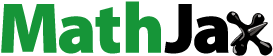
ABSTRACT
The continuous basalt fibers (BFs) dyed with cationic dyes (RhB) were prepared through one-step drawing strategy using miscible liquids of phosphorylating starch (PS) cross-linked polyvinyl alcohol (PVA) and sodium silicate (SS) as infiltration agents. The new method of dyeing was relied on the residual temperature of melt drawing, and the dye molecules were lubricated onto fibers firmly. Different means were used to characterize the color fibers, and a compact layer was formed on the fiber surface. The prepared composite fibers (PVA-SS-PS-RhB/BF) exhibited stable color fastness (washing and rubbing fastness achieved to 4–5 grade). The crosslinking significantly improved the water resistance with dissolved residue up to 80%, and the heightened glass transition temperature was observed. The breaking force of dyed fibers was observed to be 50.3% higher than that of raw fibers, and the breaking elongation was 71.8% higher than the original fibers.
摘要
采用磷酸化淀粉(PS)交联聚乙烯醇(PVA)和硅酸钠(SS)的混溶液体作为渗透剂,通过一步拉伸策略制备了阳离子染料(RhB)染色的连续玄武岩纤维(BFs). 新的染色方法依赖于熔融拉伸的残余温度,染料分子牢固地润滑在纤维上. 使用不同的方法表征彩色纤维,并在纤维表面形成致密层. 制备的复合纤维(PVA SS PS RhB/BF)表现出稳定的色牢度(洗涤和摩擦牢度达到4-5级). 交联显著提高了耐水性,溶解残留物达到约80%,并观察到玻璃化转变温度升高. 观察到染色纤维的断裂力比原纤维高50.3%,断裂伸长率比原始纤维高71.8%.
Introduction
Due to the human’s awareness of healthy, safe and comfortable life pressurizes growing demands for colorful decoration fiber/cloth in textile manufactures (Korkmaz, Nazife, and Yılmaz Citation2018). Inorganic fibers, e.g., continuous basalt fibers (BFs), have entered vision of scientific researchers’ because of their brilliant strength and environmental friendliness (Mishukova, Safonov, and Apyari Citation2020). The BFs were produced by drawing molten basalt rocks that were heated at 1400–1500ºC. Different from asbestos the BFs were not breathed into organisms due to the long aspect ratios, and the BFs have been accepted as “green industrial material for the 21th century.” This definition reveals sustainability, point of growing interest in new applications such as clothes, phone case and upholstery, etc (Chuvashov et al. Citation2020). Hence, there is an urgent task to produce colored yarns of BFs.
The existing dyeing technologies have been dedicated to post-dyeing of a series of organic fibers, such as liquid paraffin, supercritical carbon dioxide, and organic solvent dyeing methods (Cardozo-Filho et al. Citation2014; Xu et al. Citation2016). Among these approaches complicated physical and chemical modifications were always carried out at low temperatures by following an ex-situ way. For example, the utilizing UV radiation, plasma, ionic liquids, ammonia and enzyme have been developed to pretreat fibers (Chen et al. Citation2018; Panda et al. Citation2012; Periolatto et al. Citation2010). These treatments posed great burden on energy consumption, and demanded tedious procedures as well as damaged the structural integrity of fibers. For dyeing inorganic fibers, most of existing strategies were proposed to dope organic dye ingredients in starting materials, and the colored fibers were drawn from the liquid/melt directly. In case of glass fibers the high-temperature treatment would decompose dye ingredients, or the dye components would deteriorate vitrification of glass melt, and the filamentation was impossible (Selim et al. Citation2019). It was known that the BFs were similar to glass fibers (Toropina et al. Citation1995), however the production of BFs was more stringently controlled (Chen Citation1997). The present hindrances in dyeing BFs were found as: (1) the glassy surface was less prone to absorption and diffusion of dyes into interior of BFs, (2) the inorganic fibers lack of functional groups for immobilizing dye molecules, (3) the inherent golden BFs would hide colors of dye molecules, and (4) the high glass transition temperature at around 700ºC would burn dye molecules if one applied organic molecules (Demir et al. Citation2010). Considering perspective of energy-saving, cost-effects as well as the state-of-art, we propose a drawing-remained temperature dyeing method for in-situ lubricating BFs fibers as a sizing agent, i.e., the dye molecules were grafted on BFs during wire drawing.
The phosphorylating starch (PS) cross-linked with polyvinyl alcohol (PVA) was a kind of sizing agent for modifying inorganic fibers that comprised with variety of functional groups (Ralph et al. Citation2019). This composite coating offered fibers with excellent film-forming capability, better tensile strength, flexibility and resistance to oxidation, oil and/or solvent treatments (Sun Citation2003). It was known that the composite colored BFs displayed disadvantages of low water resistance which would lead to poor color fastness (Wang et al. Citation2015). To overcome these drawbacks, the PVA molecules were blended with other inorganic crosslinking agents. Sodium silicate (SS) was a thermal-resistant, water-soluble, non-environmental hazard, and was able to form inorganic silicon networks (El-Sayed et al. Citation2015). In this strategy the cross-linking between SS and PVA through hydrolytic polymerization would consume hydroxyl groups (−OH), and the less numbered -OH would improve hydrophobicity with assistance of phosphoric acid using as “catalyst.” Herein, we reported a prototype design of colored BFs through grafting Rhodamine B (RhB) using PS, PVA and SS as cross-linkers. The obtained sample was called PVA-SS-PS-RhB/BF. The composite fibers were prepared at a certain temperature (280ºC) using one-step drawing method to lubricate raw BFs with mixtures of PVA-SS-PS-RhB (coating reagents) to form a colored film on the fiber. The water stability, mechanical properties and color fastness were investigated. This strategy would envisage practical applications for colored BFs.
Experimental methods
Materials
The BFs were prepared on a lab-scale 16-spinneret drawing equipment. Chemicals including PVA, SS, phosphoric acid and soluble starches (PS) were purchased from Aladdin Shanghai Co., Ltd. Rhodamine B (RhB) of analytical purity was purchased from Sinopharm.
