ABSTRACT
This paper describes a study of a nonwoven sample constructed of kapok/polypropylene used as a thermal insulation layer in cold weather. The needle punching technique was used to create three nonwoven fabrics with varying kapok/polypropylene blend ratios of 70/30, 60/40, and 50/50 and characterized for thermal insulation application. Considering higher thermal insulation of 70/30 blend ratio, further experimental designs were created by changing punch density, depth of penetration, and web areal density in the 70/30 blend ratio. The structural features of kapok fiber and the cohesiveness of nonwoven fabric are demonstrated using SEM analysis. The effect of web areal density and machine parameters on thermal insulation of nonwoven was investigated. Nonwoven fabric characteristics such as thickness, air permeability, porosity, and bulk density were also investigated. Thermal resistance increases with increase in depth of penetration and 12.5 mm showed higher thermal resistance and decreased with higher punch density. The higher number of layering of the optimized sample number 2 showed a higher thermal resistance value of 0.5272°C m2/W.
摘要
本文描述了一种由木棉/聚丙烯制成的非织造样品在寒冷天气中用作隔热层的研究. 使用针刺技术制造三种不同木棉/聚丙烯共混比为70/30、60/40和50/50的非织造织物,并表征其隔热应用. 考虑到70/30混合比的更高隔热性,通过在70/30的混合比中改变冲头密度、穿透深度和腹板面积密度来创建进一步的实验设计. 用SEM分析证明了木棉纤维的结构特征和非织造布的粘结性. 研究了纤维网面密度和机器参数对非织造布隔热性能的影响. 还研究了非织造布的厚度、透气性、孔隙率和堆积密度等特性. 热阻随着穿透深度的增加而增加,12.5 mm显示出更高的热阻,并且随着冲头密度的增加而降低. 优化样品编号2的层数越高,热阻值越高,为0.5272°C m2/W.
Introduction
Layering fabrics and materials with the potential to trap air provide higher thermal insulation, which is why they are used as cold weather clothing. For the middle insulating layer in cold weather apparel, thermal bonded and needle punched nonwoven textiles, as well as multi-layered fabrics, are preferred. Many experts have tried to produce nonwoven materials for thermal insulation and researched the features of various materials. However, synthetic fiber nonwovens are the main focus. According to researchers, nonwovens with a high polypropylene content provide excellent thermal insulation. Natural fiber-based nonwoven materials are utilized for thermal insulation applications and are being explored by various researchers in the context of sustainable principles. The material has a hollow lumen structure that traps a lot of air. Because of its hollowness, kapok fiber finds a place in the thermal barrier layer among all the fibers. Kapok fiber has a low thermal conductivity and is utilized as a mattress in fill (Voumbo et al. Citation2010). Polypropylene with high packed density and elongation properties is utilized to promote structural consolidation.
Hosseini Varkiyani et al. (Citation2011) have developed a needle punched nonwoven with a different punched density from polyester and a polypropylene blend. It was observed from their study that heat transfer decreased with an increase in punch density and blending of fiber types, which caused a reduction in heat transfer. A few other researchers discovered similar results (Debnath Citation2011; Debnath and Madhusoothanan Citation2010). Barker and Ryan (Citation2011) studied the thermal insulation of hydro-entangled nonwovens, and the results showed that web layering enhanced the thermal insulation without adding weight or thickness of the batting.
Purnawati et al. (Citation2018) studied physical and chemical properties of kapok fiber. They showed in their result that kapok had large lumen filled with air and low wettability. Cui et al. (Citation2010) have studied the performance of kapok and down-blend wadding using air-laid technology. They observed from their study that thermal conductivity decreased with an increase in kapok percentage and kapok had good resistance to collapse, but elastic resilience became weaker after multiple compressions.
Ganesan and Karthik (Citation2016) developed a nonwoven blend with kapok and milkweed. They observed from their results that the thermal conductivity decreased with an increase in kapok. Duran (Citation2016) created a low-cost nonwoven out of wool and recycled wool fiber, and their findings demonstrated that single- and double-layer nonwovens improve thermal insulation properties.
