ABSTRACT
The paper presents the effect of using cashew nut shell liquid (CNSL), a low-priced agro by-product and a renewable resource, to treat coir geotextiles. Large-scale direct shear tests were performed on sands reinforced with untreated and treated coir geotextiles at different normal stresses (50 kPa, 100 kPa, and 150 kPa). Sands of three different particle sizes (coarse, medium, and fine) were used for the study. The shear strength parameters of reinforced soil samples were measured in terms of interface friction angle, apparent cohesion, and interface coefficient. The experimental investigations revealed that cashew nut shell liquid-treated coir geotextiles improved the interface shear strength parameters for all sand fractions. The tensile strength of CNSL-treated coir geotextile was also improved by 13%. Two-dimensional finite element simulations of large-scale direct shear tests using PLAXIS 2D were also carried out to study the mechanism in detail. Mohr’s circles were drawn using the data from numerical analyses, and the plane strain friction angles obtained were compared with the direct shear friction angles obtained from experimental studies. The plane strain friction angle was higher for soil reinforced with treated coir geotextile than soil reinforced with untreated coir geotextile, indicating the shifting of the failure envelope.
摘要
本文介绍了利用腰果壳液(CNSL)处理椰壳土工织物的效果. 在不同法向应力(50kPa、100kPa和150kPa)下,对用未处理和处理的椰壳土工布加固的砂进行了大规模直剪试验. 研究中使用了三种不同粒度(粗粒、中粒和细粒)的沙子. 根据界面摩擦角、表观黏聚力和界面系数测定了加筋土样的抗剪强度参数. 实验研究表明,腰果壳液体处理的椰壳土工织物改善了所有砂组分的界面剪切强度参数. CNSL处理的椰壳土工布的拉伸强度也提高了13%. 还使用PLAXIS 2D进行了大规模直剪试验的二维有限元模拟,以详细研究机理. 使用数值分析的数据绘制莫尔圆,并将获得的平面应变摩擦角与实验研究获得的直接剪切摩擦角进行比较. 用处理过的椰壳土工布加固的土壤的平面应变摩擦角比用未处理过的椰子壳土工织物加固的土壤高,表明破坏包络线发生了移动.
Introduction
Natural fibers are a vital replacement for synthetic fibers because of their low density, high specific properties, ecological benefits, low energy intake, and emissions during manufacturing and production. Compared to natural fibers like jute, sisal, and hemp, coir fibers have high strength retention capacity and resistance to the saline environment, making them suitable for use in coastal areas for reinforcement purposes. They have high resistance to adverse weather conditions due to the higher constitution of lignin, and the water absorption rate is lower because of their lower constitution of cellulose content. The microfibrils of coir fibers are arranged helically at 450, which enables them to elongate beyond the elastic limit without causing failure and less vulnerable to squeeze and pucker due to air-filled pockets (Satyanarayana, Kulkarni, and Rohatgi Citation1981). When used with foundation soils, reinforcement helps to increase the bearing capacity and reduce the settlements (Muhammad and Shukla Citation2020, Citation2021). It was also observed that the wrap around reinforced model resulted in less settlement than planar reinforced models for the same reinforcement material.
The challenge posed by natural fibers is the high moisture attraction leading to microbial growth and weakening of bonding between fibers. This is due to numerous fine pores on the surface of coir fibers and their irregular textures, leading to moisture absorption from the soil, which causes the fibers to expand. To counter this problem of coir fibers, biopolymer-based products, which have less impact on the environment, can be used for surface modification. Previous studies suggest cashew nut shell liquid (CNSL), an agricultural by-product of cashew nut produced by the cashew nut tree, as a protective coating on coir geotextiles. The paper presents experimental studies on CNSL-coated coir fibers using large-scale direct shear apparatus and numerical simulations using PLAXIS 2D.
