ABSTRACT
Organic soil is a poor bearing medium and is susceptible to uncharacteristic volume change and settlement behavior. Geotechnical design of substructures in organic soil is often of high economic value to account for its weak geotechnical properties. Fiber reinforcement improves the strength of the soil. However, fibers act as a mechanical reinforcement in soil mass rather than enhancing the intrinsic nature of the soil. But chemical additives alter its behavior, thereby improving its strength. This study investigates the strength and hydraulic conductivity (HC) of low plastic organic silt reinforced with banana fiber and nano-silica. Banana fiber was added in dosages of 0.25%, 0.50%, 0.75% and 1%. The optimum fiber dosage was 0.75%. Nano-silica was added to the 0.75% banana fiber reinforced soil at dosages of 0.2%, 0.4%, 0.6% and 0.8% to improve the strength of the reinforced soil. The effect of aging on HC and unconfined compressive strength (UCS) was studied for a period of 90 d. Results showed that nano-silica and banana fiber beneficially complemented each other in the enhancement of geotechnical properties. Nano-silica increased the strength of fiber-reinforced soil, and banana fiber averted the brittle failure nature imposed by the nano-silica. Also, it improved drainage characteristics through aggregation action.
摘要
有机土是一种贫瘠的承载介质,易受体积变化和沉降行为的影响. 有机土中下部结构的岩土工程设计通常具有很高的经济价值,因为其岩土特性较弱. 纤维增强增强了土壤的强度. 然而,纤维在土体中起着机械加固的作用,而不是增强土壤的固有性质. 但是化学添加剂改变了它的行为,从而提高了它的强度. 本研究研究了香蕉纤维和纳米二氧化硅增强低塑性有机粉土的强度和导水率. 香蕉纤维以0.25%、0.50%、0.75%和1%的剂量添加. 最佳纤维用量为0.75%。将纳米二氧化硅以0.2%、0.4%、0.6%和0.8%的剂量添加到0.75%香蕉纤维增强土中,以提高增强土的强度. 在90天的时间内,研究了老化对导水率和无侧限抗压强度(UCS)的影响. 结果表明,纳米二氧化硅和香蕉纤维在增强岩土性能方面相互补充. 纳米二氧化硅提高了纤维增强土壤的强度,香蕉纤维避免了纳米二氧化硅造成的脆性破坏. 此外,它还通过聚集作用改善了排水特性.
Introduction
Numerous techniques can be adopted to improve the properties of the soil and includes a variety of options like densifying the soil using compaction techniques or installation of stone columns; draining the water to facilitate consolidation of soil using vertical drains; reinforcing the soil using geosynthetics or fibers; and by the inclusion of additives to the soil such as cement, lime, flyash, polymers and other strength-enhancing materials. The choice of such methods depends on the extent of ground improvement required, nature of the soil, type of structure and type of foundation planned to use for the structure. Treating the soil with additives or reinforcing the soil with fibers can be implemented even for a small area and can be suitably limited up to the depth of stress influence.
Soil can be reinforced by installing layered geosynthetics or by random inclusion of natural/synthetic fibers in soil. Geosynthetics has a great range of field applications, but also may create potential weak planes at the interface of soil and geosynthetics (Maher and Gray Citation1990). On the other hand, random inclusion of short fibers can give isotropic enhancement in strength (Ahmad, Bateni, and Azmi Citation2010). Random fiber inclusion is based on a nature-inspired theory of plant roots on the slope enhancing its stability (Wang et al. Citation2019). Yixian et al. (Citation2016) studied the interaction mechanism of jute fiber-reinforced expansive soil. They found that the cohesion increased upto an optimum dosage of 0.6% and the friction angle showed negligible variations. Sujatha et al. (Citation2020) showed that 0.75% alkali-resistant glass fiber and 0.75% electronic grade glass fiber enhanced lean clay strength by 1.52 times and 0.94 times, respectively. Bawadi et al. (Citation2020) used banana fibers to investigate the shear strength improvement of clay and found that the strength improved at all the investigated dosages but was maximum at 0.5% inclusion. Similarly, Modha, Sharma, and Chavda (Citation2020) noted a considerable improvement in the shear strength of the soil with 0.5% banana fiber reinforcement. Also, Khan et al. (Citation2019) observed that 4.5% NaOH-treated banana fiber would improve fiber’s roughness and bonding. Zhao, Wu, and Huang (Citation2021) used basalt and polypropylene fibers to study the strength improvement in red clay and observed that basalt fiber outperformed polypropylene. Fiber reinforcement also improves the post-peak response of soil (Sujatha et al. Citation2018). However, the strength of additive-treated soil is better by many folds than fiber-reinforced soil (Wang et al. Citation2019).