Yachmenev, Negulescu, and Yan (Citation2006) developed biodegradable cellulosic fiber-based nonwovens to improve thermal insulation properties. Debnath and Madhusoothanan (Citation2010) developed needle punched nonwoven from round, circular, and trilobal polyester and showed that trilobal nonwoven had a high thermal insulation value. Muthukumar et al. (Citation2021) developed a nonwoven with flax and low melt PET for sound and thermal insulation applications. They observed that thermal resistance decreased with an increase in low melt PET. Jabbar et al. (Citation2022) produced a nonwoven made of kapok and recycled polyester. The results revealed that increasing the punch density reduced the heat resistance. The thermal resistance of needle-punched nonwoven fabrics made of natural fibers like jute, cotton, milk weed, and kapok fibers, as well as their blends, as observed to be high.
Many researchers have explored the use of synthetic materials and goose feathers in the form of loose filling as thermal insulation layers for cold weather applications. However, this study focuses on the structuring of kapok and blends as needle-punched nonwoven fabric for thermal insulation layers. Limitation of kapok fiber is its fragileness, having slipperiness, very fine and light; hence, structuring is a challenge. The study focuses on the creation of eco-friendly nonwoven textile materials based on kapok fibers utilizing the needling technique. The effect of machine parameters such as punch density, depth of penetration, and web areal density on the thermal resistance of needle punched nonwoven fabric with kapok/polypropylene has not been studied in the literature for cold weather clothing thermal insulation application. Using the Box and Behnken experimental design, the influence of the above-mentioned process parameters on the thermal insulation of nonwoven was investigated. The best suitable nonwoven samples were optimized with two and three layers of nonwoven fabric as thermal insulation layer for cold weather clothing. The interaction effect of the number of layers on the thermal resistance was also studied. The nonwoven that has been produced is a low-cost, environmentally friendly thermal insulation for cold-weather jackets. As a result, kapok can be used as a substitute for synthetic fibers.
Materials and methods
In this experiment, kapok and polypropylene were used to develop nonwovens for cold weather clothing applications. Kapok fibers were taken from organic farms in Coimbatore, India. Fibers made of polypropylene were obtained from (Zenith Company, India). In the fiber length measurement study, a sample of 100 fibers was used. The length of kapok and polypropylene fiber is 17.463 mm and 51.039 mm and was measured using the single-fiber length measurement method. Fibers were spread across the black chart. Individual fibers were plucked at 1 mm intervals and kept near a measuring scale. The results of the tests were collected and averaged. The airflow approach was used to study the fiber fineness. 15–20 g of sample was opened and cleaned before being fed into the airflow instrument at a constant pressure for five consecutive readings, which were then averaged. The diameter of kapok is 24.66 µm and polypropylene 45.149 µm was measured using SEM analysis.
Nonwovens were developed by blending of kapok with polypropylene at different blend ratios of 70/30, 60/40, and 50/50. Using a laboratory model carding machine, the nonwoven webs were created using kapok fiber combined with polypropylene fiber. The webs were then needle punched using a DILO needle punching machine with processing parameters of 50 punches per cm2 and a penetration depth of 5 mm. The fibrous web’s structure is stabilized by interlocking fibers without the use of a binder. The length of the fiber has a significant impact on the processing performance. Polypropylene fiber was added as carriers and carded to ensure the compact configuration because kapok fibers are shorter. Three nonwoven samples were made with three different blend ratios.
Design of experiment
In the nonwoven, the punch density (50, 75, and 100 punches/cm2), penetration depth (7.5, 10, and 12.5 mm), and web areal density (200,300, and 400 g/m2) were taken as independent variable. The factor levels were labeled as −1 (low), 0 (middle), and +1 (high). Design expert 8.1 trial version was used to optimize the data that was analyzed.
A fabric thickness gauge with a capacity of 0.01 mm was used to measure the thickness of the nonwovens generated under 2 kPa pressure. The areal density of the produced nonwoven textiles was measured using an electronic balance with a capacity of 0.001 g, in accordance with ASTM D-1910. The Textest air permeability tester was used to assess the air permeability of the nonwoven fabrics in accordance with ASTM D737. FESEM was used to investigate the fiber morphology. Using a double-sided adhesive tape, samples (5 mm to 2 mm) were adhered to the SEM sample stab. The samples were coated with platinum (200 A°) for 2 min at a pressure of 0.001 torr using ion sputtering equipment. Under ultraviolet light, the platinum-coated samples were examined. Porosity (H) is calculated using the following equation.
