Abstract
The use of ultraviolet germicidal irradiation (UVGI) to combat disease transmission has come into the international spotlight again because of the recent SARS-CoV-2 pandemic and ongoing outbreaks of multidrug resistant organisms in hospitals. Although the implementation of ultraviolet disinfection technology is widely employed in healthcare facilities and its effectiveness has been repeatedly demonstrated, the use of such technology in the commercial sector has been limited. Considering that most disease transmission occurs in commercial, public, and residential indoor environments as opposed to healthcare facilities, there is a need to understand whether ultraviolet (UV) disinfection technology can be effective for mitigating disease transmission in these environments. The results presented here demonstrate that the installation of fixed in-room UVGI air cleaners in commercial buildings, including restaurants and offices, can produce significant reductions in both airborne and surface-borne bacterial contamination. Total airborne reductions after UV implementation at six separate commercial sites averaged 73% (p < 0.0001) with a range of 71–88%. Total non-high touch surface reductions after implementation averaged 55% (p < 0.0001) with a range of 28–88%. All reductions at the mitigated sites were statistically significant. The mean value of indoor airborne bacteria was 320 CFU/m3 before intervention and 76 CFU/m3 after. The mean value of indoor non-high touch surface borne bacteria was 131 CFU/plate before intervention and 47 CFU/plate after. All test locations and controls had their required pandemic cleaning procedures in place for pre- and post-sampling events. Outdoor levels of airborne bacteria were monitored and there was no significant correlation between the levels of airborne bacteria in the outside air as opposed to the indoor air. Rooms with fixed in-room UVGI air cleaners installed had significant CFU reductions on local surface contamination, which is a novel and important finding. Installation of fixed in-room UVGI air cleaners in commercial buildings will decontaminate the indoor environment and reduce hazardous exposure to human pathogens.
Introduction
The current SARS coronavirus (SARS-CoV-2) pandemic has heightened global awareness of exposure to pathogens in contaminated indoor environments. Increasing evidence of airborne spread of the COVID-19 coronavirus has accumulated (Zhang et al. Citation2020). The use of ultraviolet germicidal irradiation (UVGI) as a supplemental treatment to control SARS-CoV-2 in indoor environments has been addressed by the American Society of Heating, Refrigerating, and Air-conditioning Engineers (ASHRAE) and the Food and Drug Administration (ASHRAE Citation2020a; FDA Citation2020). ASHRAE has taken the position that airborne infectious diseases are a growing threat to human health and that building designers, owners, and operators should give high priority to enhancing well-designed, installed, commissioned, and maintained HVAC systems with supplemental filtration and UVGI systems (ASHRAE 2014/Citation2020).
Ultraviolet disinfection technology has been in use for the past century and has been widely implemented in both healthcare and other environments for the past few decades. The effectiveness of ultraviolet light has been demonstrated against hundreds of bacteria, viruses, and fungi in laboratory studies (Kowalski Citation2009). Numerous recent studies have demonstrated that ultraviolet light can significantly reduce both surface contamination and hospital associated infections (Anderson et al. Citation2017; Marra et al. Citation2018; Rutala et al. 2018). Although the primary focus of research into ultraviolet disinfection has been in healthcare, the fact remains that most infectious diseases are transmitted within the community and not in healthcare environments. Qian et al. (Citation2021) determined that at least 99.9% of COVID-19 transmission events in China occurred indoors as opposed to outdoors. Human pathogenic viruses and bacteria, in general, do not survive in the outside air due to sunlight and oxygenation, and rapidly mix or diffuse to harmless concentrations. These same pathogens, expelled or shed by humans, can survive long enough indoors to be transmitted to other hosts.
Indoor environments where people work or gather, foster the transmission of airborne infections by providing a habitat where microbes can survive long enough to cause secondary infections. The mean survival time for any microbe in indoor air or on indoor surfaces ranges from minutes to months, depending on the species (Kramer et al. Citation2006). Common bacteria such as Acinetobacter baumannii, Staphylococcus aureus (MRSA), and Pseudomonas aeruginosa can persist for months on dry surfaces. Viruses such as coronavirus (3 hr), influenza virus (1–2 days), and rhinovirus (7 days) also persist on dry indoor surfaces (Kramer et al. Citation2006). SARS-CoV-2 is reported to survive for up to 3 hr in air and up to 72 hr on stainless steel surfaces (van Doremalen et al. Citation2020).