Among various soil types, those with organic content are often regarded as problematic, owing to their inadequate bearing capacity, excessive settlement, reduced HC and poor drainage upon loading. Several authors have worked on various additive-based improvement methods to mitigate the undesirable effects of organic soil (Latifi et al. Citation2016; Nath, Molla, and Sarkar Citation2017; Paul and Hussain Citation2020; Randhawa, Chauhan, and Kumar Citation2021) Latifi et al. (Citation2016) reported that usage of 2% environmental friendly xanthan gum on peat improved unconfined compressive strength (UCS) from 13 kPa to 83 kPa after 28 d of curing. Nath, Molla, and Sarkar (Citation2017) investigated the behavior of flyash amended high plastic organic soil and suggested that class C flyash offered better strength enhancement than class F flyash. Paul and Hussain (Citation2020) studied the compressibility behavior of four different types of peat mixed with ordinary Portland cement and observed that at a higher dosage of 15% of cement, the coefficient of consolidation increased, and the HC reduced. Randhawa, Chauhan, and Kumar (Citation2021) studied the enhanced performance of lime-treated black cotton soil of Indian origin. Results showed that adding 9% lime to the soil improved the UCS by 80.5% after 28 d of curing.
Chemical admixtures such as cement, lime would exhibit phenomenal strength improvement in problematic soils and an excellent reduction in their HC. But they are required in larger quantities and impact the soil environment adversely as they leave a carbon footprint during their manufacturing process (Barcelo et al. Citation2014). Recent developments in biotechnology and nanotechnology introduced many innovative materials for ground improvement such as geopolymer stabilization, biopolymer treatment and nanomaterial inclusion as a viable alternative to stabilize weak soils. Nanomaterial-based soil stabilization has various advantages like high specific surface area (SSA) and reactivity of materials owing to its smaller size, effective in achieving enhanced performance in relatively lesser dosages than conventional additives. Commonly researched nanomaterials are nano-silica, nano-clay and carbon-based nanomaterials. Nano-clay is not effective on adding with existing expansive clay minerals (Taha and Taha Citation2012). Carbon-based nanomaterials have higher efficacy in strength improvement; but, are costlier (Esawi and Farag Citation2007). Nano-silica remains a relatively budget-friendly and known for its proven results in concrete and mortar strength enhancements. Also, many researchers demonstrated that nano-silica could show assuring response in stabilizing soil (Ahmadi and Shafiee Citation2019; Changizi and Haddad Citation2017). Changizi and Haddad (Citation2017) showed a 56% strength improvement on clay treated with 0.7% nano-silica. Ahmadi and Shafiee (Citation2019) compared the strength enhancement mechanism in micro-silica and nano-silica-treated clay and reported that both micro-silica and nano-silica contributed to strength improvement. Kannan and Sujatha (Citation2022) noted that 0.6% nano-silica exhibited a tendency to improve the strength of organic silt by 2.21 times after 180 d due to viscous CSH gel formation. However, they also reported brittle behavior in the treated soil with aging. A thorough literature search clearly indicates that nano-silica can improve soil strength at a relatively lesser dosage but impart brittle failure in the treated sample (Changizi and Haddad Citation2017; Kannan and Sujatha Citation2022).
Changizi and Haddad (Citation2015) used 1% nano-silica and 0.3% polyester fiber and improved the shear strength by 190%. Boz et al. (Citation2018) infused lime amended clay with basalt and polypropylene fiber. A maximum strength improvement of 8.5 times was achieved after curing for 90 d on 0.75% of 19 mm basalt fiber and 9% lime. Choobbasti, Samakoosh, and Kutanaei (Citation2019) combined carpet waste fibers and nano-calcium carbonate and observed that 0.6% carpet waste fiber and 1.2% nano-calcium carbonate increased the UCS by 50%. Gobinath et al. (Citation2020) showed that the addition of 1% sodium silicate and 0.5% banana fiber improved the UCS by 445%.
Banana fibers are obtained from the pseudo stem of the banana tree and are used for various industrial applications in making paper, natural filters, textiles and rope. They are lightweight with high strength, durable, have less elongation tendency (Adeniyi et al. Citation2021; Bharathi, Vinodhkumar, and Saravanan Citation2021), eco-friendly and biodegradable in contrast to synthetic fibers. India is a leading producer of banana at global scale and contributes by nearly 20.08% of worlds banana production. Nano-silica has proved to be an effective additive that can give enhanced strength at a relatively lesser dosage than conventional additives. Research on fibers and nano-silica blended soil are trending in recent times with very less attention being paid to the choice of natural fibers (Cui et al. Citation2018). The present study investigates the choice of banana fiber and nano-silica to enhance the strength of low plastic silt with organic content. The study reports the effect of the combined treatment on decisive geotechnical parameters such as compaction characteristics, UCS & HC of the fiber-reinforced nano-silica treated soil. The results are supplemented with micro-morphology observations and study on chemical changes using SEM, FESEM, XRD and FTIR to understand the mechanism of modification of geotechnical properties.