Preparation of PVA-SS-PS-RhB/BF
To prepare dye wetting agents (PVA-SS-PS-RhB), 2.00 g of PVA was dissolved in 50.0 mL deionized water at 95°C, and the solution was then mixed with 1.00 g SS solution in 50.0 mL water after the temperature was cooled to 50°C (PVA-SS). To prepare solution of PS, 4.00 g starch was dissolved in 50.0 mL deionized water (95°C) followed by adding 0.16 mol phosphoric acid, and the PS solution was obtained after vigorous stirring for 30 min. The above two sorts of solutions were mixed by adding certain amounts of RhB. Mixture were stirred for 2.0 hrs at room temperature to get PVA-SS-PS-RhB wetting agents. The synthetic diagram was shown in .
Scheme 1. The schematic preparation of dye lubricant (sizing agent) of PVA-SS-PS-RhB and reaction mechanism.
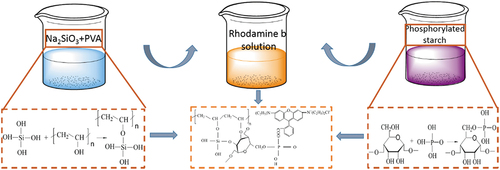
The controlled samples including PS-RhB/BF and PVA-PS-RhB/BF were prepared by applying one- or two-component reagents with presence of RhB. Typically, the prepared PVA-SS-PS-RhB was used as a sizing agent for BFs lubrication during the drawing procedure (the temperature was 280ºC which was determined by a digital infrared temperature sensor (FT-H40K, KEYENCE). The drawing art of BFs from melted basalt rocks can be found in our previous publication (Si et al. Citation2021). The final fibers were dried at 105ºC temperatures to obtain colored BFs. This protocol was illustrated in , and the experiment video (see Video S1 in supporting information, SI) was provided.
Material characterizations
The phase identification was examined by X-ray diffraction (×RD) with Cu-Kα radiation (λ = 1.5418 Å), and the scanning speed was 8º/min within 2θ = 5°-80°. The Fourier transform infrared (FTIR) spectra were recorded within wavenumber of 400 1200 cm −1, and the tested sample was mixed with KBr and crushed into a wafer. The X-ray photoelectron spectra (×PS) were recorded on a Thermo ESCALAB 250XI spectrometer using an Mg/Al anode as an X-ray source. Scanning electron microscopy (SEM) analysis was performed on an Hitachi S-4700 microscope to evaluate coating layers and microstructures. The tensile strength of BFs was measured on an Hounsfield H100K-S universal testing machine. The thermal stability and crystallization properties of fibers were studied by differential scanning calorimetric (DSC) measurement on a STA Jupiter 449C (Netzsch) equipped with a high temperature furnace (t = 40 750ºC; heating rates: 5 ºC/min). The thermogravimetric (TG) analysis was performed on an HENVEN HCT-3 apparatus from 30 to 600ºC under nitrogen atmosphere with a flow rate of 40 mL/min. The heating rate was 10 ºC/min. The color measurements were performed within wavelength of 400 700 nm under D65/10° illuminant using a TU-1901 dual beam UV-Vis reflectance spectrophotometer.
K/S value measurement
The absorbance/scattering (K/S) value represents color strength of the dyed fabrics, and the quantification was calculated based on the Kubelka – Munk equation: K/S=(1-R)2/2R, where K was the absorbance coefficient, S was the scattering coefficient, and R referred to reflectance of dyed fabrics measured from a color spectrophotometer.
Color fastness testing
The washing fastness and rubbing fastness were tested according to standards of GB/T3921–2008 (eqv. ISO 105-C10: 2006) and GB/T 3920–2008 (eqv. ISO 105-X12: 2001) (Wang, Herricks, and Xia Citation2003).
Measurement of dye uptake (E%)
The absorbance of diluted dye solution was measured at the maximum wavelength (λmax) of dye (RhB) using a UV-Visible spectrophotometer (SHIMADZUUV2700). The uptake of dye molecules (E%) was evaluated according to equation: E%=(A0-A1)/A0 ×100%, where A0 and A1 are the absorbance values of the dye-bath before and after the dyeing process.
Surface energy calculation
The surface energy of films was studied by measuring the contact angles. Water and diiodomethane were used as the probe liquids. The titration was a static drop method, and the liquid volume was 1.0 μl. The surface energy γs was evaluated based on equation of Owens-Wendt geometric as followed:
where γsd (dispersion component) and γsp (polar component) were determined by contact angle and using two sets of data with two known liquids (water and diiodomethane), and parameters were listed in Wang et al. Citation2020).
Table 1. Parameters of the tested liquids at room temperature (25ºC) which were used to evaluate the surface energy γs.
Residue rate of hydrolysis (R%)
The water resistance evaluation of PVA-SS-PS-RhB film was expressed by residuals of dissolution. The colored fibers were immersed in 50ºC water for 2 hours, and were taken out followed by washing thoroughly, and then were dried to constant weight. The dissolution residual was used to evaluate properties of water resistance. The calculation formula was: R (%) =M1/M2 ×100% where M1 was the original mass (g) of fibers, and M2 was the mass (g) of fibers after water immersion and drying.
Tensile strength measurements
The tensile strength of dyed fibers was measured on a Hounsfield H100K-S universal testing machine. Each batch of samples were tested for 50 times from arbitrarily chosen 50 pieces of fibers. A stretching speed 20 mm/min was employed on stretching these fibers, and the tested accuracy was ±0.1 cN. The average value was recorded as the tensile strength. Methods of the tensile strength measurements were performed according to ISO 11,566:1996 “Carbon fiber-determination of the tensile properties of single-filament specimens.”
Loss on ignition (LoI)
Based on the Chinese standard GB/T 29,761–2013, the temperature of 450ºC was chosen to decompose sizing agents in N2 atmosphere, and this treatment would not damage the fiber structure. The initial mass (M0) of each sample was 2.000 g, and the mass after burning was M1. The calculation formula of LoI was: LoI (%) =(M0-M1)/M0.
Results and discussions
Morphology analysis
Photographs of the raw fibers and dyed PVA-SS-PS-RhB/BF fibrous bundles were shown in , respectively. Different from the golden colored BFs, the composite fibers appeared as purplish red with metallic luster after infiltrating with PVA-SS-PS-RhB, suggesting the successful dyeing of BFs.
