ABSTRACT
The Sesamum indicum bast contains more hemicellulose, lignin, and flaky parenchymal cells than the commonly used ramie bast, which are rigid for the degumming process. We explored a facile and greener method by using glycolic acid microwave-assisted treatment combined with alkaline treatment to effectively and low-cost prepare the high-quality Sesamum indicum single fibers. The chemical and physical properties of fibers were analyzed by chemical composition analysis, SEM, FTIR, XRD, TG, and GPC analysis. The residual gum content was decreased from 34.4% to 4.2%, resulting in a cleaner micro-surface of the Sesamum indicum fibers with increased cellulose crystallinity (75.2%–82.5%) and thermal stability (357°C–365°C). The fiber fineness (2.5 dtex) and breaking tenacity (4.9 cN/dtex) of the fibers produced by the novel treatment method exhibited the same level compared with the traditional two-step alkaline fibers. Besides, both the consumption of water and the chemicals was decreased compared with traditional degumming. This work provided a novel green and facile degumming method for processing the natural bast fibers.
摘要
胡麻韧皮部比常用的苎麻韧皮部含有更多的半纤维素、木质素和片状薄壁细胞,这些物质在脱胶过程中难以去除. 我们探索了一种简单、绿色的方法,将乙醇酸微波辅助处理与碱处理相结合,有效且低成本地制备优质胡麻单纤维. 通过化学成分分析、SEM、FTIR、XRD、TG和GPC分析分析了纤维的化学和物理性质. 残余胶含量从34.4%降至4.2%,胡麻纤维的微观表面更清洁,纤维素结晶度(75.2%-82.5%)和热稳定性(357°C-365°C)提高. 与传统的两步碱煮得到的纤维相比,新处理方法制备的纤维的纤维细度(2.5dtex)和断裂强度(4.9cN/dtex)表现出相同的水平. 此外,与传统脱胶相比,水和化学物质的消耗都有所减少. 这项工作为天然韧皮纤维的加工提供了一种新的绿色、简便的脱胶方fa.
Introduction
With the shortage of fossil energy, petroleum resource, and environmental crisis, natural cellulosic fibers have received attention due to their large quantity, renewable and degradable properties (Baral et al. Citation2019; Cao et al. Citation2019). The utilization of natural cellulosic fibers in the textile industry has attracted growing interest in recent years (Song et al. Citation2018). As new resources of natural cellulosic fiber, ramie, Apocynum ventum (Li et al. Citation2020), kenaf (Lv et al. Citation2021), Sesamum indicum (Zykova, Pantyukhov, and Popov Citation2017), and hemp fibers (Li et al. Citation2019) are being developed and utilized. Among them, Sesamum indicum fibers have attracted industrial and researchers’ interest due to their excellent fiber properties, such as good breathability, moisture absorption, etc (Amoo et al. Citation2017). Before high-value textile fiber utilization, Sesamum indicum bast needs to be degummed to remove non-cellulosic components including hemicellulose and lignin (Wang et al. Citation2021). However, the Sesamum indicum bast contains more hemicellulose, lignin, and flaky parenchymal cells compared to the ramie bast, which would make the degumming process more difficult. Therefore, it is necessary to develop a facile and greener degumming process for Sesamum indicum fiber preparation (Lv et al. Citation2021; Nie et al. Citation2020).
To remove gummy matters of bast fibers, the two-step alkali boiling process is most commonly used. This process requires two boiling procedures with large amounts of sodium hydroxide, which leads to high costs and serious environmental pollution. To avoid these problems, some novel degumming approaches have been extensively investigated and applied in the last few decades, such as biological degumming, steam explosion, microwave radiation, and organosolv pretreatment (Huang et al. Citation2021; Xu et al. Citation2020; Yu et al. Citation2020; Zhao et al. Citation2020). However, these methods still have limitations on the preparation of fibers due to the complexity of biomass structure, low heating rate, and temperature unevenness (Wang et al. Citation2020). To solve these problems, the combined use of physical and chemical treatments has been studied recently.
