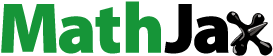
ABSTRACT
Respiratory tract infections represent the main cause of death from infectious diseases worldwide. SARS-CoV-2 infection (i.e. COVID-19) added to the existing global burden of respiratory tract infections, including tuberculosis. Among nanomaterials for fabric functionalization, graphene, in combination with hydrophobic molecules such as phytochemicals, represents a promising low-cost alternative to antibiotics. In this work, we used graphene and curcumin to create fabric coatings on cotton and polyester for the production of personal protective equipment resistant to infective agents. These coatings ensure the trapping of microorganisms via interaction with SARS-CoV-2 or mycobacteria surface and inhibit microbial infections.
摘要
呼吸道感染是全球传染病死亡的主要原因. SARS-CoV-2感染(即新冠肺炎)增加了包括结核病在内的现有呼吸道感染的全球负担. 在用于织物功能化的纳米材料中,石墨烯与疏水分子(如植物化学物质)结合,是一种有前途的低成本抗生素替代品. 在这项工作中,我们使用石墨烯和姜黄素在棉花和聚酯上制造织物涂层,用于生产抗感染剂的个人防护设备. 这些涂层通过与SARS-CoV-2或分枝杆菌表面的相互作用确保捕获微生物,并抑制微生物感染.
Introduction
Respiratory infections and pneumoniae remain a significant public health concern on a global scale and the top cause of morbidity and mortality from infectious diseases. SARS-CoV-2 infections rapidly went on top of the World Health Organization (WHO) Blueprint priority list, because of the morbidity and mortality associated with the disease (COVID-19). SARS-CoV-2 transmission was rapid and abrupt worldwide, posing a major threat that forced governments in most countries to impose several public health measures (Brauner et al. Citation2021; Talic et al. Citation2021).
Besides, other airborne-transmitted infectious diseases with epidemic or pandemic potential pose major challenges to public health authorities. Tuberculosis (TB), for example, is an ancient disease that kills approximately 1.8 million years and killed more than 1 billion people in the last 2000 years (Barbier and Wirth Citation2016) and its etiologic agent, Mycobacterium tuberculosis (Mtb) is usually transmitted in domestic and workplace settings and use of effective preventive measures may reduce contagion. Recently, the emergence of multidrug and extensively drug-resistant Mtb strains represent a global challenge that requires the adoption of urgent, effective and innovative preventive measures (WHO Citation2021).
The need for protection against SARS-CoV-2 and other airborne pathogens boosts the design of innovative personal protective equipment (PPE) (Palmieri et al. Citation2021). Nanoparticles, nanofibers, and other pioneering technologies based on nanomaterials have been introduced in PPE production chains to improve performance and confer properties that would limit microbial spreading through clothing. As an example, it has recently been demonstrated how Cu and Zn cotton functionalization can create human breath-activated fabric against Escherichia coli, Staphylococcus aureus, and Enterovirus 71 (Zhang et al. Citation2022). Similarly, chitosan and silver have been used to functionalize PPE (Zhang, Zhang et al. Citation2022; Zhu et al. Citation2022).
Among nanomaterials, graphene, the two-dimensional carbon-based material with exceptional light absorption and biological properties, has been studied recently as an antimicrobial agent for its ability to interact mechanically and chemically with lipid membranes, breaking the membranous ultrastructure of bacteria and enveloped viruses. Furthermore, graphene can be easily functionalized with hydrophobic molecules such as phytochemicals (Bugli et al. Citation2018; De Maio, F., V. Palmieri, A. Salustri et al. Citation2019; Palmieri and Papi Citation2020; Palmieri et al. Citation2018) plant derivatives that represent a promising alternative to synthetic chemical compounds, with antibacterial, antiviral, and immunomodulatory properties (Ayaz et al. Citation2019).
Curcumin, the principal curcuminoid of turmeric (Curcuma longa) alone or in combination with antibiotics, is known for its antibacterial properties for a long time both as coating and in soluble form (Bugli et al. Citation2018; Palmieri et al. Citation2018). Curcumin protects against pulmonary inflammation and acute lung injury generated during Klebsiella pneumoniae induced lung infection in vivo (Bansal and Chhibber Citation2010) and inhibits 3CL protease of SARS-CoV (Wen et al. Citation2007). Furthermore, curcumin is known to enhance human macrophage control of Mtb infection (Bai et al. Citation2016).
