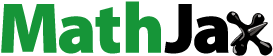
ABSTRACT
Bauhinia vahlii (BV) is a great source of cellulosic biomass, and it is gaining popularity as a renewable resource. BV bast fiber is proposed to have the potential to replace synthetic fibers in biopolymer composites as a reinforcing material. This investigation deals with the extraction, surface modification and comprehensive characterization of BV bast fibers which could be used for the production of sustainable fiber-reinforced polymer composites. The extracted fibers were chemically treated with sodium hydroxide, sodium chlorite, and benzoyl chloride. Then, the chemical properties, mechanical properties, surface morphology and thermal properties were investigated. An improvement in chemical and mechanical properties was observed after surface modification of fibers. Benzoylation treated BV bast fibers revealed highest tensile strength of 128.56 MPa and Young’s modulus of 8.34 GPa. In addition, after treatment, the fibers had rougher surface as seen from SEM images. The surface treatments removed a specific quantity of hemicelluloses, lignin, and pectin from the natural fiber surface, according to FTIR analysis. The surface treatments had a good impact on the crystallinity index of the natural fibers, according to XRD analysis. The characterization results confirmed that BV bast fibers could be used for the production of sustainable fiber reinforced polymer composites.
摘要
紫荆(Bauhinia vahlii,BV)是纤维素生物质的重要来源,作为一种可再生资源越来越受欢迎. BV韧皮纤维被认为有潜力取代生物聚合物复合材料中的合成纤维作为增强材料. 本研究涉及BV韧皮纤维的提取、表面改性和综合表征,可用于生产可持续纤维增强聚合物复合材料. 提取的纤维用氢氧化钠、亚氯酸钠和苯甲酰氯进行化学处理. 然后,对其化学性能、力学性能、表面形貌和热性能进行了研究. 在纤维表面改性后,观察到化学和机械性能的改善. 苯甲酰化处理的BV韧皮纤维显示出128.56MPa的最高拉伸强度和8.34GPa的杨氏模量. 此外,在处理后,如SEM图像所示,纤维具有更粗糙的表面. 根据FTIR分析,表面处理从天然纤维表面去除了特定数量的半纤维素、木质素和果胶. 根据XRD分析,表面处理对天然纤维的结晶指数有很好的影响. 表征结果证实BV韧皮纤维可用于生产可持续纤维增强聚合物复合材料.
Introduction
In the current global scenario, environmental consciousness has paved the road for researchers and academics to investigate and develop new materials. It has also encouraged the researchers to explore new natural fibers as an alternative to synthetic fibers. Synthetic fibers like Kevlar, nylon, glass fibers, etc. pose major environmental risks during disposal (Sanjay et al. Citation2018, Citation2019; Sarasini and Fiore Citation2018). It is a non-biodegradable material that contributes to landfills and has an impact on water and air quality (Thakur, Thakur, and Gupta Citation2014). So, the need of eco-friendly materials has forced the scientists to develop biocomposite materials for various sectors like defense, medical sector, aviation, construction and automobile sectors (Liu et al. Citation2019a, Citation2019b; Singh et al. Citation2019). Natural fiber reinforced composites are preferred over synthetic fiber composites because of various advantages like light weight, nontoxicity, low cost and biodegradability (Vaisanen, Das, and Tomppo Citation2017). Natural fibers have gained popularity in recent years as an environmentally friendly and cost-effective alternative to synthetic fibers in the reinforcing of composite products (Barari et al. Citation2016; Sepe et al. Citation2018). Natural fibers have a number of benefits and appealing characteristics, including abundance, light weight, biodegradability, environmental friendliness, low cost, and high specific stiffness (Indran et al. Citation2018; Liu and Tisserat Citation2018; Sanjay et al. Citation2019).
Bauhinia vahlii (BV) is a member of the Caesalpiniaceae family and is primarily found in India, Nepal, and Pakistan (Elbanna et al. Citation2016). It has anti-inflammatory qualities as well as a lot of fiber. The barks, stems, leaves, and seeds, as well as other parts of the BV, have a number of traditional uses. The seed is edible raw or fried, and it also has therapeutic properties. The seeds are aphrodisiac and tonic. The leaves are mucilaginous and demulcent. Matting, basketry, and wickerwork are all made with the stems.