Literature review
High lignin content in coir fiber accounts for their strength retention ability and rigidity among the various natural fibers (Rajan et al. Citation2005). Sheela, Sayida, and Girish (Citation2021) conducted Benkelman Beam Deflection, Dynamic Cone Penetration, and Field California Bearing Ratio tests on five coir geotextile-reinforced roads and compared the results with unreinforced one. It was observed that with the use of coir geotextiles, a characteristic reduction in deflection and a decrease in Dynamic Cone Penetration Index was noted. The field CBR values for the five cases considered improved with coir geotextiles and ranged from 22% to 178%. Joy, Balan, and Jayasree (Citation2011) did burial studies on coir geotextiles. Wide-width tensile strength was conducted for coir geotextiles in the machine and cross-machine direction at regular intervals (30, 60, 75, 105, and 120 days). They concluded that the biodegradation of coir geotextiles was more reflected up to 75 cm. The advantages of untreated coir geotextiles motivated researchers (Enriquez et al. Citation2010; Liu et al. Citation2009; Sinha and Panigrahi Citation2009; Sumi, Unnikrishnan, and Mathew Citation2016, Citation2018; Vivek, Dutta, and Kumari Citation2020) to conduct surface modification studies on coir geotextiles to enhance the interfacial bonding, strength, and resistance against microbial degradation. Surface modifications of coir geotextiles using bio-based materials are preferred due to the adverse after-effects of chemically treated coir geotextiles. Sumi, Unnikrishnan, and Mathew (Citation2016, Citation2018) conducted experimental work to study the biological resistance and long-term performance of untreated and CNSL-treated coir geotextiles. Coir geotextiles modified using CNSL reported 70–80% of tensile strength retention when buried in the soil and tested after 1 year. The interfacial shear strength of sand and geotextile materials largely depends on the interlocking mechanism developed during shearing. The interlocking mechanism established depends on the particle size and morphology of sands and the surface roughness of the geotextiles used (Latha and Murthy Citation2007; Palmeira Citation2009; Vangla and Latha Citation2015).
Furthermore, many numerical simulation studies based on finite element models of direct shear tests have been conducted in past years. Tejchman (Citation2004) performed finite element method-based numerical simulation on direct shear tests in plane strain conditions and reported that the simulated results were reasonably in good agreement with the experimental results. According to Hegde and Roy (Citation2018), while conducting experiments, the boundary effects, the disparity in the testing procedure, soil placement, and compaction patterns can significantly influence the laboratory results. To overcome the limitations mentioned above, finite element analysis is widely used to understand the soil – geosynthetic interaction effects in the behavior of soils reinforced with geosynthetics.
Literature showed a paucity of studies on the interface behavior of granular soils reinforced with CNSL-treated coir geotextiles. In this paper, experimental studies were conducted using large-scale direct shear apparatus to study the effect of varying the particle size on treated and untreated coir geotextile-granular soil interface shear response under different normal stress. Numerical simulations were carried out using the finite element program PLAXIS 2D to validate the experimental results. The models developed were used to study the influence of reinforcement on the stress-strain characteristics of coarse, medium, and fine soils.
Material used
Three different grains of sand, namely coarse sand (4.75 mm−2 mm), medium sand (2 mm−0.425 mm), and fine sand (0.425 mm−0.075 mm), were used in the study (). Locally available granular soils were sieved into specific size fractions. The sands are classified as poorly graded sands (SP) as per the Unified Soil Classification System. The mean particle size of sand varies from 2.9 to 0.31 mm. The properties of soil are listed in . Experimental studies were carried out on woven-type coir geotextiles () procured from Easwary Coir Mills Private Ltd., India. Coir geotextile has an opening size of 10 mm x 10 mm and a tensile strength of 13.1 kN/m along the machine direction. Detailed properties of coir geotextile are given in . Cashew nut shell liquid (CNSL) was used to perform surface modifications on coir geotextiles. shows the CNSL used in the experiments procured from Kumarasamy Industries, India. It has a viscosity of 0.13 Pa-s, and specific gravity of 0.96 at 30°C. CNSL is a dark viscous brown liquid extracted from the cashew nut shell having cardol and cardanol as the main constituents. Previous studies have shown that CNSL has a high solubility in all solvents, making it ideal as a surface coating material in cellulosic and non-cellulosic materials.
Figure 1. Materials used in the study (a) fine sand (b) medium sand and (c) coarse sand (d) untreated coir geotextile (e) CNSL.
