ABSTRACT
Textile substrates incorporated with antimicrobial agents offer a great advantage in treating chronic wounds infected with microbes. In this work, a simple one-step process is proposed to develop cellulosic nonwoven incorporated with silver nanoparticles using wet laying technique. In order to improve the dispersion of the fibers during wet laying and better binding of silver nanoparticles, the fibers were cationized using CHPTAC. The decrease in absorbance value of the drain at 470 nm confirms binding of nanosilver to the fiber. A maximum zone of inhibition of 18 mm and 19 mm was obtained for cationized web. The developed web can be used for wound dressing applications.
摘要
含有抗菌剂的纺织品基质在治疗感染微生物的慢性伤口方面具有很大优势. 在这项工作中,提出了一种简单的一步工艺,以使用湿铺技术开发掺有银纳米颗粒的纤维素非织造布. 为了改善湿铺过程中纤维的分散性和银纳米粒子的更好结合,使用CHPTAC对纤维进行阳离子化. 470 nm处漏极吸光度值的降低证实了纳米银与纤维的结合. 阳离子化纤维网的最大抑制区为18 mm和19 mm. 开发的网可用于伤口敷料应用.
Introduction
Infections found on the injured skin are the primary cause for delaying the healing of chronic wounds as they are caused due to the damage to the subcutaneous layer of skin. Utilization of antibiotics, antiseptics, and disinfectants in the area of wound management to promote healing has its own limitation as the materials do not offer resistance against the broad spectrum of microbes. Silver in ionic form gains a wide range of acceptance for treating the bacteria which are resistant to antibiotic. Metallic silver possesses no antibacterial activity, but silver in ionic form acts against infection-causing microbes such as bacteria, fungi, virus, and yeast. The bactericidal activity of the silver ion is due to the binding of the protein to the microbial cell wall leading to cell death (Khansa et al. Citation2019; Prabhu and Poulose Citation2012). Silver as nanoparticles is deployed in the area of antimicrobial applications due to its high reactivity and surface area (El-Aassar et al. Citation2020; Huang et al. Citation2022). Most of the time silver nanoparticles are incorporated in a medium, and in the case of dressing they are applied either by means of exhaustion, coating, doping into molten polymer or by spraying technique (El-Aassar et al. Citation2021; Gokarneshan and Velumani Citation2017; Gun Gok et al. Citation2019). Silver halide can be reduced into silver ions by physical, biological, or chemical means with the aid of capping agents such as polyvinyl pyrrolidone, poly(ethylene terephthalate), and polyvinyl alcohol in order to stabilize the silver nanoparticles (Gun Gok et al. Citation2021a; Gun Gok, Karayel, and Yigitoglu Citation2021b; Iravani et al. Citation2014). Silver ions from silver nitrate was formed by chemical means with the aid of the reducing agent dimethyl formamide which results in formation of nanoparticles with varying diameter (Pastoriza-Santos and Liz-Marzán Citation2000). The textile-based wound dressing gains an interest as it offers structural integrity thereby providing moist environment and also acts as secondary skin for healing of the chronic wounds (Leaper Citation2006). Textile-based wound dressings can be produced with ease with desired contour and with directional dimensional stability. Textile-based dressings are mostly in form of fibers, knits, and wovens. Among the fibrous dressing, nonwoven-based dressings are widely used as wound dressing as they are cost effective and drapeable. In the case of nonwoven web laying technique, wet laid technique utilizes aqueous solvent for dispersion of fibers followed by laying of web with fiber of shorter length (Hemamalini and Giri Dev Citation2021). Cellulosic short fibers from wood pulp are widely used for wound dressing application due to their liquid retention property which is a prerequisite for wound dressing. The interaction between fibrous substrate and nanoparticles can be enhanced by surface modification of the substrate as it adsorbs higher concentration of nanoparticles. Cationization of the cellulose substrate using CHPTAC enhances better distribution of fibers during wet laying technique and resists the microbial growth (Khalil-Abad, Mohammad, and Reza Nateghi Citation2009). The agent interacts with cotton by means of two steps such as chlorohydrin converting the reagent into epoxy intermediate followed by the reaction with cellulose thus resulting in the formation of cationized cellulose. Silver nanoparticles are firmly attached to the cationized textile substrate due to its extensive surface morphology that helps the nanoparticles to have better contact with the microbes. The usage of cationized fiber enhances the better binding of the silver nanoparticles on to the textile fibers and the resultant fibers can be used to produce nonwoven web. In the present work, surface modification of cellulosic fibers was carried out followed by adsorption of silver nanoparticles on to the fiber and then forming the fibrous web using wet laying technique. The resultant web was characterized for in vitro antibacterial activity for the efficacy against Gram-positive and Gram-negative bacteria.