It is well reported in literature that all natural fiber’s weakness is their poor bonding with matrix material. This is due to their hydrophilic nature which absorbs water molecules and that leads to degradation in strength and thermal stability of the material (Zhou, Fan, and Chen Citation2016). Surface modification is a fundamental step in the preparation of the reinforcing fibers used in biocomposite materials, and it is rapidly becoming an important topic of research due to their vast industrial potential. Chemical treatment aids in the removal of hemicellulose, lignin, wax, and oils that surround the fiber’s external surface, increasing surface roughness and improving fiber/matrix interaction. The presence of hydroxyl and polar groups in natural fibers enhances the moisture absorption tendency. Chemicals may activate hydroxyl groups or introduce new moieties that can effectively interlock with the matrix. Several studies have been conducted which deal with the chemical treatments of natural fibers in order to not only modify the interphase but also produce morphological changes on the fiber surfaces (Aziz and Ansell Citation2004; Cyras et al. Citation2001; Khalil et al. Citation2000; Nayak and Mohanty Citation2018; Rong et al. Citation2001; Stocchi et al. Citation2007). Amuthakkannan et al. reported that the chemical treatment of fibers reduces the water absorption ability and the surface properties are enhanced leading to better fiber-matrix compatibility (Amuthakkannan et al. Citation2013). In fact, alkali treatment, benzoylation, bleaching and peroxide treatment are widely used by various researchers (Kumar, Duraibabu, and Subramanian Citation2014; Liu et al. Citation2019a, Citation2019b; Venkata Krishna and Kanny Citation2016). The effects of NaOH solution treatment on the physical, chemical, and surface properties of Indian hemp fibers were studied by Sangappa et al. (Sangappa et al. Citation2014). They reported an increase in surface roughness after the chemical treatments were done. Ray et al. (Ray et al. Citation2001) and Mishra et al. (Mishra et al. Citation2001) used a 5% aqueous NaOH solution to treat jute and sisal fibers for 2 to 72 hours at ambient temperature. Morrison et al. (Morrison et al. Citation2000) sought to treat flax fiber with similar techniques. They reported two benefits of alkali treatments on the fiber: (a) achieved a better mechanical interlocking due to enhanced surface roughness of the fiber and (b) the amount of cellulose exposed on the surface of the fiber was increased. Treating the sisal fibers with benzoyl chloride improves the adhesion between fiber and matrix. It also reduces the water absorption properties to a large extent and enhances the thermal stability (Joseph et al. Citation2000). Murasing et al. (Murasing, Kumar, and Kumar Citation2021) investigated the effect of altitude on the mechanical strength of Grewia optiva fiber in Garhwal Himalaya in India. They found that the experimental parameters of fiber have shown that the properties were found suitable in higher altitudes. Research is being done to identify new lignocellulose fibers in order to improve the reinforcement in matrix composites and adapt them for various uses (Nayak et al. Citation2022; Premalatha et al. Citation2021; Vinod et al. Citation2021).
Although many studies have been dedicated to the chemical surface treatment of natural fibers, the effects of different surface treatments on the chemical properties, surface morphology, mechanical behaviors, and thermal stability of Bauhinia vahlii bast fibers have been rarely reported. Here, an attempt is made in this research to improve the chemical, mechanical, water absorption and morphological properties of BV fibers by conducting various chemical treatments. The effect of chemical treatments like alkali, benzoylation and bleaching on various properties of BV fibers was presented in this paper. The main aim is to determine an optimum chemical treatment method which gives the best results in improving the fiber’s properties which could be used as a potential reinforcement for fabrication of sustainable fiber-reinforced polymer composites. The treated and untreated fibers were characterized by FTIR and XRD to study the structural and chemical changes, if any, in the fibers. The surface morphology was studied by scanning electron microscopy (SEM). The mechanical properties of the treated fibers were studied and compared with untreated fibers. The information gathered can be useful to conclude the optimum chemical treatment process for BV fibers and can be used as reinforcement in polymeric matrices for various applications.
Materials and methods of experiment
Materials
Bauhinia vahlii stems were collected from local areas of Bhubaneswar, Odisha, India. Chemicals like sodium hydroxide, sodium chlorite and benzoyl chloride of analytical grades were purchased from Mohapatra Chemicals, Bhubaneswar, Odisha, India.
Methods
Extraction procedure of BV fibers
The stems that were collected were soaked in water for 2 days to make the outer bark soft for easy removal. The barks were then carefully removed with a scalpel. shows how the fibers were manually extracted from the bark. The extracted fibers were then thoroughly rinsed with distilled water to eliminate any contaminants (if any) before being dried in the sun for 2 days to remove moisture. The fibers collected were termed as untreated fibers.
Surface treatment of BV fibers
Bauhinia vahlii bast fibers were treated with sodium hydroxide (alkali treatment), benzoyl chloride (benzoylation) and sodium chlorite (bleaching).