Figure 1. Photographs of the fibrous bundle of raw BFs (a) and composite fibers PVA-SS- PS-RhB/BF (b).
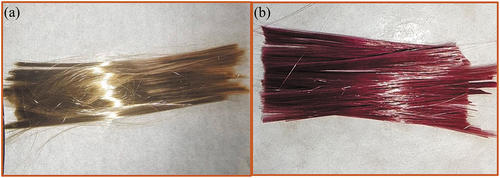
showed the SEM topographies of raw fibers (BF) and colored fibers of PS-RhB/BF, PVA-PS-RhB/BF and PVA-SS-PS-RhB/BF. As seen from , the raw BFs possessed a smooth surface, and no grooves or defects were observed on the surface. The PS-RhB/BF displayed a rougher surface after coating with PS, and some colloidal particles (starch) were directly exposed on fibers (see inset ). Since there were no “protective layers,” it would lead to a possible loss of color fastness when encountered with an aqueous environment. showed that starch particles on the surface of PVA-PS-RhB/BF were fully wrapped by the PVA layer, which would improve the mechanical properties of fibers with color fastness. In the PVA-SS-PS-RhB/BF displayed much denser surface coatings, and a uniform layer was observed. Due to the introduction of Si-O-C bonds the composite fibers were expected to have improved water resistance and temperature stability when SS was used.
Dye uptake
For method of direct dyeing in the one-step drawing process, the most important factor affecting the dyeing uptake would be the flow rate (amount of dyes solution spread on fibers per unit of time) of the dyestuff (sizing agent). showed the absorbance and dyeing uptake before and after dyeing at different flow velocities of PS-RhB, PVA-PS-RhB and PVA-SS-PS-RhB. According to higher flow velocity was found to have higher dyeing uptake, which suggested more dyes grafted onto the BFs. Comparing the three types of sizing, the PVA-SS-PS-RhB was found to have the highest dyeing uptake when an equal flow rate was employed to lubricate BFs. The PVA-PS-RhB ranked the second (). The experimental results indicated that the addition of PVA introduced more hydroxyl groups to react with RhB carboxyl groups and promoted the grafting of dye molecules. In addition, the dye coating was much denser due to the cross-linking effect between SS and PVA, which was validated by the larger LoI for sample PVA-SS-PS-RhB/BF as shown in Table S1 (see the SI). With exceeding the flow rate from 10.0 mL/min to 15.0 mL/min, the dyeing uptake was found to increase suddenly, i.e., the absorbance increased at the wavelength of 554 nm. In this one-step drawing the dye was not fully grafted to the fiber surface, and certain amount of dye lubricant solutions was thrown away by the high-speed of fiber drawing. Therefore, the flow rate of 10.0 mL/min was a better choice, and the efficiency of dyeing uptake was 63.0% with respect to the initial amount of dye molecules.
Figure 3. (A c) the UV-Visible spectra of PS-RhB (a), PVA-PS-RhB (b) and PVA-SS-PS-RhB (c); (d) Percentages of dyeing uptake evaluated based on the absorbance at λmax = 555 nm.
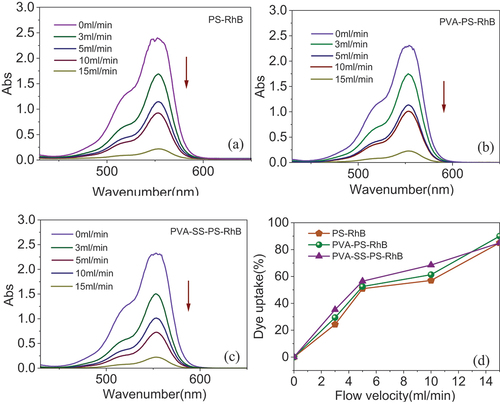
The absorbance/scattering (K/S) values are inversely proportional to reflectance of dyed material. The reflectivity and K/S values of PS-RhB/BF, PVA-PS-RhB/BF, and PVA-SS-PS-RhB/BF at different flow velocities were given in . For the method of directly one-step dyeing, the increase of flow velocities was positive to enhance the dyeing depth, but excessive speed had almost no obvious influences on K/S values (). The reason was that the wetting agent could not infiltrate the fiber effectively with uneven coating when the dye flow velocities were too slow, and this was particularly prominent for dyeing with solution of PS-RhB/BF (seen from inset topography images of fiber bundles in ). The K/S values of PVA-PS-RhB/BF were higher than that of PS-RhB/BF but lower than PVA-SS-PS-RhB/BF (). Exception for addition of phosphoric acid, the agent PVA had a positive effect in grafting RhB dye, and the incorporation of SS has cross-linking effect with PVA to form network structure, which further improves the dyeing depth. While the drying temperature and dye concentration effect on color fastness of PVA-SS-PS-RhB/BF were also studied and demonstrated in Figure S1 (see the supporting information, SI). The increase of drying temperature would promote penetration of cationic dyes through the fiber surface defects into the interior (Figure S1a), however the dyeing depth will not be increased and result in decomposition of dyes when the temperature was higher (Figure S1c). The K/S values tended to balance (Figure S1b, S1d) with increasing dye concentration that could react with carboxyl groups, and the number of chromophores was reflected by the dye depth (Cardozo-Filho et al. Citation2014).
Surface contact angle and surface energy
Advancing the contact angles of PS-RhB, PVA-PS-RhB and PVA-SS-PS-RhB, measurements were performed in water and diiodomethane, respectively. The contact angles were displayed in and surface energy was provided in . As can be seen, the wetting angle of PVA-PS-RhB dyed films increased a little bit, and the surface energy exhibited a slight decrement by 1.0 mN/m by comparison with PS-RhB. This could be reasoned by the fact that the starch and PVA tend to form strong hydrogen bonds, and the new bonds would result in synergistic stability and better compatibility (Zhang et al. Citation2011). The contact angle increased to 68.33° (), suggesting the surface energy declined after modification with SS. The crosslinking reaction between PVA and SS formed a large number of hydrogen bonds and Si-O-C cross networking structure, which would lead to declined number of hydroxyl groups. This indication was proved in the infrared spectrum. This can be also explained by the relatively lower dispersive components and the larger polar components. Meanwhile, It was well known that both low surface energy and surface roughness were beneficial for a hydrophobic surface (Li et al. Citation2018). Therefore, the PVA cross-linked phosphorylated starch modified by SS had a positive promotion on the water resistance of dyed fibers.