Materials and methods
Soil was excavated from 1 m deep trenches made in the agricultural fields at Ariyalur, Tamil Nadu avoiding topsoil and agricultural waste. The soil was black in color, and had a characteristic pungent odor indicating the presence of organic content. Organic content was determined based on loss on ignition method (ASTM D2974 Citation2020). Soil had a organic content of 13.6%. The soil is classified as low plastic silt with organic content (OL) based on the Unified Soil Classification System (USCS). XRF analysis () indicated the presence of abundant silica and noticeable calcium oxide indicating the presence of calcium content in the soil. Calcium content was of particular interest as the soil was extracted from an area with abundant limestone mines (Mohan et al. Citation2021).
Table 1. XRF analysis of the study soil.
Banana fibers of 1 mm average diameter and tensile strength 715 MPa was purchased from Fiber Region, Chennai, India. Banana fibers used for the study were cut to 10 mm length as authors Estabragh, Ranjbari, and Javadi (Citation2017) suggested that fiber length upto 10 mm aids in the increase of UCS but lengths beyond 10 mm causes bundling effect while mixing and impacts the strength adversely. Nano-silica with an average particle size less than 17 nm, 99.88% purity and a specific surface area of 202 m2/g was procured from Astraa Chemicals, Chennai, India. lists the properties of the soil, banana fiber & nano-silica. Specifications for banana fiber and nano-silica are provided by the supplier.
Table 2. Material properties of soil, banana fiber and Nano-Silica.
Experimental investigation
Trial investigations at dosages of 0.25%, 0.50%, 0.75%, 1.00% and 1.25% were conducted to determine the banana fiber dosage and was fixed as 0.25%, 0.50%, 0.75% and 1.00% by dry weight of the soil based on UCS test. UCS tends to decrease beyond 0.75% addition of banana fibers. Nano-silica stabilization was adopted for the optimum fiber dosage of 0.75% and nano-silica content varied as 0.2%, 0.4%, 0.6% and 0.8% after trial testing in the dosage range of 0.2% to 1.00% as UCS registered a decrease beyond 0.6% nano-silica addition. The test plan of the geotechnical investigation is presented in and tests were conducted in triplicate to ensure the repeatability of the results.
Table 3. Test plan for the experimental investigation.
The soil sample was dried at a temperature of 100°C in a thermostatically controlled oven to remove the field moisture, rammed and sieved to the required particle sizes as per the specifications of the experimental investigation. Treated banana fibers were thoroughly hand-mixed to ensure uniform spreading in the soil. In case of the combination study involving banana fiber and nano-silica, the soil was dry-mixed with the predefined quantity of nano-silica manually, followed by the addition of fibers to prevent improper spreading of nano-silica if added along with fibers. The soil was initially divided into ten parts with appropriated quantity of nano additive and dry mixed for uniform blending and were then combined and mixed for ten minutes to achieve a homogeneous mixture after which banana fibers were added.
Microscopic images obtained through a handheld digital microscope with a complementary metal-oxide semiconductor sensor having a focus range of 15 mm to 45 mm was used to study the surface cracks developed in the failed sample. Micrographs of soil were also obtained from Tescan Veega 3 scanning electron microscope (SEM) and Zeiss Gemini field emission scanning electron microscope (FESEM) to study the surface morphology after treatment. X-Ray Diffraction (XRD) analysis for a 2θ range of 20° to 80° was carried out to investigate the formation of new compounds using Bruker X-ray diffractometer. Perkin Elmer’s FTIR spectrometer was used for analyzing the modifications in the functional group after treatment in the soil.
Light compaction tests according to ASTM D698 (Citation2012) were conducted on samples after curing them in airlock bags for a period of two hours. Cylindrical samples of 38 mm diameter and 76 mm height were prepared for unconfined compression (UCC) test at their maximum dry unit weight (MDUW) to determine the strength of modified soil (ASTM D2166 Citation2006) after curing in airlock covers till their respective curing periods, namely 0d (two hours after sample preparation), 7d, 14d, 28d, 56d and 90d. The samples were loaded at a strain rate of 1.25 mm/min. Sample preparation of banana fiber reinforced soil (BFRS) and nano-silica stabilized banana fiber reinforced soil (NBFRS) is illustrated in . Hydraulic conductivity (ASTM D5856 Citation2015) of BFRS and NBFRS was investigated for the same curing periods as that of strength. Samples were in continuous saturation for the entire test period to evaluate the prolonged effects.
Results and discussion
Compaction characteristics
Compaction characteristics of soil, BFRS and NBFRS were studied to determine the MDUW and OMC (). The MDUW of BFRS decreased from 16.8 kN/m3 for soil to 15.75 kN/m3 at 1.00% banana fiber addition. The marginal decrease in MDUW can be attributed to the two reasons – (i) replacing heavier soil with lighter fibers and (ii) resistance offered by fibers to compaction effort. A similar trend in MDUW was observed by authors Soltani, Deng, and Taheri (Citation2018) and Murthi, Saravanan, and Poongodi (Citation2020) on polypropylene fiber-reinforced expansive soil and black cotton soil. The OMC was not affected by the addition of banana fibers as the treated fibers do not tend to absorb water.