There are three primary modes of disease transmission: (1) contact transmission, (2) droplet transmission, and (3) airborne transmission (Delikhoon et al. Citation2021). Many pathogens transmit by more than one of these routes. Multiple routes can be involved in any case of transmission. At any point in time contamination may exist in both the air and on surfaces. Influenza, for example, can transmit by inhalation, ingestion (contact), and by contact with fomites. Fomites, or contaminated surfaces, may be produced by droplets or aerosols that settle on surfaces. In addition, pathogens on surfaces such as floors or beds can become aerosolized and re-entrained into the air when disturbed by normal activities including routine cleaning. Simply walking on a floor is sufficient to re-aerosolize pathogens and create an inhalation hazard (Rosati et al. Citation2008; Paton et al. Citation2015).
The use of UVGI for air disinfection has a long history of successful applications but recent concerns over airborne SARS-CoV-2 virus have heightened awareness of this approach for mitigation of infection transmission and resulted in guidance and recommendations from a variety of agencies and organizations. The American Society of Refrigerating and Air Conditioning Engineers (ASHRAE) Epidemic Task Force has recommended using combinations of filters and air cleaners for air recirculated by HVAC systems (ASHRAE Citation2020b). The Environmental Protection Agency (EPA) has long suggested the use of UVGI for air disinfection in building retrofits to address biological releases (EPA Citation2007). Although the EPA does not yet impose performance requirements on UV devices, they have stated that manufacturers of such devices must maintain records, with information and data, to substantiate any claims (EPA Citation2020). In response to the COVID-19 pandemic, the FDA has issued nonbinding recommendations for the use of UV air disinfection systems which they believe may help reduce the risk of viral exposure (FDA Citation2020).
The subject system is a fully enclosed UVGI air treatment unit with forced air that is permanently installed in a room or is installed in large areas as part of an array of installed units intended to clean the air in a building or any zone within a building. These indoor air UVGI treatment systems are fully enclosed and pose no safety hazard to occupants. An alternate type of UVGI air treatment system, Upper Room UVGI, treats air in the upper part of the room but depends entirely on local air currents for circulation rather than forced air. Guidelines for Upper Room systems have long been available due to their effectiveness against airborne tuberculosis. The Centers for Disease Control (CDC)/National Institute for Occupational Safety and Health (NIOSH) provide Guidelines for Upper-Room UVGI Systems, which are intended for tuberculosis control in healthcare settings, but are also commonly used as a design basis for Upper Room UVGI systems in commercial, school, or home applications (CDC/NIOSH Citation2009; First et al. Citation1999). Although Upper Room UVGI systems are relatively simple to install, and are tested and adjusted for safety, their adoption in large commercial facilities has been hindered by concerns over the safety hazards of employing UV radiation in occupied rooms (APHC Citation2020).
The outdoor air is relatively free of hazardous pathogens and tends to be self-sterilizing because of sunlight, temperature, and oxygenation, as well as air mixing and diffusion which reduces concentrations. Outdoor air is used to replace indoor air as a percentage of the total supply airflow while the remainder of the supply air is recirculated. Both the outside air and the supply air are typically filtered with dust filters (i.e., MERV 6–8 filters) but these may have minimal effect on bacteria and viruses although they tend to remove larger spores (Kowalski Citation2009). ASHRAE has recommendations for improving indoor air quality by improving filtration in their recommendations for reducing aerosol exposure to infectious pathogens such as COVID-19 (ASHRAE Citation2020b).
In the case of the current pandemic, the transmission of the SARS-CoV-2 virus via small, exhaled aerosols (<5–10 microns) is recognized as an important pathway of spread for COVID-19. Once the liquid in an aerosolized droplet evaporates, a smaller virus-containing dried residue is left behind. These smaller aerosols could carry more viral load than larger droplets since they originate from deep in the respiratory tract where there are higher concentrations of virus. Numerical modeling with computational fluid dynamics (CFD) has been used to help determine the spread and transport of droplets via sneezing, coughing, and other expiratory events and there have been several quantitative studies simulating the spread and deposition of viral droplets and aerosols in enclosed spaces through CFD simulations (Narayanan and Yang Citation2021). These studies support the idea that ventilation, airflow streamlines, aerosol/droplet size, and modes of aerosol injection are important factors that affect the transport, deposition, and suspension of airborne droplets and aerosols. When general room ventilation was combined with the use of room air purifiers, Narayanan and Yang (Citation2021) concluded that the use of room air purifiers could offer significant benefits if the purifiers were strategically placed to capture the injected aerosols.
