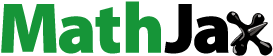
ABSTRACT
Barium Titanate (BaTiO3) nanoparticles were mixed with a stock paste containing an acrylate-based binder to prepare pigment paste. The formulated paste was applied to the cotton fabric by utilizing screen printing followed by drying and curing. The coated fabric was characterized for surface and elemental composition and uniform deposition of BaTiO3 nanoparticles was observed with minimal agglomeration. The resultant fabric showed an effective bacterial reduction greater than 99% against Escherichia coli and Staphylococcus aureus, and after 15 laundering cycles, the modified fabric showed a bacterial reduction of over 98%. The loaded fabric is capable of blocking harmful ultraviolet (UV) radiations. The modification of the cotton surface did not significantly change its intrinsic properties like water vapor permeability, air permeability, fabric stiffness, and other mechanical properties. The developed product has considerable possibilities and provides a good substitute for antibiotic materials for use in medical products and everyday apparel.
摘要
将钛酸钡(BaTiO3)纳米颗粒与含有丙烯酸酯基粘合剂的浆料混合以制备颜料浆料. 通过丝网印刷,然后干燥和固化,将配制的糊剂应用到棉织物上. 对涂层织物的表面和元素组成进行了表征,并观察到BaTiO3纳米颗粒的均匀沉积. 所得织物对大肠杆菌和金黄色葡萄球菌的有效细菌减少率大于99%,经过15次洗涤循环后,改性织物的细菌减少率超过98%. 负载织物能够阻挡有害的紫外线(UV)辐射。棉花表面的改性并没有显著改变其固有性能,如水蒸气渗透性、透气性、织物硬度和其他机械性能. 开发的产品具有相当大的可能性,为医疗产品和日常服装中使用的抗生素材料提供了良好的替代品.
Introduction
Cotton fabrics are a popular choice in our society. In addition to apparel and home textile, cotton is widely used in the manufacturing of sportswear, health care, and medical textile including surgical, sanitary, and hygiene products (Elhalawany et al. Citation2020; Liu et al. Citation2019; Montaser et al. Citation2020; Nhlapo et al. Citation2019; Sela et al. Citation2020; Wong, Li, and Yeung Citation2005). At present, many researchers have reported their studies to enhance the functionality of cotton including wrinkle-free, fire resistance, photo-yellowing, dye fixation, antibacterial, ultraviolet (UV) protection, and self-cleaning activity (Fu et al. Citation2021; Jabar, Adedayo, and Odusote Citation2021; Li et al. Citation2020; Qi et al. Citation2016).
Natural fibers, including both cellulose and protein-based, have higher moisture regain and porosity due to which these are easily attacked by microorganisms. This results in unpleasant odor, allergies, infections, and many other diseases (Dev et al. Citation2009; Ranjbar-Mohammadi Citation2018). In addition, the UV radiation that reaches the earth’s surface UVA (315–400 nm; 95%) and UVB (280–315 nm; 5%) are biologically active. They cause skin tanning and burning, wrinkling, and premature aging (photo-aging) and long exposure can result in skin cancers (Ferrara et al. Citation2021; Valacchi et al. Citation2012). Thus, taking into account consumer health and cleanliness, the antibacterial and UV-resistant treatment of the textile product has become a significant objective in the clothing industry.
Industrially, different chemical agents like quaternary ammonium salts, oxidizing agents, halogens, metal complexes, onium salts, antibiotics, and metals like silver (Ag), zinc (Zn), copper (Cu), titanium (Ti), and their derivatives are widely used to enhance antibacterial and UV protective functionality to textile, respectively (ElShafei and Okeil Citation2011; Gao et al. Citation2021; Kang et al. Citation2016). These properties can be imparted to cotton fabric by different approaches including coating, laminating, impregnation, soaking, adsorbing, grafting, or by melt, dry, or electrospinning techniques. Xu et al. (Citation2017) prepared bacterial-resistant cotton fabric by coating Ag nanoparticles (NPs) onto the fabric surface through carboxymethyl chitosan (CMC). Ibrahim & Hassan (Citation2016) soaked cotton in a colloidal solution of butyl acrylate binder and Ag-NPs and Zhang et al. (Citation2013) grafted cotton with amino-functional Ag-NPs. Cu-NPs synthesized antibacterial cotton was reported by Marković et al. (Citation2018) while ElShafei and Okeil (Citation2011) prepared suspension of ZnO and CMC composite. Qingbo Xu et al. (Citation2021) applied CMC & Ag/TiO2 colloid solution by pad-cure technique to develop antibacterial and UV protective cotton, while Kumar, Sharma, and Vaish (Citation2022a) spray-coated cotton fabric with molybdenum disulfide particles. All these findings demonstrated strong bacterial reduction against both gram-positive and gram-negative bacteria.