In this work, we used graphene and curcumin to create fabric coatings for PPE resistant to infective agents for the management of pandemic emergencies. Here, we report the functionalization of two commonly used fabrics for PPE production, i.e. cotton and polyester (PES), and first analyzed the effects on SARS-CoV-2 (wild-type clinical isolate) infectivity using African green monkey kidney cells (VERO cells, ATCC CCL-81) in biosafety laboratory level 3 (BCL3). Subsequently, we measured the efficacy of coatings on Mycobacterium smegmatis (Ms, mc2 155) as a model for Mtb infection. We demonstrate how the coating of graphene and curcumin represents a feasible functionalization for a broad-spectrum antimicrobial effect.
Curcumin–graphene coating on textiles inhibits SARS-CoV-2 infectivity
Fabric coatings have been prepared using graphene alone (G), curcumin alone (C), or a combination of the two (G/C); the resulting materials are shown in . Graphene nanoplatelets (G+, Directa-Plus) have been produced according to a proprietary patented technology that involves three different steps: expansion, exfoliation, and drying (Bonetti et al. Citationn.d.). A representative image of G+ obtained by atomic force microscopy and the corresponding height profile is reported in Figure S1. Full characterization of this nanomaterial is reported elsewhere (Cesareo, Parrini, and Rizzi Citation2020). Non-woven (200 gsm, 100% Polyester PES) and cotton fabrics (110 gsm, 100% cotton) were functionalized with G+ using impregnation method. In , we report experimental FTIR spectra for cotton and PES showing the functionalization of fabrics. Data indicate a successful coating for both samples. Coated cotton depicted two peaks in the range between 700 and 800 cm−1, which are associated with the stretching vibration of C–H in curcumin () (Ching et al. Citation2019). Two peaks (1270 and 1560 cm−1) can be observed, which correspond to C–O vibrations and C=N vibrations of curcumin, respectively (Ismail et al. Citation2014). A peak at 1603 cm−1 is associated with the stretching of the two phenolic groups of curcumin, and a peak at 1730 cm−1 indicates the stretch of the amide I band (Banerjee Citation2014). Finally, a peak at 3000 cm−1 indicates the presence of asymmetric and symmetric stretches typical of graphene (Hayyan et al. Citation2015). In , as visible for coated cotton, a clear peak at 800 cm−1 indicates stretching vibrations of C–H in curcumin on PES samples (Ching et al. Citation2019). Another peak, which is present only on coated PES, is visible at 1020 cm−1 and is associated with enhanced C–O–C stretching vibrations of curcumin (Chen et al. Citation2015). Similar to cotton, a peak at 3000 cm−1 indicates CH2 asymmetric and symmetric stretch of graphene.
Figure 1. (a) Representative images of coated cotton and PES fabrics functionalized with curcumin C graphene G (G+ Directa-Plus) and graphene/curcumin G/C. Functionalization with curcumin (CAS: 458-37-7, TCI Zentek), graphene or G/C has been performed according to literature (Cesareo, Parrini, and Rizzi Citation2020; De Maio et al. Citation2021). For the preparation of G/C fabrics, a ratio of 1:0.5 was used, corresponding to 1.4% of graphene and 0.7% of curcumin. (b) FTIR spectroscopy (OPUS spectrometer Bruker) of cotton and cotton G/C and (c) of PES and PES G/C is reported with characteristic peaks highlighted with dark circles. The material was directly laid on the ATR crystal and the spectra were recorded in the wavenumber range of 4000–5500 cm−1 according to the literature (De Maio et al. Citation2020). (d) Schematic representation of the experimental setting used to assay the antiviral activity of functionalized fabrics: 0.1 mL of SARS-CoV-2 solution (105 particles/mL) has been placed on the surface of each textile (1 × 1 cm) in separate wells of a sterile plate. After 2 h of incubation, fabrics were transferred in a new tube containing 5 mL of fresh cell medium and vigorously vortexed. Then 0.1 mL of recovered supernatants were used to infect VERO cells. Cell viability was assessed by using Crystal violet staining (Supplementary Figure 2) and quantitative analysis presented in the graphs for cotton (e) and PES (f). Monolayer integrity has been normalized on not infected cells. All experiments were analysed by using one-way ANOVA comparison tests followed by Tukey’s correction.