New buildings with appropriately designed ultraviolet disinfection systems may have a general effect on reducing indoor airborne pathogens because they are delivering clean air through a clean HVAC system. There is no guarantee that any existing ventilation system will adequately distribute clean air to every part of the building. The distinction between the three traditional routes of transmission: contact, droplet, and airborne transmission is often blurred in real-world situations. The current SARS-CoV-2 pandemic has further demonstrated multimodal transmission and has made it obvious that these routes of transmission can overlap as expelled particles are subject to variable environmental conditions of temperature, humidity, and air currents in the indoor environment. Social distancing is an administrative control that can help avoid transmission with large droplets and certainly cleaning surfaces will help with prevention of contact transmission and fomite recirculation, but the indoor environment is like a snow globe; what goes up must come down and the very small particles generated by talking, coughing, breathing, and sneezing will disperse, circulate, and settle on surfaces or become re-entrained by air currents in the breathing zone within each the room. Commercial building ventilation systems were designed for comfort and not intended to disinfect air, and even when retrofitted with UV lamps there may be limitations related to air distribution and with removal of contaminated air at the source (Nardell Citation2021).
Commercial buildings frequented by consumers or patrons, are often cleaned manually at the end of the day (i.e., terminal cleaning) according to standard practices and appropriate regulations. The cleaning methods are like those used in hospitals, with detergents and disinfectants or disinfectant wipes used to clean restrooms on all high touch surfaces as well as the floors and walls. Studies in hospitals have shown that the traditional cleaning and disinfection protocols are often substandard, with contaminated areas sometimes being missed, and with insufficient concentrations of chemical disinfectants being used for shorter lengths of time than the manufacturers recommend (Armellino et al. Citation2020; Omidbakhsh Citation2010). Recent studies have demonstrated that the combined effects of routine chemical cleaning and ultraviolet disinfection are synergistic and provide greater reductions of contaminants than either approach by itself (Rutala et al. 2018). The use of UV technology as an adjunct to chemical disinfection has been recommended by the CDC and is commonly used in most large hospitals, spurred by the COVID-19 pandemic.
Publications on the use of UV air disinfection in commercial buildings outside of healthcare have been limited. One major epidemiological study by Menzies et al. (Citation2003) showed that the use of UV air disinfection in a commercial office building could reduce respiratory symptoms (i.e., coughing, sneezing, etc.) from a normal level of 3.9% to 2.9%, representing a net decrease of 27% in respiratory symptoms. Studies have shown that people are the ultimate source of disease transmission spread in indoor environments, and this has become more evident during the pandemic with super spreader events. The advantage of controlling pathogens near the source, in the rooms where people gather and work together to share common activities and where pathogen concentrations are higher, was the focus of this study.
This study examines whether fixed UVGI room air cleaners, installed in high-occupancy rooms, can provide significant reductions of both airborne and surface microbial contamination in occupied commercial indoor environments. These real-world environments include restaurants (QSR or Quick Service Restaurant), offices, meeting rooms, waiting areas, restrooms, and other facilities where occupants may disseminate pathogens and where these pathogens may survive in air or on surfaces for extended periods of time.
Method and materials
Study setting and design
This intervention study was performed at six sites where airborne (AB) and surface-borne (SB) bacteria were measured before and after room-based UVGI air treatment. Four separate sites were used as controls while two of the sites included their own control sites, which were physically separate, virtually identical, areas within the same building. A total of 2,673 plates were used for airborne and surface Pre- and Post-sampling. Between eight and 16 plates were used as sterility controls for each site and were handled identically to the sample plates. For air samples, 36 plates were used to sample Outside Air for comparison purposes. provides a breakdown of the Mitigated locations where the UV room air cleaners were installed, and the Control locations and the number of plates used in each case. Missing plate data in was due to damaged or missing plates. Air samples were collected using a SAS 180 impaction sampler on blood agar (TSA with 5% sheep blood). Surface samples were collected using Replicate Organism Detection and Counting (RODAC) contact plates with blood agar with tween and lecithin. Irregular (non-flat) surfaces were sampled using swabs. All samples were processed by independent third-party labs EMSL (Denver, CO) or the Veteran’s Administration (Cleveland, OH).
Table 1. Number of sample plates used at each site.
The selected sites met the criteria for normal human-occupied indoor environments and were tested based on availability. These sites included popular fast-food restaurants, coffee shops, an office building, and a production studio. The controls for four of the sites were selected based on similarity to the mitigated site while two of the sites used onsite rooms as controls.
Description of the UV-C air disinfection devices
The air disinfection system (UV Angel Clean Air, UV Partners, Inc., Grand Haven, MI) consists of a UV germicidal lamp (254 nm, 27 W UV output) enclosed within a chamber through which air is drawn by fans through a MERV 6 filter. The total power draw for the unit is 115 W. A constant airflow of 0.0236 m3/sec (50 cfm) airflow is exposed to the UV germicidal lamp inside the irradiation chamber during each pass, with an exposure time of at least 0.7 seconds. The exit velocity of the airstream is at least 293 fpm. These units have internal MERV 6 prefilters and high intensity sealed UV chambers that are integrated into a 2′ × 4′ light fixture installed in the ceiling (see ). The unit weighs about 22 lb. This system is certified as safe for its intended use and can easily be installed by most facility and HVAC technicians without the need for adjustments or additional UV safety testing. Periodic maintenance includes annual replacement of the UV lamps and replacement of the prefilter.