H = 1-(ρb/ ρa)
Where ρb the bulk density of nonwoven and ρa the density of fibers
Thermal resistance characterization (ASTM 1868-02)
A sweating guarded hot plate apparatus is used to test the thermal resistance of needle punched nonwovens as per ASTM standard 1868–02. During testing, temperature of test plate, guard section, and bottom plate were maintained at a constant temperature of 35 ± 0.5°C with no more than a 0.1°C variation. By placing a fabric or fabric assembly on the test plate, total thermal resistance (Rct) was measured. Measurements were taken every 3 min for a minimum of 30 min after it reached steady state conditions to calculate the overall thermal resistance of the fabric plus the air layer (Rct).
Results and discussion
SEM analysis of kapok, polypropylene, and nonwoven
The morphology of kapok, polypropylene, and kapok/polypropylene nonwoven samples was investigated using FESEM. The images of kapok fiber showed a hollow lumen structure, which could be due to its structural properties (). The measured internal and external diameter of the kapok was 20.32 µm and 24.66 µm, respectively, and cell wall thickness was 2.17 µm. The polypropylene fiber is smooth and solid (). The polypropylene fiber has 51.039 mm length and 45.149 µm diameter. The thermal resistance properties of kapok and polypropylene character were confirmed by loose filling method using sweating guarded hot plate method. shows SEM images of the needle punched Kapok/Polypropylene blended nonwoven. The presence of polypropylene and kapok fiber is marked in (). It can also be seen from the figure that a large number of kapok fibers are trapped between few polypropylene fibers.
Physical characteristics of nonwoven
It was observed that the 50/50 and 60/40 kapok/polypropylene blend ratios showed higher porosity, bulk density, and air permeability than 70/30. This may be due to higher inter-fiber cohesiveness in the structure due to equal blending proportion leading to reduction in thickness and higher porosity as shown in . When compared to the other two blend ratios, the bulk density of the 70/30 blend is found to be low (Ganesan and Karthik Citation2016). This may be due to the higher proportion of kapok, which is lighter and finer. Air permeability and still air in the structure is a critical consideration for thermal insulation layers, and air should not be permitted for better insulation. Another reason for higher thermal resistance in the 70/30 kapok/polypropylene nonwoven is due to higher amount of hollow fiber in the assembly offering better air entrapment within the hollow channels (Gnanauthayan, Rengasamy, and Kothari Citation2017). Hollowness in the kapok is as confirmed by SEM . The heat retention of kapok was better due to static immobile air held in the large lumen region of kapok (Vignesh Dhanabalan et al. Citation2015).
Table 1. Physical characteristics of nonwoven.
Analysis of the thermal resistance of nonwoven
shows that the blend ratio was the most important factor in affecting heat resistance. The linear regression curve (R2) has a value of 0.993, indicating that it is well fitting. According to the and , increasing the kapok blend fraction increases heat resistance while decreasing bulk density and porosity. This could be owing to kapok’s decreased density (Ganesan and Karthik Citation2016). The type of vegetable fibers used in nonwovens, as well as the cellulosic/synthetic fiber blend ratios, influence their thermal resistance (Yachmenev, Negulescu, and Yan Citation2006). The negative coefficient shows a decrease in thermal resistance because of an increase in polypropylene in the nonwoven.
Physical properties of 70/30 kapok/polypropylene nonwoven (Box and Behnken factorial design)
Based on the above findings, 70/30 kapok/polypropylene was taken for further study. shows the effects of punching density, depth of penetration and web areal density on thickness, fabric areal density, air permeability, porosity, and bulk density of nonwoven fabrics using the Box and Behnken factorial design. It was observed from the study that the thickness of the fabric was in the range of 1.32 to 3.67 mm and the porosity was in the range of 0.836 to 0.930. The air permeability was in the range of 11.0 to 34.50 cm3/cm2/s. It was also observed from the study that air permeability decreases prominently with the increase in fabric weight and decrease in thickness. It is not much influenced by the depth of penetration and needling density (Debnath and Madhusoothanan Citation2010). The bulk density was obtained from 0.04230 g/cm3 to 0.07318 g/cm3. An increase in needle penetration causes higher web consolidation and increasing density(Muthukumar et al. Citation2021). It was also observed in our study that the increase in mass per unit area increases the bulk density(Kopitar, Skenderi, and Matijasic Citation2017).
Table 2. Experimental results for selected design.
Effect of machine parameter on thermal resistance
The influence of process parameters like punch density and depth of penetration on the thermal resistance was performed in surface plots. shows that the heat resistance of nonwoven increases as the punch density increases from 50 to 75 punches/cm2. There was a decrease in thermal resistance as punch density increased further. This may be due to poor structural consolidation beyond a certain level of punch density. The change in bulk density was obtained by modifying the punch density on the laid webs. The surface plot shows that the thermal resistance increases from 0.1230°C m2/W to 0.1878°C m2/W during the depth of penetration increment due to better fiber interlocking. When the punch density increases, the thickness of the nonwoven decreases, which reduces the thermal resistance. A similar observation was made by (Raeisian et al. Citation2013). In their study, they reported that thermal resistance increased due to increased thickness and areal weight.
