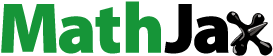
ABSTRACT
Water-soluble “sulfated polysaccharides of Chaetomorpha antennina” (CMsps) seaweed were isolated and fractionated (charged & neutral) using column chromatography followed by modification with acrylamide (AAm) to get novel hybrid materials. This polysaccharide comprises 6.356 polydispersity which ascribes to their branching behavior. The crude polysaccharides consist of ribose, arabinose, xylose, and galactose, carbohydrates units. The peak that appeared in the FT-IR spectrum at 1250 cm−1 confirms the sulfated nature of polysaccharides. The charged polysaccharides, pronounced “control CMsps” before the modification, were modified with AAm under microwave irradiation and characterized to investigate potential grafting. FT-IR, SEM, C13NMR, TGA, XRD, CD, and optical rotation were applied for control CMsps and CMsps-g-PAAm (grafted product). The FT-IR and SEM tools have significant capability to prove occurring successful potential grating with fluctuating peaks and morphology, respectively. XRD graphs for materials “control CMsps and CMsps-g-PAAm” reaffirm the successful grafting, leading to symmetrical molecular construction. TGA graphs support the exclusive thermal stability of CMsps-g-PAAm rather than control CMsps. The varying peak/trough ratio was evaluated by the CD spectrum, which identified the reversal change in the chirotopical profile with the insertion of polyacrylamide (PAAm) in polysaccharide chains. The materials, control CMsps and CMsps-g-PAAm were capable to develop hydrogel hence it can be exploited as a promising rheological modifier for food industry applications. The materials can also be useful in the biomedical field as demulcent drug formulation, dental impression product, and drug delivery for local therapy.
摘要
采用柱色谱法分离和分离(带电和中性)水溶性“安氏角藻硫酸多糖”(CMsps)海藻,然后用丙烯酰胺(AAm)修饰,以获得新的杂交材料. 该多糖包含6.356个多分散性,这归因于它们的分支行为. 粗多糖由核糖、阿拉伯糖、木糖和半乳糖组成. FT-IR光谱中1250 cm-1处出现的峰证实了多糖的硫酸化性质. 在微波辐射下,用AAm对修饰前的荷电多糖进行修饰,并对其进行表征,以研究潜在的接枝. 采用FT-IR、SEM、C13NMR、TGA、XRD、CD和旋光法对CMsps和CMsps-g-PAAm(接枝产物)进行了控制. FT-IR和SEM工具具有显著的能力来证明分别出现具有波动峰值和形态的成功的势光栅. 材料控制CMsps和CMsps-g-PAAm的XRD图证实了成功的接枝,导致对称的分子结构. TGA图支持CMsps-g-PAAm的专属热稳定性,而不是对照CMsps. 通过CD光谱评估了变化的峰/谷比率,该光谱确定了聚丙烯酰胺(PAAm)插入多糖链后脊骨局部轮廓的逆转变化. 该材料、对照CMsps和CMsps-g-PAAm能够开发水凝胶,因此它可以作为食品工业应用的一种有前途的流变改性剂. 这些材料还可用于生物医学领域,如去乳化药物制剂、牙印模产品和局部治疗药物输送.
Introduction
Polysaccharides are natural and renewable feedstock. It has taken advanced routes to prepare various high-performance macromolecules and derivatives for promising carriers in controlled drug delivery systems (Cheng et al. Citation2021; Cumpstey Citation2013; Hu et al. Citation2015; Qi et al. Citation2017). In recent times, microwave irradiated modification has received an advanced place for grafting with various monomers owing to their convenient approaches (Singh, Kumar, and Sanghi Citation2012). On the other hand, microwave irradiation progressing reaction concepts in organic chemistry were introduced in 1980 and more recently in polymer modification (Petit Citation2014). The microwave stimulation for the chemical reaction has been proved to alleviate limitations in the synthesis of grafted polysaccharides. Microwave treatment over to polysaccharides backbone has been recently classified in two manners: First, microwave initiated synthesis, in which alone microwave irradiation is used to create free radicals over to polysaccharides backbone, from where grafting start. Second, microwave-assisted synthesis, in which microwave irradiation and radical initiators are incorporated simultaneously to engender radical moiety over to polysaccharide backbone, thereby triggering grafting action (Mishra et al. Citation2011; Sen et al. Citation2012). The beauty of graft copolymerization is that the adjoined polymeric chains are held together really through chemical bonding as well as bring beneficial effects on their properties. By exploiting microwave irradiation, graft copolymers were efficiently synthesized by reacting numerous organic fragments such as acrylamide, acrylonitrile, and poly L-lactic acid (Jing et al. Citation2021; Salimi et al. Citation2016; Sorour et al. Citation2013).