Materials and methodology
Materials
Wood pulp was purchased from SITRA (South Indian Textile Research Association) Coimbatore. Dimethyl formamide (DMF), Acetic acid (AA), Sodium hydroxide (NaOH) were purchased from SRL chemicals (Sisco research laboratories) Mumbai. CHPTAC (3-chloro 2- hydroxy propyl trimethyl ammonium chloride) and silver nitrate (AgNO3) were purchased from Sigma Aldrich Corporation, USA.
Methodology
Surface modification of wood pulp was carried out by treating with CHPTAC cited in the literature (Hemamalini et al. Citation2021). In short, the opened fibers were treated with 10% NaOH at normal temperature for 15 minutes with material to liquor ratio of 1:30. The cationizing agent (65%) was further added to the slurry. The reaction was carried out at 80°C for 45 minutes. The slurry was filtered to drain the liquid and fibers were subjected to hot and cold wash followed by neutralization with 1% acetic acid, and subsequent aqueous washing. The treated fibers were dried to vaporize the moisture and used for web laying using wet laying non-woven. Incorporation of silver nanoparticle to the wood pulp was carried out by reducing the silver nitrate in DMF. The change in color indicates the conversion of AgNO3 to Ag+ ion. The fibers were treated with AgNO3 of different concentration such as 4 mM, 8 mM, 16 mM, and 20 mM with DMF to water ratio maintained at 1:2 prior to non-woven formation. The preparation of wet laid nonwoven webs are shown in . Laboratory-scale miniature wet laid non-woven setup was used for the production of non-woven comprising normal, cationized, and silver incorporated wood pulp fibers. The fibers of 1.5 g were dispersed uniformly in the water using mechanical stirring for a particular duration (15 minutes). The slurry was filtered and the web was formed on the sieve which was taken out and dried at vaporizing temperature to remove surface bound water.
Characterization
The interaction between cationizing agent and the fibrous web was studied using FTIR (Fourier Transform Infrared Radiation) spectrophotometer (Bruker, Japan). The spectrum was carried from 500 to 4000 cm−1 at room temperature. The conversion of silver nitrate to silver nanoparticle was confirmed using UV visible spectrophotometer. The drain from each sample were collected and the absorbance value was measured. The deposition of silver nanoparticles on the fibers in the web was observed using SEM-EDX (Edax Scanning Electron Microscope). The formation of silver nanoparticles was confirmed using transmission electron microscopy (TEM) micrographs (Tecnai G220 TEM, USA) with an accelerating voltage of 200 keV. The samples were prepared by evaporating a drop of nanoparticle solution onto a 200-mesh copper grid, which was coated with a carbon support film. The thermo stability of the developed samples was studied using thermo gravimetric analysis at a flow rate of 30 ml/min under nitrogen atmosphere with the rate of temperature increase of 20°C/min from room temperature to 700°C. The antibacterial activity of the control, cationized and samples incorporated with silver were evaluated using agar diffusion method against Gram-positive bacteria (E. coli) and Gram-negative bacteria (S. aureus). The agar medium was prepared and cast onto the petri plate over which 1 L of bacteria was inoculated. The samples of dimension (1 cm2) were placed and incubated for 24 hours to study the zone of inhibition.
Statistical analysis
Experiments were repeated three times and the data were expressed as mean ± standard deviation. Statistical analysis was carried out and p value ≤0.05 was considered significant based on the analysis of variance.
Results and discussion
Silver nanoparticles offers wide spectrum against the microbes by the interaction between silver ions and negatively charged cell wall of bacteria. The formation of silver nanoparticles was characterized using UV Visible spectrophotometer. The reduction of silver nitrate into silver nanoparticles were carried out using DMF as reducing agent which results in change in color from colorless to yellowish brown color. The drain from normal, cationized non-woven web was collected and the absorbance value was studied to correlate the adsorption of silver nanoparticles on the fibrous web as shown in . The peak around 470 nm confirms the reduction AgNO3 to Ag+ ions (Ashraf et al. Citation2016). It was clear from the figure that the absorbance value for cationized web was lower and it may be due to the interaction between cationizing agent and silver ions compared to that of the drain collected from the control wet laid samples.