Figure 3. Effect of depth of penetration and punch density on thermal resistance of 70/30 kapok/PP nonwoven.
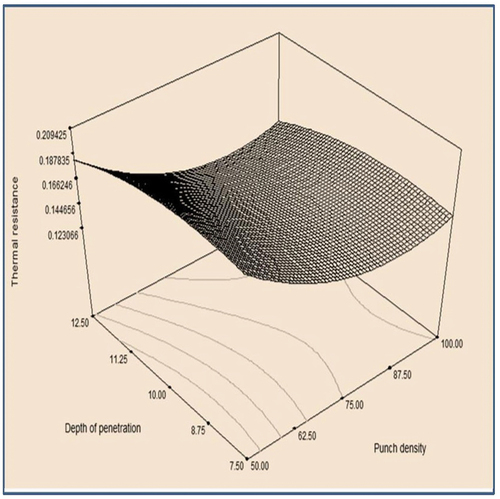
shows the influence of punch density and web areal density on thermal resistance. The surface plot revealed that there is no statistically significant difference between increasing web areal density on thermal resistance. Among the three levels of punch density, 75 punches/cm2 gives better thermal resistance. Further increases reduced the thermal resistance. As the punch density rises, the structure becomes more packed; resulting in more tortuosity or a shorter mean free route, and therefore more heat is blocked. This is due to increased needling action, which decreases the thickness of the fabric, resulting in improved structural consolidation (Shabaridharan and Das Citation2013). The degree of entanglement between fibers and web layers improved as punching density increased (Rasheed et al. Citation2022), but the effect was not linear with punching density.
Figure 4. Effect of punch density and web areal density on thermal resistance of 70/30 kapok/PP nonwoven.
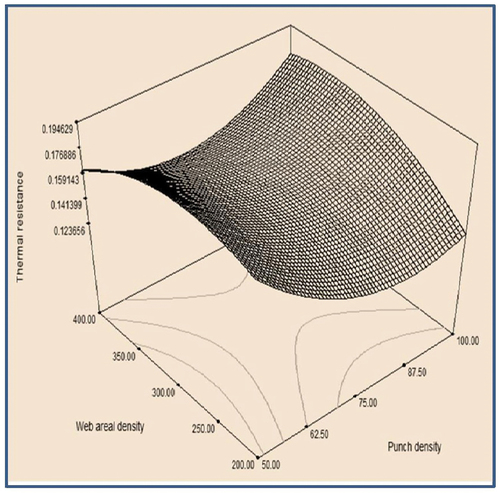
shows that the higher the depth of penetration, the higher is the thermal resistance. This may be due to better structural linking due to high penetration depth. It was also observed that the minimum web areal density had a higher insulation value. This may be due to layering of web compassing air pocket and also higher thickness (Pividal et al. Citation2021). Further increases in web areal density had no influence on thermal resistance.
Statistical analysis
The regression coefficient (R2) value demonstrates a relationship between the physical parameters of the nonwoven fabric and the experimental region. The “P” values are used to check the significance of each coefficient, as well as the strength of the interaction between each independent variable. The percentage of variation in response that the model can explain is represented by R2 and also can be used to check the model’s accuracy. The independent variable, with an R2 value of 0.6117, is responsible for 61.17% of the entire variation in thermal resistance is given in . The correlation is better when R2 is close to 1 (Kaleeswaran and Kothari Citation2017).
Table 3. Response surface equation.
The numerical optimization method is used to optimize process parameters versus responses, which is done with the use of design expert software. Numerical optimization will find solutions in the design space, using the models developed throughout the investigation to determine factor settings that meet the objectives. Attaining improved results in the thermal resistance of the developed nonwoven fabric is the goal of this study. Based on the above-mentioned criteria, the numerical optimization analysis generates a list of the top 10 process parameter combinations that result in better physical attributes (). In each of the selected responses, the increase in penetration depth had a positive result. The thermal resistance increases with increasing penetration depth. Hence, the selected top 10 experiment sets contain the maximum amount of penetration depth of 12.5 mm. In the case of web areal density, an increase in web areal density decreases the inter-fiber bonding. After a certain level, the cohesiveness is reduced, so the thermal resistance is reduced at higher web areal density. Hence, the optimum of 283.37 g/m2 web areal density was selected. Beyond that limit, the nonwoven fabric loses its required thermal resistance. Since the punch density has very little effect on the selected response. The 75 punches/cm2 punch density of the selected response gives better thermal resistance.
