peak in the range of 200–300 nm (Afzal, Daoud, and Langford Citation2013). More interestingly, a significant enhancement toward the absorbance of composite fabric can also be witnessed in the wavelength range from 200 to 400 nm compared to the CF and CMCF, attributed to the electron transfer transition of oxygen to molybdenum (O→Mo) in the POM (Sun et al. Citation2017) (). The changes in the UV absorption of CCF indicate that the composite fabrics were successfully prepared following the preset route. As shown in , the transmittance of the composite fabric was lower than CF and CMCF, following the results of the UV–Vis investigation. In addition, the transmittance and calculated Ultraviolet Protection Factor (UPF) value of the composite fabric with different reaction time was performed, and the effect of UV-resistant performance (UPF: 397.19) was best when the reaction was 4 h (Table S2). The difference in the anti-ultraviolet performance can ascribe to different amount of POM. It was confirmed that the in-situ assembly of POM on fabric can provide adequate protection against UV irradiation. To investigate the aging resistance, CCF was exposed to the UV light irradiation ranging from 0 to 120 h with the sampling interval of 24 h. The UPF value (100+) was constantly and consistently higher than that of pristine cotton fibers (7.09) (). Meanwhile, for textile-based wearable devices, washing fastness is also a necessary practical application performance index, which determines the device’s service life (Samanta, Agarwal, and Datta Citation2009). Here, the washing fastness tests were carried out to study the durability of the POM attached to cotton fabrics. As shown in , the UV resistance maintains at a high level with UPF 100+ after five washing cycles. In addition, UPF values of CCF seem little change after 3 to 5 days and 3 to 5 times washing. These results powerfully indicated that CCF has promisingly outstanding anti-UV properties. Moreover, the device’s service life has superior performance in prolonged exposure to UV light and washing fastness.
Figure 4. (a) the UV-Vis solid diffuse reflectance spectra of CF, CMCF and CCF; (b) the transmittance of different samples, including CF, CMCF and CCF; (c) the transmittance of CF, CCF after the UV light irradiation process ranging from 0 to 120 h; (d) UPF value of CF and CCF with different UV light irradiation time; (e) UPF value of CF and CCF with different washing time; (f) the transmittance of CF, CCF after five times washing; (g) the color change of the photosensitive material in the present of CCF.
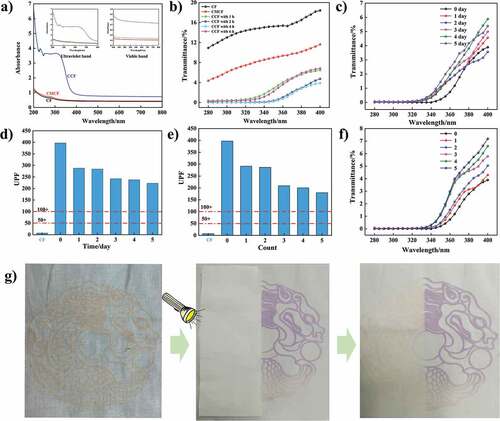
To investigate the UV resistance more clearly, we carried out the experiment with the help of a light-sensitive material reported by Fu et al. (Yang, Li, and Fu Citation2021). As is shown, the composite fabric was placed over one half of the photosensitive material and the other half was exposed (). When the UV irradiation was applied (λ = 365 nm), the color of one half of the light-sensitive material changed rapidly from the original pale-yellow state to dark violet color. In contrast, the remaining covered with composite fabric remained unchanged. The color change of the photosensitive material in the optical picture showed the excellent UV absorbance ability of the composite fabric, revealing its application prospect of UV resistance.
In addition, the increase in UPF values of fabric can be attributed to the intrinsic UV absorption properties of POM, which can be explained by the band theory. When POM is activated with light energy greater than its bandgap, the electrons will absorb UV light; we speculate that is why POM can protect against ultraviolet radiation.
Conclusions
In the present work, the composite material constructing by the phosphomolybdic as a functional group and pristine cotton fiber as a base were successfully fabricated via an in-situ conventional aqueous solution treatment strategy. This is the first example of introducing phosphomolybdic into the cotton fiber via -COOH groups. The composite fabric was characterized by IR, SEM-EDS, PXRD, UV–Vis spectroscopy. Furthermore, the UPF value of composite fabric reaches 397.19, demonstrating excellent UV resistance. The color contrast of photosensitive fabrics also verifies this feature. Meanwhile, the UV resistance also maintains at a high level with UPF 100+ after 5 days aging and five time washing. Considering the diversities and versatilities of POMs, the proposed fabrication strategy could promisingly endow cotton fibers with great application potential in other fields such as fiber-based sensors.
Highlights
Cotton fibers and polyoxometalate were combined via carboxy group as a bridge.
The composite fabric sample exhibited excellent UV shielding effect.
The UPF value remained 100+ after washing and long-term UV exposure.
Disclosure statement
No potential conflict of interest was reported by the author(s).
Additional information
Funding
References
- Afzal, S., W. A. Daoud, and S. J. Langford. 2013. Visible-light self-cleaning cotton by metalloporphyrin-sensitized photocatalysis. Applied Surface Science 275:36–10. doi:10.1016/j.apsusc.2013.01.141.