Table 2. Surface contact angle and surface energy of different film samples.
Water resistance or dissolution residue
It was well known that the hydrolysis of dye could influence the fixation of dye chromophores (Pei, Gu, and Wang Citation2021). The water resistance tests were carried out by immersing films of PS-RhB, PVA-PS-RhB, and PVA-SS-PS-RhB into water at different temperatures, and the residue mass of films was measured prior to being dried to constant weight. As shown in , the percentage of dissolved residue of PVA-SS-PS-RhB film was higher than that of PVA-PS-RhB and PS-RhB. According to the reflectance in , it was clearly to see that the dyeing depth of PVA-SS-PS-RhB/BF changed slightly before and after water dissolution, while the K/S of PVA-PS-RhB/BF and PS-RhB/BF changed enormously. This means huge amount of dye molecules were lost during the washing. The result demonstrated that SS promoted the water resistance of PVA positively. Based on the infrared characterization, we found that a new chemical bond Si-O-C was constructed between PVA and SS through cross-linking. The cross-linking not only increased strength of films but also creates a more dense structure. This prevented the swelling of starch and decreased the accessible regions, i.e., the non-cross-linked leading to an improvement in the resistance to dissolution. The cross-linking or the esterification reactions were able to reinforce the starch network both chemically and physically, resulting in lower water solubility.
Figure 6. (a) Dissolution residual percentages of PS-RhB, PVA-PS-RhB, and PVA-SS-PS-RhB films: (b) the IR spectra showing characteristic peak at 560 nm for samples of PS-RhB/BF, PVA-PS-RhB/BF, and PVA-SS-PS-RhB/BF films that were measured before and after water dissolution, demonstrating the dyeing depth.
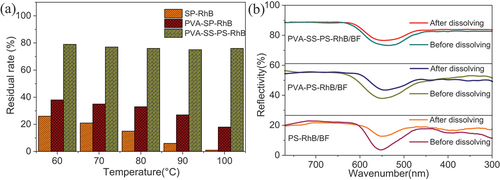
Color fastness testing
It could be seen from that samples modified by SS showed better color stiffness after rubbing/wash fastness in contrast to PS-RhB/BF and PVA-PS-RhB/BF samples. This was more prominent for samples treated with PVA merely, showing significantly lower color difference values for wash and fastness tests in comparison with samples treated with PVA-SS. The gray scale evaluation of change of shade was found to be very good (4–5 or 5 grade) in water system of PVA-SS-PS-RhB/BF and the color was almost not changed after twice washing (Figure S2). Moreover, the other two samples suffered from fading phenomenon after three and four times of washing. Similarly, the dry and wet rubbing fastness of the samples modified by PVA-SS were found to remain prominent colorful (4–5 grade). Therefore, the experimental results showed that the fiber fastness properties would not be affected by direct dyeing with dyestuff as an infiltration agent. The product quality was fully compatible to high level of color fastness.
Table 3. Color fastness of the prepared samples PS-RhB/BF, PVA-PS-RhB/BF and PVA-SS-PS-RhB/BF.
Tensile strength measurements
To investigate influences of dye lubrication on the mechanical properties of BFs, the tensile strength and elongation were measured, and results were shown in , respectively. It was seen that the modification of phosphorylated starch dyeing solution and PVA-PS-RhB dyeing solution could improve the strength and elongation (). The PVA dyeing was observed to be more prominent in improving the mechanical property, i.e., strength increment by 48%, and elongation by 68% (see the average value). The experimental results suggested that the tensile strength of PVA-SS-PS-RhB/BF was the best with 50.3% promotion. Except for this, the modulus of fibers coated with dye was reduced and facilitated the toughness. This effect could be more prominent by the PVA coatings that decreased elastic modulus of the modified fibers (see the Table S2). The effect of SS on elastic modulus was not obvious. This was consistent with the trend of tensile strength. Reasons were summarized as: (1) phosphorylation impeded starch retrogradation under lower temperature, enhancing the adhesion of starch to fiber substrates, (2) the starch and PVA tended to form strong hydrogen bonds with each other and increased density and thickness of the layered network, (3) the phosphoric acid acted as a catalyst for the cross-linking of PVA and SS, and (4) PVA molecular chains can align highly oriented in the fiber axis, resulting in a multi-dimensional spatial network which afforded the conversion from van der Waals force to chemical bonding (Onggar et al. Citation2016). This facilitation confirmed no adverse effect on their integrity and mechanical properties. Evidences can be drawn from the following IR and XPS analyses.
Mechanism indicated
The FTIR spectra of PS-RhB/BF, PVA-PS-RhB/BF, PVA-SS-PS-RhB/BF and raw BFs were shown in . The bending vibration in BFs appearing at 463 cm−1 should be attributed to the coupling band of Si-O-Si (Al) bending vibration, but bands in other samples at 493 cm−1 appeared, indicating these samples contained P-O bond, and this observation proved the success of incorporation of phosphoric acid (Destainville et al. Citation2003). The characteristic peak of the three colored samples at 875 cm−1 was attributed to the presence of ester bonds, but this band shifted to a lower wavelength of PVA-PS-RhB/BF and PVA-SS-PS-RhB. This result showed that the cross-linking between PVA and starch afforded intermolecular esters formation. A wide band appears at 3400–3500 cm−1 and 1386 cm−1 corresponding to -OH bond stretching and bending vibrations, respectively (Sun et al. Citation2019). Surprisingly, compared to raw BFs the hydroxyl band of PS-RhB/BF decreased due to the phosphorylated starch bound to hydroxyl group. For PVA-PS-RhB/BF, the mode showed an increased trend because of the addition of PVA providing more hydroxyl groups. Furthermore, the – OH of color fibers modified by SS was weakened again, which suggested the crosslinking reaction between SS and PVA. The stretching vibration of Si-O-C bond around 1187 cm−1 only appeared in PVA-SS-PS-RhB, which further proved the above conclusion (Tee et al. Citation2013). Only the two samples of PVA-PS-RhB/BF and PVA-SS-PS-RhB/BF appeared the band at 1020 cm−1 corresponding to C-N stretching vibrations due to the incorporation of RhB (Sarkodie and Zhu Citation2018; Sugiura et al. Citation2001). This fully proved that the addition of PVA was able to make Rhodamine dye molecules grafted firmly and improved the color fastness (Idan et al. Citation2017). It was also observed that PS-RhB/BF exhibited a C=O stretching band at 1724 cm−1 and further red-shifted after adding PVA and SS, e.g., the PVA-PS-RhB/BF and PVA-SS-PS-RhB/BF samples, which indicated more C=O bond was generated(Zahed and Monfared Citation2015). The stretching vibration band of -CH2 appeared at 2930 cm−1 of BFs due to adventitious contamination (Kang et al. Citation2007). The decrease of -CH2 in PVA-SS-PS-RhB/BF compared to samples PVA-PS-RhB/BF, which originated from the starch cross-linked with PVA. Therefore, the infrared test results not only proved the dye was successfully grafted on the surface of BF in the form of sizing agent, but also validated the fact that cross-linked structure of PVA and SS PVA anchored more dyes.