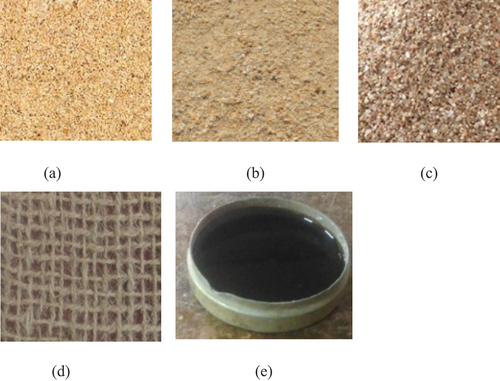
Table 1. Material properties.
Surface modifications
Coir geotextiles were washed using alkaline water to obtain a proper binding with the CNSL coating. Then, the coir geotextiles were pre-treated with 1% copper sulfate solution and dried at 70°C (Sumi, Unnikrishnan, and Mathew Citation2016). CNSL was mixed in kerosene, a solvent, and nitric acid (1% weight of CNSL). The above mixture was applied over the pre-treated coir geotextiles. Based on the literature, a CNSL concentration of 40% was used in the experiments to study the influence of surface modifications on interface behavior.
Experimental procedure
The tests were performed using a large-scale direct shear setup consisting of a shear box of size 300 mm x 300 mm x 150 mm. shows the test setup used in the present study. According to ASTM D5321 (2008), the size of the shear box must be a rectangle or square with a minimum dimension greater than 300 mm or 15 times D85 of the soil used in the experiment or a minimum of 5 times the opening size of the reinforcing material used. The depth of the shear box should be at least 50 mm or six times the maximum particle size of soil used in the study, whichever is greater. The setup used in the study satisfies the above criteria. The direct shear box was filled with sand in four equal layers. All tests were conducted at a relative density of 60%. The required weight of sand for each layer was calculated and filled into the shear box. The poured sand was manually compacted to a predetermined layer thickness using a 5 × 5 cm wooden tamping rod. To obtain uniform compaction, the number of blows to compact the sand was increased from the bottom to the top layer. The coir geotextile was placed above the lower shear box during the direct shear test on the reinforced specimen. One end of the coir geotextile is clamped on a steel block placed on the front edge of the lower shear box.
After sample filling, the loading block was kept on top of the granular soil sample. The loading block was tightly fixed to the shear box to ensure symmetric loading conditions. The shear boxes were correctly fixed using loading pins during sample filling. The loading pin was removed before testing to allow the shear movement of boxes. The tests were carried out at three normal stresses −50 kPa, 100 kPa, and 150 kPa (). All tests were performed at a 1 mm/min shear displacement rate. The shear resistance of the sample was measured using a proving ring connected to the upper shear box. Shear displacement was measured using a dial gauge attached to the front of the shear box.
Table 2. Testing program.
Finite element modeling
The finite element program PLAXIS 2D was used to perform numerical simulations in the present work. The developed two-dimensional model is shown in . The dimensions of the direct shear test were the same as the experimental setup.
The entire simulation was performed based on plane strain conditions to simulate the mechanical behavior of the granular soil tested in the large-scale direct shear box (El-Emam et al. Citation2013). The behavior of the sand was simulated using a linear elastic-perfectly plastic model with the Mohr–Coulomb failure criterion. The behavior of the coir geotextile was using the isotropic linear elastic model. The parameters used in the analyses are summarized in .
Table 3. Properties of soil used in the numerical simulation.
The sand was discretized using 15-noded triangular elements and geogrid structural elements with zero compressive and bending strength to discretize the coir geotextile. The size of the mesh was decided based on several trial analyses with a different number of elements. Vertical and horizontal fixities were applied to the upper shear box. The movement of the lower shear box was restricted in the vertical direction on both sides. This was done to simulate the proper shearing action under the prescribed displacements. Coir geotextile was placed between the upper and lower boxes. The interface was modeled using interface elements, and the interface was assigned to the lower surface of the geotextile (Hegde and Roy Citation2018). The analyses were carried out with three normal stresses of 50 kPa, 100 kPa, and 150 kPa. Initially, the normal stress was applied at the top surface of the model, followed by the application of prescribed displacements for the shearing action to develop. Boundary effects and contact stresses from the walls of the shear box are neglected in the present work.