- Bao, B., J. Fan, Z. Wang, Y. Wang, W. Wang, X. Qin, and D. Yu. 2020. Sodium deca-tungstate/polyacrylic acid self-assembled flexible wearable photochromic composite fabric for solar UV detector. Composites Part B 202:108464. doi:10.1016/j.compositesb.2020.108464.
- Cheng, M., Y. Liu, W. Du, J. Shi, J. Li, H. Wang, K. Li, G. Yang, and D. Zhang. 2022. Two Dawson-type U(VI)-containing selenotungstates with sandwich structure and its high-efficiency catalysis for pyrazoles. Chinese Chemical Letters 33 (8):3899–902. doi:10.1016/j.cclet.2021.11.059.
- Chung, C., M. Lee, and E. K. Choe. 2004. Characterization of cotton fabric scouring by FT-IR ATR spectroscopy. Carbohydrate Polymers 58 (4):417–20. doi:10.1016/j.carbpol.2004.08.005.
- Erdem, N., A. A. Cireli, and U. H. Erdogan. 2009. Flame retardancy behaviors and structural properties of polypropylene/nano-SiO2 composite textile filaments. Journal of Applied Polymer Science 111 (4):2085–91. doi:10.1002/app.29052.
- Hu, Q., W. Wang, T. Ma, C. Zhang, J. Kuang, and R. Wang. 2022. Anti-UV and hydrophobic dual-functional coating fabrication for flame retardant polyester fabrics by surface-initiated PET RAFT technique. European Polymer Journal 173:111275. doi:10.1016/j.eurpolymj.2022.111275.
- Liang, Y., S. Li, D. Yang, P. Ma, J. Niu, and J. Wang. 2015. Controllable assembly of multicarboxylic acids functionalized heteropolyoxomolybdates and allochroic properties. Journal of Materials Chemistry C 3 (18):4632. doi:10.1039/C5TC00297D.
- Li, B., Y. Dong, and L. Li. 2015. Preparation and catalytic performance of Fe(III)-citric acid-modified cotton fiber complex as a novel cellulose fiber-supported heterogeneous photo-Fenton catalyst. Cellulose 22 (2):1295–309. doi:10.1007/s10570-015-0562-x.
- Li, Z., J. Meng, W. Wang, Z. Wang, M. Li, T. Chen, and C.-J. Liu. 2017. The room temperature electron reduction for the preparation of silver nanoparticles on cotton with high antimicrobial activity. Carbohydrate Polymers 161:270–76. doi:10.1016/j.carbpol.2017.01.020.
- Liu, Z.-J., X.-L. Wang, C. Qin, Z.-M. Zhang, Y.-G. Li, W.-L. Chen, and E.-B. Wang. 2016. Polyoxometalate-assisted synthesis of transition-metal cubane clusters as artificial mimics of the oxygen-evolving center of photosystem II. Coordination Chemistry Reviews 313:94–110. doi:10.1016/j.ccr.2015.12.006.
- Magnaldo, T., and A. Sarasin. 2004. Xeroderma pigmentosum: From symptoms and genetics to gene-based skin therapy. Cells, Tissues, Organs 177 (3):189–98. doi:10.1159/000079993.
- Ma, P., F. Hu, J. Wang, and J. Niu. 2016. Carboxylate covalently modified polyoxometalates: From synthesis, structural diversity to applications. Coordination Chemistry Reviews 306:217–34. doi:10.1016/j.ccr.2018.02.010.
- Marković, D., C. Deeks, T. Nunney, Ž. Radovanović, M. Radoičić, Z. Šaponjić, and M. Radetić. 2018. Antibacterial activity of Cu-based nanoparticles synthesized on the cotton fabrics modified with polycarboxylic acids. Carbohydrate Polymers 200:173–82. doi:10.1016/j.carbpol.2018.08.001.
- Matsumi, Y., and M. Kawasaki. 2003. Photolysis of atmospheric ozone in the ultraviolet region. Chemical Reviews 103 (12):4767–82. doi:10.1021/cr0205255.
- Ong, B. C., H. K. Lim, C. Y. Tay, T.-T. Lim, and Z. L. Dong. 2022. Polyoxometalates for bifunctional applications: Catalytic dye degradation and anticancer activity. Chemosphere 286:131869. doi:10.1016/j.chemosphere.2021.131869.
- Pérez-Álvarez, L., L. Ruiz-Rubio, B. Artetxe, M. M. Vivanco, J. M. Gutiérrez-Zorrilla, and J. L. Vilas-Vilela. 2019. Chitosan nanogels as nanocarriers of polyoxometalates for breast cancer therapies. Carbohydrate Polymers 213:159–67. doi:10.1016/j.carbpol.2019.02.091.
- Pospíšil, J., and S. Nešpurek. 2000. Photostabilization of coatings. Mechanisms and performance. Progress in Polymer Science 25 (9):1261–335. doi:10.1016/S0079-6700(00)00029-0.