The surface chemical composition of BFs with and without dyeing was investigated. The full survey XPS spectra of BF and PVA-SS-PS-RhB/BF were displayed in , revealing the dyed fiber had more peaks of N and P elements. presented the high-resolution XPS profile of C1s and their deconvoluted bands in samples of the dyed/undyed BFs. The C1s of BF sample appeared at 284.4 eV, 286.3 eV, and 286.95 eV were ascribed to C – C (Ramezanzadeh et al. Citation2020) from adventitious contamination, C – OH and O – C–O from deconvoluted peaks, respectively (Nakatani et al. Citation2011). Compared to raw BFs, the C1s peak (285.65 eV) in PVA-SS-PS-RhB/BF was more prominent, and small concentration of nitrogen could be distinguished. The fitted peak situated at 284.1 eV represented C-Si groups, and two other peaks were located at 285.65 eV and 286.75 eV ascribing to C-N (Ramezanzadeh et al. Citation2020) and -C=O groups, which revealed the presence of three different types of carbon atoms (Yuan et al. Citation2017). The characteristic C-OH peak disappeared, and this was just in consistence with the IR and XRD results. Furthermore, the high-resolution spectra of Si, O, P, and N elements were scanned for detailed analysis (). shows the Si2p spectrum of BF, which was deconvoluted into three peaks. Peaks at 101.82 eV, 102.40 eV, and 102.95 eV were related to [SiO4]4- (tetrahedron structure), [Si2O5]2- (layer structure), and [Si2O6]4- (chain structure) of the BF surface () (Wang et al. Citation2007). Similarly, peaks of Si2p could also be found in PVA-SS-PS-RhB/BF sample, but the content of Si-OH seemed to be decreased and the Si-O-Si increased due to the evaluation of integrated areas, which demonstrated the fiber surface was modified with inorganic Si – OH groups with presence of SS, and the organic-OH was also formed by linking with phosphate groups. Two peaks could be deconvoluted in the O1s spectrum of BFs as shown in . The peak located at 532.75 eV was assigned to nonbridging oxygen, and the other presented at 533.40 eV was attributed to the bridging oxygen of silicate structure in BFs (Calas, Henderson, and Stebbins Citation2006). Different from the peak at 531.59 eV related to non-bridging oxygen of O=P or O=C bond of PVA-SS-PS-RhB/BF. A new peak assigned to single bonded oxygen in the C-O-H function was observed, typically in the phosphorylated starch fibers. (Shi et al. Citation2014). Compared to the raw BFs, the non-bridging oxygen showed notable decline in intensity and a slight shift to a lower BE value, confirming the successful grafting of composite coating to BFs (Shi et al. Citation2014). According to the P2p in of PVA-SS-PS-RhB/BF, the deconvoluted band centered at 133.31 eV was attributed to the pentavalent phosphorus (PO4 tetrahedron, phosphate-like structure) (Elkins et al. Citation2003). A new peak assigned to single bonded oxygen in P-O-H appeared in the phosphorylated fibers confirming the chemical grafting of phosphate to BFs. The N1s spectrum of PVA-SS-PS-RhB/BF in was fitted into two distinct peaks, and these two peaks at 401.89 eV and 399.768 eV were assigned to C – N and N – H bonds (Ramezanzadeh et al. Citation2020), which demonstrated the successful bonding of RhB. The N – H (band at 1513 cm−1 in FTIR profile) appeared as a result of bonding between phosphoric acid and amino groups (-NR2) of RhB.
Figure 9. (a) Full-survey XPS profile of PVA-SS-PS-RhB/BF and BF (a), the corresponding high-resolution XPS spectra of C1s (b), Si2p (c), O1s (d), N1s (e), and P2p (f).
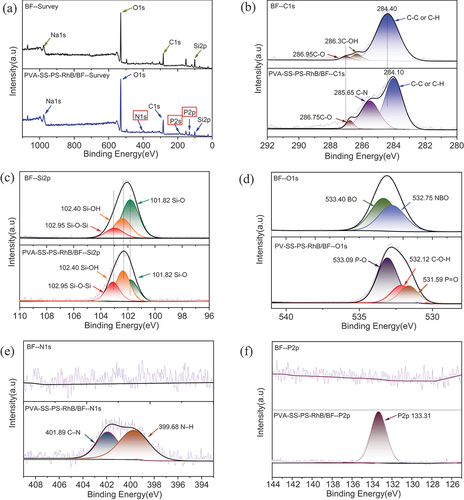
To further demonstrate phase of PVA and SS coated fibers, the XRD were further characterized on pastes of PS-RhB, PVA-SS-PS-RhB and PVA-PS-RhB, as shown in . Typical peaks at 2θ = 16.9°, 31.2° and 34.8° were indexed to starch (PDF: 46–1421) embedded in sample PS-RhB. When PVA was added into the PS-RhB system, two peaks at 2θ = 31.2° and 34.8° in PVA-PS-RhB disappeared, and the relative intensity of peak 2θ = 16.9° was greatly weakened. This weakened diffraction was attributed to the fact that PVA formed a strong hydrogen bond with starch, and would decrease crystallinity of starch. We also observed a sharp peak at 2θ = 19.6° for PVA-PS-RhB that originated from the reacted PVA (PDF21–0952) with orderly arrangement and chemical binding of hydroxyl groups. The crystallinity of PVA-PS-RhB was evaluated to be 54% by comparison with PS-RhB, while the crystallinity of PVA-SS-PS-RhB film was 46%. The binding forces and arrangement of macromolecules was probably changed. This phenomenon also reflected the fact that SS and PVA form Si-O-C bonds through hydroxyl cross-linking. There was no significant differences in the XRD patterns of fibers and other three composite samples when the paste was coated on BFs (), which suggested the tight binding of sizing agents on the BFs’ surface.