Microwave radiation is a very promising heating method for the degumming process due to its high heating rate and even heating (Zhu et al. Citation2021). In addition, microwave irradiation can break the hydrogen bonds between cellulose molecules, changing the supramolecular structure of biomass raw materials. The changes of the supramolecular structure make cellulose lose its swelling property, which further improves its reactivity and accessibility (Peciulyte et al. Citation2015). Organic solvent treatment is a widely studied method for biomass fractionation and utilization (Nie et al. Citation2020; Qiao et al. Citation2021). Many researchers are utilizing organic solvents to prepare lignocellulose fibers or extract lignin. The organic solvent shows the high separation efficiency of each component in the process (Cheng, Zhao, and Hu Citation2018; Thoresen et al. Citation2020). A combination of lactic acid treatment and microwave radiation was applied to separate kenaf bast into single fibers (Lv et al. Citation2021). The co-activity resulted in low lignin content (1.2%) and high yield (55.1%) of high-quality kenaf single fibers at 130°C for 30 min. However, bast fiber is readily hydrolyzed by lactic acid leading to poor physical properties (Bhambure, Rao, and Senthilkumar Citation2021). Therefore, it is necessary to select the appropriate organic solvents to effectively remove non-cellulose substances while avoiding serious damage to cellulose. Glycolic acid has been used to remove and extract eucalyptus hemicellulose, showing high selectivity for hemicellulose separation. The integrity of the cellulose structure was preserved (Luo et al. Citation2021). The depolymerization and re-polymerization of lignin were inhibited, and the separation of hemicellulose was as high as 65.48% under the optimal conditions (acid concentration of 5.4%, 140°C for 3.0 h) (Luo et al. Citation2021).
Therefore, a combination of microwave and glycolic acid treatment was selected for the efficient separation of Sesamum indicum fibers for this study. The fiber chemical composition, Fourier transform infrared (FTIR) spectra, x-ray diffraction (XRD) spectra, gel permeation chromatography (GPC) curves, thermogravimetric (TG) analysis, and scanning electron microscopy (SEM) morphology were characterized to evaluate the degumming ability of the pretreatment by this method. Furthermore, the basic chemical and physical properties of the refined dry fibers produced by the alkali degumming and the conventional alkali treatment were compared. Finally, the chemical reagent consumption and time cost caused by the two treatment methods are compared.
Materials and methods
Materials
Raw Sesamum indicum was cultivated from Hengfeng Co., Ltd, Dezhou China. The Sesamum indicum bast and stem were closely connected and difficult to separate. Therefore, we put the Sesamum indicum in 1% sulfuric acid solution for soaking at 50°C for 1 h and then peel off the bast after washing. The stripped bast was dried (the sample was named Raw) for subsequent experiments. All chemicals were purchased from Aladdin Industrial Corporation, and all of them were used as received without further purification.
Methods
Glycolic acid microwave-assisted pretreatment
Through the exploration of pre-experiments, we determined the concentration of glycolic acid solution and the duration of microwave treatment. Longer processing times and higher temperatures lead to the strength loss of fibers. The Sesamum indicum bast (1 g) was soaked in the 20-mL glycolic acid (10% w/w) solvent in the Teflon tubes. Then, the mixture was treated at Microwave Dissolver (CEM Mars 6 Classic, USA) at a power of 600 W at 100°C for 30 min. The glycolic acid treatment Sesamum indicum fiber assisted with microwave irradiation (the sample was named Gam) was collected for further analysis.
Glycolic acid microwave-assisted pretreatment synergistic alkali treatment
To obtain more in line with the needs of the textile industry, the Gam fiber prepared by most optimal conditions was processed further using dilute alkali treatment. The glycolic acid microwave pretreatment fiber (Gam) was further treated 2 h by 1% NaOH solvent at 100°C for obtaining the fiber (samples was named Gaa).