Barium Titanate (BaTiO3) being ferroelectric at room temperature possesses multifunctional properties such as self-cleaning or antimicrobial and UV protection. The titanium (Ti4+) and oxygen (O2-) ions shift relative to barium (Ba2+) and produce spontaneous polarization showing antimicrobial and antibiofilm activities against certain gram-positive and gram-negative bacteria (Acosta et al. Citation2017; Kumar et al. Citation2019; Raja et al. Citation2015). Also, BaTiO3 (BT) shows a UV absorption peak around 300–330 nm and, therefore, is highly efficient in blocking harmful UV radiations (Qin et al. Citation2010). Ferroelectric materials have been reported for their antibacterial properties using several effects, i.e., piezocatalysis, photocatalysis, pyrocatalysis, and ferrocatalysis. These are well documented in the recent literature (Kumar et al. Citation2021). Due to their multifunctional properties, these families of materials are a promising and popular choice for next-generation devices and gadgets. These materials are already in the electronic market for various sensors and actuator’s viewpoint. On the other hand, their uses for environmental applications have recently been explored using piezocatalysis and photocatalysis. Various polymer composites and ceramics are extensively researched; however, at the same time, their possible utilization in clothing has not been well studied. Incorporation of ferroelectric material on clothes/fabric/fiber may be useful for smart or functional applications. In order to make the fabric self-cleaned, TiO2 is a largely reported material, since TiO2 is a UV active photocatalytic material and can demonstrate antibacterial performance in the presence of solar light (Abualnaja et al. Citation2021).
In this study, the screen printing technique was utilized for coating BT NPs on the pristine cotton fabric surface. The prepared fabric samples were characterized by ATR-FTIR, XRD, XPS, FE-SEM, and UV spectrophotometer. The antibacterial durability along with water and air permeability, tensile strength, fabric stiffness, and abrasion resistance of the modified fabric was evaluated. It was found that BT NPs coated fabric offers exceptional protection against bacterial growth and UV rays.
Experimental
Materials
Nanoparticles of BaTiO3, urea, and liquid ammonia were supplied by Sigma Aldrich. One hundred percent pure gray cotton fabric (136 gsm) was received from Vardhman, Baddi. The Luprintol Soft F-SIG (silicone-based softener), Luprintol Antifoam TC (antifoaming agent), Helizarin (Hel) Binder ET ECO (thermally cross-linkable acrylate copolymer), Luprintol MCL (Silicone-containing emulsifier), thickener Lutexal F-HIT, and Luprintol WB-FR (fixing agent) were obtained from Archroma, India.
Preparation of the stock paste
Before screen printing, the stock paste preparation was done by together mixing liquid ammonia (1.2%), urea (2.8%), cross-linkable acrylate copolymer (22%), antifoaming agent TC (0.5%), silicone-containing emulsifier (0.5%), silicone-based softener (0.5%), and fixing agent (1.4%) and continuously stirring in water (67.5%) at room temperature for 10 min. The paste desired viscosity level was obtained by adding thickener (3.5%) to the resultant mixture. Then, the mixture was stirred continuously for 10 min to get a uniform stock paste. The fixing agent improved wet fastness while the defoamer reduced foam production during stock paste preparation and deaerated print pastes throughout the printing process, thus, reducing the formation of foam streaks. The thickener provided the print pastes with outstanding flowing qualities and gave the prints strong edges and luster.
Pigment paste preparation
For pigment paste preparation, BT NPs were added to the stock paste mixture in two varying concentrations of 10% and 20% and stirred to prepare a homogenous paste. The 10% BT pigment concentration entails mixing 9 g of stock paste with 1 g of BT, and the 20% signifies mixing 8 g of stock paste with 2 g of BT. Pigment paste concentrations of less than 10% were disregarded because it was impossible to achieve a uniform distribution on the cotton surface, and concentrations of more than 20% BT were neglected because the investigation goal was to obtain the best results at the lowest possible cost of BT.
Fabric coating process
The pristine cotton was initially washed with deionized water at room temperature to remove any impurity and then dried for 24 h. Pigment paste was applied uniformly onto the fabric surface through a screen having 165 mess size, followed by drying and curing at 120°C for 05 and 07 min, respectively. Additionally, the preparation of samples coated solely with stock paste was done, so that the impact of the binding agents on the antibacterial and UV protection qualities could be assessed. The softener imparted softness to a coated fabric. The use of softener in print paste strengthened the prints’ durability to dry rubbing and produced a pleasantly dry, soft, non-sticky handle with better luster.
Characterization
Attenuated total reflection-Fourier transform infrared (ATR-FTIR) spectroscopy
ATR-FTIR spectra of pure cotton, BT, and coated cotton were recorded to identify the various chemical groups introduced in the samples before and after coating. The sample measurements (50 scans/sample) were done on Spectrometer-2 (Perkin Elmer).
X-ray diffraction (XRD)
XRD analysis for pure cotton, BT, and coated cotton (before and after washing) were done on a powder X-ray diffractometer (Rigaku Corporation Smart Lab 9 kW) with 2θ scanned from 10° to 70°.
Field emission-scanning electron microscope (FE-SEM) with energy-dispersive X-ray spectrometer (EDS)
To observe the distribution of BT on the cotton surface, the samples before and after coating were analyzed using FE-SEM (JFEI SEM-450) with an energy-dispersive X-ray spectrometer (EDS). Before scanning, the samples were provided with gold spray.