Figure 10. (a) the XRD patterns of lubrication paste of PS-RhB, PVA-PS-RhB and PVA-SS-PS-RhB, and (b) the coated fiber composites PS-RhB/BF, PVA-PS-RhB/BF and PVA-SS-PS-RhB/BF on BFs.
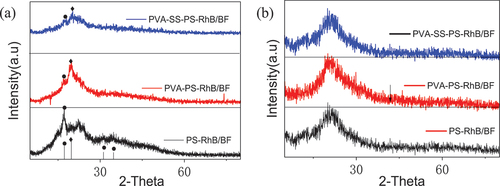
The thermal stability of two dye lubricants (PVA-SS-PS-RhB/BF and PVA-PS-RhB/BF) was analyzed by TG-DSC. The mass loss in TG curves of illustrated that fibers were successfully coated with PVA compared to the TG curve of BF shown in Figure S3 (see the SI). The DTA profile of PVA-PS-RhB/BF samples in revealed that the decomposition started temperature of the second and the third stage which were delayed by 20ºC in comparison to PVA-SS-PS-RhB/BF. The initial endothermic peak occurred in 90-110ºC with a weight loss of 0.66%, attributed to water evaporation and the enthalpy ΔH = 22.4 J/g was obtained from DSC profile (). The endothermic small peak at 60ºC was assigned to glass transition temperature (Tg) (Gómez et al. Citation2015), and we discovered that Tg at about 70ºC shown as the of PVA-SS-PS-RhB which was higher than pure PVA of Tg=60ºC. In accordance with the infrared analysis, it could be attributed to a certain cross-linked structure in the polyvinyl SS composite increased the stability. As a result, the decomposition of PVA molecular chain was hindered, and the glass transition temperature was raised. We also found that the melting temperature (TS) became as low as 210°C in the PVA-SS-PS-RhB, and the melting peak area was much smaller. Reasons were that the a cross-linked structure caused the number of hydroxyl groups and endothermic effect of the composite coating was not prominent (Zhou et al. Citation2008). However, the sample with no Na2SiO3 has an endothermic effect after 230ºC, indicating that PVA was partly decomposed, which proved that the decomposition temperature of composite coating became higher after SS incorporation. This was consistent with results of DTA shown in .
Figure 11. Profiles TG (a), DTA (b), DSC profiles (c), and retested for the second time of PVA-SS-PS-RhB and PVA-PS-RhB (d).
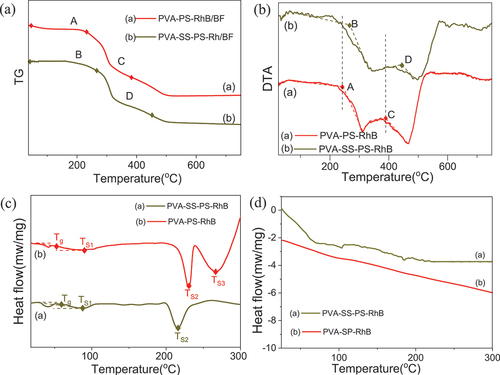
To study the thermal stability of composite fibers, and the PVA-SS-PS-RhB/BF was heated to 300ºC was retested for a second time (25 300ºC, shown as ). It was found that the thermal effect of PVA-PS-RhB samples disappeared, indicating that all the macromolecular segments of the PVA were broken, while the PVA-SS-PS-RhB still had brilliant thermal stability, and the melting peak area was basically unchanged. Mechanism of crosslinking has been proposed to form strong hydrogen bonds between phosphoric acid and starch, which would bind with BFs tightly, and the thermal stability and water resistance were improved (Reddy and Yang Citation2010).Therefore, the thermal stability of the PVA-SS-PS-RhB composite coating was successfully prepared.
Conclusions
In conclusion, the colored BFs PVA-SS-PS-RhB/BF has been successfully prepared during the drawing of BFs through an in-situ means of using PVA-SS-PS-RhB dye lubricant. The color fastness tested result (4–5 grade) showed that this strategy was feasible to sustain harsh washing, and similar values were also achieved in the color rubbing experiments. This dyeing method also brings the additional properties of higher strength and better temperature stability. SS modified PVA was a promising strategy to improve network structure and reduce the content of hydroxyl for enhancing the heat stability while lowering the hydrolysis dye molecules. Therefore, this dyeing method endowed BF with bright color, flexibility, woven ability, and high-value utilization in various fields.
Supplemental Material
Download MS Word (2.8 MB)Acknowledgement
We acknowledge the National Natural Science Foundation of China (51874145), financial supports by Scientific and Technological Developing Scheme of Jilin Province (20200401028GX), Exploration project of State Key Laboratory of Automotive Simulation and Control (ascl-zytsxm-202005), China Ocean Mineral Resources R&D Association (COMRA) Special Foundation (DY135-R2-1-01, DY135-46), and Province/Jilin University co-construction project - funds for new materials - (SXGJSF2017-3).
Disclosure statement
No potential conflict of interest was reported by the author(s).
Supplementary material
Supplemental data for this article can be accessed online at https://doi.org/10.1080/15440478.2022.2145408.
Additional information
Funding
References
- Calas, G., G. S. Henderson, and J. F. Stebbins. 2006. Glasses and melts: Linking geochemistry and materials science. Elements 2 (5):265–17. doi:10.2113/gselements.2.5.265.