Figure 2. MDUW and OMC plots (a) Soil reinforced with varying dosages of banana fiber and (b) Soil reinforced with 0.75% banana fiber and treated with varying dosages of nano-silica.
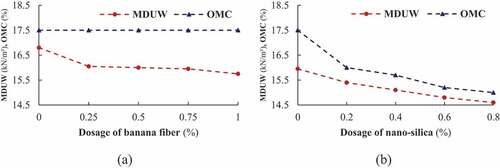
The soil reinforced with 0.75% banana fiber had an MDUW of 15.95 kN/m3 and an OMC of 17.5%, as observed from . The addition of nano-silica reduced both MDUW and OMC of the fiber-reinforced soil. shows that the MDUW and OMC decreased to 14.6 kN/m3 and 15.0%, respectively, after treating the reinforced soil with 0.8% nano-silica. The addition of nano-silica along with fiber caused the absorption of water due to the presence of nano-silica particles, owing to its hydrophilic nature and higher specific surface area (Mirzababaei et al. Citation2021). Further, nano-additives initially filled the voids and then prompted the aggregation of soil particles (Changizi and Haddad Citation2017), forming a flocculated structure preventing further compression of soil mass at a significantly lesser water content. A similar trend on the reduction of both MDUW and OMC with an increase in dosage of additives was observed by Jasim and Cetin (Citation2016) in clayey silt treated with sawdust, where the sawdust filled the voids and absorbed the moisture from the soil, causing a drop in MDUW and OMC.
Strength characteristics
Failure strain and failure pattern
The organic soil failed at a strain of 4.61% exhibiting a small lateral expansion in the sample, indicating a ductile failure mode. The addition of fibers produced a gradual failure with improved post-peak strength. For all the fiber-reinforced samples, the stress corresponding to a strain of 10% was selected as the UCS (Sujatha et al. Citation2018) as the stress-strain response was gradual. Failure mode of soil, nano silica-treated soil and NBFRS are shown in . A trial study on samples treated with nano-silica showed that the samples failed at a strain of 4.28%, 4.28%, 2.96% and 2.96% corresponding to the dosages 0.2%, 0.4%, 0.6% and 0.8% respectively with slight bulging accompanied by diagonal cracks (), corresponding to a semi-brittle shear failure. Literature shows that with aging, samples failed completely with strong signatures of brittle nature (Changizi and Haddad Citation2017) indicating that nano-silica increased the resistance to deformation causing the soil to fail at a comparatively lesser strain. The addition of fibers in the nano-silica treated sample altered the brittle behavior of soil as observed from the stress-strain response with a relatively gradual and smooth post-peak response (). shows the NBFRS sample at 0d, exhibiting a combination of vertical tensile cracks and bulging. Although surface cracks were visible, the fibers bridged the gap (Changizi and Haddad Citation2015; Sujatha et al. Citation2018). The magnified image () clearly shows that the fiber is embedded in the soil matrix, thereby establishing a firm grip. The tendency of the fibers to hold soil particles in the matrix on the application of load to failure and beyond results in a gradual failure response of the treated soil.
Unconfined compression strength
The organic soil has a UCS of 172.4 kPa at a water content of 17.5%. At 0.25% and 0.50% fiber reinforcement, the strength reduced to 120 kPa and 154.97 kPa, respectively, lesser than that of the organic soil. The reason for the reduced strength could be attributed to the fact that at lower doses, the fiber-soil contact is minimal and weak; hence, the fiber net cannot be formed efficiently, resulting in poor performance (Gao et al. Citation2015). The strength of the soil increased to 201.01 kPa with a 0.75% fiber addition but then again reduced to 185.02 kPa at 1.00% banana fiber reinforcement. At higher fiber dosages, fiber balling/clumping could have caused the strength reduction (Sujatha et al. Citation2018). Hence, 0.75% banana fiber by dry weight of the soil is the optimum fiber dosage. This dosage was used in the NBFRS. Fiber reinforcement enhanced the bonding in the soil matrix at the fiber-soil interfaces, leading to strength improvement. Beyond optimum dosage, adding fibers in excess made the soil lose its cohesive strength (Puppala et al. Citation2000), owing to more fiber per unit volume. This clearly indicates that fiber reinforcement does not alter the chemical nature of the soil and the strength improvement was through mechanical reinforcement only. Controlled curing conditions adopted for the study ensured negligible variations in the moisture and microstructural state of the fiber-reinforced soil and therefore effect of aging on strength was ignored for the BFRS.