- Qi, L., Y. Ding, Q. Dong, B. Wen, F. Wang, S. Zhang, and M. Yang. 2014. Photostabilization of polypropylene by surface modified rutile-type TiO2 nanorods. Journal of Applied Polymer Science 131 (16):16. doi:10.1002/app.40601.
- Rashid, M. M., B. Tomšič, B. Simončič, I. Jerman, D. Štular, and M. Zorc. 2022. Sustainable and cost-effective functionalization of textile surfaces with Ag-doped TiO2/polysiloxane hybrid nanocomposite for UV protection, antibacterial and self-cleaning properties. Applied Surface Science 595:153521. doi:10.1016/j.apsusc.2022.153521.
- Salla, J., K. K. Pandey, and K. Srinivas. 2012. Improvement of UV resistance of wood surfaces by using ZnO nanoparticles. Polymer Degradation and Stability 97 (4):592–96. doi:10.1016/j.polymdegradstab.2012.01.013.
- Samanta, A. K., P. Agarwal, and S. Datta. 2009. Studies on Color Interaction Parameters and Color Fastness Properties for Dyeing of Cotton Fabrics with Binary Mixtures of Jackfruit Wood and other Natural Dyes. Journal of Natural Fibers 6 (2):171–90. doi:10.1080/15440470902958124.
- Stamate, A.-E., O. D. Pavel, R. Zavoianu, and I.-C. Marcu. 2020. Highlights on the Catalytic Properties of Polyoxometalate-Intercalated Layered Double Hydroxides: A Review. Catalysts 10 (1):57. doi:10.3390/catal10010057.
- Sun, X., R. Ban, P. Ma, J. Wang, D. Zhang, J. Niu, and J. Wang. 2017. Four transition-metal-bridging risedronate-based polyoxomolybdates: Syntheses, structures, characterizations and magnetic properties. Synthetic Metals 223:19–25. doi:10.1016/j.synthmet.2016.11.035.
- Walsh, J. J., A. M. Bond, R. J. Forster, and T. E. Keyes. 2016. Hybrid polyoxometalate materials for photo(electro-) chemical applications. Coordination Chemistry Reviews 306:217–34. doi:10.1016/j.ccr.2015.06.016.
- Wang, J., A. S. Jeevarathinam, A. Jhunjhunwala, H. Ren, J. Lemaster, Y. Luo, D. P. Fenning, E. E. Fullerton, and J. V. Jokerst. 2018. A Wearable Colorimetric Dosimeter to Monitor Sunlight Exposure. Advanced Materials Technologies 3 (6):1800037. doi:10.1002/admt.201800037.
- Wang, S.-S., and G.-Y. Yang. 2015. Recent Advances in Polyoxometalate-Catalyzed Reactions. Chemical Reviews 115 (11):4893–962. doi:10.1021/cr500390v.
- Xu, X., J. Chen, S. Cai, Z. Long, Y. Zhang, L. Su, S. He, C. Tang, P. Liu, H. Peng, et al. 2018. A real-time wearable UV-radiation monitor based on a high-performance p-CuZnS/n-TiO2 photodetector. Advanced Materials. 30 (43):1803165. doi:10.1002/adma.201803165.
- Xu, S., L. Dang, J. Yu, L. Xue, C. Hu, and Y. Que. 2018. Evaluation of ultraviolet aging resistance of bitumen containing different organic layered double hydroxides. Construction and Building Materials 192:696–703. doi:10.1016/j.conbuildmat.2018.10.172.
- Yan, Y., Y. Dong, and L. Bian. 2022. Surface functionalization of cotton fabric with Ag3PO4 via citric acid modification using paddrycure process for enhancing selfcleaning performance. Cellulose 29 (7):4203–27. doi:10.1007/s10570-022-04507-8.
- Yang, Y., M. Li, and S. Fu. 2021. Screen-printed photochromic textiles with high fastness prepared by self-adhesive polymer latex particles. Progress in Organic Coatings 158:106348. doi:10.1016/j.porgcoat.2021.106348.
- Yang, D., S. Li, P. Ma, J. Wang, and J. Niu. 2013. Carboxylate-Functionalized Phosphomolybdates: Ligand-Directed Conformations. Inorganic Chemistry 52 (15):8987–92. doi:10.1021/ic401176j.
- Zhang, X., H. Pi, and S. Guo. 2011. The mechanism for inorganic fillers accelerating and inhibiting the UV irradiation aging behaviors of rigid poly (vinyl chloride). Journal of Applied Polymer Science 122 (5):2869–75. doi:10.1002/app.34396.
- Zheng, S.-T., and G.-Y. Yang. 2012. Recent advances in paramagnetic-TM-substituted polyoxometalates (TM = Mn, Fe, Co, Ni, Cu). Chemical Society Reviews 41 (22):7623–46. doi:10.1039/C2CS35133A.
- Zou, W., M. Sastry, J. J. Gooding, R. Ramanathan, and V. Bansal. 2020. Recent advances and a roadmap to wearable UV sensor technologies. Advanced Materials Technologies 5 (4):1901036. doi:10.1002/admt.201901036.