Validation of the model
The experimental results’ data were used to validate the model developed. A comparison of experimental and numerical results at 150 kPa for coarse sand in the unreinforced and reinforced case is shown in . The numerical results agree with the experimental work results, which validated the developed model.
Results and discussions
Interface shear stress-displacement response
Unreinforced granular soil
The interface shear stress-displacement response of coarse, medium, and fine sand at a normal stress of 50, 100, and 150 kPa is shown in . Results indicate that the interface shear increases with increased shear displacement, and the peak shear strength is constant for large shear displacements. The interface shear stress developed was higher for soil samples with large particle sizes. The soil samples’ average particle size (D50) is 0.21 mm, 1.2 mm, and 2.9 mm for fine, medium, and coarse sand. The peak interface shear stress was 81.3 kPa for fine sand, 93.7 kPa for medium sand, and 105.3 kPa for coarse sand at 150 kPa. The peak interface shear stress increases with the average particle size and is accredited to an increase in the internal friction angle, ϕ (Vangla and Latha Citation2015). The internal friction angles for coarse, medium and fine sand are 35°, 32° and 28°,’ respectively (). This supports the studies that the friction angle of materials increased with an increasing uniformity coefficient of samples and D50 (Vangla and Latha Citation2015).
Coir geotextile reinforced sandy soil
shows the reinforced soil sample’s interface shear stress – displacement response. The interface shear strength for all coir geotextile-reinforced soil samples was higher than that of unreinforced soil. At normal stress of 150 kPa, the peak interface shear strength obtained for coarse, medium, and fine sand are 108.4 kPa, 95.3 kPa, and 83.7 kPa, respectively. The shear strength of geotextile-reinforced soil follows the elastic-plastic model, and the Mohr–Coulomb criterion is used to draw the failure envelope. It is evident from that no cohesion was developed at the interface, and the interface shear strength is only due to interface friction. The interaction between the surface of coir geotextiles and individual soil particles, followed by interlocking, contributes to the interface shear strength. The variation of peak interface shear strength of reinforced soil samples relating to particle size is shown in . It shows that coarse sand shows maximum and fine sand shows minimum shear strength under all normal stresses. Studies by Sayida et al. (Citation2020) have shown that the chemical composition of soil plays a major role in the biodegradation of coir geotextile with time which in turn reduces the tensile strength of the coir geotextile. Hence, the durability studies on untreated and treated coir geotextiles are critical and need to be considered in future studies.
CNSL-treated coir geotextile-reinforced granular soil
shows the stress displacement response of sand samples reinforced with CNSL-treated coir geotextile. The peak interface shear stress is 113.2 kPa, 97.3 kPa, and 88.2 kPa for coarse, medium, and fine sand. It is evident from that both interface peak shear strength and interface friction angle have increased due to the surface modification of coir geotextile using CNSL. This increase can be attributed to the use of cashew nut shell liquid would have led to the formation of a coating over the coir geotextile fibers, which will mask the pores on the coir geotextile, increasing the contact area between soil particles and geotextile. Vivek, Dutta, and Raman (Citation2018) reported that the surface treatments on coir geotextile would reduce the voids on its surface.
Figure 5. Behavior of CNSL-treated coir geotextile reinforced granular soil (a) Stress displacement response at 150 kPa (b) Failure envelope (c) Variation of peak shear stress with average particle size.