Strength of soil treated with 0.2% to 0.8% nano-silica after 2 hours of curing showed that the UCS increased to 211.01 kPa, 216.07 kPa, 207.06 kPa and 190.81 kPa for the dosages from 0.2% to 0.8%, respectively. The UCS of NBFRS increased upto 0.4% nano-silica and decreased thereafter (). The strength increased with aging for both BFRS and NBFRS. At 0d and 90d of curing, strength improved to 220.94 kPa and 270.35 kPa, respectively, at the optimum dosage of 0.4% nano-silica and 0.75% fiber-reinforced soil.
A strength improvement by nearly 57% than the organic soil and 34.50% than the fiber-reinforced soil was observed on combining nano-silica stabilization and fiber reinforcement.
Failure modulus
Failure modulus shows the ease or ability of the material to stretch or deform and is calculated as the ratio of stress to 10% strain. The soil possessed a failure modulus of 3739.70 kPa. Reinforcing soil with banana fiber resulted in a decrease in the magnitude of the modulus as 1216 kPa, 1570.36 kPa, 2036.90 kPa and 1874.87 kPa at dosages ranging between 0.25% and 1.00%. During the trial study, nano-silica treated soil showed higher modulus of 4930.14 kPa, 5048.36 kPa, 6995.27 kPa and 6446 kPa for the dosages ranging from 0.2% to 0.8%, respectively. Failure modulus of BFRS and NBFRS increased with aging () indicating the strength gain and resistance to deformation with ageing.
Table 4. Failure modulus of fiber and nano-silica treated samples.
Reaction mechanism
shows the mechanism of strength change with various dosages of fiber and nano-silica. The addition of fiber upto optimum dosage bridged the voids in the soil matrix; however, further addition of fibers beyond the optimum dosage caused separation in the soil matrix, and fibers failed to compensate the cohesive strength of the soil when they occupied the clay’s space in case of BFRS. Similarly, up to optimum dosage, the nano-silica filled the voids in the soil mass at initial curing periods, but with time became a viscous gel (Changizi and Haddad Citation2017); however, beyond the optimum dosage nano-silica caused aggregation in the soil particle increasing the void spaces in case of NBFRS. Despite the presence of fiber, the aggregated soil mass, therefore, was not able to attain strength gain. Also, Changizi and Haddad (Citation2017) reported that the presence of nano silica reduces the distance between the clay particles and improves the interfacial friction in the soil particles leading to higher strength. The presence of banana fibers in soil matrix causes the viscous gel to adhere to the fiber and thereby improves the interfacial strength of the soil (Changizi and Haddad Citation2015).
XRD pattern () reveal that the organic soil has quartz, traces of calcium carbonate, silicon dioxide, clay minerals like halloysite and saponite and NBFRS exhibited calcium silicate hydrate (CSH) peaks indicating the gel phase in the treated soil, causing strength enhancement. Authors like Pastor et al. (Citation2019) observed that the reaction of calcium and silica ions to form CSH is a long-term slow process and this explains the slow rate of strength improvement with aging. The viscous gel formed induced a higher degree of aggregation at dosages beyond optimum values, thereby reducing strength (Changizi and Haddad Citation2017). FTIR spectra of the soil and treated soil are shown in . The intensity variation around 1033 cm−1 in the organic soil and treated soil hints the presence of alumino-silica lattice of clay minerals, such as kaolinite, smectite, illite (Tinti et al. Citation2015). This result augments the presence of saponite (a clay mineral that belongs to the smectite group) as identified from XRD. Also, it indicates the stacking of soil hinting the formation of hydration products (Lv et al. Citation2018). The change in the shape of spectral response around 3400 cm−1 confirms the stretching of the OH bond, indicating the adsorption of water molecules (Vakili et al. Citation2020). This phenomenon signifies the water adsorption involved in the reduced OMC and the CSH gel formation phase.
Hydraulic conductivity (HC)
HC is an important geotechnical parameter that ensures the suitability of the soil for various geotechnical applications like earthen dam constructions and landfill liners. The organic soil has a HC of 8.8 × 10−8 cm/s. The addition of banana fiber and nano-silica increased the HC of the treated soil, indicating the enhanced drainage properties of the treated soil. However, the HC decreased with aging. shows that the inclusion of fiber reduced the HC upto 0.75% banana fiber but HC increased at dosages higher than 0.75%. HC of 0.75% BFRS are 2.68 × 10−7 cm/s and 5.15 × 10−9 cm/s at 0 and 90 d respectively. Fiber reinforcement bridges the voids in the soil leading to reduced HC, but beyond optimum dosage, more fibers are available per unit volume, causing increased fiber-soil contact regions, creating more flow paths and increased HC (Divya, Viswanadham, and Gourc Citation2018).
Figure 10. HC plots of (a) Soil reinforced with varying dosages of banana fiber and (b) Soil reinforced with 0.75% banana fiber and varying dosages of nano-silica.