Two-step alkali boiling treatment
For comparison, the Sesamum indicum bast was degummed by a two-step alkali boiling method according to the described method of the traditional chemical degumming process (Song et al. Citation2018). The 5 g Sesamum indicum bast was boiled in 100 mL 1% (wt/wt) sodium hydroxide solution for 1 h first. In the second stage, the washed Sesamum indicum fibers were subjected to an alkali boiling solution for 3 h and then washed with hot deionized water to neutrality. The two-step alkali boiling Sesamum indicum fibers (Ta) were collected and dried. The schematic diagram of the experiment is shown in .
Characterization of Sesamum indicum fibers
The chemical composition analysis of the different fibers sample was determined based on the NREL standards. The carbohydrate polymer (cellulose, hemicellulose) content in the solid residue was measured by high-performance liquid chromatography (HPLC) (Agilent 1260-II) (Bhagia et al. Citation2016). The FTIR spectra of the different sample fibers were acquired with a Fourier-transform infrared-spectrometer NICOLET 5700 (Thermo Scientific, Germany) for observing the chemical structure and functional group changes. The fiber surface morphology was observed with scanning electron microscopy (SEM, JSM-6390LV, Japan) for analyzing the degumming effect. The longitudinal features of the raw and fibers isolated from the Sesamum indicum bast were monitored. XRD spectra were measured with a D8 Advanced X-ray diffractometer (Bruker, Germany). The crystalline index (CrI) of fiber was calculated according to the Segal method for native cellulose (Nie et al. Citation2020). The molecular weights of cellulose were measured for comparing the damaging effect on the fiber manufactured from the different degummed methods (Song et al. Citation2021). Thermogravimetric analysis of the fiber samples was conducted on Pyris TGA, (PerkinElmer, USA). The fiber fineness, breaking tenacity and fiber length were tested to compare the fiber’s physical properties. Before testing, samples were conditioned in a constant temperature and humidity environment (23 ± 2°C and 50 ± 5% RH) for 2 days. Detailed experimental parameters can be found in the supplementary information.
Result and discussion
Chemical composition analysis and FTIR analysis
Glycolic acid has the potential to separate the noncellulosic part from the biomass. The changes in the main compositions of different Sesamum indicum fibers prepared from various pretreatment methods were summarized in . There is a clear trend of increasing cellulose and decreasing lignin and hemicellulose with the pretreatment process (). As shown in , the content of cellulose increased from 44.7% to 77.1%. Glycolic acid microwave-assisted treatment could effectively remove the noncellulosic (gum) part at a gentle condition (100°C, 30 min). However, an amount of gummy matter was still present on the surface of Sesamum indicum fibers. To obtain the high-quality fibers, the Gam fibers were further treated with a diluted alkaline solution (Gaa). The cellulose content was further promoted from 77.1% to 86.4%, which reach the same level with two-step alkaline treatment fibers (Ta 90.9%). Then, the residual gum was tested to illustrate the quality level of the prepared fibers. The residual gum of Gaa and Ta was reach the same level of 4.2% and 4.7%, respectively. This result was consistent with previous research that the hemicellulose composition was readily removed by glycolic acid (Luo et al. Citation2021). This result confirmed the effectiveness of glycolic acid microwave-assisted pretreatment in the separation of hemicellulose from the Sesamum indicum bast. By combining glycolic acid and alkaline treatment, it is possible to facile prepare Sesamum indicum fibers of comparable quality to the traditional method.
Figure 2. (a) Primary chemical composition contents and (b) the FTIR spectroscopy of different Sesamum indicum fibers.
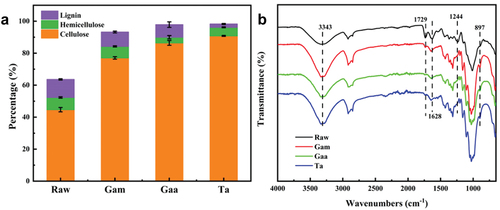
Table 1. Chemical compositions of different Sesamum indicum samples.