The interaction between cationizing agent and silver ions with the wood pulp was confirmed using FTIR as shown in . The characteristic peak around 1690 cm−1 corresponds to the incorporation of cationic agent into the structure of cellulose which is due to the stretching of quaternary ammonium groups (NH3+) from CHPTAC which was not found in the normal wet laid nonwoven. This confirms the cationization of wood pulp which resulted in the surface modification of the fibrous substrate with cationic groups on the structure. The spectra showed the characteristic peak around 2900 cm−1 which corresponds to the vibrational stretching of C-H groups. The peak between 3000 and 3500 cm−1 can be related to the stretching of – OH (hydroxyl groups). The peak around 1200 cm−1 and 1730 cm−1 can be attributed to the C-N and C=O stretching in both cationized and normal incorporated with silver ions. There was no new peak indicating the silver ions forming chemical bonding with cationizing agent and wood pulp. Thus, the silver nanoparticles were physically adsorbed onto the structure of cationized and normal wood pulp nonwoven.
The deposition of silver nanoparticles onto the surface of fibers was analyzed using SEM analysis as shown in )-(4). Upon cationizaton of wood pulp fibers, the fibers were rough and flatten compared to normal wood pulp fibers due to incorporation of cationic groups onto the polymeric backbone as they exist interstitial position of the fiber (Ristić and Ristić Citation2012). The deposition of silver nanoparticles was more on the surface modified wood pulp fibers compared to normal wood pulp fibers due to the incorporation of quaternary ammonium groups on the surface of fibers which enhances the molecular interaction between nanoparticles and substrate. The EDX spectrum of the silver treated normal and cationized samples were shown in )-(4). The reduced silver nanoparticles were subjected to analysis with an optical absorption characteristic peak at 3keV. The relative composition of Ag on cationized samples was 79% and normal treated samples were 41% which can be attributed to the surface modification of wood pulp fibers. The silver nanoparticles were formed by reducing the silver nitrate using DMF which was then added to the slurry for the dispersion of normal and cationized fibers. The reducing agent converts the silver ion into zero valent silver which initiates the nucleation process, thereby resulting in nanoparticles formation (Andra et al. Citation2021). The reduction of silver nitrate to nanoparticles involves color change from colorless to reddish-brown color. The adsorption silver nanoparticles on the cationized fibrous web were analyzed using TEM techniques and it is shown in . It was found that the formed silver nanoparticles were in the range of 34 nm with irregular sphere morphology.
Figure 4. SEM of a) Normal b) Cationized wet laid samples, silver incorporated c) normal wet laid d) cationized wet laid samples, EDX of silver incorporated e) normal wet laid f) cationized wet laid samples.
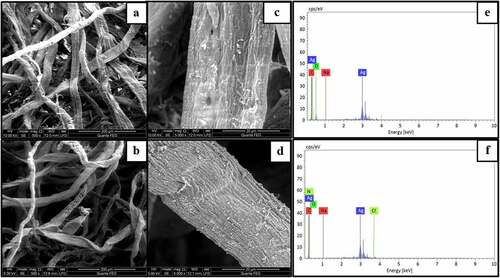
Figure 5. Different magnification of TEM images of silver incorporated cationized wet laid nonwoven (a) 200 nm (b) 50 nm (c) 20 nm.
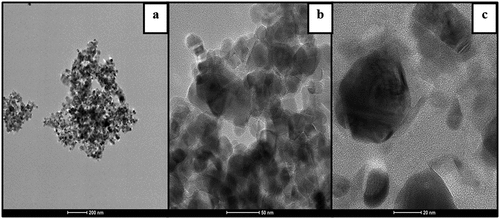
The thermal stability of the developed nonwoven with and without silver was analyzed and the thermo gram is shown in . The weight loss (%) of the test samples took place in three stages. The onset degradation of first stage began around 100°C which can be attributed to the loss of surface bound water. The second stage of degradation began at around 350°C corresponding to the decomposition of cellulose molecules in the case of normal and treated samples. In the case of the cationized samples, the degradation began around 300°C, indicating the breakage of bond between the cellulose polymeric chain caused due to the cationizing agent (CHPTAC). The third onset of degradation began around 400°C which can be attributed to the degradation of cellulosic polymer backbone. The addition of silver ions on the surface of the fibers increased the thermal stability and also reduced the weight loss (%) of the normal and cationized treated samples.