For alkali treatment, the BV fibers were treated with 5% NaOH solution for 1 h at 80°C. Then, the fibers were exposed to atmosphere to bring these down to room temperature. In order to remove the traces of NaOH on the surfaces for maintaining pH level 7, these were shown to running water. Finally, the fibers were dried at of 60°C for 24 h with the help of oven (Dehury, Nayak, and Mohanty Citation2021). The important modification done by alkaline treatment is the disruption of hydrogen bonding in the network structure, thereby increasing surface roughness. Addition of aqueous sodium hydroxide (NaOH) to natural fiber promotes the ionization of the hydroxyl group to the alkoxide Eq 1 (Agrawal et al. Citation2000).
Benzoylation is an important transformation in organic synthesis (Paul, Puja, and Rajive Citation2003). In fiber treatment, benzoyl chloride is most commonly employed. Benzoyl chloride contains benzoyl (C6H5C=O), which is responsible for the treated fiber’s lower hydrophilicity and enhanced interaction with the hydrophobic polymer matrix. During benzoylation treatment alkali pre-treatment is used. The alkali-treated BV fibers were undergone a process of treatment with a solution of 10% NaOH in benzyl chloride for a time period of 15 min. Then, with the help of ethanol, the fibers were made free of benzyl chloride traces sticking to the surface. Then, the fibers were stored in an oven at 80°C for 24 h after being washed with distilled water (Mobarak et al. Citation2018).
Usually, sodium chlorite (NaClO2) is used for bleaching treatment. Here, a 5% NaOH solution was prepared to soak the BV fibers. Then, these were treated in the solution of NaClO2 in water (in a mass ratio of 1:25) for a period of 3 h at a temperature of 70°C. Finally, after all these treatments, the fibers were washed perfectly and dried for 5 h at 60°C.
From , a very smooth surface with presence of impurities (white spots in image) is observed in untreated fiber due to the presence of amorphous materials. It was also observed that the surface of BV fibers became free from all impurities and amorphous materials after the treatment (). The SEM image of benzyl chloride treated BV fibers is shown in . A large number of pinholes (crater like structure) and rough surface are clearly visible on the fiber surface. This is due to the removal of amorphous constituents surrounding the fiber surface. The rougher surface improves the fibers’ mechanical interlocking adherence to the matrix. Here, more OH groups were exposed. Again, in the benzoylation process, benzoyl groups replaced OH groups and improvement in fiber hydrophobic nature was noticed (Nayak and Mohanty Citation2018). Further chemical treatment of fibers enhances the exposure of cellulose with benzoylation treated BV fibers showing highest cellulose content ().
Figure 2. Scanning electron microscope images of Bauhinia vahlii fiber (a) untreated; (b) alkalization; (c) benzoylation; (d) bleaching.
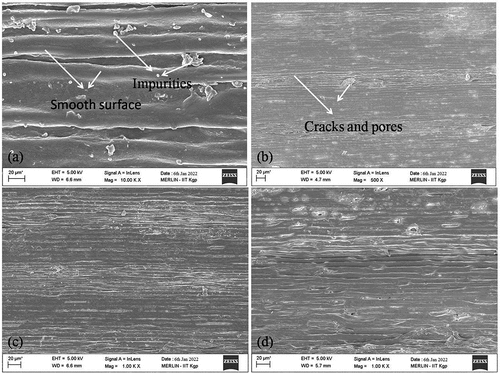
Table 1. Chemical composition of the Bauhinia vahlii fiber before and after treatment.
Single fiber tensile strength
Various mechanical properties like tensile strength, young’s modulus, elongation at break were measured for all the untreated and treated fibers as per ASTM D 3379–75. In this measurement, Instron 3369 UTM was used. For holding the specimen, a paper frame made of a thick cut piece of cardboard was prepared (). A single fiber was attached to both ends of the slot in the paper, which was prepared by cutting the center of the paper frame with length equal to gage length of the specimen. During the process of testing, 1 N load and 0.5 mm/min feed rate were maintained. With necessary precaution, cutting of both sides at mid position of frame was done.
Fourier transform infrared (FTIR) spectroscopy
The FTIR spectra of untreated and chemically treated BV fibers were recorded in a Nicolet 6700 spectrophotometer in the form of KBr Pellets. The test apparatus was equipped with Germanium Attenuated Total Reflectance (ATR). About 200 scans were collected for each measurement over the spectral range 4000–500 cm −1 with a resolution of 4 cm −1.