The FTIR spectra were measured to investigate the changes in the main chemical compositions (cellulose, hemicellulose, lignin) of different fiber samples (). The band at 3343 cm−1 was attributed to polysaccharides/lignin hydroxyl groups (−OH) and the band at 897 cm−1 confirmed the presence of cellulose β-1,4 glycosidic linkages (Li et al. Citation2016; Ong et al. Citation2020). As shown in , there was no significant change in the absorbance bands at 3343 and 897 cm−1 before and after different treatments. The feature absorption peaks (1729 cm−1) related to hemicellulose were weakened or vanished after degumming treatment, verifying hemicellulose removal during treatment (Kacurakova et al. Citation2000). By comparing the infrared spectra, it was suggestive that glycolic acid pretreatment has excellent hemicellulose potential, especially when combined with subsequent alkaline treatment.
Morphology and SEM images analysis
The longitudinal surface morphologies of Sesamum indicum fibers treated by different methods were illustrated in . The raw Sesamum indicum bast () exhibited a rough and irregular surface with a large number of gummy matter, which wrapped the fibers together (Song et al. Citation2020). The Gam fibers () treated with microwave-assisted glycolic acid adhered to a small amount of gum. The fibers were dispersed with the partial removal of hemicellulose and other non-cellulose substances. The Gaa fibers showed a smooth surface and better dispersion with the degradation of a large gum matter, similar to the effect of traditional two-step alkali boiling degumming (). These results confirmed that the new degumming method combined with glycolic acid pretreatment and alkali boiling has excellent degumming performance.
Crystalline analysis
The X-ray diffraction patterns of raw sesamum indium bast and fibers treated with different methods are presented in . The spectra with the typical (1–10), (110), (200), as well as (004) lattice of cellulose I were observed, revealing that different treatment processes didn’t change the cellulose type and fibers’ crystal structure (Amiralian et al. Citation2015). Based on the calculation method of Segal, the crystallinity index of fiber samples was summarized in . With the removal of amorphous substances, the crystallinity index of the different fiber samples gradually increased from (raw Sesamum indicum bast 75.2%) to (Gam 80.2%) and (Gaa 82.5%), respectively. The increase in crystallinity was mainly due to the dissolution of gum and some non-cellulosic substances in the amorphous region so that the cellulose content was increased relatively (Feng et al. Citation2015). It was proved that the novel degumming process has high degumming efficiency. These results were similar to the alkaline degumming treatment reported previously (Nie et al. Citation2019).
Table 2. Crystallinity index of different Sesamum indicum samples.
GPC analysis
The molecular weight distribution of Sesamum indicum fibers was analyzed with GPC to investigate the damaging effect of different treatment procedures. The GPC spectra of Gaa and Ta cellulose was shown in . The weighted average molecular weight (Mw), number average molecular weight (Mn), Z average molecular weight (Mz), the molecular weight of the highest peak (Mp), and polydispersity (PD Mw/Mn) were summarized in . Compared with the spectra of Gaa, it can be seen that the signal of the Ta cellulose sample was quite wide from 104-107 g/mol consistent with the polydispersity (PD) result. As shown in the spectra, there was a significant PD difference between the two cellulose samples 2.304 and 6.357, respectively. The result might be due to the degradation or hydrolyzing in the alkaline boiling. The weighted average molecular weight of Gaa (2361 kg/mol) and Ta (2328 kg/mol) remains at the same level. The molecular weight of the highest peak of two Sesamum indicum cellulose was 2398 kg/mol, and 2783 kg/mol, respectively. These results also indicate that there is little further damage to fibers prepared by glycolic acid microwave-assisted pretreatment synergistic dilute alkali treatment (Huang et al. Citation2021).
Figure 5. The molecular weight distributions (Mw) of the (Gaa) Glycolic acid microwave-assisted pretreatment synergistic alkali treatment fibers and (Ta) Two-steps alkaline treatment fibers.
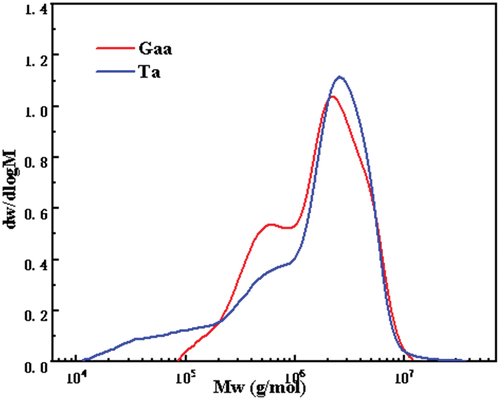
Table 3. The molecular weight and degree of polymerization of different Sesamum indicum samples.