The antibacterial activity of silver incorporated samples was studied using Gram-positive (Escherichia Coli) and Gram-negative (Staphylococcus Aureus) bacteria using agar diffusion method. The activity was seen on the cationized samples due to the cationic charge whereas in normal treated samples inhibition of bacteria was not seen due to the presence of cellulosic material. It was found that increasing the concentration of silver nitrate resulted in increased inhibition of growth of bacteria. The zone of inhibition of developed samples is given in and the images are shown in . The anti-bacterial efficiency of cationized samples was higher compared to normal wood pulp which can be attributed to the presence of cationic groups in the fibers as it increases the interaction between the nanoparticles and bacteria, resulting in increase of reactive oxygen groups causing cell death of bacteria (Salvioni et al. Citation2017). The silver incorporated nonwoven web offered bacterial activity due to the presence of Ag+ attacks the negatively charged cell wall of bacteria, thereby causing cell lysis. Thus, deposition of silver nanoparticles was found to be higher in cationized samples with the maximum zone of inhibition of 18 mm and 19 mm against E. Coli and S. Aureus, thereby having a potential in antibacterial wound dressing. The cationization process was found to influence the adsorption of silver nanoparticles by means of immobilization and binding of reduced silver ions to increase the antibacterial efficacy.
Figure 7. Zone of Inhibition of developed control and cationized with silver nanoparticles incorporated wet laid samples of different concentration a) 0mm, b) 4mm, c)8 mM, d)12mm, e)16mm, f)20mm.
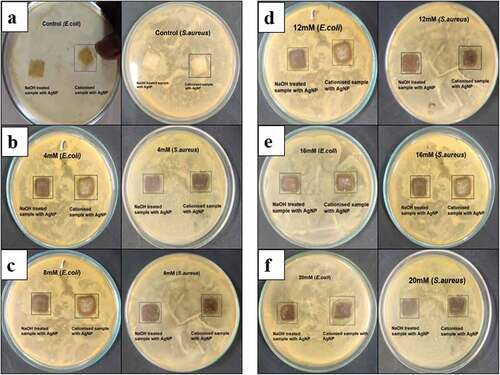
Table 1. Zone of inhibition of developed nonwoven samples.
Conclusion
In the present study, two major inferences can be drawn: that the cationization of fibers enhances the dispersion of fibers during web laying process and ensures the better binding of silver nanoparticles which inhibits the growth of bacteria. Moreover, as web laying involves dispersion of fibers in liquor, the incorporation of silver nanoparticles can be carried out during the process itself; thereby a cost-effective wound dressing can be produced. The developed fibrous web comprising silver nanoparticles offered activity against Gram-positive and Gram-negative bacteria with a Zone of Inhibition of 18 mm and 19 mm respectively with a potential of the substrate in wound dressing applications.
Highlights
Silver nanoparticles incorporated cellulosic nonwoven web was developed using wetlaying technique
Cationization of fibers was carried out to increase the binding of silver nanoparticles on the fibrous web
The developed fibrous web offered antibacterial activity of 18 mm and 19 mm against Gram-positive and Gram-negative bacteria
Consent for publication
All authors have agreed to publish this version of the manuscript.
Disclosure statement
No potential conflict of interest was reported by the author(s).
References
- Andra, S., S. Kumar Balu, J. Jeevanandam, M. Muthalagu, and M. K. Danquah. 2021. Surface cationization of cellulose to enhance durable antibacterial finish in phytosynthesized silver nanoparticle treated cotton fabric. Cellulose 28(9): 5895–9. Springer Netherlands. doi:10.1007/s10570-021-03846-2.
- Ashraf, J. M., M. Azam Ansari, H. M. Khan, M. A. Alzohairy, and I. Choi. 2016. Green synthesis of silver nanoparticles and characterization of their inhibitory effects on AGEs formation using biophysical techniques. Scientific reports 6(June 2015): 1–10. Nature Publishing Group. doi:10.1038/srep20414.
- El-Aassar, M. R., O. M. Ibrahim, M. M. G. Fouda, N. G. El- Beheri, and M. M. Agwa. 2020. Wound healing of nanofiber comprising Polygalacturonic/Hyaluronic acid embedded silver nanoparticles: In-vitro and in-vivo studies. Carbohydrate polymers 238:116175. doi:10.1016/j.carbpol.2020.116175.
- El-Aassar, M. R., O. M. Ibrahim, M. M. G. Fouda, H. Fakhrya Jamaan Ajarem, S. N. Maodaa, A. A. Allame, and E. E. Hafez. 2021. Wound dressing of chitosan-based-crosslinked gelatin/polyvinyl pyrrolidone embedded silver nanoparticles, for targeting multidrug resistance microbes. Carbohydrate polymers 255:117484. doi:10.1016/j.carbpol.2020.117484.
- Gokarneshan, N., and K. Velumani. 2017. Application of nano silver particles on textile materials for improvement of antibacterial finishes. Global Journal of Nanomedicine 2 (3):42–45. doi:10.19080/GJN.2017.02.555586.