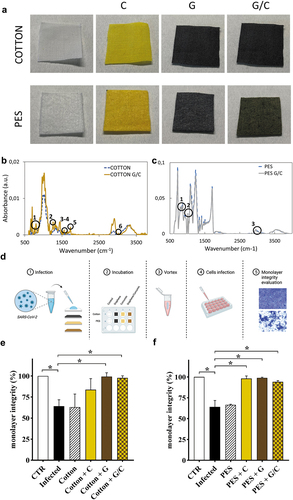
In , the experimental setting used to evaluate the effects of coatings on the virus is shown. The quantification of the effects on SARS-CoV-2 has been performed by evaluating the viability of VERO epithelial cells, by measuring monolayer integrity, after exposure to solutions obtained from fabrics washed after contact (2 h) with SARS-CoV-2. Cell monolayer integrity has been evaluated on each image using ImageJ software using a threshold for image binarization and calculating the percentage of black pixels (uncoated petri) and white pixels (cells). ANOVA test was used to evaluate significanct differences among infected cells and uninfected cells, or cells infected with SARS-CoV-2 exposed to fabrics. Values of p < .05 were considered as significant. In Figure S2, representative images of cell monolayers are reported.
displays how cotton or PES alone are not effective in blocking SARS-CoV-2. Concerning cotton, the addition of C slightly improves the ability of cotton to reduce SARS-CoV-2 infection.
Additionally, we then calculate the viral infectivity reduction from the following equation:
where Treated, CTR and Infected are respectively the percentage of monolayer integrity observed for cells infected treated with viruses recovered from fabrics, cells not infected and cells infected with the virus (De Maio et al. Citation2021, Citation2022). A ≈50% reduction of viral load is visible for cotton C treatment, though a marked reduction in infectivity has been achieved only after coating cotton with G or G/C (≈99% and ≈98% reduction) (). Interestingly, PES coating with C, G or G/C show a significant reduction in SARS-CoV-2 infectivity (≈98%, 98%, and 94%, respectively) ().
It has been previously shown that graphene is efficient in inhibiting SARS-CoV-2 infectivity thanks to the trapping effect on viral particles (De Maio et al. Citation2021, Citation2022); viral particles are stacked to graphene flakes on fabric coatings and consequently are not released from fabrics (Ye et al. Citation2015). The interaction between the SARS-CoV-2 particle and graphene, which occurs via π−π stacking and hydrophobic interactions, significantly affects the secondary S protein structure (Du et al. Citation2021). The activity of curcumin against other coronaviruses is known since 2007 (Wen et al. Citation2007). Here we demonstrate the antiviral potential of curcumin-coated surfaces that, given curcumin insolubility in water, may result from the accumulation of the lipophilic molecule on the envelope membrane, thus affecting lipid order and overall viral stability (Duda, Cygan, and Wisniewska-Becker Citation2020). Our findings are in line with recent in silico studies showing that curcumin has a high-affinity for the S glycoprotein of SARS-CoV-2, ACE2 receptor and the transmembrane protein serine protease 2 (TMPRSS2), which facilitates the entry of SARS-CoV-2 in the host cells (Maurya et al. Citation2020; Rattis, Ramos, and Celes Citation2021).
Curcumin–graphene coating disrupts the integrity of mycobacteria membrane and prevents spreading from contaminated fabric
Besides SARS-CoV-2, many viral and bacterial agents are airborne transmitted and are responsible for major infectious diseases, first and foremost Mtb. We analyzed the effects of coatings on Ms, which in this context can be considered an experimental model for Mtb infection and eventually other mycobacteria known to cause human disease following aerogenic exposure () (De Maio, F., V. Palmieri, M. De Spirito et al. Citation2019; De Maio, F., V. Palmieri, A. Salustri et al. Citation2019; De Maio et al. Citation2020). ANOVA test was used to evaluate significant differences among Ms exposed to fabrics and mycobacteria of the infecting solution. Values of p < .05 were considered as significant.