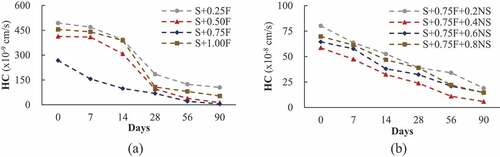
The HC of NBFRS decreased up to a dosage of 0.4% nano-silica addition and increased beyond the optimum dosage of 0.4% nano-silica (). At 0.4% nano-silica, HC at 0d and 90d curing period are 5.85 × 10−7 cm/s and 5.74 × 10−8 cm/s respectively. Aggregation increased with the dosage of nano-silica (Ahmadi and Shafiee Citation2019). Mirzababaei et al. (Citation2021) suggested that the addition of nanoparticles would cause a flocculated structure in the soil mass as an effect of aggregation and this flocculation causes more voids in the soil. The voids at saturated conditions allow more water to pass through them, causing an increase in HC. This also explains the more permeable nature of the NBFRS sample than the BFRS samples. However, with time, the viscous gel formed tends to reduce the ease of water flow, resulting in a slightly lesser HC with aging. points that the organic soil has lesser voids, but in NBFRS void spaces have increased due to particle aggregation (). Although the fibers bridge the gap between the aggregated soil mass, the agglomeration led to void formation in the soil (Changizi and Haddad Citation2017), facilitating easy drainage of water. SEM images could not show the presence of CSH due to their lower magnification, hence FESEM micrographs were used to visualize the viscous gel formation. The FESEM images of the treated soil in show the CSH gel formation caused by the nano-silica addition in the soil after 28d of curing confirming the changes in strength and HC of NBFRS.
Conclusion
A combination of an additive like nano-silica with fibers not only enhanced the strength but also provided appreciable post-peak behavior. The results of the experimental investigation showed that reinforcing soil with banana fibers reduced the MDUW and OMC. OMC decreased negligibly. Strength improved appreciably by 16.6% for BFRS at 0.75% banana fiber dosage and exhibited gradual failure post-peak. On nano-silica stabilization to BFRS a considerable increase in strength by 56.82% was observed in comparison with virgin soil. Also, 34.50% increase was noted in comparison with 0.75% BFRS at 0.4% nano-silica dosage. HC showed a decreasing trend upto the optimum dosage and increased on further addition of fiber and nano-silica. Also, with aging HC decrease for both BFRS and NBFRS. After 90d curing, HC decreased by 88.35% in case on NBFRS compared to that of BFRS. The changes in strength and HC can be attributed to the formation CSH gel as observed from chemical analysis and surface morphology studies. The study advocates random fiber inclusion in additive stabilized soils showing brittle failure to improve the post-peak failure characteristics and the choice of natural fibers like banana, jute, sisal, areca, coconut, etc to aid sustainable development as they provide the option for minimal intervention to the soil environment.
Highlights
Organic silt showed 16.6% strength improvement with 0.75% banana fiber reinforcement
Nano-silica enhanced the shear strength of the soil with CSH formation but exhibited brittle failure
0.4% nano-silica enhanced the strength of 0.75% banana fiber treated soil by 34.5%
Banana fiber reinforcement suppressed the brittle failure in nano-silica treated soil
Acknowledgments
The authors thank the Vice-Chancellor of SASTRA Deemed University, Thanjavur, India, for the support and facilities provided to carry out this work successfully.
Disclosure statement
No potential conflict of interest was reported by the author(s).
Additional information
Funding
References
- Adeniyi, A. G., A. S. Adeoye, J. O. Ighalo, and D. V. Onifade. 2021. FEA of effective elastic properties of banana fiber-reinforced polystyrene composite. Mechanics of Advanced Materials and Structures 28 (18):1869–15. doi:10.1080/15376494.2020.1712628.
- Ahmad, F., F. Bateni, and M. Azmi. 2010. Performance evaluation of silty sand reinforced with fibres. Geotextiles and Geomembranes 28 (1):93–99. doi:10.1016/j.geotexmem.2009.09.017.
- Ahmadi, H., and O. Shafiee. 2019. Experimental comparative study on the performance of nano-SiO2 and microsilica in stabilization of clay. European Physical Journal Plus 134 (9):459. doi:10.1140/epjp/i2019-12918-1.
- ASTM D2166. 2006. Standard test method for unconfined compressive strength of cohesive soil. West Conshohocken, Pennsylvania, USA: ASTM International.
- ASTM D2974. 2020. Standard test methods for determining the water (moisture) content, ash content, and organic material of peat and other organic soils. West Conshohocken, Pennsylvania, USA: ASTM International.
- ASTM D5856. 2015. Standard test method for measurement of hydraulic conductivity of porous material using a rigid-wall, compaction-mold permeameter. West Conshohocken, Pennsylvania, USA: ASTM International.
- ASTM D698. 2012. Standard test methods for laboratory compaction characteristics of soil using standard effort (12,400 ft-lbf/ft3 (600 kN-m/m3)). West Conshohocken, Pennsylvania, USA: ASTM International.