Table 4. Numerically optimized top 10 process parameters.
Development of two and three-layer thermal insulation layer
Based on contour plot analysis, the best two samples (sample numbers 2 and 12) were used for double and triple thermal insulation layer preparation.
shows the physical and thermal properties of single, two, and three-layer assemblies. It was observed in the study that the layering of nonwoven (Deniz Citation2016) has a better influence on thermal resistance than the single layer of nonwoven. From , it is observed that sample 2 has higher resistance value than sample 12. This may be attributed to the higher areal density, lower porosity, and lower air permeability of sample 2 (Kaleeswaran and Kothari Citation2017; Al-Hakimi et al. Citation2021). Furthermore, it is observed from the capillary flow analysis that the mean flow pore diameter is 78.34 µm in sample 2 and is 79.44 µm in sample 12, and the pore size distribution shows that the sample 2 has more number of pores in less than 10 µm, but sample 12 has more pores in above 68 microns.
Table 5. Physical and thermal properties of layered nonwoven.
Conclusion
The sustainable, biodegradable, and low-cost thermal insulating materials have benefits for both the environment and human comfort. Needle punched nonwoven textiles produced from kapok and polypropylene fibers were used for thermal insulation in this study. The nonwoven is produced from kapok and polypropylene fibers with blend ratios of 70/30, 60/40, and 50/50. Physical and thermal resistance characteristics of the produced nonwoven sample were investigated. It was observed that the higher % kapok fiber had higher thermal resistance of nonwovens. The best combination has been selected as a 70/30 blend ratio based on the test result for further study. The selected blend ratio was used to produce nonwoven with different combinations of selected input parameters (web areal density, punch density, and depth of penetration) and investigated thermal resistance and physical properties. Among the variables selected in the experiment, the depth of penetration has a significant influence on thermal resistance. Higher number of layering of the optimized sample number 2 showed higher thermal resistance value of triple- and double-layer nonwoven 0.5272°C m2/W and 0.3720°C m2/W than its single layer is 0.2112°C m2/W. The nonwoven that has been produced is a low-cost, environmentally friendly thermal insulation material for cold-weather jackets. As a result, this study has explored structuring kapok and hence can be used as a substitute for synthetic fibers.
Highlights
The blend ratio of the nonwoven was found to be the major factor influencing heat resistance with an R2 of 0.993.
Nonwoven punch density increases are positively correlated with heat resistance.
The increase in the depth of penetration of the needle punching process increases the thermal resistance from 0.123 to 0.183 (up to a 48.78% increase in thermal resistance).
Layering of nonwoven increases areal density and positively helps in increasing the thermal resistance.
Disclosure statement
No potential conflict of interest was reported by the author(s).
References
- Al-Hakimi, M. T. A., A. R. Azrin Hani, G. M. Q. Didi, and N. A. Anis Amirah. 2021. “Porosity and thermal resistance properties of needle-punched nonwovens cotton and polyester.” 8th International Conference on Advanced Materials Engineering and Technology (ICAMET 2020), Malaysia, AIP Conference Proceedings 2347 (1). doi:10.1063/5.0052717.
- Barker, R. L., and C. H. Ryan. 2011. Factors affecting the thermal insulation and abrasion resistance of heat resistant hydro-entangled nonwoven batting materials for use in firefighter turnout suit thermal liner systems. Journal of Engineered Fibers and Fabrics 6 (1):1–11. doi:10.1177/155892501100600101.
- Cui, P., F. M. Wang, A. Wei, and K. Zhao. 2010. The performance of kapok/down blended wadding. Textile Research Journal 80 (6):516–23. doi:10.1177/0040517508097522.
- Debnath, S. 2011. Thermal resistance and air permeability of jute-polypropylene blended needle-punched nonwoven. Indian Journal of Fiber and Textile Research 36 (2):122–31.
- Debnath, S., and M. Madhusoothanan. 2010. Thermal insulation, compression and air permeability of polyester needle-punched nonwoven. Indian Journal of Fiber and Textile Research 35:38–44.