Figure 2. (a) Schematic representation of the experimental setting performed to test anti-mycobacterial activity of functionalized fabrics using Mycobacterium smegmatis (Ms). 0.1 ml of a solution containing ≈ 105 CFU/ml of Ms were directly placed on the surface of each textile (1 × 1 cm2) in separate wells of a sterile plate. After 4 h of incubation, textiles were transferred in a new tube containing 1 ml of sterile PBS. Tubes containing textiles were vigorously vortexed and serial dilutions of supernatants were plated on 7H11 solid medium to determinate colony forming units (CFUs) per mL and reported in (b) for PES and (c) for cotton. Furthermore, after vortexing, each fabric was placed on 7H11 solid medium with the infected functionalized side facing towards the plate. One hour post incubation at 37°C, textiles were removed from the medium and petri dishes were incubated at standard atmosphere conditions, results are in (d) for PES and (e) for cotton. (f) Representative SEM images of Ms on cotton fabric with different coatings have been acquired using SEM SUPRA 25 (ZEISS) after dehydration and sputter coating. Scale bar is 2 μm; yellow arrows indicate membrane damages. All experiments were analysed by using one-way ANOVA comparison tests followed by Tukey’s correction.
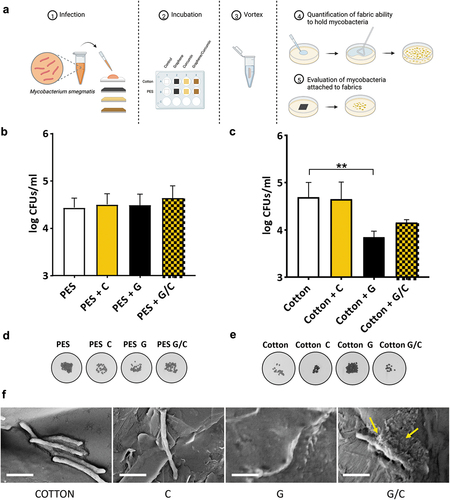
To measure the effect of coatings, we quantified the ability to hold bacteria after vortexing contaminated fabrics and evaluated the bacteria that remained attached ().
PES or PES coated with C, G or G/C displayed similar effects on bacteria with an overall reduced recovery of colonies after vortexing compared to bacteria in the infecting solution 105 CFU/mL (). By plating the colonies that remained on the fabric (), it is possible to observe that bacteria continued growing on PES during the incubation, while the functionalized fabric exerted a bacteria-killing effect similar for all conditions.
Unfunctionalized and C-coated cotton had a similar ability to retain bacteria (), yet CFU plating results shown in , demonstrate that bacterial loads are not reduced following contact with cotton or Cotton C.
Conversely, coating cotton with G led to a significantly stronger mycobacteria capture/trapping, as indicated by the reduced bacterial release after vortexing (≈40%). The combined use of G/C did not provide any additive effect (≈58% of released mycobacteria) (). Interestingly, in , the plating results show that while G functionalized cotton fabric retained a high number of living bacteria, a small number of colonies remained on G/C-coated cotton. In summary, Cotton G/C can avidly capture bacteria cells without provoking cell damage that may affect bacterial viability.
Scanning electron microscopy images confirmed that the contact between G/C functionalized cotton and mycobacteria affected the Ms cell wall integrity, that was otherwise unaffected by other conditions (). Indeed, as highlighted by the yellow arrows in , the mycobacterial surface loses its typical ultrastructure when in contact with G/C coatings, with debris released from the bacterial cell. Bacterial cell walls appear also damaged following contact with G but not following contact with control fabrics or C-treated samples.
We need to consider that the effects of coating hydrophobicity may differ depending on microbe size. Specifically, since cotton is hydrophilic and tends to retain moisture, the overall release of bacteria compared to PES is lower (one order of magnitude) and the addition of graphene enhances microbial trapping, alone or when combined with curcumin. This effect is probably mediated by the increase in fabrics’ hydrophobicity: hydrophobicity is an hallmark of Mtb and other pathogenic mycobacteria, suggesting that strong hydrophobic interaction may explain the ability of graphene-based fabrics to capture mycobacteria (Jankute et al. Citation2017). Hence, Cotton G/C or PES can properly expose curcumin, which is also a liposoluble drug, so to exert its known anti-mycobacterial and anti-biofilm properties (Barua and Buragohain Citation2021).