Raw materials of polysaccharides obtained from marine species are exclusive as they are found abundantly in nature, environmental friendliness, inexpensive, renewable resource, stable and modifiable, as well as they express tremendous potential to produce commercial products (Jing et al. Citation2021). Marine polysaccharide-based products are in the form of hydrogel, sponges, film, and foam, and are widely used in the medical field. Marine sulfated polysaccharide exhibits antiviral activity against enveloped and non-enveloped viruses. This sulfated polysaccharide has become a significant upcoming source for making a broad range of antiviral drugs (Hans, Malik, and Naik Citation2021). So far, many research works on the modification of polysaccharides by exploiting multi-process involving prolonged reaction time have been mainly reported for triggering polysaccharide functionality (Cui and Zhu Citation2021; De Paepe et al. Citation2002). Seaweed polysaccharides have been attracted for a long time to research in the biomedical field, for instance: agarose provides service in electrophoresis and bacterial culture. Seaweeds polysaccharides are turned into hydrogel form and novel structure by a simple modification that exhibits revolutionary characteristics and has been employed as an antibacterial, tissue repairing, drug delivery, 3D cell culture, bioimaging, wastewater remediation, and other fields (Beaumont et al. Citation2021; Bi et al. Citation2021). The polysaccharide can be readily modified with small organic fragments through radical polymerization approaches as radical approaches have greater procurer-ability. Grafted or chemically amended materials of polysaccharides are quite sensitive to present absorption proficiently (De Paepe et al. Citation2002; Kumar et al. Citation2021a, Citation2021b).
As per our best knowledge, the first time GC-MS was applied for crude polysaccharides, charged polysaccharides, and neutral polysaccharides of CMsps and determined the composition of carbohydrate moieties (). In this context, the one-pot route of microwave triggered water-based grafting copolymerization over to charged polysaccharide leading free radical mechanism was carried out employing AAm moiety as monomeric units. This grafting action followed the free radical mechanism by applying water-soluble redox radical initiator “potassium persulfate.” This designed route was promising to engender CMsps-g-PAAm. FT-IR, SEM, NMR, TGA, and BET analysis tools were applied to confirm occurring potential grafting. The FT-IR, SEM, and NMR tools predominantly support the potential grafting to form novel hybrid material with fluctuating peaks and morphology. By investigating XRD graphs, the CMsps-g-PAAm was carried with crystalline peaks, which indicates that the reaction occurred leading to the symmetrical arrangement. The decrement observed in pore diameters of novel hybrid material in comparison to the control CMsps deduces the role of polyacrylamide (PAAm) to create hybrid material with a different type of molecular construction. The sufficient pore diameter of novel hybrid material compared to natural gases to be harnessed for adsorption to natural gases. The CMsps-g-PAAm was identified as enhanced thermal stability, weaker gelling strength in water, and a partially hydrophobic nature. This grafted copolymer exhibited gel thinning properties and water-holding capabilities, consequently, having the potential to be used as a formulation of moisturizer, catalytically, and active drug carrier.
Table 1. Physicochemical properties of control CMsps and CMsps-g-PAAm.
Table 2. Molecular weight determination of CMsps.
Table 3. The retention time for standard sugar and CMsps samples.
Experimental section
Materials and chemicals
Chaetomorpha antennina was harvested from Diu (020° 042.364 N, 070° 058.276 E) and Veraval (20° 54.932 N and 070° 20.842 E) that are on the west coast of India. That was much tufted, elongated, thick, and has around 4 to 5 cm long, emerging tufted parts of species enveloped firmly with rock segments to the coastal areas. Seaweed was collected by handpicking during low tide.
Analytical grades of methanol, potassium persulfate, acrylamide (AAm), isopropanol, and toluene were used and were purchased from Sigma-Aldrich, Mumbai, and Ranbaxy Chemicals, Mumbai, respectively.
Apparatus
A domestic microwave oven (LG make; 2700 watt) operating over a temperature range 40-100oC (Magnetrons are set at a frequency of 2450 MHz). A MILESTONE-START S microwave lab station for synthesis was also used (fixed frequency of 2,450 MHz) over set temperature parameters to prepare the hybrid material (CMsps-g-PAAm).
Isolation and purification
The flow chart of the isolation and purification process for polysaccharides is framed in . Seaweed was firstly washed with clean water and converted into powdered form by using a smaller pore of sieves. Before getting water-soluble polysaccharide, the dry algal powder was immersed in MeOH until ensuring green color become faint, that process was carried out to remove fat and pigment components from the taken algal powders. Defatted and depigmented alga (100 g) was soaked in 1 liter of distilled water and heated at 80°C for 3 h (twice) on a water bath (1:10 ratio of biomass and water). Thus, the prepared slurry was simply filtered out by using a fine pore sieve to isolate solid residue, and then the collected liquid portion was passed through the celite bed under vacuum using a Buckner funnel. The liquid portion was concentrated up to 1/3, centrifuged to remove the smaller debris, and precipitated by adding Isopropyl alcohol (IPA). Thereafter, the precipitate was dissolved in water, deproteinated with Sevag reagents, dialyzed against distilled water, and lyophilized to get crude polysaccharides. To conduct the fractionation of formed crude polysaccharide, this was applied to the DEAE cellulose column (45 × 8 cm) and collected fractions with eluting of ultrapure water, and various gradient concentrations of NaCl solution with a flow rate of 25 ml/h. The fraction obtained using ultrapure water as an eluting agent was kept in the successive process; concentrated, dialyzed, and freeze-dried, and eventually obtained neutral polysaccharides whereas obtained other fractions with various gradients of NaCl solution as an eluting agent was kept in the same successive processes, finally obtained charged polysaccharides. This charged polysaccharide was passed thoroughly from the column due to electrostatic interaction amid sulfate and sodium content of eluent.