X-ray diffraction (XRD)
The effect of chemical treatment on crystallinity of BV fibers for both treated and untreated fibers was investigated using a Mini Flex diffractometer (shimadzu, XRD-7000). The graph of the samples was obtained at 40 kV and 150 mA in reflection mode, with a step of 0.05° and 1 s of checking time under CuKα radiation. The crystalline structure of cellulose-I of BV fiber was measured by crystallinity index (C.I.) from the empirical method proposed by Segal et al. (Segal et al. Citation1959) as per the following equation.
Where I002 is the maximum intensity of the (0 0 2) crystalline peak and Iam is the minimum intensity of the amorphous material between and (0 0 2) peaks.
Thermogravimetric analysis (TGA)
The thermal stability of BV fibers was characterized using a thermogravimetric analyzer (TA instrument, Q50 V20.13 Build 39). About 10 mg of BV fiber was exposed to atmospheric air with a heating rate of 10°C min −1 from room temperature to 600°C.
Results and discussion
Fourier transform infrared (FTIR) spectroscopy
shows the FTIR spectrum of untreated and various treated BV fibers. The peaks observed at wavenumber 3347 cm−1, 2920 cm−1, 1725 cm−1, 1510 cm−1, 1245 cm−1, 890 cm−1 correspond to the O – H, C – H, C=O, C=C, C – O, C1–H bonds, respectively. The characteristic peaks of cellulose, hemicellulose, pectin and lignin were visible in the spectrum of untreated fiber (), which was found to be removed in the treated fibers (Sepe et al. Citation2018). The peak 1725 cm−1 in the untreated fibers, which was assigned to C=O bonds stretching from the hemicelluloses and pectin, was no longer evident in the treated fibers. The C=C group stretching vibration in the carbonyl of hemicellulose caused a faint peak at around 1513 cm−1, which had a lower absorbance than the untreated fiber (Maache et al. Citation2017). The partial removal of lignin from the natural fiber surface resulted in a weak peak and decreased absorbance in treated fibers. In treated BV fibers, the peak at around 1243 cm−1 (C – O bond stretching in untreated BV fiber) was no longer visible. This suggested that lignin and hemicellulose in the treated fibers had been eliminated in some way (Shanmugasundaram, Rajendran, and Ramkumar Citation2018).
X-ray diffraction (XRD)
The XRD pattern of untreated and various chemically treated BV fibers are shown in . From the figure, two prominent peaks at 2Ɵ value of 16.7°, 22.8° and lower peak at 34.7° 2Ɵ were observed. Those peaks correspond to lattice plane (110), (002) and (004) for cellulose I crystallites (Dong et al. Citation2014; French Citation2014). It was also observed that the peak at (110) plane is more prominent after chemical treatment due to elimination of amorphous materials from the surface of the fibers. Similarly, peak 2 for all the samples lie at 22.8° irrespective of type of chemical treatment used. However, after chemical treatment, the same peak becomes sharper indicating an improvement in crystallinity of the material. shows the CI% of all the samples. From the table, it was observed that the CI% of treated fibers was improved after surface modification with benzoylation treated fibers showing highest crystallinity index (70.10%). Overall, the CI% follows the order of benzoylation > alkali > bleaching > untreated. Chemical treatments removed a portion of non-crystalline materials in natural fibers, such as hemicelluloses or lignin (“FTIR analysis” section), possibly allowing cellulose fibers to adopt a more crystalline structure (Obi Reddy et al. Citation2013).
Table 2. Crystallinity Index of untreated and treated BV fibers.
Mechanical property analysis
Single fiber tensile tests of untreated and various treated BV fibers were performed. Tensile strength, young’s modulus and elongation at break were investigated. Due to chemical treatment of the fibers and the changes occurred in the fiber surface texture, it was observed that it has affected the mechanical properties a lot.