In summary, the G/C coating of fabrics is an effective option to reduce transmission of mycobacteria and SARS-CoV-2, and certainly other similar viruses and bacteria.
Conclusions
WHO constantly highlights the need for prioritization of personal protective equipment supplies for frontline health-care workers. Graphene–curcumin coatings can minimize the risk of transmission limiting pandemic spreading, with huge social and economic impact.
The poorest and most marginalized people across the world, who suffer most from TB and other infections, likely the most affected by the COVID-19 epidemic, can benefit from the use of PPE enriched with graphene–curcumin wide-spectrum antimicrobials.
Importantly, the prevention offered by graphene–curcumin coatings will avoid further transmission to health-care workers and limits pathogen spreading through waste in the environment. Furthermore, the combined mechanical and chemical effect of the two antimicrobial agents might reduce the appearance of resistant strains.
Supplemental Material
Download MS Word (3.7 MB)Acknowledgment
We acknowledge Directa-Plus Srl for providing materials used in this study. V.P. acknowledges for funding “One Health Basic and Translational Research Actions addressing Unmet Needs on Emerging Infectious Diseases – INFACT” – PNRR NextGenerationEU” Project. The authors declare no conflict of interests.
Disclosure statement
No potential conflict of interest was reported by the author(s).
Supplemental material
Supplemental data for this article can be accessed online at https://doi.org/10.1080/15440478.2022.2156020.
References
- Ayaz, M., F. Ullah, A. Sadiq, F. Ullah, M. Ovais, J. Ahmed, and H. Prasad Devkota. 2019. Synergistic interactions of phytochemicals with antimicrobial agents: potential strategy to counteract drug resistance. Chemico-Biological Interactions 308:294–8. doi:10.1016/j.cbi.2019.05.050.
- Bai, X., R. E. Oberley‐deegan, A. Bai, A. R. Ovrutsky, W. H. Kinney, M. Weaver, G. Zhang, J. R. Honda, and E. D. Chan. 2016. Curcumin enhances human macrophage control of mycobacterium tuberculosis infection. Respirology 21 (5):951–57. doi:10.1111/resp.12762.
- Banerjee, R. 2014. Inhibitory effect of Curcumin-Cu(II) and Curcumin-Zn(II) complexes on amyloid-beta peptide fibrillation. In Bioinorganic chemistry and applications, ed. I. O. Fritsky, Vol. 2014, 325873. doi:10.1155/2014/325873.
- Bansal, S., and S. Chhibber. 2010. Curcumin alone and in combination with augmentin protects against pulmonary inflammation and acute lung injury generated during Klebsiella Pneumoniae B5055-induced lung infection in BALB/c mice. Journal of Medical Microbiology 59 (4):429–37. doi:10.1099/jmm.0.016873-0.
- Barbier, M., and T. Wirth. 2016. The evolutionary history, demography, and spread of the mycobacterium tuberculosis complex. Microbiology Spectrum 4 (4):4. doi:10.1128/microbiolspec.TBTB2-0008-2016.
- Barua, N., and A. K. Buragohain. 2021. Therapeutic potential of curcumin as an antimycobacterial agent. Biomolecules 11 (9):1278. doi:10.3390/biom11091278.
- Bonetti, L., A. Fiorati, A. Serafini, G. Masotti, F. Tana, A. D’Agostino, L. Draghi, L. Altomare, R. Chiesa, and S. Farè. n.d. Graphene nanoplatelets composite membranes for thermal comfort enhancement in performance textiles. Journal of Applied Polymer Science 138:e49645.
- Brauner, J. M., S. Mindermann, M. Sharma, D. Johnston, J. Salvatier, T. Gavenčiak, A. B. Stephenson, G. Leech, G. Altman, and V. Mikulik. 2021. Inferring the effectiveness of government interventions against COVID-19. Science 371 (6531):eabd9338. doi:10.1126/science.abd9338.