X-ray photoelectron spectroscopy (XPS)
XPS analyses were carried out on an X-ray photoelectron spectrometer (Nexsa base, Thermofisher Scientific) to measure elemental composition as well as the chemical and electronic state of the atoms within the uncoated and coated cotton.
Antibacterial assessment
The gram-positive bacteria, Staphylococcus aureus (S. aureus), and gram-negative bacteria, Escherichia coli (E. coli) were used for the antibacterial activity test as per AATCC 100–2004 test method. By using the following equation, the bacterial reduction (R) percentage was determined:
where D and C, respectively, represent the control and BT NPs loaded samples bacteria colony forming units (CFU) (Kumar, Sharma, and Vaish Citation2022b).
Further, the antibacterial test was carried out for fabric obtained after 15 washing cycles.
Water vapor and air permeability, mechanical properties, and coating durability measurement
The fabric water vapor permeability, air permeability, tensile, and abrasion resistance measurements were performed as per ASTM E-96, ASTM D-737, ASTM D-5034, and ASTM D-4685 standards, respectively. The fabric stiffness was evaluated in terms of bending length and flexural rigidity. The coating’s endurance was measured using ISO 105-C10:2006.
Ultraviolet-visible (UV-VIS) spectroscopy
The UV spectrophotometer (Shimadzu UV-2600) was utilized to analyze the UV absorption spectra of the samples. The UV protection factor (UPF) was measured using a Perkin Elmer UV-vis Spectrophotometer as per Australian/New Zealand Test Standard AS/NZS 4399:1996.
Results and discussion
Characterization of pristine cotton and BT embedded fabric
ATR-FTIR analysis
depicts the ATR-FTIR of pure cotton, BT NPs, and BT-coated fabric. In pure cotton (), the broad band observed from 3272 to 3336 cm−1 refers to the hydroxyl (OH) group present in cotton and water. The C-H stretching vibration bands were observed at 2902 and 2851 cm−1. The band at 1634 cm−1 is attributed to the water molecule OH bending vibration present in cotton. The CH2 symmetric bending of cellulose was observed at 1425 cm−1. The C-H and C-O bending vibrations of the cellulose are related to the bands at 1364 and 1312 cm−1. A band at 1159 cm−1 is attributed to the C-O-C stretching, and 1028 cm−1 is assigned to the C-O stretching of C-6 in cellulose (Portella et al. Citation2016). In BT FTIR analysis (), a sharp prominent absorption band observed at 494 cm−1 and a weak band seen at 858 cm−1 can be attributed to Ti-O bond vibration (Kumar and Luthra Citation2021). In BT-NP-coated cotton fabric, the band appearing at 1737 cm−1 can be contributed to C=O stretching, while the C=C bond band was seen at 1657 cm−1 which relates to the acrylate-based binder. After coating, a band observed at 493 cm−1 on the cotton surface relates to Ti-O bond vibration. Thus, the presence of spectrum bands of acrylate-based binder and BT on the coated cotton confirms the successful embedding of BT NPs on the fabric surface.
XRD analysis
XRD patterns of the pristine cotton, pure BT, and BT coated sample (before and after laundering) are shown in . In pure cotton, the diffraction peaks observed at 2θ = 14.7°,16.72°, 22.79°, and 34.46° are attributed to the (110), (110), (200) and (004) crystallographic planes of the cellulose (Gaspar et al. Citation2014; Pandiyarasan et al. Citation2017). In pure BT, XRD peaks observed at 22.04° (100), 31.41° (110), 38.78° (111), 45.06° (200), 50.75° (210), 56.02° (211), and 65.69° (220) were assigned to tetragonal BT NPs (JCPDS card no. 31-0174) (Khan et al. Citation2021; Wang et al. Citation2013). After coating, the XRD peaks of cotton did not significantly shift. In the coated fabric, the diffraction peaks observed at 2θ = 31.5° (110), 38.88° (111), 45.27° (200), 50.90° (210), 56.21° (211), and 65.80° (220) correspond to BT NPs embedded on the cotton surface. The washed (15 times) BT coated cotton shows a similar diffraction pattern as observed for unwashed fabric, with reduced intensities of few peaks, which confirms that BT NPs remained adhered to the fabric surface and possess excellent durability.
FE-SEM and EDS analysis
shows the FE-SEM analysis carried out to examine the surface morphologies. The pure cotton fibers had a twisted ribbon-type appearance, while on applying the stock paste, the fibers were seen sticking together by some gummy-like substance. When pigment paste was applied, the uniform deposition of BT NPs was observed on the fabric surface for both 20% and 10% concentrations with minimal agglomeration. The coated particles were so firmly attached to the fabric surface by the acrylate binder that after 15 laundry cycles they continued to be retained inside the binder matrix.
Figure 3. SEM images of (a) Pure cotton fabric, (b) Only stock paste applied fabric, (c) Fabric coated with 20% BT, (d) Fabric coated with 10% BT, (e) Washed fabric (20%), (f) Washed fabric (10%), and (g) EDX Analysis of fabric coated with 20% BT.