TG analysis
The TG analysis was carried out to determine the thermal degradation characteristics of fibers. The thermogravimetry (TG) and differential thermogravimetry (DTG) curves of different Sesamum indicum fibers were summarized in . As shown in , there is a clear downward trend between 80-100°C and 300-380°C, which were attributed to the evaporation of water and the cellulose degradation, respectively (Shen et al. Citation2011). The thermogravimetry curve of raw Sesamum indicum showed a sustained decline between 200 and 480°C, which is mainly due to the presence of a large amount of noncellulosic fraction (Toledano et al. Citation2010). Compared with the raw materials (357°C), the degradation temperatures of the Gam, Gaa, and Ta Sesamum indicum fibers (365°C) were increased. At the same time, the solid residue of raw materials (23.5%) is significantly higher than that of Gam, Gaa, and Ta Sesamum indicum fibers (~15%). These results suggested that the gummy matter was extensively removed by the novel methods. The glycolic acid microwave-assisted treatment could achieve the purpose of fiber preparation.
Physical properties and the consumption of time and chemical reagents for preparation Sesamum indicum fibers analysis
The average length, fineness, breaking strength, and breaking elongation of the two treated Sesamum indicum fibers were compared in . The fiber length extracted by the two treatment methods is similar (23.3 mm and 23.4 mm, respectively). The fineness of fibers obtained from the new method was slightly higher than that from the traditional method (2.5 dtex and 2.3 dtex, respectively). Both kinds of fibers met the first-class requirement of the Chinese national standards. The breaking strength of refined fiber obtained by microwave-assisted degumming with glycolic acid is lower than that of traditional chemical degumming. The reason is that the fiber prepared by the microwave-assisted method of glycolic acid contains less gum and the number of single fibers in the process fiber is reduced, so the diameter of the process fiber becomes smaller and its degree of polymerization is low (). In addition, the dispersion of fiber strength is mainly due to the defects of random distribution in fiber (Xia et al. Citation2009). In microwave-assisted organic acid pretreatment, glycolic acid had an obvious effect on hemicellulose removal, which increased the contact between cellulose and glycolic acid, causing slight damage to cellulose structure. Therefore, the breaking strength of Gaa fiber is slightly lower than that of Ta fiber. However, the breaking tenacity still meets the requirements of the spinning standard.
Table 4. Physical properties of Sesamum indicum fibers.
The chemical reagents and treatment time usage for 1 kg raw bast in the process of Sesamum indicum fibers preparation are summarized in . The usage of chemical reagents was significantly reduced during the glycolic acid microwave-assisted treatment. For the degumming of a 1 kg raw bast, the new method only requires 1.2 kg of reagent, while the traditional method requires 3 kg, which exhibited huge advantages in terms of industrial costs. Furthermore, the degumming time was shortened from 4 h for the traditional method to 2.5 h for the new one. Therefore, the glycolic acid microwave-assisted treatment is more environmentally friendly and efficient.
Table 5. Chemical reagents usage and treatment time for the treatment of 1 kg raw Sesamum indicum bast.
Conclusion
The glycolic acid and microwave irradiation were conducted to effectively and low-cost prepare the biomass single fibers. The Sesamum indicum fiber was prepared by a facile and greener method using glycolic acid microwave-assisted treatment combined with alkaline treatment. The characteristics of fibers were analyzed by SEM, chemical composition analysis, FTIR, XRD, TGA, and GPC. The components such as the gummy part (34.4%–4.2%) and hemicellulose, lignin were largely removed, resulting in a cleaner micro-surface of the Sesamum indicum fibers, and increased cellulose crystallinity (75.2%–82.5%) and thermal stability (357°C–365°C). Chemical and physical properties were comparable with the fibers produced by the two-step traditional alkaline degumming method. Finally, both the consumption of water and the chemicals was decreased compared with traditional chemical degumming. Thus, this work provided a novel green degumming method for processing the plant bast fibers.