Preparation of CMsps-g-PAAm
The preparation scheme for CMsps-g-PAAm through microwave grafting approaches is presented in . To carry out the grafting reaction of polysaccharide, firstly 1 g (w/v) charged polysaccharide (control CMsps) was dissolved in 100 ml of distilled water followed by the addition of 0.01 g (0.00037 mol/L) of potassium persulfate to ensure radical moiety over polysaccharide backbone. Thereafter, AAm was added in the prior solution with a range of 3.10 to 7.76 per mole equivalent of polysaccharide. This solution was then microwave irradiated under the set of 1000 C temperatures and sustained for 1.5 minutes ensuring a complete reaction. A microwave instrument was inbuilt of a magnetic stirrer the reaction mixture was stirred throughout the reaction. The reaction mixture altered milky whitish, seemingly owing to the conversion of AAm to polyacrylamide (PAAm), which was kept for cooling and then poured isopropyl alcohol (1:2.25 v/v) to obtain precipitate, followed by centrifugation was carried out at 8000 rpm for 3 minutes. The off-whitish precipitate (CMsps-g-PAAm) was obtained and kept for air drying. The non-reacted homo-polymer (PAAm) indulged with grafted polymer was detached by applying the Soxhlet extraction process with toluene for 1 h followed by further washing with isopropyl alcohol and vacuum drying.
Characterization
The samples prepared were characterized using various characterization techniques. Fourier transform infrared (FT-IR) spectrum was obtained by a Perkin-Elmer spectrum GX FT-IR system (USA). Samples were grinded by using the grinder, and changed to powders and were pressed with KBr followed by prepared pallets were used to measurement of FT-IR spectroscopy.
The morphology of the sample was studied by scanning electron microscope (SEM) using Carl-Zeiss Leo VP 1430 instrument. SEM was recorded by applying 20kV accelerating voltage and 9700× for control polysaccharides and 2600× for CMsps-g-PAAm.
13C NMR spectrum was analyzed using Bruker Ultrashield Spectrometer, Switzerland at 125 MHz. To record of NMR spectrum, the sample was thawed in D2O and DMSO (5:1) and performed at 70°C.
The Thermogravimetric (TGA) analysis for control CMsps and CMsps-g-PAAm was carried out using the Mettler Toledo TGA system, Switzerland, which temperature program was 30° to 600°C at a heating rate of 10°C per min.
Crystalline nature of control CMsps and CMsps-g-PAAm were assessed using Powder X-ray diffraction (XRD) analysis on a Philips X’ pert MPD Powder Diffractometer using 2Ɵ = 5 to 60°.
For the determination of molecular weight, the gel permeation chromatography was carried out on Waters alliance HPLC, with Waters 2695 separation modules and 2414 refractive index detector. The column was operated at 30oC. The molecular weight was determined by using standard curve which was calibrated 10 Dextran standards (Molecular weight:6.68 × 105; 2.73 × 105; 4.86 × 104; 5.2 × 103 Da). Polydispersity and area measurement was calculated using standard calibration curve by inbuilt Empower2 software.
The metals were detected using Inductively Coupled Plasma Optical Emission Spectrometer (ICP-OES) with RF power 1.20 kW, plasma flow 15.0 L/min, and auxiliary flow 1.50 L/min.
GC-MS analysis of the alditol acetates of polysaccharides samples as well as standard sugar samples were carried out on a Shimadzu GC-MS-QP2010 machine, using a SGE BP-225 capillary column (25 m, 0.25 µm, 0.22 mm), employing temperature programming (160oC to 230oC, 10oC per min) using helium as a carrier gas at a constant flow rate of 1 ml/min. The electron impact (EI) mass-spectra were recorded at 70 eV and the retention time of the polysaccharide’s samples were compared with those of alditol acetate of individual standard sugar.
Optical rotation for control CMsps and their CMsps-g-PAAm were designed at 589 nm and 30°C by using Rudolph Digi-Pol 781 polarimeter instrument, USA. The circular dichroism of this polysaccharide was identified on a JASCO model J-815 CD spectrometer. The range of wavelength and concentration for studying CD was determined, 190 nm to 250 nm and 0.8 mg/ml (800 ppm) respective.
The specific surface area, pore-volume, and pore size distribution for calcined samples were evaluated from N2 adsorption-desorption isotherms at 77.4 K employing volumetric equation adsorption equipment (ASAP 2010, MicroMetrics, NH), using BET and BJH models.
Result and discussion
The estimations were employed to determine the percentage of total sugar content and sulfate content by using the Dubois method and turbidimetric method, respectively. This estimation showed the percentages such as 39.6% and 11.9% exhibiting the amount of total sugar content and sulfate content in polysaccharides, respectively.