- Bugli, F., M. Cacaci, V. Palmieri, R. Di Santo, R. Torelli, G. Ciasca, M. Di Vito, A. Vitali, C. Conti, M. Sanguinetti, et al. 2018. Curcumin-loaded graphene oxide flakes as an effective antibacterial system against methicillin-resistant Staphylococcus aureus. Interface Focus 8 (3):20170059. doi:10.1098/rsfs.2017.0059.
- Cesareo, G., M. R. Parrini, and L. G. Rizzi. 2020. “Continuous process for preparing pristine graphene nanoplatelets.”
- Chen, X., L.Q. Zou, J. Niu, W. Liu, S.F. Peng, and C.M. Liu. 2015. The stability, sustained release and cellular antioxidant activity of curcumin nanoliposomes. Molecules 20 (8):14293–311. doi:10.3390/molecules200814293.
- Ching, Y. C., T. M. S. U. Gunathilake, C. Hock Chuah, K. Yong Ching, R. Singh, and N.S. Liou. 2019. Curcumin/Tween 20-incorporated cellulose nanoparticles with enhanced curcumin solubility for nano-drug delivery: characterization and in vitro evaluation. Cellulose 26 (9):5467–81. doi:10.1007/s10570-019-02445-6.
- De Maio, F., V. Palmieri, G. Babini, A. Augello, I. Palucci, G. Perini, A. Salustri, P. Spilman, M. De Spirito, and M. Sanguinetti. 2021. Graphene nanoplatelet and graphene oxide functionalization of face mask materials inhibits infectivity of trapped SARS-CoV-2. Iscience 24 (7):102788. doi:10.1016/j.isci.2021.102788.
- De Maio, F., V. Palmieri, M. De Spirito, G. Delogu, and M. Papi. 2019. Carbon nanomaterials: a new way against tuberculosis. Expert Review of Medical Devices 16 (10):863–75. doi:10.1080/17434440.2019.1671820.
- De Maio, F., V. Palmieri, A. Salustri, G. Perini, M. Sanguinetti, M. De Spirito, G. Delogu, and M. Papi. 2019. Graphene oxide prevents mycobacteria entry into macrophages through extracellular entrapment. Nanoscale Advances 1 (4):1421–31. doi:10.1039/c8na00413g.
- De Maio, F., V. Palmieri, G. Santarelli, G. Perini, A. Salustri, I. Palucci, M. Sali, J. Gervasoni, A. Primiano, and G. Ciasca. 2020. Graphene oxide-linezolid combination as potential new anti-tuberculosis treatment. Nanomaterials 10 (8):1431. doi:10.3390/nano10081431.
- De Maio, F., E. Rosa, G. Perini, A. Augello, B. Niccolini, F. Ciaiola, G. Santarelli, F. Sciandra, M. Bozzi, and M. Sanguinetti. 2022. 3D-Printed graphene polylactic acid devices resistant to SARS-CoV-2: sunlight-mediated sterilization of additive manufactured objects. Carbon 194:34–41. doi:10.1016/j.carbon.2022.03.036.
- Duda, M., K. Cygan, and A. Wisniewska-Becker. 2020. Effects of curcumin on lipid membranes: an EPR spin-label study. Cell Biochemistry and Biophysics 78 (2):139–47. doi:10.1007/s12013-020-00906-5.
- Du, J., C. Yang, X. Ma, and L. Qifeng. 2021. Insights into the conformation changes of SARS-CoV-2 spike receptor-binding domain on graphene. Applied Surface Science 578:151934. doi:10.1016/j.apsusc.2021.151934.
- Hayyan, M., A. Abo-Hamad, M. AbdulHakim AlSaadi, and M. Ali Hashim. 2015. Functionalization of graphene using deep eutectic solvents. Nanoscale research letters 10 (1):324. doi:10.1186/s11671-015-1004-2.
- Ismail, E. H., D. Y. Sabry, H. Mahdy, and M. M. H. Khalil. 2014. Synthesis and characterization of some ternary metal complexes of curcumin with 1,10-phenanthroline and their anticancer applications. Journal of Scientific Research 6 (3):509–19. doi:10.3329/jsr.v6i3.18750.