Supplemental Material
Download Zip (35.1 KB)Acknowledgement
This work was supported by the National Natural Science Foundation of China (51903131), Natural Science Foundation of Shandong Province (ZR2019QEM007, ZR2020ME076, and ZR2020QE239), Key Research and Development Program of Shandong Province (2020CXGC011101), State Key Laboratory of Bio-Fibers and Eco-Textiles (Qingdao University) (ZKT16 and ZKT21) and Special Foundation of “Taishan Scholar” Construction Program (ts20190932).
Disclosure statement
No potential conflict of interest was reported by the author(s).
Supplemental material
Supplemental data for this article can be accessed online at https://doi.org/10.1080/15440478.2022.2156965.
Additional information
Funding
References
- Amiralian, N., P. K. Annamalai, P. Memmott, and D. J. Martin. 2015. Isolation of cellulose nanofibrils from Triodia pungens via different mechanical methods. Cellulose 22 (4):2483–11. doi:10.1007/s10570-015-0688-x.
- Amoo, S. O., A. O. M. Okorogbona, C. P. Du Plooy, and S. L. Venter. 2017. Chapter 26 - Sesamum indicum. In Medicinal spices and vegetables from Africa, ed. V. Kuete, 549–79. Amsterdam, Netherlands: Academic Press.
- Baral, N. R., E. R. Sundstrom, L. Das, J. Gladden, A. Eudes, J. C. Mortimer, S. W. Singer, A. Mukhopadhyay, and C. D. Scown. 2019. Approaches for more efficient biological conversion of lignocellulosic feedstocks to biofuels and bioproducts. ACS Sustainable Chemistry & Engineering 7 (10):9062–79. doi:10.1021/acssuschemeng.9b01229.
- Bhagia, S., A. Nunez, C. E. Wyman, and R. Kumar. 2016. Robustness of two-step acid hydrolysis procedure for composition analysis of poplar. Bioresource Technology 216:1077–82. doi:10.1016/j.biortech.2016.04.138.
- Bhambure, S. S., A. S. Rao, and T. Senthilkumar. 2021. Characterization of control and chemically modified Kenaf fiber. Journal of Natural Fibers 19: 10320–30. Advance online publication. doi:10.1080/15440478.2021.1993500.
- Cao, Y., S. S. Chen, S. C. Zhang, Y. S. Ok, B. M. Matsagar, K.C. -W. Wu, and D. C. W. Tsang. 2019. Advances in lignin valorization towards bio-based chemicals and fuels: Lignin biorefinery. Bioresource Technology 291:121878. doi:10.1016/j.biortech.2019.121878.
- Cheng, F. C., X. Zhao, and Y. C. Hu. 2018. Lignocellulosic biomass delignification using aqueous alcohol solutions with the catalysis of acidic ionic liquids: A comparison study of solvents. Bioresource Technology 249:969–75. doi:10.1016/j.biortech.2017.10.089.
- Feng, X., X. H. Meng, J. P. Zhao, M. Miao, L. Y. Shi, S. P. Zhang, and J. H. Fang. 2015. Extraction and preparation of cellulose nanocrystals from dealginate kelp residue: Structures and morphological characterization. Cellulose 22 (3):1763–72. doi:10.1007/s10570-015-0617-z.
- Huang, H., Q. Tang, G. Y. Lin, C. W. Yu, H. Wang, and Z. L. Li. 2021. High-efficiency and recyclable ramie cellulose fiber degumming enabled by deep eutectic solvent. Industrial Crops and Products 171:113879. doi:10.1016/j.indcrop.2021.113879.
- Kacurakova, M., P. Capek, V. Sasinkova, N. Wellner, and A. Ebringerova. 2000. FT-IR study of plant cell wall model compounds: Pectic polysaccharides and hemicelluloses. Carbohydrate Polymers 43 (2):195–203. doi:10.1016/S0144-8617(00)00151-X.