shows the stress–strain curve obtained from the tensile test. The sudden drop in the stress values for both untreated and treated fibers indicated that BV fibers are brittle in nature (Maache et al. Citation2017). The mechanical properties of the untreated and treated BV fibers are shown in . The mechanical properties of the natural fiber varied substantially after chemical treatments. It was observed that BV fibers treated with benzoyl chloride exhibit the highest tensile strength of 128.56 MPa, followed by the bleaching treated fibers (88.76 MPa). The tensile strength of alkali treated fiber was 75.21 MPa, and untreated fiber was 62.28 MPa. Young’s modulus shows the similar trend of results as in tensile strength. It was observed that the Young’s modulus value of treated fibers was higher than untreated fibers. The highest value was obtained for benzoylation treated BV fiber (8.34 GPa), followed by bleaching (7.10 GPa) and then alkali treated with 4.32 GPa. The enhancement in tensile strength and Young’s modulus after surface modification can be attributed to the elimination of hemicelluloses, pectin, and lignin from the BV bast fibers (“FTIR analysis” section) and the improved crystallinity of the natural fibers (“XRD analysis” section). Shanmugasundaram et al. (Shanmugasundaram, Rajendran, and Ramkumar Citation2018) also discovered that removing noncellulose elements from natural fibers improved their tensile strength and Young’s modulus. Furthermore, Sang et al. (Sang et al. Citation2017) said that fiber surface modification could improve interfacial adhesion and stress transfer from the matrix to natural fibers to some extent. Compared to the untreated fiber, the elongation at break improved by 34.22%, 20% and 23.55% after alkali, benzoylation and bleaching treatments, respectively.
Thermogravimetric analysis (TGA)
shows the thermal degradation of untreated and chemically treated fibers from room temperature till 600°C. From the figure, it was observed that all the fibers degraded in three phases. In the first phase of degradation which takes place from 30 to 140°C, mass loss of 15%, 10%, 8% and 5% was presented for untreated, bleaching, benzoylation and alkali treated fibers, respectively. This loss was attributed to the vaporization of moisture in the natural fibers (Mayandi et al. Citation2018; Rajan, Balaji, and Saravanakumar Citation2018). It was observed that the mass loss in untreated fibers was more as compared with treated fibers due to hydrophilic nature of fibers. In the second phase, a major mass loss was observed for all the samples which took place from temperature of 210–320°C. However, if we look at benzoylation treated fiber, the second phase degradation starts at 240°C with a mass loss of around 44.5%. The highest mass loss of 55% was encountered by untreated fibers followed by 48%, 51% by alkali and bleaching fibers. This may be attributed to the thermal decomposition of the α-cellulose (Belouadah, Ati, and Rokbi Citation2015). In the final phase, the final degradation takes place with char residue. It was observed that the untreated fibers degraded at a temperature of 345°C, whereas after chemical treatment the temperature increased up to 370°C.
It was further noticed that alkali treated fibers show highest resistance to thermal degradation followed by benzoylation treated fibers. However, the mass loss encountered by benzoylation treated fibers was the lowest as compared with other treated fibers.
Conclusions
The impact of various surface chemical treatments on the chemical characteristics, surface morphology, mechanical characteristics, and thermal stability of Bauhinia vahlii bast fibers was studied, and the results were reviewed and reported in this study. Chemical treatment has enhanced the surface roughness of the fibers, which was seen from SEM images. Non-cellulosic components were removed as a result of chemical treatments, according to the findings of the FTIR investigation. The crystallinity indexes of BV fibers were enhanced after the chemical treatments, according to XRD data. Crystallinity Index of benzoylation treated BV bast fiber was found to be 70.10%. Chemical treatments increased the mechanical qualities of the fibers, which could lead to changes in chemical structures and molecular rearrangements in the BV fibers. The optimum mechanical properties were obtained for benzoylation treated BV fibers (128.56 MPa tensile strength, 8.34 GPa Young’s modulus). TGA confirms the thermal stability of the treated fibers which were improved after treatments. From the above comparison of results, it was confirmed that benzoylation treatment is the optimum method of treatment to improve the properties of BV fibers. The advances demonstrated in this work are vital for producing natural cellulosic fibers efficiently for manufacturing of diverse biopolymer composite applications in the aviation, automobile, and marine industries.
Highlights
The Bauhinia vahlii (BV) fibers were successfully extracted from the bast of the stem by retting process.
The fibers were undergone through various chemical treatments which enhanced the exposure of cellulose on the fiber surface as well as increased the surface roughness.
Benzoylation treated fibers revealed better mechanical properties as compared to untreated and other treated BV fibers.
The crystallinity indexes of BV fibers were enhanced after the chemical treatments, according to XRD data.
Bauhinia vahlii (BV) fibers can be a potential candidate as reinforcement in fabrication of polymeric composites.
The advances demonstrated in this work are vital for producing natural cellulosic fibers efficiently for manufacturing of diverse biopolymer composite applications in the aviation, automobile, and marine industries.
Acknowledgement
We are very much grateful to LARPM, Bhubaneswar for allowing us to carry out all the experiments in their lab.
Disclosure statement
The authors declare that they have no known competing financial interests or personal relationships that could have appeared to influence the work reported in this paper.