Performance of grafting over to polysaccharide backbone fluctuates with changing amount of chemical influencer and physical influencer. We had carried out the study of reaction influencers and then displayed the grafting parameters that were assigned to successful grafting reactions. Chemical influencer deals with the “ratio of AAm to polysaccharide,” and “initiator concentration.” A physical influencer deals with the time and temperature to get optimized results.
Evidence of grafting
Effect of ratio of AAm (acrylamide) to polysaccharide
The ratio of AAm and polysaccharide affects to grafting parameters was studied and concluded when the ratio was 2:1 respectively then Gr and Ge were reached at maximum value and thereafter decreased gradually (). That reaction occurs when added water-soluble potassium persulfate because this has accountability to generate radicals over to polysaccharide backbone. When increases the ratio of polysaccharide to AAm with the presence of reaction initiator then engendering CMsps-g-PAAm moiety that could act as a self-emulsifier to adsorb more monomers on polysaccharide surface, subsequently enhanced the rate of graft reaction until the ratio of AAm to polysaccharide reached 2:1 respectively. That optimizing ratio signified the maximum grafting, and the below value of this ratio led to promote the preparing graft polymer and above the value of this ratio led to decreasing graft reaction.
Effect of initiator concentration
Potassium persulfate (KPS) as chemical initiator was used to trigger graft reaction in radical polymerization style with ranging 0.007 to 0.15 g (2.5 × 10−4 to 5.5 × 10−3 mol/L) and studied their impact on to chemical reaction (). It was found that the lower concentration of potassium persulfate was not sufficient to break the O-H bond in a homolytic manner under applying microwave irradiation conditions. The grafting parameters gently increased up to 0.08 g and beyond this amount grafting parameters decreased which symbolized side reaction i.e., homopolymerization.
Effect of reaction time
Grafting reaction carries reaction time under microwave stimuli was optimized and the given assumption for this grafting reaction was that the grafting reaction follows maximum toward the graft product until a specific time (). As anticipated, that polysaccharide and AAm grafting reaction has taken 110 seconds to reach maximum Ge and Gr and beyond this value ratio gently declined. The trace amount of sulfuric acid and KOH produces with exposure to microwave irradiation. When increased microwave exposure time, the balance of generating sulfuric acid and KOH become unbalanced thereby medium travel in lower pH, disintegrating the product.
Effect of reaction temperature
The graft reaction triggers fluctuating temperature when constant to other reaction conditions (). In which we had determined the level of optimized temperature where Gr and Ge receive peak points. This microwave-induced reaction was studied with changing temperature range and investigated that the particular temperature was reliable for individual reaction. That applying temperature uplifts the vibration of molecules causing activation energy for graft reaction to decrease with increasing rate of reaction. When the applied temperature is uplifted excessively thereby vibration of molecules becomes faster, causing the destruction of the polymer chain, resulting in Gr and Ge values becoming lower. These noteworthy results were not achieved in terms of generating products when the temperature was lesser than 700C. For this reaction, the temperature was optimized for maximum grafting ratio and grafting efficiency, to be 95°C.
Yield and grafting parameters
Yield of grafted product was 2.51 g. Grafting parameters such as total conversion (Ct), Grafting ratio (Gr), grafting efficiency (Ge), add-on (Ad) and homopolymer content (Hp) was determined using equation 1 to 5 respectively. To study of these parameters, the value for weights of initial substrate (W0), monomer used (W1), product mixture (W2) (i.e., copolymer and homopolymer) and pure graft copolymer (W3) were Wo = 1 g; W1 = 2 g; W2 = 2.51 g and W3 = (2.51–0.10) g = 2.41 g (unreacted PAAm homopolymer was 0.10 g) thereby grafting parameters value were calculated following; Ct = 1.26 (±0.010); Gr = 2.30 (±0.020); Ge = 0.96 (±0.033); Ad = 0.58 (±0.025); Hp = .04 (±0.0002).
Physicochemical studies
Physicochemical studies were carried out for control CMsps and CMsps-g-PAAm to know what changes are exhibited in their physical properties when executing graft reactions. Comparative ideas are expressed in . The control CMsps of the soft hydrogel of gelling strength is a little bit larger as compared to modified polysaccharides at 200C. A similar thought process was established when calculating gelling and melting temperature of the hydrogels. Hence, evaluating all reports shares the information that CMsps-g-PAAm gel was weaker than the corresponding control CMsps gel. The bulk density, true density, porosity, and pore volume have been marginally altered through modification, control CMsps to CMsps-g-PAAm, and could be due to somewhat transformation of particles size, shape, and distribution of the materials. The decrement found in porosity by modification leads to the formulation of nano-porous structure of copolymer.
Determination of molecular weight
The gel permeation chromatography was applied to determine the molecular weight for taken seaweeds in which value calibration was done using standard dextran curve (). The assigned average molecular weight and polydispersity were 1.12 × 106 and 6.356 respectively. The high polydispersity index pointed out that the polymer of CMsps was highly branched leading to non-uniform construction (Tsoukatos, Pispas, and Hadjichristidis Citation2001).