- Li, Z. L., J. Chen, J. J. Zhou, L. Zheng, K. C. Pradel, X. Fan, H. Y. Guo, Z. Wen, M. -H. Yeh, C. W. Yu, et al. 2016. High-efficiency ramie fiber degumming and self-powered degumming wastewater treatment using triboelectric nanogenerator. Nano Energy 22:548–57. doi:10.1016/j.nanoen.2016.03.002.
- Li, Z. G., J. H. Liu, Z. P. Gao, and Z. P. Guan. 2019. Preparation of environmentally friendly hemp fiber reinforced polylactic acid composites. Journal of Jilin University: Engineering and Technology Edition 49 (2):536–43.
- Li, C. H., S. Y. Liu, Y. Song, K. Nie, H. X. Ben, Y. M. Zhang, G. T. Han, and W. Jiang. 2020. A facile and eco-friendly method to extract Apocynum venetum fibers using microwave-assisted ultrasonic degumming. Industrial Crops and Products 151:112443. doi:10.1016/j.indcrop.2020.112443.
- Luo, Y. D., Y. Li, L. M. Cao, J. T. Zhu, B. J. Deng, Y. J. Hou, C. Liang, C. X. Huang, C. R. Qin, and S. Q. Yao. 2021. High efficiency and clean separation of eucalyptus components by glycolic acid pretreatment. Bioresource Technology 341:125757. doi:10.1016/j.biortech.2021.125757.
- Lv, W. W., Z. G. Xia, Y. Song, P. X. Wang, S. Y. Liu, Y. M. Zhang, H. X. Ben, G. T. Han, and W. Jiang. 2021. Using microwave assisted organic acid treatment to separate cellulose fiber and lignin from kenaf bast. Industrial Crops and Products 171:113934. doi:10.1016/j.indcrop.2021.113934.
- Nie, K., B. X. Liu, T. Zhao, H. Y. Wang, Y. Song, H. X. Ben, A. J. Ragauskas, G. T. Han, and W. Jiang. 2020. A facile degumming method of kenaf fibers using deep eutectic solution. Journal of Natural Fibers 19 (3):1115–25. doi:10.1080/15440478.2020.1795778.
- Nie, K., Y. Song, S. Y. Liu, G. T. Han, H. X. Ben, A. J. Ragauskas, and W. Jiang. 2019. Preparation and characterization of microcellulose and nanocellulose fibers from Artemisia Vulgaris bast. Polymers 11 (5):907. doi:10.3390/polym11050907.
- Ong, H. C., W. H. Chen, Y. Singh, Y. Y. Gan, C. Y. Chen, and P. L. Show. 2020. A state-of-the-art review on thermochemical conversion of biomass for biofuel production: A TG-FTIR approach. Energy Conversion and Management 209:112634. doi:10.1016/j.enconman.2020.112634.
- Peciulyte, A., K. Karlstrom, P. T. Larsson, and L. Olsson. 2015. Impact of the supramolecular structure of cellulose on the efficiency of enzymatic hydrolysis. Biotechnology for Biofuels 8:56. doi:10.1186/s13068-015-0236-9.
- Qiao, X., S. Ni, H. Lu, X. Wang, and X. Zhou. 2021. A novel method to prepare chemical fibers by plasticizing cotton with 1-allyl-3-methylimidazolium chloride. International Journal of Biological Macromolecules 166:1508–12. doi:10.1016/j.ijbiomac.2020.11.030.
- Shen, D. K., R. Xiao, S. Gu, and K. H. Luo. 2011. The pyrolytic behavior of cellulose in lignocellulosic biomass: A review. RSC advances 1 (9):1641–60. doi:10.1039/c1ra00534k.
- Song, Y., W. Jiang, H. X. Ben, X. Z. Meng, Y. M. Zhang, G. T. Han, and A. J. Ragauskas. 2020. The production of hydrogen–deuterium exchanged cellulose fibers with exchange-resistant deuterium incorporation. Cellulose 27 (11):6163–74. doi:10.1007/s10570-020-03230-6.