References
- Agrawal, R., N. S. Saxena, K. B. Sharma, S. Thomas, and M. S. Sreekala. 2000. Activation energy and crystallization kinetics of untreated and treated oil palm fibre reinforced phenol formaldehyde composites. Material Science and Engineering A 277:1–12. doi:10.1016/S0921-5093(99)00556-0.
- Amuthakkannan, P., V. Manikandan, J. T. W. Jappes, and M. Uthayakumar. 2013. Hybridization effect on mechanical properties of short basalt/jute fiber-reinforced polyester composites. Science and Engineering of Composite Materials 20:343–50. doi:10.1515/secm-2012-0144.
- Aziz, S. H., and M. P. Ansell. 2004. The effect of alkalization and fibre alignment on the mechanical and thermal properties of kenaf and hemp bast fibre composites. Composite Science and Technology 64 (9):1219–30. doi:10.1016/j.compscitech.2003.10.001.
- Barari, B., E. Omrani, A. D. Moghadam, P. L. Menezes, K. M. Pillai, and P. K. Rohatgi. 2016. Mechanical, physical and tribological characterization of nano-cellulose fibers reinforced bioepoxy composites: An attempt to fabricate and scale the ‘Green’ composite. Carbohydrate Polymer 147:282–93. doi:10.1016/j.carbpol.2016.03.097.
- Belouadah, Z., A. Ati, and M. Rokbi. 2015. Characterization of new natural cellulosic fiber from Lygeum spartum L. Carbohydrate Polymer 134:429–37. doi:10.1016/j.carbpol.2015.08.024.
- Cyras, V. P., S. Iannace, J. M. Kenny, and A. Vazquez. 2001. Relationship between processing conditions and properties of a biodegradable composites based on PCL/starch and sisal fibers. Polymer Composites 22 (1):104–10. doi:10.1002/pc.10522.
- Dehury, J., S. Nayak, and J. R. Mohanty. 2021. Comprehensive characterization sea purslane (Sesuvium portulacastrum) fiber and the effect of surface modifications on physical, mechanical and thermal properties. Journal of Natural Fibers 19:1–13. doi:10.1080/15440478.2021.1904476.
- Dong, Z., X. Hou, F. Sun, L. Zhang, and Y. Yang. 2014. Textile grade long natural cellulose fibers from bark of cotton stalks using steam explosion as a pretreatment. Cellulose 21 (5):3851–60. doi:10.1007/s10570-014-0401-5.
- Elbanna, A. H., E. A. H. Mahrous, A. E. S. Khaleel, and T. S. El-Alfy. 2016. Morphological and anatomical features of Bauhinia vahlii Wight & Arnott. Grown in Egypt. Journal of Applied Pharmaceutical Science 6 (12):84–93. doi:10.7324/JAPS.2016.601212.
- French, A. D. 2014. Idealized powder diffraction patterns for cellulose polymorphs. Cellulose 21 (2):885–96. doi:10.1007/s10570-013-0030-4.
- Indran, S., R. D. E. Raj, B. S. S. Daniel, and J. S. Binoj. 2018. Comprehensive characterization of natural Cissus quadrangularis stem fiber composites as an alternate for conventional FRP composites. Journal of Bionic Engineering 15 (5):914–23. doi:10.1007/s42235-018-0078-9.
- Joseph, K., L. H. C. Mattoso, R. D. Toledo, S. Thomas, L. H. de Carvalho, L. Pothen, S. Kala, and B. James. 2000. Natural Fiber Reinforced Thermoplastic Composites. In Natural polymers and agrofibers composites, ed. E. Frollini, A. L. Leao and L. H. S. Mattoso, 159–201. Embrapa, USP-IQSC, UNESP, Brazil: Scientific Research.
- Khalil, H. P. S. A., H. D. Rozman, M. N. AHMAD, and H. ISMAIL. 2000. Rice-husk polyester composites: The effect of chemical modification of rice husk on the mechanical and dimensional stability properties. Polymer-Plastics Technology and Engineering 39:757–81. doi:10.1081/PPT-100100057.
- Kumar, S. M. S., D. Duraibabu, and K. Subramanian. 2014. Studies on mechanical, thermal and dynamic mechanical properties of untreated (raw) and treated coconut sheath fiber reinforced epoxy composites. Material Design 59:63–69. doi:10.1016/j.matdes.2014.02.013.
- Liu, Y. C., Y. H. Ma, J. T. Yu, J. Zhuang, S. Y. Wu, and J. Tong. 2019b. Development and characterization of alkali treated abaca fiber reinforced friction composites. Composite Interfaces 26 (1):67–82. doi:10.1080/09276440.2018.1472456.