Figure 1. Illustration of the UVGI air cleaner fixed in-place above the ceiling, showing return air and supply air paths to the zone or room below.
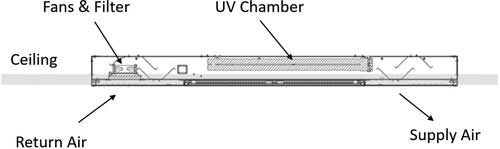
Once installed, the unit becomes part of the facility infrastructure and is out-of-sight, thus preventing obstacles, a tripping hazard or people interfacing with the unit. The air cleaner operates automatically and does not require staff to turn it on or off and operates quietly at about 41 dBA. The air is drawn directly from the room or local area, treated, and delivered back to the room. The air cleaning unit is not connected to the HVAC system or duct and acts independently of those systems. It also does not disrupt the HVAC system or affect room pressurization. The air treatment systems are installed per the manufacturer’s instructions to provide local coverage per the range of floor areas shown in . The device operates continuously to treat the recirculated room air and treats the air with a 0.7-sec exposure time with each passage through the chamber. The number of devices installed at each site is listed in , which shows the coverage of the unit in cubic meters (m3) per each unit, for each respective ceiling height. All existing building ventilation systems operated normally during the testing periods.
Table 2. Floor area and unit coverage per facility volume.
Room cleaning and disinfection protocols
At each site, the normal cleaning procedures were performed by staff and consisted of enhanced pandemic cleaning methods. The facilities personnel followed their normal location cleaning procedures prior to installation pre-testing and post installation testing and at the Control sites as well. The protocols were maintained consistently throughout the testing period and the staff were blinded to the intervention study.
Sampling protocols
Trained personnel followed best practices, using three sampling techniques; (1) air sampling using a SAS Impactor, (2) RODAC contact plate surface sampling, and (3) swab sampling for non-flat surfaces. All plates were labeled in advance with a unique ID number and were blinded for counting until final reporting of results. All plates were delivered to an independent third-party laboratory for enumeration and counting. Standard analytical methods were used for enumerating total viable bacteria CFUs (CFU/m3 or CFU/plate). Non-high touch surface samples were collected over 2 days during a 16-hr period each day to randomize sampling during the normal the work shift and irrespective of cleaning schedules. The pre-testing and the post-testing procedures for all intervention and control sites were matched with each other on location, day of the week, and approximate time.
Air sampling
All air samples were collected with a SAS Super 180 microbial air sampler (Bioscience International, Rockville, MD) employing 90-mm Petri dishes containing blood agar. The D50 particle cutoff size for the SAS sampler was 1.51 μm. The aspirating head was cleaned with isopropyl alcohol pads and allowed to dry before use. After insertion of the sample plate, the SAS impactor was operated for approximately 5.5 min, drawing a total of 1,000 L of air. The sampling unit was in current calibration tolerance and has a preprogrammed timer to ensure accuracy of air volume, and a correction factor was applied to account for the sampler holes per the manufacturer’s instructions. All plates were delivered to an independent third-party laboratory for enumeration and counting. Bacterial colony forming units were enumerated and the concentration, CFU/cubic meter of air, was calculated.
A minimum of two air samples, not counting controls, were taken at each location and these were spaced 1 hr apart over approximately 16 hr over the course of 2 days. Samples were taken in designated indoor areas during normal occupied periods and samplers were positioned between 1 and 1.5 m above the floor to collect breathing zone samples.
Surface sampling
Surface samples were taken using RODAC plates for flat areas and swab sampling for irregular shapes like computer keyboards. The RODAC plates included blood agar, supplemented with lecithin and Tween to neutralize disinfectant residues on antimicrobial surface cleaning agents. Bacterial samples from flat non-high-touch surfaces were taken using TSA contact plates (RODAC). Firm pressure was applied to each plate for 30 sec.
Swab samples (ESwab 480 C regular flocked swab) were used for irregular or curved surfaces and employed liquid Amies medium for collection. An area of 25 cm2 was defined with a template within which the area was swabbed, an area equivalent to the surface area of the RODAC contact plates used for flat surfaces.