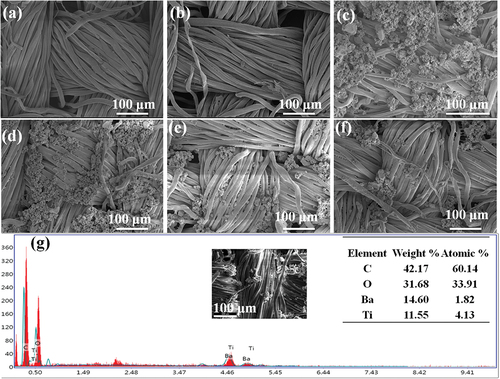
EDX analysis was performed to find out the surface elemental composition of coated cotton. In pristine cotton, only carbon and oxygen atoms were found (Supplementary Material Fig. S1); however, in coated fabric (), barium (Ba) and titanium (Ti) atoms were also observed with decreased carbon and oxygen content. This demonstrates that BT NPs were present in good concentration on cotton. Further, EDX mapping of coated fabric (Supplementary Material Fig. S2) showed a slight decrease in Ba and Ti content after 15 washing cycles with increased O and C atoms due to the formation of more hydrogen bonds on the cotton surface during washing. Therefore, both SEM and EDX results confirm that BT NPs were incorporated on the fabric surface.
XPS analysis
shows the chemical state of C, O, Ba, and Ti evaluated in pure cotton and coated fabric by XPS. Both spectra showed two intense signals of C 1s and O 1s at 285 and 532 eV, which correspond to the binding energy of the C–C and C–H bonds and cellulose, respectively (Belgacem et al. Citation1995; Bhat et al. Citation2011; Kang et al. Citation2017). In coated fabric, Ba 4d peaks were observed at 88.9 and 90.7 eV, corresponding to 5/2 and 3/2 spin-orbit components, respectively, while Ti 2p peak was observed at 458 eV (Khan et al. Citation2021; Wegmann, Watson, and Hendry Citation2004).
Antibacterial activity assessment
presents a study of the bacterial reduction activity of BT NPs embedded fabric against E. coli and S. aureus. After 24 h bacterial treatment, only 5% bacteria reduction was observed for stock paste applied fabric, while the 20% and 10% BT NPs coated fabric showed a bacterial reduction of 99.8% and 99.4%, respectively. The treated fabric retained its antibacterial activity of more than 98% for both BT NPs concentrations even after 15 laundering cycles, indicating that BT NPs remained firmly attached to the cotton surface. shows the antibacterial activity of coated cotton fabric for E. coli. After 24-h contact period, many damaged areas were noticed by the SEM analysis throughout the sample (). Cotton has higher moisture regain and porosity due to which it is easily attacked by microorganisms. After incubation, the bacterial cells adhered to the pristine cotton swatches, and bacterial colony formation took place, thus damaging the fabric surface. However, BT being ferroelectric killed bacteria colonies on the exposed 10% BT coated surface (washed fabric) and thus prevented the fibers from bacterial damage (). So, even after washing, fabric treated with a 10% pigment concentration can effectively reduce microorganisms.
Table 1. The antibacterial effect of coated cotton.
Figure 5. Antibacterial effect of E. coli on (a) Pure cotton, (b) Fabric coated with 20% BT, (c) Washed fabric (20%), (d) Fabric coated with 10% BT, (e) Washed fabric (10%); and SEM images after 24 h Bacteria contact period (f) Pure Cotton, and (g) Washed fabric (10%).
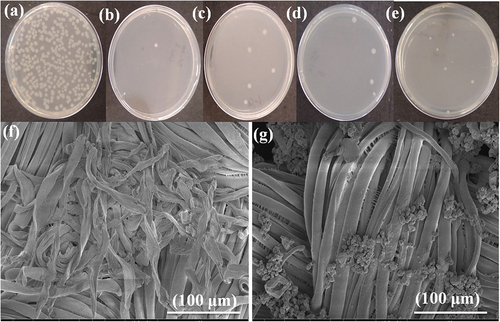
BT bacterial reduction property is assigned to its ferroelectric nature resulting in spontaneous polarization, which kills bacteria when they come into contact with coated cotton’s surface. It is assumed that the negatively charged cell wall of both gram-positive and gram-negative bacteria interacts with NPs or ions and is significantly impacted by the size, shape, charge properties, etc., of the nanoparticles. The antibacterial mechanism of BaTiO3 is not examined comprehensively. However, Sasikumar et al. (Citation2018) have reported that BT NPs can attach and interact with the membrane components, which disrupts cells and kills bacteria. Marin et al. (Citation2021) have elaborated in their study that in an acidic environment, Ba2+ releases from BaTiO3 spontaneously apart from TiO2 which can react with water and produces hydroxyl and free radicals. It was believed that the presence of such radicals damages nucleic acid, bacterial cell walls, and other structures of the bacteria. In this study, we anticipate screen-printed BT NPs generating an analogous impact. It is also expected that this antibacterial effect can be further increased in the presence of some external stimuli such as UV light, mechanical strain, or temperature variation. This is due to the fact that BT is known for photocatalysis, piezocatalysis, and pyrocatalysis.