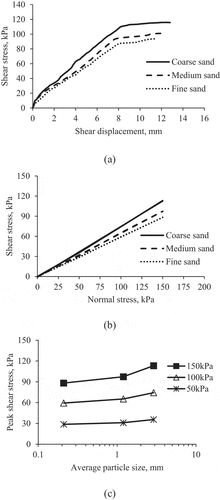
Among the soil samples, coarse sand exhibited high interface friction angle and peak shear strength. The roughness and angularity of coarse soil particles influenced the number of contact areas and groove depth. Coarse sand particles can make strong impressions on the surface, causing plowing and wearing. Groove depth depends on contact stress per individual particle and particle size. Groove depth increases with an increase in particle size. Therefore, coarse particles can cause more extended groove depths, increasing the interaction as shown in , in which data indicate an increase in interface shear strength with particle size. Medium sand particles also made impressions on the surface of geotextiles but with less groove depth. Vangla and Latha (Citation2015) reported that the effective contact between soil particles and the reinforcing layer could be inferred from the groove depth on the reinforcement material. The number of contacts per unit area is highest for fine sand particles. However, fine particles slide along the surface of geotextiles due to their smaller size and hardly make impressions on the surface of geotextiles. The interface characteristics of granular soils were represented using three parameters: interface friction angle (δ), apparent cohesion (ca), and interface coefficient (I.C), which is the ratio of shear strength of reinforced soil samples and unreinforced soil samples. gives the interface parameters for all the cases considered in the present study. The interface coefficient value was greater than one for all reinforced soil samples, indicating that incorporating CNSL-treated coir geotextile has improved soil shear strength.
Table 4. Interface parameters.
Ftir
Fourier transform infrared spectroscopy (FTIR) was taken for untreated and treated coir geotextiles to identify new functional groups after surface treatment. shows that there is broad peak at 3325 cm−1, 2951 cm−1, 1670 cm−1 and 1280 cm−1 for untreated coir geotextiles. These peaks were due to the O–H and C=O bonds in the lignin and hemicellulose. The peaks for treated coir geotextiles were at 1550 cm−1, 1300 cm−1, and 1100 cm−1 (). This shows that the earlier peaks have reduced, and new peaks have formed. The formation of new peaks indicates new functional groups in the form of C = N bonds. These functional groups could have masked the voids in the surface of coir geotextiles. The increase in tensile strength of coir geotextile can be attributed to this fact.
Comparison of plane strain friction angles and direct shear friction angles
The numerical analysis was performed at a normal stress of 150 kPa, which was applied by a uniformly distributed loading. The experimental and numerically obtained stress-displacement response of sand (coarse, medium, and fine) for the three conditions – unreinforced, reinforced with untreated coir geotextile, and reinforced with treated coir geotextile was analyzed. shows that shear failure in unreinforced granular soils happens at lower shear stress. In the case of reinforced soil models, the shear stress at failure is increased, as shown in . shows that the inclusion of treated coir geotextile has improved the shear stress at failure compared to the untreated coir geotextile reinforced model. At normal stress of 150 kPa, the value of failure stress for unreinforced coarse sand is 109.2 kPa. The value for coarse sand reinforced with untreated coir geotextile is 112.8 kPa, and the corresponding value for coarse sand reinforced with CNSL-treated coir geotextile is 121.5 kPa. The value of failure stress for medium sand is 97.8 kPa, 101.2 kPa, and 109.6 kPa for the three test cases. The respective values for fine sand are 85.6 kPa, 88.4 kPa, and 95.6 kPa. The failure stress value is higher for coarse sand than medium sand and fine sand, corresponding to reinforced untreated and treated coir geotextile. The percentage increase in failure stress for coarse sand, medium sand, and fine sand corresponding to unreinforced and reinforced treated coir geotextile models are 11.2%, 12.06%, and 11.68%, respectively. The percentage increase in failure stress for coarse, medium, and fine sand corresponding to unreinforced and reinforced untreated coir geotextile models are 3.29%, 3.4%, and 3.27%.
In most cases, the finite element model has over predicted the laboratory results. The difference in accuracy between numerical and laboratory tests can be attributed to the interaction modeled between the granular soil surface and the reinforcing layer. Although the numerical results are conservative, the finite element model in this study gives proper results based on reinforced and unreinforced specimens. In numerical simulations based on the finite element method, the worst scenario underestimates the possible impact of failures. The numerical analysis provides the total stresses acting on each element of the simulated model.