Statistical methods
Surface contamination data are reported as CFU/plate for the RODAC plates and swab samples. Air contamination data are reported as CFU/cubic meter of air. Pre- and post-samples were compared for each of the three conditions, Mitigated, Control, and Outside Air, as an additional control. Because of their non-normal distribution, data were analyzed using the Wilcoxon Signed Rank Sum test. Correlations were analyzed between Outside Air and indoor air contamination levels using a Two Sample t-Test (NCSS 11).
Results
A summary of all the mitigated site results is provided in and includes the % Reduction (% Red.) for the individual rooms sampled in each case. Overall, there was a 73% reduction (p < 0.0001) for airborne bacteria and a 55% reduction (p < 0.0001) for surface bacteria, relative to the control sites. provides the detailed information on the control sites. The % reductions for the control sites, which included both positive negative reductions, were mostly not statistically significant and were within the normal range of variation for all data sets. Statistically significant reductions may have been due to variable occupancy, which was not controlled. Missing plate data in and was due to damaged or missing plates.
Table 3. Average mitigated pre and post CFUs for airborne and surface samples with p-values shown for Wilcoxon signed rank sum test.
Table 4. Average control pre- and post-CFUs for airborne and surface samples with p-values shown for Wilcoxon signed rank sum test.
There was an overall 12% reduction (p < 0.0001) for airborne bacteria and a 14% reduction (p < 0.0001) for surface bacteria when all data were pooled. illustrates the average airborne Pre- and Post-sample CFU/m3 for the mitigated results (sites 1–6) and the control results (sites 7–12). shows the average surface borne sample CFU/plate showing the mitigated sites and the control sites. For the control sites, no significant differences could be demonstrated between the Pre- and Post-samples. compares the Pre- and Post-CFUs for all mitigated sites for airborne and surface samples.
Figure 2. Average airborne CFU/m3 for each of the mitigated sites (Sites 1–6) and each of the control sites (Sites 7–12). Error bars indicate the standard error of the mean (SEM).
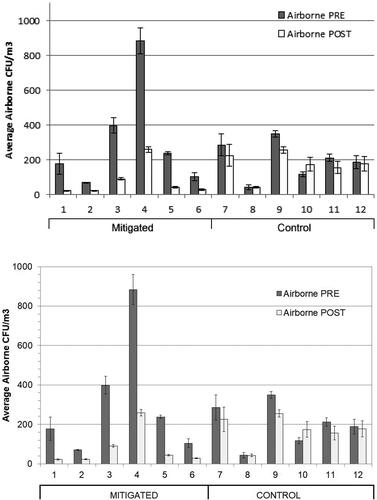
Figure 3. Average surface borne CFU for each of the mitigated sites (Sites 1–6) and each of the control sites (Sites 7–12). Error bars indicate the SEM. No surface samples were taken for site 5.
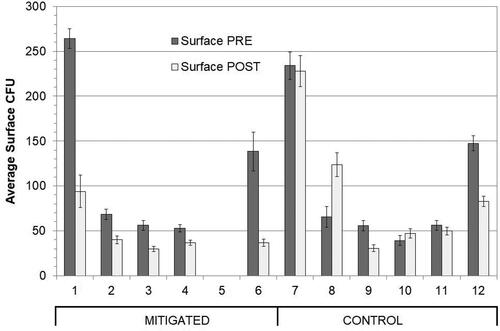
Figure 4. Comparison of pre and post CFUs for all mitigated sites for airborne and surface samples. Error bars indicate the SEM.
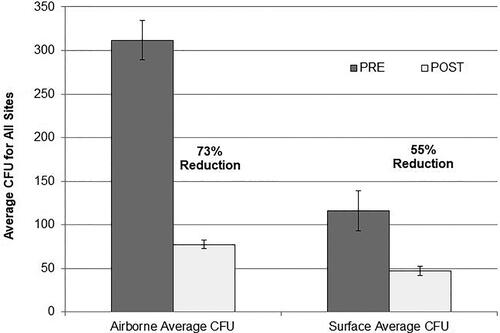
displays a comparison of average Pre- and Post-CFUs for Outside Air, Control, and Mitigated sites for airborne samples. The average airborne reduction for Outside Air was 21% (p = 0.349) but the p-value indicates this reduction was not significant. The Outside Air Pre- and Post-samples were compared site-by-site with the average indoor airborne samples to check for any correlation using a statistical t-test. Results indicated there were no significant differences (p = 0.44).