- Jankute, M., V. Nataraj, O. Y. C. Lee, H. H. T. Wu, M. Ridell, N. J. Garton, M. R. Barer, D. E. Minnikin, A. Bhatt, and G. S. Besra. 2017. The role of hydrophobicity in tuberculosis evolution and pathogenicity. Scientific reports 7 (1):1–10. doi:10.1038/s41598-017-01501-0.
- Maurya, V. K., S. Kumar, A. K. Prasad, M. L. B. Bhatt, and S. K. Saxena. 2020. Structure-based drug designing for potential antiviral activity of selected natural products from ayurveda against SARS-CoV-2 spike glycoprotein and its cellular receptor. Virusdisease 31 (2):179–93. doi:10.1007/s13337-020-00598-8.
- Palmieri, V., F. Bugli, M. Cacaci, G. Perini, F. De Maio, G. Delogu, R. Torelli, C. Conti, M. Sanguinetti, M. De Spirito, et al. 2018. Graphene oxide coatings prevent Candida albicans biofilm formation with a controlled release of curcumin-loaded nanocomposites. Nanomedicine 13 (22):2867–79. doi:10.2217/nnm-2018-0183.
- Palmieri, V., F. De Maio, M. De Spirito, and M. Papi. 2021. Face masks and nanotechnology: keep the blue side up. Nano Today 37:101077. doi:10.1016/j.nantod.2021.101077.
- Palmieri, V., and M. Papi. 2020. Can graphene take part in the fight against COVID-19? Nano Today 33:100883. doi:10.1016/j.nantod.2020.100883.
- Rattis, B. A. C., S. G. Ramos, and M. Celes. 2021. “Curcumin as a potential treatment for COVID-19.” Frontiers in Pharmacology 1068.
- Talic, S., S. Shah, H. Wild, D. Gasevic, A. Maharaj, Z. Ademi, L. Xue, X. Wei, I. Mesa-Eguiagaray, and J. Rostron. 2021. Effectiveness of public health measures in reducing the incidence of Covid-19, SARS-CoV-2 transmission, and Covid-19 mortality: systematic review and meta-analysis. BMJ 375:e068302. doi:10.1136/bmj-2021-068302.
- Wen, C.C., Y.H. Kuo, J.T. Jan, P.H. Liang, S.Y. Wang, H.G. Liu, C.K. Lee, S.T. Chang, C.J. Kuo, and S.S. Lee. 2007. Specific plant terpenoids and lignoids possess potent antiviral activities against severe acute respiratory syndrome coronavirus. Journal of Medicinal Chemistry 50 (17):4087–95. doi:10.1021/jm070295s.
- WHO. 2021. “Global TB Report.” 25.
- Ye, S., K. Shao, Z. Li, N. Guo, Y. Zuo, Q. Li, Z. Lu, L. Chen, Q. He, and H. Han. 2015. Antiviral activity of graphene oxide: how sharp edged structure and charge matter. ACS Applied Materials & Interfaces 7 (38):21571–79. doi:10.1021/acsami.5b06876.
- Zhang, S., H. Dong, H. Ruidong, N. Wang, Q. Zhao, L. Yang, Q. Zhenghai, L. Sun, S. Chen, and M. Jianwei. 2022. Hydro electroactive Cu/Zn coated cotton fiber nonwovens for antibacterial and antiviral applications. International Journal of Biological Macromolecules 207:100–09. doi:10.1016/j.ijbiomac.2022.02.155.
- Zhang, S., Q. Zhang, J. Chen, H. Dong, A. Cui, L. Sun, N. Wang, L. Jiwei, and Q. Zhenghai. 2022. Cost-effective chitosan thermal bonded nonwovens serving as an anti-viral inhibitor layer in face mask. Materials Letters 318:132203. doi:10.1016/j.matlet.2022.132203.
- Zhu, S., C.W. Lou, S. Zhang, N. Wang, L. Jiwei, Y. Feng, H. Ruidong, X. Changgang, and J.H. Lin. 2022. Clean surface additive manufacturing of aramid paper-based electrically heated devices for medical therapy application. Surfaces and Interfaces 29:101689. doi:10.1016/j.surfin.2021.101689.