Detection of metal ions
ICP-OES detected various metal ions in polysaccharides as follows: K (37.14 ppm), Mg (2.85ppm), Na (8.27ppm), Zn (0.056ppm), Sr (0.077), and S (1.7ppm). The absence of highly toxic metals such as Cd, Pb, Ba, and As, in polysaccharides, thereby suggested that it may be suitable for ingestible application. The detection of sulfur in polysaccharides verifies to sulfate contained polysaccharides.
GC-MS analysis
The retention time (in minutes) for standard sugar moieties was calculated by the GC-MS tool and compared the retention time of the CMsps sample (crude, charged, & neutral) (). It was observed that the retention values of those weren’t an appreciable difference, which help the design of carbohydrate profile. The highly matched retention value of standard sugar moieties and CMsps sample discloses the presence of carbohydrate profile in crude, charged, neutral fraction of polysaccharides. showed that charged polysaccharides constructed only arabinose and galactose sugar. The crude part was constructed with ribose, arabinose, xylose, and galactose units. However, the neutral polysaccharides constructed with ribose, arabinose, xylose, and galactose.
Structural characterization of polysaccharide
FTIR analysis
The FTIR spectroscopy data for control CMsps and CMsps-g-PAAm are illustrated in . The demonstrated validates that control CMsps has been modified with AAm under microwave irradiation. The stronger and broader stretching bands appeared between 3435 cm−1 to 3438 cm−1 reveal to the existence of O-H groups. The stronger, stretching peak that appeared (Crude & control CMsps) at 1250 cm−1 implies the presence of sulfate contents, thus verifying the sulfated nature of polysaccharides. The sulfate content determining peak (1250 cm−1) did not appear in neutral polysaccharides. The characteristics bending IR peaks emerged around 830 cm−1 to 995 cm−1 assigns the β-skeleton between the basic carbohydrates moieties and the peaks emerged at 897 cm−1 to 1160 cm−1 range endorse to the β-linking between carbohydrate moieties (Zhua et al. Citation2022). Compared FT-IR spectrum of CMsps-g-PAAm and control CMsps, CMsps-g-PAAm spectrum accommodated with unique peak at 1665 cm−1 that confirmed the grafted of amide group (Ye and Yang Citation2015). These characteristic bands have suggested the successful grafting reaction transported under microwave irradiation.
SEM analysis
To understand the surface morphology for control CMsps and CMsps-g-PAAm, their SEM pictures are displayed in and manifested after carefully observing SEM pictures that both are morphologically different resulting in successfully conveyed grafting action. The control CMsps appeared compact and cloudy morphology however it being modified their morphology was altered in form of definable shapes similar to distorted rectangular/triangular that also endorsed in XRD graphs. This point recommends the substantial grafting of PAAm over to the polysaccharide backbone to make integrated molecular construction.
13C NMR spectroscopy
NMR is a proficient tool to provide detailed structural information about carbohydrates moieties. The charged polysaccharides containing arabinose and galactose units are proved by CP-MAS13CNMR (). The peaks originated at [98.8 ppm], [74.3 ppm & 71.3 ppm], [66.03 ppm & 65.0 ppm], [95.2 ppm], [74. 38 ppm, 66.0 ppm & 71.3 ppm], and [74.3 ppm & 62.1 ppm] were designated for C-1 of arabinose ring (anomeric carbon), C-2 & C-3 of arabinose moiety, C-4 & C-5 of arabinose moiety, C-1 of galactose moiety (anomeric carbon), C-2, C-3 & C-5 of galactose moiety, and C-4 & C-6 of galactose moiety, respectively (Pomin Citation2012; Ye and Yang Citation2015). The peak appeared at 179.45 ppm () assigned for the carbonyl carbon of grafted PAAm () (Al-Sabagh et al. Citation2013). The altered δ values were observed, which cedes the endorsement of integrated molecular construction. In the CMsps-g-PAAm the peaks of anomeric carbon of arabinose and galactose moieties were displayed at 98.1 ppm and 99.85 ppm whereas in control CMsps the peaks displayed 98.8 ppm and 95.2 ppm, respectively, thus we may say that the chemically fixing of monomeric moieties have been proficiently done over to polysaccharide backbone. In ), the peaks assigned for control CMsps and their modified product (CMsps-g-PAAm) are fluctuating, which endorses the successfully conveyed grafting action under microwave irradiation.
Thermogravimetric analysis (TGA)
The consequences of thermal properties for control CMsps and CMsps-g-PAAm were depicted in . The weight losses for CMsps-g-PAAm happened in three stages; ca. 10% weight loss carried between 45-182°C presumably owing to emit of bounded water content (moisture) to the polysaccharide, 37% weight loss carried between 183-248°C presumably due to degradation of polysaccharide chains, and finally ca. 57% weight loss was observed up to 600°C presumably due to the degradation of the cross-linked grafted product. Control CMsps also displayed weight loss in three steps, the first one (13%) happened in the range 44-207°C, presumably due to emitting of moisture content. The following mass losses were 33% and 88%, carried out on this temperature ranges 210-251°C and 251-550°C respectively, presumably owing to degradation of polymer branches (Shi et al. Citation2019). Thereby, we conclude that the grafted moiety altered exclusively thermal stability compared to control CMsps; presumably as a result, the executed grafting reaction is evident for somehow systematic molecular association of PAAm with respective polysaccharides.