Based on the stress values obtained from numerical analyses, Mohr circles were drawn corresponding to three normal stresses for unreinforced soil and soil reinforced with untreated and treated coir geotextiles. From the Mohr circles shown in for unreinforced and reinforced (untreated and CNSL-treated) coarse, medium, and fine sand, plane strain friction angles were calculated and compared with direct shear friction angles obtained from the experimental results. A comparison was carried out between plane strain (numerical friction angle) and direct shear friction angle (experimental friction angle). The plane strain friction angle values were higher than direct shear friction angle values, and they differ by an average of 3°, depending upon the type of sand. Plane strain and direct shear friction angle values are higher for all granular soils reinforced with treated coir geotextiles. The direct shear test does not have the provision for measuring the direction of principal stresses at failure. Mohr circle of stress helps to predict the principal stress assuming a geometrical similarity exists between the Mohr circle of stress and the Mohr circle of strain. From the Mohr circle, it is clear that the failure envelope of granular soils reinforced with treated coir geotextile shifted above the failure envelope of granular soils reinforced with untreated coir geotextile.
Conclusions
In the present work, large-scale direct shear tests were performed on three different types of soil reinforced with CNSL treated and untreated coir geotextile. The following conclusions can be drawn from the study.
A reduction or increase in the interface shear strength is defined by the parameter Interface Coefficient (I.C). At 150 kPa, the Interface Coefficient values for coarse, medium, and fine sands reinforced with treated coir geotextile were 1.1, 1.078, and 1.152, respectively. This was found to be around 6.8%, 5.8%, and 12.0% higher than untreated coir geotextile.
The average percentage increase in failure stress for coarse, medium, and fine sand reinforced with treated coir geotextile was 10.4%, 11.6%, and 15.4%, respectively.
The tensile strength of CNSL-treated coir geotextile improved by 13%. The tensile strength possessed by coir geotextile resists the propagation of failure within the granular soil sample during shearing, which helps to improve the strength characteristics of soil samples.
The friction angle obtained from the laboratory large-scale direct shear test and plane strain friction angle obtained by drawing the Mohr circle using numerical analyses output was compared, and the variation was under 3°. This variation is because, during testing, shear stresses and displacements are nonuniformly distributed within the specimen.
The plane strain friction angle was higher for soils reinforced with treated coir geotextile model than soils reinforced with untreated coir geotextile, indicating the shifting of the failure envelope.
The plane strain friction angle values increased with an increase in soil particle size irrespective of reinforcement condition.
Highlights
Coir geotextiles suffer from the problem of waxy particles getting deposited on their surface, affecting the interaction with soil grains.
Cashew Nut Shell Liquid (CNSL), a cheap agro by-product, and a renewable resource was used on coir geotextiles to address these issues.
The experimental investigations revealed that CNSL treated coir geotextiles improved the interface shear strength parameters for all sand fractions.
Mohr’s circles were drawn using the output of numerical analyses to provide further insights on the response of CNSL treated coir geotextile reinforced soils.
Acknowledgments
The authors wish to acknowledge NIT Calicut for the financial support for this research work through the Faculty Research Seed Grant (FRG): FRG/2019/CED/16
Disclosure statement
No potential conflict of interest was reported by the author(s).
Additional information
Funding
References
- Enriquez, J. K. E. D. V., P. J. M. Santiago, T. F. Ong, and S. Chakraborty. 2010. Fabrication and characterization of high density polyethylene-coconut coir composites with stearic acid as a compatibilizer. Journal of Thermoplastic Composite Materials 23 (3):361–16. doi:10.1177/0892705709344560.
- Hegde, A., and R. Roy. 2018. A comparative numerical study on soil–geosynthetic interactions using large scale direct shear test and pullout test. International Journal of Geosynthetics and Ground Engineering 4 (1). doi:10.1007/s40891-017-0119-1.
- Joy, S., K. Balan, and P. K. Jayasree. 2011. Biodegradation of coir geotextile in tropical climatic conditions. In Proceedings of Indian Geotechnical Conference, Kochi, India, 604–06.
- Latha, G. M., and V. S. Murthy. 2007. Effect of reinforcement form on the behaviour of geosynthetic reinforced sand. Geotextiles and Geomembranes 25 (1):23–32. doi:10.1016/j.geotexmem.2006.09.002.