- Gun Gok, Z., A. Demiral, O. Bozkaya, and M. Yigitoglu. 2021a. In situ synthesis of silver nanoparticles on modified poly(ethylene terephthalate) fibers by grafting for obtaining versatile antimicrobial materials. Polymer Bulletin 78 (12):7241–60. doi:10.1007/s00289-020-03486-9.
- Gun Gok, Z., K. Gunay, M. Arslan, M. Yigitoglu, and İ. Vargel. 2019. Coating of modified poly (ethylene terephthalate) fibers with sericin‑capped silver nanoparticles for antimicrobial application. Polymer Bulletin 77 (4):1–17. doi:10.1007/s00289-019-02820-0.
- Gun Gok, Z., M. Karayel, and M. Yigitoglu. 2021b. Synthesis of carrageenan coated silver nanoparticles by an easy green method and their characterization and antimicrobial activities. Research on Chemical Intermediates 47 (5):1843–64. doi:10.1007/s11164-021-04399-6.
- Hemamalini, T., and V. R. Giri Dev. 2021. Wet laying nonwoven using natural cellulosic fibers and their blends: Process and technical applications. A review. Journal of Natural Fibers 18 (11):1823–33. Taylor & Francis. doi:10.1080/15440478.2019.1701606.
- Hemamalini, T., J. Vrishni Ritvic, R. Premitha, A. K. Divya Dharshini, and V. R. Giri Dev. Taylor & Francis 2021. Facile and cost-effective development of silver based cellulosic wound dressing using electrospraying process. Journal of Natural Fibers 19 (14):1–9. doi:10.1080/15440478.2021.1946887.
- Huang, Y., L. Bai, Y. Yang Zhanhai Yin, and B. Guo. 2022. Biodegradable gelatin/silver nanoparticle composite cryogel with excellent antibacterial and antibiofilm activity and hemostasis for Pseudomonas aeruginosa-infected burn wound healing. Journal of Colloid and Interface Science 608 (3):2278–89. doi:10.1016/j.jcis.2021.10.131.
- Iravani, S., H. Korbekandi, S. V. Mirmohammadi, and B. Zolfaghari. 2014. “Synthesis of silver nanoparticles: Chemical, PhysicIravani, S., Korbekandi, H., Mirmohammadi, S. V, & Zolfaghari, B. (2014). Synthesis of Silver Nanoparticles: Chemical, Physical and biological methods. Research In pharmaceutical sciences, 9(6), 385–406.” Research in Pharmaceutical Sciences 9 (6): 385–406. http://www.ncbi.nlm.nih.gov/pubmed/26339255%0Ahttp://www.pubmedcentral.nih.gov/articlerender.fcgi?artid=PMC4326978.
- Khalil-Abad, S., M. E. Y. Mohammad, and M. Reza Nateghi. 2009. Effect of cationization on adsorption of silver nanoparticles on cotton surfaces and its antibacterial activity. Cellulose 16 (6):1147–57. doi:10.1007/s10570-009-9351-8.
- Khansa, I., A. R. Schoenbrunner, C. T. Kraft, and J. E. Janis. 2019. Silver in wound care - friend or foe?: A comprehensive review. Plastic and Reconstructive Surgery - Global Open 7 (8):1–10. doi:10.1097/GOX.0000000000002390.
- Leaper, D. J. 2006. Silver dressings: Their role in wound management. International Wound Journal 3 (4):282–94. doi:10.1111/j.1742-481X.2006.00265.x.
- Pastoriza-Santos, I., and L. M. Liz-Marzán. 2000. Reduction of silver nanoparticles in DMF. Formation of monolayers and stable colloids *. (May 2014). 10.1351/pac200072010083.
- Prabhu, S., and E. K. Poulose. 2012. Silver nanoparticles: Mechanism of antimicrobial. International Nano Letters 2 (1):32–41. doi:10.1186/2228-5326-2-32.
- Ristić, N., and I. Ristić. 2012. Cationic modification of cotton fabrics and reactive dyeing characteristics. Journal of Engineered Fibers and Fabrics 7 (4):113–21. doi:10.1177/155892501200700408.
- Salvioni, L., E. Galbiati, V. Collico, G. Alessio, S. Avvakumova, F. Corsi, P. Tortora, D. Prosperi, and M. Colombo. 2017. Negatively charged silver nanoparticles with potent antibacterial activity and reduced toxicity for pharmaceutical preparations. International Journal of Nanomedicine 12:2517–30. doi:10.2147/IJN.S127799.