The results of this study were also compared to those in the literature, as shown in . The results were compared with work previously reported on chitosan, Ag, Zn, Cu, Ti NPs, and their derivatives because there is no study available that evaluates the antibacterial activity of BT NPs on textile. In contrast to results published in other studies in the literature, the prepared samples demonstrated effective antibacterial performance. Since BT has been claimed to be nontoxic, environmentally acceptable, and inexpensive, this study has opened a new window to investigate the potential application of ferroelectric materials in textiles.
Table 2. Comparison of various antibacterial finishes applied on cotton.
Assessment of UV property
Due to the depletion of the ozone layer, long-term exposure to these UV radiations can cause serious health hazards like skin reddening, acne, photoaging, and skin cancer. shows that BaTiO3 is UV active in the region of UVA and UVB with absorption peaks in the range of 300–330 nm. The coated fabric is capable of blocking harmful UV rays and thus can prevent humans from different skin diseases for a longer time due to excellent laundering durability. The UPF value for the pristine cotton fabric was measured as 7.23. Since BT deposition made the fabric UV active, the coated fabric had a UPF measurement of 77.61 and 76.83 for 20% and 10% BT NPs concentrations, respectively, indicating excellent UV protection. After laundering (15 cycles), the 20% and 10% BT coated cotton showed UPF values of 73.48 and 68.77, respectively. The UPF ratings before and after washing do not alter noticeably, demonstrating that the finished fabric has good washing durability and excellent UV protection.
Effect of coating on cotton properties
After coating, it becomes essential to evaluate the water vapor permeability, air permeability, stiffness, and other mechanical properties of the modified fabric. illustrates the effect of BT coating on cotton intrinsic properties. The water vapor permeability for pure cotton, 20% BT, and 10% BT were observed as 44.7, 41.9 ± 2, and 42.6 ± 2 g/m2/h, respectively. As compared to coating work reported in the literature, herein prepared modified cotton shows better permeability (Xu et al. Citation2017; Zhou et al. Citation2021). The air permeability of pristine cotton was measured as 174 cm3/s/cm2, while for 20% and 10% BT coated cotton fabric, the air permeability values were found as 161 cm3/s/cm2, and 166 cm3/s/cm2, respectively. The fabric stiffness of pristine cotton and coated cotton was measured in terms of bending length and flexural rigidity. For pure cotton, the bending length was observed as 43 mm with a flexural rigidity of 14.009 μN.m. After 10% BT coating, the bending length and flexural rigidity were measured as 47 mm and 18.966 μN.m, respectively. The 20% BT coated cotton showed bending length and flexural rigidity of 49 mm and 21.933 μN.m, respectively. The tensile strength for pristine cotton and BT coated (20% and 10%) was measured as 124 N, 122 N, and 123 N, while abrasion resistance was observed as 38,000, 35000, and 37,000 rubs, respectively. These findings suggest that the intrinsic qualities of pure cotton were not significantly affected by surface modification.
Table 3. Effect of BT coating on cotton intrinsic properties.
Conclusions
A durable cotton fabric with antibacterial and UV protection properties was prepared by successfully coating pristine cotton with BaTiO3 nanoparticles in two different concentrations of 10% and 20%. A simple and versatile screen printing technique was employed for embedding these BT NPs on cotton fabric. The results of FTIR, XRD, FE-SEM, and XPS demonstrated that the BT NPs were effectively loaded on the cotton surface. The coated BT NPs remained strongly adhered to the fabric surface even after 15 laundering cycles. The modified fabric showed excellent reduction greater than 99% for both E. coli and S. aureus. After laundering (15 cycles), the 20% and 10% BT coated cotton depicted UPF values of 73.48 and 68.77, respectively, showing excellent UV protection. Additionally, the BaTiO3 coating had no discernible impact on the inherent qualities of cotton, including air and water permeability, fabric stiffness, tensile strength, and abrasion resistance. For use in technical textiles and everyday clothing, the BaTiO3-coated cotton has great potential.
Highlights
BaTiO3 nanoparticles were embedded onto a cotton fabric surface by a crosslinkable acrylate copolymer.
The resultant fabric exhibited excellent antibacterial properties against E. coli and S. aureus even after 15 consecutive washing cycles.
The loaded fabric is capable of blocking harmful ultraviolet (UV) radiations.
The modification of cotton surface did not change its intrinsic properties.
Supplemental Material
Download MS Word (1.5 MB)Acknowledgments
The authors gratefully acknowledge the Advanced Materials Research Center (AMRC), IIT Mandi for providing characterization support and facility.
Disclosure statement
No potential conflict of interest was reported by the author(s).
Supplementary material
Supplemental data for this article can be accessed online at https://doi.org/10.1080/15440478.2022.2139325
References
- Abualnaja, K. M., M. R. ElAassar, R. Y. Ghareeb, A. A. Ibrahim, and N. R. Abdelsalam. 2021. Development of photo-induced Ag0/TiO2 nanocomposite coating for photocatalysis, self-cleaning and antimicrobial polyester fabric. Journal of Materials Research and Technology 15:1513–14. doi:10.1016/j.jmrt.2021.08.127.