- Cardozo-Filho, L., H. R. Mazzer, J. C. Santos, J. Andreaus, A. C. Feihrmann, C. Beninca, V. F. Cabral, and E. F. Zanoelo. 2014. Dyeing of polyethylene terephthalate fibers with a disperse dye in supercritical carbon dioxide. Textile Research Journal 84 (12):1279–87. doi:10.1177/0040517513515317.
- Chen, H. C. 1997. Dyeing of glass fiber fabrics having amino groups with acid dyestuff and after‐chroming. Journal of Applied Polymer Science 66 (6):1039–48. doi:10.1002/(sici)1097-4628(19971107)66:6<1039:aid-app4>3.0.co;2-j.
- Chen, K. L., W. W. Gou, X. M. Wang, C. J. Zeng, F. Q. Ge, Z. J. Dong, and C. X. Wang. 2018. UV-cured fluoride-free polyurethane functionalized textile with pH-induced switchable superhydrophobicity and underwater superoleophobicity for controllable oil/water separation. ACS Sustainable Chemistry & Engineering 6 (12):16616–28. doi:10.1021/acssuschemeng.8b03851.
- Chuvashov, Y., O. Jashchenko, I. Diduk, and V. Gulik. 2020. The investigation of fiber surface condition from basalt-like rocks for enhanced industrial applications. Journal of Natural Fibers 19 (8):1–10. doi:10.1080/15440478.2020.1838987.
- Demir, A., B. Arık, E. Ozdogan, and N. Seventekin. 2010. The comparison of the effect of enzyme, peroxide, plasma and chitosan processes on wool fabrics and evaluation for antimicrobial activity. Fibers and Polymers 11 (7):989–95. doi:10.1007/s12221-010-0989-5.
- Destainville, A., E. Champion, D. Bernache-Assollant, and E. Laborde. 2003. Synthesis, characterization and thermal behavior of apatitic tricalcium phosphate. Materials Chemistry and Physics 80 (1):269–77. doi:10.1016/s0254-0584(02)00466-2.
- Elkins, K. E., T. S. Vedantam, J. P. Liu, H. Zeng, S. H. Sun, Y. Ding, and Z. L. Wang. 2003. Ultrafine FePt nanoparticles prepared by the chemical reduction method. Nano letters 3 (12):1647–49. doi:10.1021/nl034734w.
- El-Sayed, S., T. Abel-Baset, A. A. Elfadl, and A. Hassen. 2015. Effect of nanosilica on optical, electric modulus and AC conductivity of polyvinyl alcohol/polyaniline films. Physica B: Condensed Matter 464:17–27. doi:10.1016/j.physb.2015.02.016.
- Gómez, I., E. M. Otazo, H. Hernández, E. Rubio, J. Varela, M. Ramírez, I. Barajas, and A. J. Gordillo. 2015. Thermal degradation study of PVA derivative with pendant phenylthionecarbamate groups by DSC/TGA and GC/MS. Polymer Degradation and Stability 112:132–36. doi:10.1016/j.polymdegradstab.2014.12.027.
- Idan, I. J., L. C. Abdullah, S. N. Ain, B. M. Jamil, M. K. Obaid, and T. S. Y. Choong. 2017. Fixed-bed system for adsorption of anionic acid dyes from binary solution onto quaternized kenaf core fiber. BioResources 12 (4):8870–85. doi:10.15376/biores.12.4.8870-8885.
- Kang, Y. Q., M. S. Cao, X. L. Shi, and Z. L. Hou. 2007. The enhanced dielectric from basalt fibers/nickel core-shell structures synthesized by electroless plating. Surface & Coatings Technology 201 (16–17):7201–06. doi:10.1016/j.surfcoat.2007.01.037.
- Korkmaz, M., G. K. Nazife, and D. Yılmaz. 2018. Development of a novel hybrid yarn production process for functional textile products. Journal of Industrial Textiles 48 (9):1462–88. doi:10.1177/1528083718766847.
- Li, J. L., Z. Q. Huang, C. Xue, Y. X. Zhao, W. B. Hao, and G. D. Yang. 2018. Facile preparation of novel hydrophobic sponges coated by Cu2O with different crystal facet structure for selective oil absorption and oil/water separation. Journal of Materials Science 53 (14):10025–38. doi:10.1007/s10853-018-2299-z.
- Mishukova, A. S., V. V. Safonov, and V. V. Apyari. 2020. One-step dyeing of polyurethane fibers. Fibre Chemistry 51 (6):427–29. doi:10.1007/s10692-020-10126-5.
- Nakatani, H., K. Iwakura, K. Miyazaki, N. Okazaki, and M. Terano. 2011. Effect of chemical structure of silane coupling agent on interface adhesion properties of syndiotactic polypropylene/cellulose composite. Journal of Applied Polymer Science 119 (3):1732–41. doi:10.1002/app.32873.
- Onggar, T., G. Amrhein, A. Abdkader, R.-D. Hund, and C. Cherif. 2016. Wet-chemical method for the metallization of a para-aramid filament yarn wound on a cylindrical dyeing package. Textile Research Journal 87 (10):1192–202. doi:10.1177/0040517516651099.
- Panda, P. K., D. Rastogi, M. Jassal, and A. K. Agrawal. 2012. Effect of atmospheric pressure helium plasma on felting and low temperature dyeing of wool. Journal of Applied Polymer Science 124 (5):4289–97. doi:10.1002/app.35410.
- Pei, L. J., X. M. Gu, and J. P. Wang. 2021. Sustainable dyeing of cotton fabric with reactive dye in silicone oil emulsion for improving dye uptake and reducing wastewater. Cellulose 28 (4):2537–50. doi:10.1007/s10570-020-03673-x.
- Periolatto, M., F. Ferrero, M. Giansetti, R. Mossotti, and R. Innocenti. 2010. Enzyme-aided wool dyeing with a neutral protease at reduced temperatures. Engineering in Life Sciences 10 (5):474–79. doi:10.1002/elsc.201000089.