- Liu, Z., and B. H. Tisserat. 2018. Coating applications to natural fiber composites to improve their physical, surface and water absorption characters. Industrial Crops and Products 112:196–99. doi:10.1016/j.indcrop.2017.12.002.
- Liu, Y. C., J. Xie, N. Wu, L. D. Wang, Y. H. Ma, and J. Tong. 2019a. “Influence of silane treatment on the mechanical, tribological and morphological properties of corn stalk fiber reinforced polymer composites. Tribology International 131:398–405. doi:10.1016/j.triboint.2018.11.004.
- Maache, M., A. Bezazi, S. Amroune, F. Scarpa, and A. Dufresne. 2017. Characterization of a novel natural cellulosic fiber from Juncus effusus L. Carbohydrate Polymer 171:163–72. doi:10.1016/j.carbpol.2017.04.096.
- Mayandi, K., N. Rajini, P. Pitchipoo, J. T. W. Jappes, and A. V. Rajulu. 2018. Properties of untreated and chemically treated Cissus quadrangularis natural fibers and their composites with polyester as the matrix. Polymer Composite 39 (3):876–86. doi:10.1002/pc.24011.
- Mishra, S., M. Misra, S. S. Tripathy, A. K. Mohanty, and A. K. Mohanty. 2001. Graft co-polymerization of acrylonitrile on to chemically modified sisal fibers: Characterization and physico-mechanical properties. Macromolecular Materials and Engineering 286:107–13. doi:10.1002/1439-2054(20010201)286:2<107:AID-MAME107>3.0.CO;2-0.
- Mobarak, T. A., M. A. Gafur, M. A. Gafur, S. A. Dhar, and S. A. Dhar. 2018. Effect of chemical modifications on surface morphological, structural, mechanical, and thermal properties of sponge-gourd natural fiber. Fibers and Polymers 19:31–40. doi:10.1007/s12221-018-7199-3.
- Morrison, I. W. H., D. D. Archibald, S. Sharma, and D. E. Akin. 2000. Chemical and physical characterization of water- and dew-retted flax fibers. Industrial Crops and Products 12:39–46. doi:10.1016/S0926-6690(99)00044-8.
- Murasing, K. K., M. Kumar, and A. Kumar. 2021. Effect of altitude on the mechanical strength of Grewia optiva fiber in Garhwal Himalaya, India. Journal of Natural Fibers 19:1–10. doi:10.1080/15440478.2021.1929652.
- Nayak, S., and J. R. Mohanty. 2018. Influence of chemical treatment on tensile strength, water absorption, surface morphology, and thermal analysis of areca sheath fibers. Journal of Natural Fibers 16 (4):589–99. doi:10.1080/15440478.2018.1430650.
- Nayak, S. Y., M. T. H. Sultan, S. B. Shenoy, C. R. Kini, R. Samant, A. U. M. Shah, and P. Amuthakkannan. 2022. Potential of natural fibers in composites for ballistic applications–a review. Journal of Natural Fibers 19 (5):1648–58. doi:10.1080/15440478.2020.1787919.
- Obi Reddy, K., C. U. Maheswari, M. Shukla, J. I. Song, and A. V. Rajulu. 2013. Tensile and structural characterization of alkali treated Borassus fruit fine fibers. Composite B Engineering 44 (1):433–38. doi:10.1016/j.compositesb.2012.04.075.
- Paul, S., N. Puja, and G. Rajive. 2003. PhCocl-Py/Basic alumina as a versatile reagent for benzoylation in solvent-free conditions. Molecules 8:374–80. doi:10.3390/80400374.
- Premalatha, N., S. S. Saravanakumar, M. R. Sanjay, S. Siengchin, and A. Khan. 2021. Structural and thermal properties of chemically modified luffa cylindrica fibers. Journal of Natural Fibers 18 (7):1038–44. doi:10.1080/15440478.2019.1678546.
- Rajan, B. S., M. A. S. Balaji, and S. S. Saravanakumar. 2018. Effect of chemical treatment and fiber loading on physico-mechanical properties of Prosopis juliflora fiber reinforced hybrid friction composite. Material Research Express 6 (3):035302. doi:10.1088/2053-1591/aaf3cf.
- Ray, D., B. K. Sarkar, A. K. Rana, and N. R. Bose. 2001. Effect of alkali treated jute fibres on composite properties. Bulletin of Material Science 24:129–35. doi:10.1007/BF02710089.