- Acosta, M., N. Novak, V. Rojas, S. Patel, R. Vaish, J. Koruza, G. A. Rossetti, and J. Rödel. 2017. BaTiO3-based piezoelectrics: Fundamentals, current status, and perspectives. Applied Physics Reviews 4 (4):041305(1)–041305(49). doi:10.1063/1.4990046.
- Belgacem, M. N., G. Czeremuszkin, S. Sapieha, and A. Gandini. 1995. Surface characterization of cellulose fibres by XPS and inverse gas chromatography. Cellulose 2:145–57. doi:10.1007/BF00813015.
- Bhat, N., A. Netravali, A. Gore, M. Sathianarayanan, G. Arolkar, and R. Deshmukh. 2011. Surface modification of cotton fabrics using plasma technology. Textile Research Journal 81 (10):1014–26. doi:10.1177/0040517510397574.
- Dev, V. R. G., J. Venugopal, S. Sudha, G. Deepika, and S. Ramakrishna. 2009. Dyeing and antimicrobial characteristics of chitosan treated wool fabrics with henna dye. Carbohydrate Polymers 75 (4):646–50. doi:10.1016/j.carbpol.2008.09.003.
- Elhalawany, N., M. E. El-Naggar, A. Elsayed, A. R. Wassel, A. T. El-Aref, and M. A. Abd Elghaffar. 2020. Polyaniline/Zinc/Aluminum nanocomposites for multifunctional smart cotton fabrics. Materials Chemistry and Physics 249:123210. doi:10.1016/j.matchemphys.2020.123210.
- ElShafei, A., and A. A. Okeil. 2011. ZnO/Carboxymethyl chitosan bionano-composite to impart antibacterial and UV protection for cotton fabric Carbohydrate Polymers. 83 (2):920–25. doi:10.1016/j.carbpol.2010.08.083.
- Ferrara, F., E. Pambianchi, B. Woodby, N. Messano, J. P. Therrien, A. Pecorelli, R. Canella, and G. Valacchi. 2021. Evaluating the effect of ozone in UV induced skin damage. Toxicology Letters 338:40–50. doi:10.1016/j.toxlet.2020.11.023.
- Fu, C., W. Ye, Z. Zhai, J. Zhang, P. Li, B. Xu, X. Li, F. Gao, J. Zhai, and D. Y. Wang. 2021. Self-cleaning cotton fabrics with good flame retardancy via one-pot approach. Polymer Degradation and Stability 192:109700. doi:10.1016/j.polymdegradstab.2021.109700.
- Gao, D., X. Li, Y. Li, B. Lyu, J. Ren, and J. Ma. 2021. Long-acting antibacterial activity on the cotton fabric. Cellulose 28:1221–40. doi:10.1007/s10570-020-03560-5.
- Gaspar, D., S. N. Fernandes, A. G. de Oliveira, J. G. Fernandes, P. Grey, R. V. Pontes, L. Pereira, R. Martins, M. H. Godinho, and E. Fortunato. 2014. Nanocrystalline cellulose applied simultaneously as the gate dielectric and the substrate in flexible field effect transistors. Nanotechnology 25 (9):094008. doi:10.1088/0957-4484/25/9/094008.
- Ibrahim H MM and Hassan M S. (2016). Characterization and antimicrobial properties of cotton fabric loaded with green synthesized silver nanoparticles. Carbohydrate Polymers, 151 841–850. 10.1016/j.carbpol.2016.05.041
- Jabar, J. M., T. E. Adedayo, and Y. A. Odusote. 2021. Green, eco-friendly and sustainable alternative in dyeing cotton fabric using aqueous extract Mucuna slonaei F dye: Effects of metal salts pre-mordanting on color strength and fastness properties. Current Research in Green and Sustainable Chemistry 4:100151. doi:10.1016/j.crgsc.2021.100151.
- Kang, C., S. S. Kim, S. J. Kim, and J. W. Lee. 2017. The significant influence of bacterial reaction on physico-chemical property changes of biodegradable natural and synthetic polymers using Escherichia coli. Polymers 9 (4):1–9. doi:10.3390/polym9040121.
- Khan, M. A. M., S. Kumar, M. Ahamed, J. Ahmed, A. Kumar, and M. A. Shar. 2021. BaTiO3@rGO nanocomposite: Enhanced photocatalytic activity as well as improved electrode performance. Journal of Materials Science: Materials in Electronics 32:12911–21. doi:10.1007/s10854-020-04514-0.
- Kumar, S., and V. Luthra. 2021. Raman and infrared spectroscopic investigation of the effects of yttrium and tin co-doping in barium titanate. The Journal of Physics and Chemistry of Solids 154 (March):110079. doi:10.1016/j.jpcs.2021.110079.
- Kumar, S., M. Sharma, T. Frömling, and R. Vaish. 2021. Antibacterial ferroelectric materials: Advancements and future directions. Journal of Industrial and Engineering Chemistry 97:95–110. doi:10.1016/j.jiec.2021.02.016.
- Kumar, S., M. Sharma, S. Powar, E. N. Kabachkov, and R. Vaish. 2019. Impact of remnant surface polarization on photocatalytic and antibacterial performance of BaTiO3. Journal of the European Ceramic Society 39 (9):2915–22. doi:10.1016/j.jeurceramsoc.2019.03.029.