- Ralph, C., P. Lemoine, A. Boyd, E. Archer, and A. McIlhagger. 2019. The effect of fibre sizing on the modification of basalt fibre surface in preparation for bonding to polypropylene. Applied Surface Science 475:435–45. doi:10.1016/j.apsusc.2019.01.001.
- Ramezanzadeh, M., B. Ramezanzadeh, M. G. Sari, and M. R. Saeb. 2020. Corrosion resistance of epoxy coating on mild steel through polyamidoamine dendrimer-covalently functionalized graphene oxide nanosheets. Journal of Industrial and Engineering Chemistry 82:290–302. doi:10.1016/j.jiec.2019.10.025.
- Reddy, N., and Y. Q. Yang. 2010. Citric acid cross-linking of starch films. Food Chemistry 118 (3):702–11. doi:10.1016/j.foodchem.2009.05.050.
- Sarkodie, B., and Z. F. Zhu. 2018. Effect of amphiphilic phosphate/octenylsuccinate starch on enhancing adhesion to hydrophobic polyester fibers in sizing. The Journal of Adhesion 95 (11):1015–30. doi:10.1080/00218464.2018.1459576.
- Selim, A., A. J. Toth, E. Haaz, D. Fozer, A. Szanyi, N. Hegyesi, and P. Mizsey. 2019. Preparation and characterization of PVA/GA/Laponite membranes to enhance pervaporation desalination performance. Separation and Purification Technology 221:201–10. doi:10.1016/j.seppur.2019.03.084.
- Shi, Y., D. Belosinschi, F. Brouillette, A. Belfkira, and B. Chabot. 2014. Phosphorylation of Kraft fibers with phosphate esters. Carbohydrate Polymers 106:121–27. doi:10.1016/j.carbpol.2014.01.070.
- Si, J. W., Z. Y. Wang, J. Y. Li, C. X. Zuo, P. P. Zhang, C. D. Wei, J. Wang, W. Q. Li, and S. D. Miao. 2021. Effects of CaO added to raw basalt on producing continuous basalt fibers and their mechanical properties. Journal of Non-Crystalline Solids 568:120941. doi:10.1016/j.jnoncrysol.2021.120941.
- Sugiura, K., M. Hashimoto, S. Matsuzawa, and K. Yamaura. 2001. Influence of degree of crystallinity and syndiotacticity on infrared spectra of solid PVA. Journal of Applied Polymer Science 82 (5):1291–98. doi:10.1002/app.1963.
- Sun, T. K. 2003. Starch, poly(lactic acid), and poly(vinyl alcohol) blends. Journal of Polymers and the Environment 11 (1):1–14. doi:10.1023/A:1023875227450.
- Sun, H., X. Shao, M. Zhang, Z. Wang, J. Dong, and D. Yu. 2019. Mechanical, barrier and antimicrobial properties of corn distarch phosphate/nanocrystalline cellulose films incorporated with Nisin and ε-polylysine. International Journal of Biological Macromolecules 136:839–46. doi:10.1016/j.ijbiomac.2019.06.134.
- Tee, Y. B., R. A. Talib, K. Abdan, N. L. Chin, R. K. Basha, and K. F. M. Yunos. 2013. Thermally grafting aminosilane onto kenaf-derived cellulose and its influence on the thermal properties of poly (lactic acid) composites. BioResources 8 (3):4468–83. doi:10.15376/biores.8.3.4468-4483.
- Toropina, L. V., G. G. Vasyuk, V. L. Kornyushina, V. M. Dyaglev, Y. M. Rassadin, and M. A. Makarushina. 1995. New cloth from basalt fibres. Fibre Chemistry 27 (1):60–61. doi:10.1007/BF00551537.
- Wang, Y. L., T. Herricks, and Y. M. Xia. 2003. Single crystalline nanowires of lead can be synthesized through thermal decomposition of lead acetate in ethylene glycol. Nano letters 3 (8):1163–66. doi:10.1021/nl034398j.
- Wang, G. J., Y. W. Liu, Y. J. Guo, Z. X. Zhang, M. X. Xu, and Z. X. Yang. 2007. Surface modification and characterizations of basalt fibers with non-thermal plasma. Surface & Coatings Technology 201 (15):6565–68. doi:10.1016/j.surfcoat.2006.09.069.
- Wang, Z. T., H. J. Luo, L. Zhang, J. Zhang, H. W. Chen, and H. Jiang. 2020. Mechanical properties of basalt fiber improved by starch phosphates sizing agent. Applied Surface Science 521:146196. doi:10.1016/j.apsusc.2020.146196.
- Wang, W., H. Zhang, Y. Dai, H. X. Hou, and H. Z. Dong. 2015. Effects of low poly(vinyl alcohol) content on properties of biodegradable blowing films based on two modified starches. Journal of Thermoplastic Composite Materials 30 (7):1017–30. doi:10.1177/0892705715614080.
- Xu, S. X., J. G. Chen, B. J. Wang, and Y. Q. Yang. 2016. An environmentally responsible polyester dyeing technology using liquid paraffin. Journal of Cleaner Production 112:987–94. doi:10.1016/j.jclepro.2015.08.114.
- Yuan, X. M., B. Zhu, X. Cai, J. J. Liu, K. Qiao, and J. W. Yu. 2017. Optimization of interfacial properties of carbon fiber/epoxy composites via a modified polyacrylate emulsion sizing. Applied Surface Science 401:414–23. doi:10.1016/j.apsusc.2016.12.234.
- Zahed, B., and H. H. Monfared. 2015. A comparative study of silver-graphene oxide nanocomposites as a recyclable catalyst for the aerobic oxidation of benzyl alcohol: Support effect. Applied Surface Science 328:536–47. doi:10.1016/j.apsusc.2014.12.078.
- Zhang, H., J. K. Wang, R. Wang, and X. P. Fan. 2011. Preparation and properties of cationic graft starch sizing agent. Advanced Materials Research 331:144–47. https://doi.org/10.4028/www.scientific.net/AMR.331.144.
- Zhou, X. Y., D. M. Jia, Y. F. Cui, and D. Xie. 2008. Kinetics analysis of thermal degradation reaction of PVA and PVA/starch blends. Journal of Reinforced Plastics and Composites 28 (22):2771–80. doi:10.1177/0731684408093872.