- Rong, M. Z., M. Q. Zhang, Y. Lui, G. C. Yang, and H. M. Zeng. 2001. The effect of fibre treatment on the mechanical properties of unidirectional sisal reinforced epoxy composites. Composites Science and Technology 6:1437–47. doi:10.1016/S0266-3538(01)00046-X.
- Sang, L., M. Zhao, Q. Liang, and Z. Wei. 2017. Silane-treated basalt fiber-reinforced poly(butylene succinate) biocomposites: Interfacial crystallization and tensile properties. Polymers 9 (8):351. doi:10.3390/polym9080351.
- Sangappa, B. L., A. S. Rao, R. M. Kumar, R. Somashekar, and R. Somashekar. 2014. Physical, chemical, and surface properties of alkali-treated Indian hemp fibers. Composite Interfaces 21:153–59. doi:10.1080/15685543.2013.855485.
- Sanjay, M. R., P. Madhu, M. Jawaid, S. Pradeep, P. Senthamaraikannan, and S. Senthil. 2018. Characterization and properties of natural fiber polymer composites: A comprehensive review. Journal of Cleaner Production 172:566–81. doi:10.1016/j.jclepro.2017.10.101.
- Sanjay, M. R., S. Siengchin, J. Parameswaranpillai, M. Jawaid, C. I. Pruncu, and A. Khan. 2019. A comprehensive review of techniques for natural fibers as reinforcement in composites: Preparation, processing and characterization. Carbohydrate Polymer 207:108–21. doi:10.1016/j.carbpol.2018.11.083.
- Sarasini, F., and V. Fiore. 2018. A systematic literature review on less common natural fibres and their biocomposites. Journal of Cleaner Production 195:240–67. doi:10.1016/j.jclepro.2018.05.197.
- Segal, L., J. J. Creely, A. E. J. Martin, and C. M. Conrad. 1959. An empirical method for estimating the degree of crystallinity of native cellulose using the x-ray diffractometer. Textile Research Journal 29 (10):786–94. doi:10.1177/004051755902901003.
- Sepe, R., F. Bollino, L. Boccarusso, and F. Caputo. 2018. Influence of chemical treatments on mechanical properties of hemp fiber reinforced composites. Composite B Engineering 133:210–17. doi:10.1016/j.compositesb.2017.09.030.
- Shanmugasundaram, N., I. Rajendran, and T. Ramkumar. 2018. Characterization of untreated and alkali treated new cellulosic fiber from an Areca palm leaf stalk as potential reinforcement in polymer composites. Carbohydrate Polymer 195:566–75. doi:10.1016/j.carbpol.2018.04.127.
- Singh, T., B. Gangil, A. Patnaik, D. Biswas, and G. Fekete. 2019. Agriculture waste reinforced cornstarch-based biocomposites: Effect of rice husk/walnut shell on physicomechanical, biodegradable and thermal properties. Material Research Express 6 (4):045702. doi:10.1088/2053-1591/aafe45.
- Stocchi, A., B. Luke, A. Vazquez, and C. Bernal. 2007. A novel fiber treatment applied to woven jute fabric/vinyl ester laminates. Composites Particle A: Applications Sciences Manufacturing 38 (5):1337–43. doi:10.1016/j.compositesa.2006.10.010.
- Thakur, V. K., M. K. Thakur, and R. K. Gupta. 2014. Review: Raw natural fiber-based polymer composites. International Journal of Polymer Analysis and Characterization 19 (3):256–71. doi:10.1080/1023666X.2014.880016.
- Vaisanen, T., O. Das, and L. Tomppo. 2017. A review on new biobased constituents for natural fiber-polymer composites. Journal of Cleaner Production 149:582–96. doi:10.1016/j.jclepro.2017.02.132.
- Venkata Krishna, K., and K. Kanny. 2016. The effect of treatment on kenaf fiber using green approach and their reinforced epoxy composites. Composite B Engineering 104:111–17. doi:10.1016/j.compositesb.2016.08.010.
- Vinod, A., R. Vijay, D. L. Singaravelu, M. R. Sanjay, S. Siengchin, Y. Yagnaraj, and S. Khan. 2021. Extraction And characterization of natural fiber from stem of cardiospermum halicababum. Journal of Natural Fibers 18 (6):898–908. doi:10.1080/15440478.2019.1669514.
- Zhou, Y., M. Fan, and L. Chen. 2016. Interface and bonding mechanisms of plant fibre composites: An overview. Composite B Engineering 101:31–45. doi:10.1016/j.compositesb.2016.06.055.