- Kumar, A., M. Sharma, and R. Vaish. 2022a. Durable antibacterial cotton fabric via spray-coating of photocatalytic MoS2. Materials Chemistry and Physics 126658. doi:10.1016/j.matchemphys.2022.126658.
- Kumar, A., M. Sharma, and R. Vaish. 2022b. Screen printed calcium fluoride nanoparticles embedded antibacterial cotton fabric. Materials Chemistry and Physics 288:126449. doi:10.1016/j.matchemphys.2022.126449.
- Liu, J., G. Gao, S. Zhang, Y. Huang, J. Wu, X. Hu, J. Lu, Q. Zhang, L. Zhou, and Y. Huang. 2019. Cotton-assisted surgical clipping of very small aneurysms: A two-center study. World Neurosurgery 127:e242–50. doi:10.1016/j.wneu.2019.02.227.
- Li, P., B. Wang, Y.-Y. Liu, Y.-J. Xu, Z.-M. Jiang, C.-H. Dong, L. Zhang, Y. Liu, and P. Zhu. 2020. Fully bio-based coating from chitosan and phytate for fire-safety and antibacterial cotton fabrics. Carbohydrate Polymers 237:116173. doi:10.1016/j.carbpol.2020.116173.
- Marin, E., F. Boschetto, T. P. M. Sunthar, M. Zanocco, E. Ohgitani, W. Zhu, and G. Pezzotti. 2021. Antibacterial effects of barium titanate reinforced polyvinyl-siloxane scaffolds. International Journal of Polymeric Materials and Polymeric Biomaterials 70 (6):425–36. doi:10.1080/00914037.2020.1725757.
- Marković, D., C. Deeks, T. Nunney, Ž. Radovanović, M. Radoičić, Z. Šaponjić, and M. Radetić. 2018. Antibacterial activity of Cu-based nanoparticles synthesized on the cotton fabrics modified with polycarboxylic acids. Carbohydrate Polymers 200:173–82. doi:10.1016/j.carbpol.2018.08.001.
- Montaser, A. S., M. Rehan, W. M. El-Senousy, and S. Zaghloul. 2020. Designing strategy for coating cotton gauze fabrics and its application in wound healing. Carbohydrate Polymers 244:116479. doi:10.1016/j.carbpol.2020.116479.
- Nhlapo, M., M. Mashego, M. Low, D. Ming, and K. Harding. 2019. Investigating the development of low-cost sanitary pads. Procedia Manufacturing 35:589–94. doi:10.1016/j.promfg.2019.05.083.
- Pandiyarasan, V., J. Archana, A. Pavithra, V. Ashwin, M. Navaneethan, Y. Hayakawa, and H. Ikeda. 2017. Hydrothermal growth of reduced graphene oxide on cotton fabric for enhanced ultraviolet protection applications. Materials Letters 188:123–26. doi:10.1016/j.matlet.2016.11.047.
- Portella, E. H., D. Romanzini, C. C. Angrizani, S. C. Amico, and A. J. Zattera. 2016. Influence of stacking sequence on the mechanical and dynamic mechanical properties of cotton/glass fiber reinforced polyester composites. Materials Research 19 (3):542–47. doi:10.1590/1980-5373-MR-2016-0058.
- Qin, S., D. Liu, Z. Zuo, Y. Sang, X. Zhang, F. Zheng, H. Liu, and X.-G. Xu. 2010. UV-irradiation-enhanced ferromagnetism in BaTiO3. The Journal of Physical Chemistry Letters 1 (1):238–41. doi:10.1021/jz900131x.
- Qi, H., J. Pan, F. Qing, K. Yan, and G. Sun. 2016. Anti-wrinkle and UV protective performance of cotton fabrics finished with 5-(carbonyloxy succinic)-benzene-1,2,4-tricarboxylic acid. Carbohydrate Polymers 154:313–19. doi:10.1016/j.carbpol.2016.05.108.
- Qiu, Q., S. Chen, Y. Li, Y. Yang, H. Zhang, Z. Quan, X. Qin, R. Wang, and J. Yu. 2020. Functional nanofibers embedded into textiles for durable antibacterial properties. Chemical Engineering Journal 384:123241. doi:10.1016/j.cej.2019.123241.
- Raeisi, M., Y. Kazerouni, A. Mohammadi, M. Hashemi, I. Hejazi, J. Seyfi, H. A. Khonakdar, and S. M. Davachi. 2021. Superhydrophobic cotton fabrics coated by chitosan and titanium dioxide nanoparticles with enhanced antibacterial and UV-protecting properties. International Journal of Biological Macromolecules 171:158–65. doi:10.1016/j.ijbiomac.2020.12.220.
- Raja, S., D. Bheeman, R. Rajamani, S. Pattiyappan, S. Sugamaran, and C. S. Bellan. 2015. Synthesis, characterization and remedial aspect of BaTiO3 nanoparticles against bacteria. Nanomedicine and Nanobiology 2 (1):16–20. doi:10.1166/nmb.2015.1014.