- Deniz, D. 2016. A research on thermal insulation properties of nonwovens produced with recycled jute and wool fibres. Textile and Apparel 26 (1):22–30.
- Duran, D. 2016. A Research on thermal insulation properties of nonwoven produced with recycled jute and wool fibers. Textile and Apparel 26(1):22–30. https://dergipark.org.tr/en/pub/tekstilvekonfeksiyon/issue/23651/251933.
- Ganesan, P., and T. Karthik. 2016. Development of acoustic nonwoven materials from kapok and milkweed fibres. The Journal of the Textile Institute 107 (4):477–82. doi:10.1080/00405000.2015.1045251.
- Gnanauthayan, G., R. S. Rengasamy, and V. K. Kothari. 2017. Heat insulation characteristics of high bulk nonwovens. The Journal of the Textile Institute 108 (12):2173–79. doi:10.1080/00405000.2017.1316697.
- Hosseini Varkiyani, S. M., H. Rahimzadeh, H. Bafekrpoor, and A. A. Jeddi. 2011. Influence of punch density and fiber blends on thermal conductivity on nonwoven. Open Textile Journal 4 (1):1–6. doi:10.2174/1876520301104010001.
- Jabbar, A., M. A. Ali, A. Shahzad, M. S. Naeem, Z. Javed, M. B. Qadir, K. Rehman, M. Irfan, and Z. Ahmad. 2022. Development of kapok/recycled-PET blended needle-punched thermal waddings. Journal of Natural Fibers 19 (3):1024–32. doi:10.1080/15440478.2020.1784816.
- Kaleeswaran, P., and V. K. Kothari. 2017. Thermal resistance of nonwoven waddings. The Journal of the Textile Institute 108 (10):1657–61. doi:10.1080/00405000.2016.1275445.
- Kopitar, D., Z. Skenderi, and G. Matijasic. 2017. Influence of nonwoven fabric pore sizes on water vapor resistance. Textile Research Journal 88 (12):1–11. doi:10.1177/0040517517700200.
- Muthukumar, N., G. Thilagavathi, S. Periyasamy, and V. Vinoth. 2021. Development of needle punched nonwovens from natural fiber waste for thermal insulation application. Journal of Natural Fibers 1–9. doi:10.1080/15440478.2021.1990175.
- Pividal, P., and A. M. Rocha. 2021. Thermal behaviour of bi-layered needle-punched nonwovens produced from 100% raw kapok fibers. The Journal of the Textile Institute 112 (6):928–35. doi:10.1080/00405000.2020.1786209.
- Purnawati, R., F. Febrianto, I. Wistara, S. Nikmatin, W. Hidayat, S. Lee, and N. Kim. 2018. Physical and chemical properties of kapok (Ceiba pentandra) and balsa (Ochroma pyramidale) fibers.”Fibers.”. Journal of the Korean Wood Science and Technology 46 (4):393–401. doi:10.5658/WOOD.2018.46.4.393.
- Raeisian, L., Z. Mansoori, R. Hosseini-Abardeh, and R. Bagherzadeh. 2013. An investigation in structural parameters of needle-punched nonwoven fabrics on their thermal insulation property. Fibers and Polymers 14 (10):1748–53. doi:10.1007/s12221-013-1748-1.
- Rasheed, A., H. Zehra, S. Ahmad, and F. Ahmad. 2022. Development of multi-layer needle-punched nonwoven electric heating pad. The Journal of the Textile Institute 1–8. doi:10.1080/00405000.2022.2049049.
- Shabaridharan, M., and A. Das. 2013. Study on thermal and evaporative resistances of multilayered fabric ensembles. Journal of the Textile Institute 104 (10):1025–41. doi:10.1080/00405000.2013.771428.
- Vignesh, D., and, S.K., Laga. 2015. Kapok Fibre: A Perspective Fibre. https://www.semanticscholar.org/paper/Kapok-Fibre-%3A-A-Perspective-Fibre-%E2%80%9D-Dhanabalan/285a59dfc301047bb66b6c086dc0e2b425b5ec82
- Voumbo, M. L., A. Wereme, S. Gaye, M. Adj, and G. Sissoko. 2010. Characterization of the thermophysical properties of kapok. Research Journal of Applied Sciences, Engineering and Technology 2 (2):143–48.
- Yachmenev, V., I. Negulescu, and C. Yan. 2006. Thermal insulation properties of cellulosic based nonwoven composites. Journal of Industrial Textiles 36 (1):73–87. doi:10.1177/1528083706066439.