Discussion
The results presented here demonstrate the context independent effectiveness of ultraviolet air treatment with significant reductions of both airborne levels of bacteria and levels of surface contamination noted following UV treatment. These reductions were achieved with good consistency across all the commercial sites tested. The overall results confirm that fixed in-room UVGI air cleaners can achieve high levels of decontamination in typical populated commercial indoor environments. These findings corroborate previous studies and confirm that a well-designed and well-implemented air disinfection system can be safe and highly effective. In addition, these systems have the added benefit of improved surface disinfection in commercial buildings. The fact that bacteria were significantly reduced from air and surfaces suggests that in room ultraviolet systems such as the unit tested will be effective against viruses like SARS-CoV-2. The UV Dose of 16.9 mJ/cm2 would result in >99.9% inactivation of SARS-COV-2 in one pass. SARS-CoV-2 is less resistant to ultraviolet disinfection than are many of the bacteria commonly found in indoor environments such as Staphylococcus aureus (D90 value of 260 mJ/cm−) and E. coli (D90 value of 400 mJ/cm2) (Kowalski Citation2009; Biasin et al. Citation2021). This study also suggests that by treating the air in the occupied rooms that contain the sources of contamination, there is a significant impact on surface bacteria loads, which could reduce the use of chemical cleaning agents.
Total airborne reductions for the six sites averaged 73% with a range of 71–88%. Although there are no comparable field studies in commercial buildings, a recent hospital study using a similar UV device showed a 27% reduction in airborne bacterial contamination with a range of −5 to +44% (Hakim et al. Citation2019). Another study using a similar in-room UVGI device showed a 62% reduction in bacterial contamination (Guimera et al. Citation2018). A hospital study employing a similar UV device showed a reduction of 42% in airborne bacteria and healthcare-associated infections (HAIs) at this facility decreased significantly (Ethington et al. Citation2018). Significant reductions in HAIs were also demonstrated with a similar device in a long-term care ventilator unit (Kane et al. Citation2018). Reductions in both airborne and surface-borne bacterial contamination were also demonstrated by a similar device when installed in inpatient units in acute care hospitals (Lee Citation2020).
The finding that non-high touch surfaces developed significant reductions of bacterial contamination levels because of the building using air disinfection system is novel and suggests air treatment may play a major role in helping keep indoor environments decontaminated. The theoretical basis is simple—airborne microorganisms continuously settle out from the air onto mainly horizontal surfaces, creating fomites. Any significant reduction in airborne levels of biocontaminants should result in a reduction of contamination on surfaces. It is likely, though not examined in this study, that treatment of air in rooms will result in a reduction of both airborne transmission and fomite mediated transmission of infectious diseases.
No significant correlations were found between the levels of airborne bacteria in outside air versus indoor air. This should not be surprising since there are few pathogenic bacteria found in outside air, with most being environmental bacteria that pose no hazard to humans (Lee et al. Citation2021). One notable exception is Pseudomonas aeruginosa which is commonly found in indoor and outdoor air and can cause opportunistic and antibiotic-resistant infections. In general, the bacteria found in indoor air consists of a mixture of ambient environmental bacteria (e.g., Bacillus subtilis), human commensal bacteria (e.g., Staphylococcus and Streptococcus species), and occasional opportunistic or pathogenic bacteria that hail from humans (e.g., Klebsiella, Acinetobacter) or sometimes bacteria that are generated in the building itself (e.g., Legionella) when systems are not properly maintained.
Limitations of this study include the fact that the sampled bacteria were not speciated; a few of the surface samples were taken using swabs while most were taken using RODAC contact plates, which is a minor inconsistency and had to be controlled for since the methods may not be equally sensitive. Occupancy levels varied depending on the commercial location but were not monitored. The outside air delivered by each building’s ventilation system may have caused some variability but because HVAC systems vary in their components and controls, the pre- and post-sampling environments within each commercial space remained unchanged during the study. Finally, the study duration was too short to determine any positive benefits in terms of reduction of disease or disease symptoms and these types of organizations do not have a formal process for contact tracing of workplace associated illnesses. Future research is warranted to investigate whether there is a reduction of both airborne infections and those infections transmitted by contact with surfaces (fomites).
Conclusions
Implementation of UV room air cleaners can significantly improve indoor environments in terms of ambient levels of biocontamination in the air and on surfaces, thereby decreasing the risk of infections for employees, patrons, students, staff, and occupants of commercial buildings such as offices and restaurants. The finding that UVGI air treatment can reduce surface levels of bacteria in rooms is novel and an approach worth serious consideration by many types of commercial and healthcare facilities. This approach may also help reduce the volume and number of chemical agents needed for cleaning and their associated environmental burden because of constant cleaning and disinfection with chemicals. This fixed in-room UVGI air cleaner can be safely used in occupied spaces, and no ozone is emitted by the process. In this age of pandemics, it may be inevitable that buildings will increasingly be retrofitted with air and surface treatment systems, particularly UV-C systems, to provide enhanced protection against infectious disease transmission. This study advances the body of literature supporting the practice of air disinfection, corroborating previous studies in both hospitals and commercial buildings that have shown the potential benefit of an enclosed UV-C room air cleaner to reduce infection transmission and protect occupants.