XRD analysis
The XRD patterns furnished the characteristic opinion about amorphous and crystalline behavior in the control CMsps and CMsps-g-PAAm (). The XRD patterns for control CMsps exhibited no significant sharp and narrow peak signifying to their amorphous nature whereas in XRD patterns for CMsps-g-PAAm visualized three distinguishable peaks; 2θ = 29.7°, 31.7o, and 49.1°. The result manifests the ordered molecular association of PAAm with respective polysaccharides whose intensifying crystallinity appeared in XRD pattern is evident (Prasad et al. Citation2006). The crystallinity index (C.I.) value for CMsps-g-PAAm was evaluated using an equation elaborated by Parihar & Kumar (Parihar et al. Citation2021).
The calculated C.I. value for CMsps-g-PAAm was 0.28 whereas control CMsps was indulged with a fully amorphous nature evoking in SEM photos.
Optical rotation and circular dichroism
The specific optical rotation {[∝]25589(c 0.20, H2O)} values for control CMsps and PAAm were [+177.8°] and [+3.21°] respectively, whereas that chemically attached grafted product, CMsps-g-PAAm, was [+77.39°] under the same conditions. The alteration of optical rotation after the modification underscored that the construction of PAAm functionalized polysaccharides has occurred.
The CD spectra to understand chiroptical profiles of control CMsps, polyacrylamides and CMsps-g-PAAm were demonstrated in . The CD spectra of control CMsps were observed entirely in the negative zone; (peak 194.5 nm, [θ] −282.46 and trough 199 nm, [θ] −1562.66), however, their modified form of CD spectra was developed in a mixed pattern having positive and negative zones; (peak 196.9 nm, [θ] +24.2 and trough 199.0 nm, [θ] −38.76). The CD spectrum of PAAm exhibited positive and negative bands, (peak at 218.0 nm, [θ] +68.3) and a trough at (c.a.214 nm, [θ] −33.9).
If the ratio of the peak of highest and trough of depth (peak/trough) observes at lesser than one value which leads to the negative CD spectrum (i.e., peak/trough <1), however, their vice versa situation led to the positive CD spectrum (i.e., peak/trough >1). The peak/trough ratio was designed using equation (θpeak– θtrough)/θtrough (Dentini et al. Citation2006; Sanandiya and Siddhanta Citation2014). The peak/trough ratio for control CMsps was 0.81 (i.e., Peak/trough <1) while grafted and PAAm were 1.50 and 2.8 respectively, i.e., peak/trough >1. Consequently, the reversal of peak/trough ratio assigns the alteration of chiroptical profiles when insertion of PAAm occurred in control CMsps leading to alteration of symmetry elements.
Average pore diameter and surface area (BET)
The pore diameter and surface area for control CMsps and CMsps-g-PAAm were determined by hiring N2 adsorption-desorption isotherms using BET and BJH models. assigns that the hybrid material has a markedly lower surface area in comparison due to molecular arrangements through the grafting of PAAm. The presence of significant pore diameter (nm) in CMsps-g-PAAm, 2.06 nm (BET), 7.47 nm (BJH-adsorption), and 3.801 nm (BJH-desorption), endorses the formulation of porous hybrid materials. Herein, may be noted that the hybrid material of pore diameter (BET) is 2.06 nm (.), which is sufficient for natural gases and adsorbents such as oxygen (0.29 nm); nitrogen (0.31 nm); CO2 (0.28–0.34 nm); NOx gases (<0.5 to 1.0 nm); preferred zeolites (0.5 to 1.0 nm), CH4 (0.29–0.38 nm), hence this hybrid material could be potentially used as an adsorbent (Prasad et al. Citation2009).
Table 4. Measurement of surface area and pore diameter by N2 adsorption-desorption isotherms using BET and BJH models.
Anticipated mechanistic approaches
illustrates the anticipated mechanistic approaches of PAAm over to charged polysaccharides to engender their novel hybrid product employing redox initiator, “potassium persulfate.” The potassium sulfate radical anion produced as a result of microwave irradiation is capable to remove a hydrogen atom from a hydroxy group of monosaccharide moieties followed by constructs correspondingly alkoxy radical on the C6 carbon atom of carbohydrate moieties (Prasad et al. Citation2009). The alkoxy radical causes to attach monomeric units over polysaccharide with successive forwarding of radical until dead polymer. Formed KOH and sulfuric acid balance the pH of the reaction medium.