- Barcelo, L., J. Kline, G. Walenta, and E. Gartner. 2014. Cement and carbon emissions. Materials and Structures 47 (6):1055–65. doi:10.1617/s11527-013-0114-5.
- Bawadi, N. F., M. A. A. Alhamidi, A. F. Mansor, and S. A. Anuar. 2020. Influence of banana fiber on shear strength of clay soil. IOP Conference Series: Materials Science and Engineering 864: 012099.
- Bharathi, S. V., S. Vinodhkumar, and M. M. Saravanan. 2021. Strength characteristics of banana and sisal fiber reinforced composites. IOP Conference Series: Materials Science and Engineering1055: 012024.
- Boz, A., A. Sezer, T. Özdemir, G. E. Hızal, and Ö. A. Dolmacı. 2018. Mechanical properties of lime-treated clay reinforced with different types of randomly distributed fibers. Arabian Journal of Geosciences 11 (6):122. doi:10.1007/s12517-018-3458-x.
- Changizi, F., and A. Haddad. 2015. Strength properties of soft clay treated with mixture of nano-SiO2 and recycled polyester fiber. Journal of Rock Mechanics and Geotechnical Engineering 7 (4):367–78. doi:10.1016/j.jrmge.2015.03.013.
- Changizi, F., and A. Haddad. 2017. Improving the geotechnical properties of soft clay with nano-silica particles. Proceedings of the Institution of Civil Engineers: Ground Improvement 170 (2):62–71. doi:10.1680/jgrim.15.00026.
- Choobbasti, A. J., M. A. Samakoosh, and S. S. Kutanaei. 2019. Mechanical properties soil stabilized with nano calcium carbonate and reinforced with carpet waste fibers. Construction and Building Materials 211:1094–104. doi:10.1016/j.conbuildmat.2019.03.306.
- Cui, H., Z. Jin, X. Bao, W. Tang, and B. Dong. 2018. Effect of carbon fiber and nanosilica on shear properties of silty soil and the mechanisms. Construction and Building Materials 189:286–95. doi:10.1016/j.conbuildmat.2018.08.181.
- Divya, P. V., B. V. S. Viswanadham, and J. P. Gourc. 2018. Hydraulic conductivity behaviour of soil blended with geofiber inclusions. Geotextiles and Geomembranes 46 (2):121–30. doi:10.1016/j.geotexmem.2017.10.008.
- Esawi, A. M. K., and M. M. Farag. 2007. Carbon nanotube reinforced composites: Potential and current challenges. Materials & design 28 (9):2394–401. doi:10.1016/j.matdes.2006.09.022.
- Estabragh, A. R., S. Ranjbari, and A. A. Javadi. 2017. Properties of a clay soil and soil cement reinforced with polypropylene fibers. ACI Materials Journal 114 (2):195–205. doi:10.14359/51689469.
- Gao, L., G. Hu, N. Xu, J. Fu, C. Xiang, and C. Yang. 2015. Experimental study on unconfined compressive strength of basalt fiber reinforced clay soil. Advances in Materials Science and Engineering 2015:561293. doi:10.1155/2015/561293.
- Gobinath, R., I. I. Akinwumi, O. D. Afolayan, S. Karthikeyan, M. Manojkumar, S. Gowtham, and A. Manikandan. 2020. Banana fibre-reinforcement of a soil stabilized with sodium silicate. Silicon 12 (2):357–63. doi:10.1007/s12633-019-00124-6.
- Jasim, O. H., and D. Cetin. 2016. Effect of the usage of sawdust and quicklime on shear strength behaviour of clayey silt soil. Sigma Journal Engineering and Natural Sciences 34 (1):31–41.
- Kannan, G., and E. R. Sujatha. 2022. Geotechnical behaviour of nano-silica stabilized organic soil. Geomechanics and Engineering 28 (3):239–53.
- Khan, M., S. Rahamathbaba, M. A. Mateen, D. V. R. Shankar, and M. M. Hussain. 2019. Effect of NaOH treatment on mechanical strength of banana/epoxy laminates. Polymers from Renewable Resources 10 (1–3):19–26. doi:10.1177/2041247919863626.
- Latifi, N., S. Horpibulsuk, C. L. Meehan, M. Z. A. Majid, and A. S. A. Rashid. 2016. Xanthan gum biopolymer: An eco-friendly additive for stabilization of tropical organic peat. Environmental Earth Sciences 75 (9):825. doi:10.1007/s12665-016-5643-0.
- Lv, Q., C. Chang, B. Zhao, and B. Ma. 2018. Loess soil stabilization by means of SiO2 nanoparticles. Soil Mechanics and Foundation Engineering 54 (6):409–13. doi:10.1007/s11204-018-9488-2.
- Maher, M. H., and D. H. Gray. 1990. Static response of sands reinforced with randomly distributed fibers. Journal of Geotechnical Engineering 116 (11):1661–77. doi:10.1061/(ASCE)0733-9410(1990)116:11(1661).