Data availability statement
The data that support the findings of this study are openly available in figshare.com at 16725346.
References
- Anderson DJ, Chen LF, Weber DJ, Moehring RW, Lewis SS, Triplett PF, Blocker M, Becherer P, Schwab JC, Knelson LP, et al. 2017. Enhanced terminal room disinfection and acquisition and infection caused by multidrug-resistant organisms and Clostridium difficile (the Benefits of Enhanced Terminal Room Disinfection study): a cluster-randomised, multicentre, crossover study. Lancet. 89(10071):805–814.
- APHC. 2020. Effectiveness and safety of ultraviolet germicidal irradiation lamps used for air and surface disinfection. U.S. Army Public Health Center. TIP No. 24–103–1020. October 2020. 1–5 [accessed 2021 Oct 20] https://phc.amedd.army.mil/PHC%20Resource%20Library/cv19-TIP_No_98-113-0420_MeasurestoModifyBldgHVACforHealthandComfort.pdf
- Armellino D, Goldstein K, Thomas L, Walsh TJ, Petraitis V. 2020. Comparative evaluation of operating room terminal cleaning by two methods: focused multivector ultraviolet (FMUV) versus manual-chemical disinfection. Am J Infect Control. 48(2):147–152. doi:https://doi.org/10.1016/j.ajic.2019.10.009
- ASHRAE. 2014/2020. ASHRAE position document on airborne infectious diseases, February 5th, 2020. American Society of Heating, Refrigerating and Air-Conditioning Engineers. [accessed 2021 Oct 27]. http://ashrae.org/file%20library/about/position%20documents/airborne-infectious-diseases.pdf.
- ASHRAE. 2020a. ASHRAE coronavirus (COVID-19) response resources, American Society of Heating, Refrigerating and Air-Conditioning Engineers. [accessed 2021 Oct 27]. http://ashrae.org/technical-resources/resources.
- ASHRAE. 2020b. ASHRAE epidemic task force: core recommendations for reducing airborne infections aerosol exposure, American Society of Heating, Refrigerating and Air-Conditioning Engineers. [accessed 2021 Oct 27]. https://www.ashrae.org/file%20library/technical%20resources/covid-19/core-recommendations-for-reducing-airborne-infectious-aerosol-exposure.pdf.
- Biasin M, Bianco A, Pareschi G, Cavalleri A, Cavatorta C, Fenizia C, Galli P, Lessio L, Lualdi M, Tombetti E, et al. 2021. UV-C irradiation is highly effective in inactivating SARS-CoV-2 replication. Sci Rep. 11(1):6260.
- CDC/NIOSH. 2009. Environmental control for tuberculosis: basic upper-room ultraviolet germicidal irradiation guidelines for healthcare settings. Atlanta (GA): Department of Health and Human Services, Centers for Disease Control and Prevention, National Institute for Occupational Safety and Health. DHHS (NIOSH) Publication Number 2009-105.
- Delikhoon M, Guzman MI, Nabizadeh R, Norouzian Baghani A. 2021. Modes of transmission of severe acute respiratory syndrome-coronavirus-2 (SARS-CoV-2) and factors influencing on the airborne transmission: a review. Int J Environ Res Public Health. 18(2):395. doi:https://doi.org/10.3390/ijerph18020395
- EPA. 2007. Building retrofits for increased protection against airborne chemical and biological releases. Washington (DC): Environmental Protection Agency, EPA 600/R-071/157.
- EPA. 2020. Compliance advisory—EPA regulations about UV lights that claim to kill or be effective against viruses and bacteria. Washington (DC): Environmental Protection Agency. EPA Document # 305F20004. October 2020.
- Ethington T, Newsome S, Waugh J, Lee LD. 2018. Cleaning the air with ultraviolet germicidal irradiation lessened contact infections in a long-term acute care hospital. Am J Infect Control. 46(5):482–486. doi:https://doi.org/10.1016/j.ajic.2017.11.008
- FDA. 2020. Enforcement policy for sterilizers, disinfectant devices, and air purifiers during the coronavirus disease 2019 (COVID-19) public health emergency—guidance for industry and Food and Drug Administration staff. Silver Spring (MD): Food and Drug Administration. March 2020. Doc. No. FDA-2020-D-1138.
- First MW, Nardell EA, Chaisson W, Riley R. 1999. Guidelines for the application of upper-room ultraviolet germicidal irradiation for preventing transmission of airborne contagion—part II: design and operational guidance. ASHRAE Trans. 105:869–876.