- Song, Y., W. Jiang, H. X. Ben, Y. M. Zhang, G. T. Han, and A. J. Ragauskas. 2021. The preparation and characterization of chemically deuterium incorporated cotton fibers. Cellulose 28 (9):5351–61. doi:10.1007/s10570-021-03869-9.
- Song, Y., W. Jiang, Y. M. Zhang, H. X. Ben, G. T. Han, and A. J. Ragauskas. 2018. Isolation and characterization of cellulosic fibers from kenaf bast using steam explosion and Fenton oxidation treatment. Cellulose 25 (9):4979–92. doi:10.1007/s10570-018-1916-y.
- Thoresen, P. P., L. Matsakas, U. Rova, and P. Christakopoulos. 2020. Recent advances in organosolv fractionation: Towards biomass fractionation technology of the future. Bioresource Technology 306. doi:10.1016/j.biortech.2020.123189.
- Toledano, A., A. Garcia, I. Mondragon, and J. Labidi. 2010. Lignin separation and fractionation by ultrafiltration. Separation and Purification Technology 71 (1):38–43. doi:10.1016/j.seppur.2009.10.024.
- Wang, X. H., M. R. Wang, G. J. Liu, Y. M. Zhang, G. T. Han, A. Vomiero, and H. G. Zhao. 2021. Colloidal carbon quantum dots as light absorber for efficient and stable ecofriendly photoelectrochemical hydrogen generation. Nano Energy 86:106122. doi:10.1016/j.nanoen.2021.106122.
- Wang, X. H., Y. K. Zhu, X. Q. Wang, G. J. Liu, J. Z. Liu, R. H. Zhao, Y. M. Zhang, X. L. Zhang, G. T. Han, H. G. Zhao, et al. 2020. In-situ growth of graphene on carbon nanofiber from lignin. Carbon 169:446–54. doi:10.1016/j.carbon.2020.07.070.
- Xia, Z. P., J. Y. Yu, L. D. Cheng, L. F. Liu, and W. M. Wang. 2009. Study on the breaking strength of jute fibres using modified Weibull distribution. Composites Part A-Applied Science and Manufacturing 40 (1):54–59. doi:10.1016/j.compositesa.2008.10.001.
- Xu, J. Y., C. Y. Li, L. Dai, C. L. Xu, Y. D. Zhong, F. X. Yu, and C. L. Si. 2020. Biomass fractionation and lignin fractionation towards lignin valorization. ChemSuschem 13 (17):4284–95. doi:10.1002/cssc.202001491.
- Yu, H. L., Y. Q. Xu, J. J. Hou, Y. H. Ni, S. W. Liu, Y. Liu, S. T. Yu, S. X. Nie, Q. Wu, and C. J. Wu. 2020. Efficient fractionation of corn stover for biorefinery using a sustainable pathway. ACS Sustainable Chemistry & Engineering 8 (8):3454–64. doi:10.1021/acssuschemeng.9b07791.
- Zhao, D., H. R. Ji, R. P. Du, Q. Wang, W. X. Ping, and J. P. Ge. 2020. Optimization of process conditions for microwave-assisted flax water retting by response surface methodology and evaluation of its fiber properties. Bioresources 15 (3):5859–70. doi:10.15376/biores.15.3.5859-5870.
- Zhu, Z. Y., Y. B. Liu, L. D. Gomez, T. Wei, X. L. Yang, R. Simister, S. J. McQueen-Mason, and D. J. Macquarrie. 2021. Thermochemical pretreatments of maize stem for sugar recovery: Comparative evaluation of microwave and conventional heating. Industrial Crops and Products 160:113106. doi:10.1016/j.indcrop.2020.113106.
- Zykova, A., P. Pantyukhov, and A. Popov. 2017. Ethylene-octene copolymer-wood flour/oil flax straw biocomposites: Effect of filler type and content on mechanical properties. Polymer Engineering & Science 57 (7):756–63. doi:10.1002/pen.24626.