Conclusion
Rapid water-based synthesis of novel hydrogel copolymer of sulfated polysaccharide with PAAm was employed under microwave irradiation in presence of water-soluble polysaccharide. GC-MS analysis indicated that the charged polysaccharides were integrated prominently with galactopyranose and arabinopyranose carbohydrate units. To obtain optimal grafting percentage, the employed polysaccharide-AAm and KPS doses were 1:2 ratio and 2.9 × 10−3 mol/L, respectively, used in the reaction mixture. FT-IR, CP-MAS 13C-NMR, SEM, CD, TGA, XRD, and BET measurements validated that grafting reaction indeed occurred. By investigating XRD graphs, the CMsps-g-PAAm was carried with crystalline peaks, which indicates that the reaction occurred leading to the symmetrical arrangement. The decrement observed in pore diameters of hybrid material in comparison to the control polysaccharides deduces the role of PAAm to create hybrid material with a different type of molecular construction. Due to the larger pore diameter of hybrid material compared to natural gases to be harnessed for adsorption to natural gases. The novel hybrid material was recognized as boosted thermal stability and crystallinity, weaker gelling strength in water, and partially hydrophobic nature. This grafted copolymer displayed gel thinning properties and water-holding capabilities accordingly this may consume as making of moisturizer, catalytically and active drug carrier. The grafting reaction has been rapidly undergone with interesting phenomenon in term of developed thermal stability, crystallinity as well as altered specific rotation that may help to popularize microwave irradiation technique for performing graft polymerization reaction.
Highlights
The water-soluble polysaccharides were isolated using reliable approaches.
Water-based chemical grafting was carried out to engender quality-maintained hybrid material.
The accomplishment time of reaction was lesser, hence evolved rapid targeting reaction.
XRD patterns endorses that the grafting process has been preceded with symmetric arrangement of molecules.
The pore diameter of hybrid material was observed bigger to some natural gases.
Disclosure statement
No potential conflict of interest was reported by the author(s).
Additional information
Funding
References
- Al-Sabagh, A. M., N. G. Kandile, R. A. El-Ghazawy, M. R. N. El-Din, and E. A. El-Sharaky. 2013. Synthesis and characterization of high molecular weight hydrophobically modified polyacrylamide nanolatexes using novel nonionic polymerizable surfactants. Egyptian Journal of Petroleum 22 (4):531–17. doi:https://doi.org/10.1016/j.ejpe.2013.11.007.
- Beaumont, M., R. Tran, G. Vera, D. Niedrist, A. Rousset, R. Pierre, V. P. Shastri, and A. Forget. 2021. Hydrogel-forming algae polysaccharides: from seaweed to biomedical applications. Biomacromolecules 22 (3):1027–52. doi:10.1021/acs.biomac.0c01406.
- Bi, S., D. Qin, S. Yuan, X. Chenga, and X. Chen. 2021. Homogeneous modification of chitin and chitosan based on an alkali/urea soluble system and their applications in biomedical engineering. Green Chemistry 23 (23):9318–33. doi:10.1039/D1GC03205D.
- Cheng, S., C. Zhang, J. Li, X. Pan, X. Zhai, Y. Jiao, Y. Li, W. Dong, and X. Qi. 2021. Highly efficient removal of antibiotic from biomedical wastewater using Fenton-like catalyst magnetic pullulan hydrogels. Carbohydrate polymers 262:117951. doi:10.1016/j.carbpol.2021.117951.
- Cui, R., and F. Zhu. 2021. Ultrasound modified polysaccharides: A review of structure, physicochemical properties, biological activities and food applications. Trends in Food Science & Technology 107:491–508. doi:10.1016/j.tifs.2020.11.018.
- Cumpstey, I. 2013. Chemical modification of polysaccharides. ISRN Organic Chemistry 2013:417672. doi:10.1155/2013/417672.
- Dentini, M., G. Rinaldi, A. Barbetta, D. Risica, and G. Skja. 2006. Acid gel formation in (pseudo) alginates with and without G blocks produced by epimerising mannuronan with C5 epimerases. Carbohydrate Polymers 63 (4):519–26. doi:https://doi.org/10.1016/j.carbpol.2005.10.017.
- De Paepe, I., H. Declercq, M. Cornelissen, and E. Schacht. 2002. Novel hydrogels based on methacrylate- modified agarose †. Polymer International 51 (10):867–70. doi:https://doi.org/10.1002/pi.945.
- Hans, N., A. Malik, and S. Naik. 2021. Antiviral activity of sulfated polysaccharides from marine algae and its application in combating COVID-19 : mini review agaran. Bioresource Technology Reports 13:100623. doi:https://doi.org/10.1016/j.biteb.2020.100623.
- Hu, X., W. Wei, X. Qi, H. Yu, L. Feng, J. Li, S. Wang, J. Zhang, and W. Dong. 2015. Preparation and characterization of a novel pH-sensitive Salecan-g-poly(acrylic acid) hydrogel for controlled release of doxorubicin. Journal of Materials Chemistry B 3 (13):2685–97. doi:https://doi.org/10.1039/c5tb00264h.
- Jing, X., Y. Sun, X. Ma, and H. Hu. 2021. Marine polysaccharides: Green and recyclable resources as wound dressings. Materials Chemistry Frontiers 5 (15):5595–616. doi:10.1039/D1QM00561H.
- Kumar, M., P. Singh, D. Parihar, P. K. Surolia, and G. Prasad. 2021a. Promising grafting strategies on cellulosic backbone through radical polymerization processes – a review. European Polymer Journal 152:110448. doi:10.1016/j.eurpolymj.2021.110448.