- Liu, C. N., J. G. Zornberg, T. C. Chen, Y. H. Ho, and B. H. Lin. 2009. Behavior of geogrid sand interface in direct shear mode. Journal of Geotechnical and Geoenvironmental Engineering. 135 (12), 1863–71.
- Magdi El-Emam, M. Attom, and Z. Khan. 2013. Numerical prediction of plane strain properties of sandy soil from the direct shear test. International Journal of Geotechnical Engineering 6:79–90. doi:10.3328/IJGE.2012.06.01.79-90.
- Muhammad, N. A. R., and S. K. Shukla. 2020. Ultimate bearing capacity of strip footing resting on soil bed strengthened by wraparound geosynthetic reinforcement technique. Geotextiles and Geomembranes 48 (6):867–74. doi:10.1016/j.geotexmem.2020.06.005.
- Muhammad, N. A. R., and S. K. Shukla. 2021. Experimental study on repeatedly loaded foundation soil strengthened by wraparound geosynthetic reinforcement technique. Journal of Rock Mechanics and Geotechnical Engineering 13 (4):899–911. doi:10.1016/j.jrmge.2021.02.001.
- Palmeira, E. M. 2009. Soil–geosynthetic interaction: Modelling and analysis. Geotextiles and Geomembranes 27 (5):368–90. doi:10.1016/j.geotexmem.2009.03.003.
- Rajan, A., R. C. Senan, C. Pavithran, and T. E. Abraham. 2005. Biosoftening of coir fiber using selected microorganisms. Bioprocess and Biosystems Engineering 28 (3):165–73. doi:10.1007/s00449-005-0023-2.
- Satyanarayana, K. G., A. G. Kulkarni, and P. K. Rohatgi. 1981. Structure and properties of coir fibres. Proceedings of the Indian Academy of Sciences Section C: Engineering Sciences 4:419–36. doi:10.1007/BF02896344.
- Sayida, M. K., Y. E. Sheela, A. Vijayan, and M. S. Girish. 2020. Durability study of coir geotextile embedded in different types of subgrade soil. Journal of Natural Fibers 19:6, 2288–2298. doi:10.1080/15440478.2020.1808146.
- Sheela, Y. E., M. K. Sayida, and M. S. Girish. 2021. Long-term performance of rural roads reinforced with coir geotextile – A field study. Journal of Natural Fibers 18 (10):1419–36. doi:10.1080/15440478.2019.1691117.
- Sinha, E., and S. Panigrahi. 2009. Effect of plasma treatment on structure, wettability of jute fiber and flexural strength of its composite. Journal of Composite Materials 43 (17):1791–802. doi:10.1177/0021998309338078.
- Sumi, S., N. Unnikrishnan, and L. Mathew. 2016. Experimental investigations on biological resistance of surface modified coir geotextiles. International Journal of Geosynthetics and Ground Engineering 2:31. doi:10.1007/s40891-016-0073-3.
- Sumi, S., N. Unnikrishnan, and L. Mathew. 2018. Durability studies of surface-modified coir geotextiles. Geotextiles and Geomembranes 46 (6):699–706. doi:10.1016/j.geotexmem.2018.07.007.
- Tejchman, J. 2004. Fe-simulations of a direct wall shear box test. Soils and Foundations 44 (4):67–81. doi:10.3208/sandf.44.4_67.
- Vangla, P., and G. M. Latha. 2015. Influence of particle size on the friction and interfacial shear strength of sands of similar morphology. International Journal of Geosynthetics and Ground Engineering 1 (1). doi:10.1007/s40891-014-0008-9.
- Vivek, R. K. Dutta, and A. Kumari. 2020. Effect of chemical treatment on the durability behavior of coir geotextiles. Journal of Natural Fibers 19:1–20. doi:10.1080/15440478.2020.1839622.
- Vivek, R. K. Dutta, and P. Raman. 2018. Effect of chemical treatment on the tensile strength behavior of coir geotextiles. Journal of Natural Fibers 1–15. doi:10.1080/15440478.2018.1503132.