- Mirzababaei, M., J. Karimiazar, E. S. Teshnizi, R. Arjmandzadeh, and S. H. Bahmani. 2021. Effect of nano-additives on the strength and durability characteristics of marl. Minerals 11 (10):1119. doi:10.3390/min11101119.
- Modha, H., N. Sharma, and H. Chavda. 2020. Shear strength parameters of soil and banana fiber mix. In Technologies for Sustainable Development, A. Mahajan, P. Patel, and P. Sharma ed., 1st ed., 101–105. London: CRC Press.
- Mohan, N., S. Vasudevan, P. C. Ranganathan, and A. Narayanasamy. 2021. Geochemical and elemental characterization of rostrum and alveolus parts of belemnite fossil from the late cretaceous formation, Tamil Nadu, India. Arabian Journal of Geosciences 14 (18):1905. doi:10.1007/s12517-021-08272-z.
- Murthi, P., R. Saravanan, and K. Poongodi. 2020. Studies on the impact of polypropylene and silica fume blended combination on the material behaviour of black cotton soil. Materials Today: Proceedings 39 (1):621–26. doi:10.1016/j.matpr.2020.09.004.
- Nath, B. D., M. K. A. Molla, and G. Sarkar. 2017. Study on strength behavior of organic soil stabilized with fly ash. International Scholarly Research Notices 2017:5786541. doi:10.1155/2017/5786541.
- Pastor, J. L., R. Tomás, M. Cano, A. Riquelme, and E. Gutiérrez. 2019. Evaluation of the improvement effect of limestone powder waste in the stabilization of swelling clayey soil. Sustainability 11 (3):679. doi:10.3390/su11030679.
- Paul, A., and M. Hussain. 2020. An experiential investigation on the compressibility behavior of cement-treated Indian peat. Bulletin of Engineering Geology and the Environment 79 (3):1471–85. doi:10.1007/s10064-019-01623-x.
- Puppala, A., L. Hoyos, C. Viyanant, and C. Musenda. 2000. Fiber and fly ash stabilization methods to treat soft expansive soils. In Proceedings of the Soft Ground Technology Conference 2004, ed. J. L. Hanson and R. J. Termaat, 136–145. ASCE.
- Randhawa, K. S., R. Chauhan, and R. Kumar. 2021. An investigation on the effect of lime addition on ucs of Indian black cotton soil. Materials Today: Proceedings 50 (5):797–803. doi:10.1016/j.matpr.2021.05.586.
- Soltani, A., A. Deng, and A. Taheri. 2018. Swell–compression characteristics of a fiber–reinforced expansive soil. Geotextiles and Geomembranes 46 (2):183–89. doi:10.1016/j.geotexmem.2017.11.009.
- Sujatha, E. R., P. Atchaya, S. Darshan, and S. Subhashini. 2020. Mechanical properties of glass fibre reinforced soil and its application as subgrade reinforcement. Road Materials and Pavement Design 22 (1):2384–95. doi:10.1080/14680629.2020.1746387.
- Sujatha, E. R., A. R. Geetha, R. Jananee, and S. R. Karunya. 2018. Strength and mechanical behaviour of coir reinforced lime stabilized soil. Geomechanics and Engineering 16 (6):627–34.
- Taha, M. R., and O. M. E. Taha. 2012. Influence of nano-material on the expansive and shrinkage soil behavior. Journal of Nanoparticle Research 14 (10):1190. doi:10.1007/s11051-012-1190-0.
- Tinti, A., V. Tugnoli, S. Bonora, and O. Francioso. 2015. Recent applications of vibrational mid-infrared (IR) spectroscopy for studying soil components: A review. Journal of Central European Agriculture 16 (1):1–22. doi:10.5513/JCEA01/16.1.1535.
- Vakili, A. H., S. I. Shojaei, M. Salimi, M. R. B. Selamat, and M. S. Farhadi. 2020. Contact erosional behaviour of foundation of pavement embankment constructed with nanosilica-treated dispersive soils. Soils and Foundations 60 (1):167–78. doi:10.1016/j.sandf.2020.02.001.
- Wang, Y., P. Guo, X. Li, H. Lin, Y. Liu, and H. Yuan. 2019. Behavior of fiber-reinforced and lime-stabilized clayey soil in triaxial tests. Applied Sciences 9 (5):900. doi:10.3390/app9050900.
- Yixian, W., G. Panpan, S. Shengbiao, Y. Haiping, and Y. Binxiang. 2016. Study on strength influence mechanism of fiber-reinforced expansive soil using jute. Geotechnical and Geological Engineering 34 (4):1079–88. doi:10.1007/s10706-016-0028-4.
- Zhao, N., H. Wu, and Z. Huang. 2021. Strength behavior of red clay reinforced by basalt chopped fiber. Arabian Journal of Geosciences 14 (1):15. doi:10.1007/s12517-020-06275-w.