- Kumar, M., P. Singh, D. Parihar, P. K. Surolia, and G. Prasad. 2021b. Application of grafted cellulosic material as bioadsorbent for segregating of non-desirable content from waste water – a review. Materials Today: Proceedings 43:2903–08. doi:https://doi.org/10.1016/j.matpr.2021.01.168.
- Mishra, S., A. Mukul, G. Sen, and U. Jha. 2011. Microwave assisted synthesis of polyacrylamide grafted starch (St-g-PAM) and its applicability as flocculant for water treatment. International Journal of Biological Macromolecules 48 (1):106–11. doi:https://doi.org/10.1016/j.ijbiomac.2010.10.004.
- Parihar, D., M. Kumar, P. S. Gehlot, P. K. Surolia, and G. Prasad. 2021. Extraction and characterization of fractionated cellulose from acacia senegal. Journal of Natural Fibers 19 (15):10499–512. doi:10.1080/15440478.2021.1994092.
- Petit, C. 2014. Microwave-assisted modifications of polysaccharides. Pure and Applied Chemistry 86 (11):1695–706. doi:https://doi.org/10.1515/pac-2014-0711.
- Pomin, V. H. 2012. Unravelling glycobiology by NMR spectroscopy, in glycosy. ed. InTech. https://www.intechopen.com/chapters/39448
- Prasad, K., G. Mehta, R. Meena, and A. K. Siddhanta. 2006. Hydrogel-forming agar-graft-PVP and κ-carrageenan-graft-PVP blends: rapid synthesis and characterization. Journal of Applied Polymer Science 102 (4):3654–63. doi:https://doi.org/10.1002/app.24145.
- Prasad, G., K. Prasad, R. Meena, and A. K. Siddhanta. 2009. Facile preparation of chaetomorpha antennina based porous polysaccharide – PMMA hybrid material by radical polymerization under microwave irradiation. Journal of Materials Science 44 (15):4062–68. doi:https://doi.org/10.1007/s10853-009-3586-5.
- Qi, X., W. Wei, J. Li, G. Zuo, X. Pan, T. Su, J. Zhang, and W. Dong. 2017. Salecan-based pH-sensitive hydrogels for insulin delivery. Molecular pharmaceutics 14 (2):431–40. doi:https://doi.org/10.1021/acs.molpharmaceut.6b00875.
- Salimi, K., M. Topuzogullari, S. Dincer, and H. M. Aydin. 2016. Microwave-assisted green approach for graft copolymerization of L -lactic acid onto starch. Journal of Applied Polymer Science 133 (6):1–8. doi:https://doi.org/10.1002/app.42937.
- Sanandiya, N. D., and A. K. Siddhanta. 2014. Chemical studies on the polysaccharides of salicornia brachiata. Carbohydrate Polymers 112:300–07. doi:https://doi.org/10.1016/j.carbpol.2014.05.072.
- Sen, G., S. Mishra, G. U. Rani, P. Rani, and R. Prasad. 2012. Microwave initiated synthesis of polyacrylamide grafted psyllium and its application as a flocculant. International Journal of Biological Macromolecules 50 (2):369–75. doi:https://doi.org/10.1016/j.ijbiomac.2011.12.014.
- Shi, Z., C. Jia, D. Wang, J. Deng, G. Xu, C. Wu, M. Dong, and Z. Guo. 2019. Synthesis and characterization of porous tree gum grafted copolymer derived from prunus cerasifera gum polysaccharide. International Journal of Biological Macromolecules 133:964–70. doi:https://doi.org/10.1016/j.ijbiomac.2019.04.128.
- Singh, V., P. Kumar, and R. Sanghi. 2012. Use of microwave irradiation in the grafting modification of the polysaccharides – a review. Progress in Polymer Science 37 (2):340–64. doi:https://doi.org/10.1016/j.progpolymsci.2011.07.005.
- Sorour, M., M. El-Sayed, N. Abd, E. Moneem, H. A. Talaat, and H. Shalaan. 2013. Grafted acrylamide using microwave (MW) and ultraviolet (UV) techniques characterization of hydrogel synthesized from natural polysaccharides blend grafted acrylamide using microwave (MW) and ultraviolet (UV) techniques. Starch - Stärke 65 (1–2):172–78. doi:https://doi.org/10.1002/star.201200108.
- Tsoukatos, T., S. Pispas, and N. Hadjichristidis. 2001. Star-branched polystyrenes by nitroxide living free-radical polymerization. Journal of Polymer Science Part A: Polymer Chemistry 39:320–25. doi:10.1002/1099-0518(20010115)39:2<320:AID-POLA80>3.0.CO;2-N.
- Ye, D., and J. Yang. 2015. Ion-responsive liquid crystals of cellulose nanowhiskers grafted with acrylamide. Carbohydrate Polymers 134:458–66. doi:10.1016/j.carbpol.2015.08.025.
- Zhua, Z., J. Chena, Y. Chena, Y. Ma, Q. Yanga, Y. Fan, C. Fua, B. Jai, L. Rui, and L. Liao. 2022. Extraction, structural characterization and antioxidant activity of turmeric polysaccharides. LWT 154:112805. doi:10.1016/j.lwt.2021.112805.