- Ranjbar-Mohammadi, M. 2018. Production of cotton fabrics with durable antibacterial property by using gum tragacanth and silver. International Journal of Biological Macromolecules 109:476–82. doi:10.1016/j.ijbiomac.2017.12.093.
- Sasikumar, M., A. Ganeshkumar, M. N. Chandraprabha, R. Rajaram, R. H. Krishna, N. Ananth, and P. Sivakumar. 2018. Investigation of Antimicrobial activity of CTAB assisted hydrothermally derived Nano BaTiO3. Materials Research Express 6 (2):025408. doi:10.1088/2053-1591/aaee3b.
- Sela, S. K., A. K. M. Nayab-Ul-Hossain, M. S. I. Rakib, and M. K. H. Niloy. 2020. Improving the functionality of raw cotton: Simultaneous strength increases and additional multi-functional properties. Heliyon 6 (8):e04607. doi:10.1016/j.heliyon.2020.e04607.
- Shaheen, T. I., M. E. El-Naggar, A. M. Abdelgawad, and A. Hebeish. 2016. Durable antibacterial and UV protections of in situ synthesized zinc oxide nanoparticles onto cotton fabrics. International Journal of Biological Macromolecules 83:426–32. doi:10.1016/j.ijbiomac.2015.11.003.
- Trivedi, M. K., G. Nayak, S. Patil, R. M. Tallapragada, O. Latiyal, and S. Jana. 2015. Impact of Biofield Treatment on Atomic and Structural Characteristics of Barium Titanate Powder. Industrial Engineering & Management 4 (3):1000166. doi:10.4172/2169-0316.1000166.
- Valacchi, G., C. Sticozzi, A. Pecorelli, F. Cervellati, C. Cervellati, and E. Maioli. 2012. Cutaneous responses to environmental stressors. Annals of the New York Academy of Sciences 1271 (1):75–81. doi:10.1111/j.1749-6632.2012.06724.x.
- Verma, M., N. Gahlot, S. S. J. Singh, and N. M. Rose. 2021. UV protection and antibacterial treatment of cellulosic fibre (cotton) using chitosan and onion skin dye. Carbohydrate Polymers 257:117612. doi:10.1016/j.carbpol.2020.117612.
- Wang, W., L. Cao, W. Liu, G. Su, and W. Zhang. 2013. Low-temperature synthesis of BaTio 3 powders by the sol–gel-hydrothermal method. Ceramics International 39 (6):7127–34. doi:10.1016/j.ceramint.2013.02.055.
- Wegmann, M., L. Watson, and A. Hendry. 2004. XPS analysis of submicrometer barium titanate powder. Journal of the American Ceramic Society 87 (3):371–77. doi:10.1111/j.1551-2916.2004.00371.x.
- Wong, A. S. W., Y. Li, and K.-W. Yeung. 2005. The influence of thermal comfort perception on consumer’s preferences to sportswear. Elsevier Ergonomics Book Series 3:321–28. doi:10.1016/S1572-347X(05)80051-5.
- Xing, H., J. Cheng, X. Tan, C. Zhou, L. Fang, and J. Lin. 2020. Ag nanoparticles-coated cotton fabric for durable antibacterial activity: Derived from phytic acid–ag complex. The Journal of the Textile Institute 111 (6):855–61. doi:10.1080/00405000.2019.1668137.
- Xu, Q., P. Wang, Y. Zhang, and C. Li. 2021. Durable antibacterial and UV protective properties of cotton fabric coated with carboxymethyl chitosan and Ag/TiO2 composite nanoparticles. Fibers and Polymers 23:386–95. doi:10.1007/s12221-021-0352-z.
- Xu, Q., L. Xie, H. Diao, F. Li, Y. Zhang, F. Fu, and X. Liu. 2017. Antibacterial cotton fabric with enhanced durability prepared using silver nanoparticles and carboxymethyl chitosan. Carbohydrate Polymers 177:187–93. doi:10.1016/j.carbpol.2017.08.129.
- Ye, Z., S. Li, S. Zhao, L. Deng, J. Zhang, and A. Dong. 2021. Textile coatings configured by double-nanoparticles to optimally couple superhydrophobic and antibacterial properties. Chemical Engineering Journal 420:127680. doi:10.1016/j.cej.2020.127680.
- Zhang, D., L. Chen, C. Zang, Y. Chen, and H. Lin. 2013. Antibacterial cotton fabric grafted with silver nanoparticles and its excellent laundering durability. Carbohydrate Polymers 92:2088–94. doi:10.1016/j.carbpol.2012.11.100.
- Zhang, S., T. Zhang, J. He, and X. Dong. 2021. Effect of AgNP distribution on the cotton fiber on the durability of antibacterial cotton fabrics. Cellulose 28:9489–504. doi:10.1007/s10570-021-04113-0.
- Zhou, S., W. Wang, Y. Sun, X. Tang, B. Zhang, and X. Yao. 2021. Antibacterial effect of Ag-PMANa modified cotton. Colloids and Surfaces A: Physicochemical and Engineering Aspects 618:126453. doi:10.1016/j.colsurfa.2021.126453.