- Guimera D, Trzil J, Joyner J, Hysmith ND. 2018. Effectiveness of a shielded ultraviolet C air disinfection system in an inpatient pharmacy of a tertiary care children's hospital. Am J Infect Control. 46(2):223–225. doi:https://doi.org/10.1016/j.ajic.2017.07.026
- Hakim H, Gilliam C, Tang L, Xu J, Lee LD. 2019. Effect of a shielded continuous ultraviolet-C air disinfection device on reduction of air and surface microbial contamination in a pediatric oncology outpatient care unit. Am J Infect Control. 47(10):1248–1254. doi:https://doi.org/10.1016/j.ajic.2019.03.026
- Kane DW, Finley C, Brown D. 2018. UV-C light and infection rate in a long term care ventilator unit. Can J Infect Control. 33(1):44–48.
- Kowalski WJ. 2009. Ultraviolet germicidal irradiation handbook: UVGI for air and surface disinfection. New York (NY): Springer.
- Kramer A, Schwebke I, Kampf G. 2006. How long do nosocomial pathogens persist on inanimate surfaces? A systematic review. BMC Infect Dis Aug. 16(6):130–137.
- Lee BG, Yang JIL, Kim E, Geum SW, Park J-H, Yeo M-K. 2021. Investigation of bacterial and fungal communities in indoor and outdoor air of elementary school classrooms by 16S rRNA gene and ITS region sequencing. Indoor Air. 31(5):1553–1562. doi:https://doi.org/10.1111/ina.12825
- Lee LD. 2020. Surface and air: what impact does UV-C at the room level have on airborne and surface bacteria? Can J Infect Control. 35(4):170–174.
- Marra AR, Schweizer ML, Edmond MB. 2018. No-touch disinfection methods to decrease multidrug-resistant organism infections: a systematic review and meta-analysis. Infect Control Hosp Epidemiol. 39(1):20–31. doi:https://doi.org/10.1017/ice.2017.226
- Menzies D, Popa J, Hanley JA, Rand T, Milton DK. 2003. Effect of ultraviolet germicidal lights installed in office ventilation systems on workers' health and wellbeing: double-blind multiple crossover trials. Lancet. 362(9398):1785–1791. doi:https://doi.org/10.1016/S0140-6736(03)14897-0
- Narayanan SR, Yang S. 2021. Airborne transmission of virus-laden aerosols inside a music classroom: effects of portable purifiers and aerosol injection rates. Phys Fluids. 33(3):033307. doi:https://doi.org/10.1063/5.0042474
- Nardell EA. 2021. Air disinfection for airborne infection control with a focus on COVID-19: why germicidal UV is essential. Photochem Photobiol. 97(3):493–497. doi:https://doi.org/10.1111/php.13421
- Omidbakhsh N. 2010. Theoretical and experimental aspects of microbicidal activities of hard surface disinfectants: are their label claims based on testing under field conditions? J AOAC Int. 93(6):1944–1951. doi:https://doi.org/10.1093/jaoac/93.6.1944
- Paton S, Thompson K-A, Parks SR, Bennett AM. 2015. Reaerosolization of spores from flooring surfaces to assess the risk of dissemination and transmission of infections. Appl Environ Microbiol. 81(15):4914–4919. doi:https://doi.org/10.1128/AEM.00412-15
- Qian H, Miao T, Liu L, Zheng X, Luo D, Li Y. 2021. Indoor transmission of SARS-CoV-2. Indoor Air. 31(3):639–645. doi:https://doi.org/10.1111/ina.12766
- Rosati J, Thornburg J, Rodes C. 2008. Resuspension of particulate matter from carpet due to human activity. Aerosol Sci Technol. 42(6):472–482. doi:https://doi.org/10.1080/02786820802187069
- Rutala WA, Kanamori H, Gergen MF, Knelson LP, Sickbert-Bennett EE, Chen LF, Anderson DJ, Sexton DJ, Weber DJ, the CDC Prevention Epicenters Program. 2018. Enhanced disinfection leads to reduction of microbial contamination and a decrease in patient colonization and infection. Infect Control Hosp Epidemiol. 39(9):1118–1121. doi:https://doi.org/10.1017/ice.2018.165
- van Doremalen N, Bushmaker T, Morris DH, Holbrook MG, Gamble A, Williamson BN, Tamin A, Harcourt JL, Thornburg NJ, Gerber SI, et al. 2020. Aerosol and surface stability of SARS-CoV-2 as compared with SARS-CoV-1. N Engl J Med. 382(16):1564–1567. doi:https://doi.org/10.1056/NEJMc2004973
- Zhang R, Li Y, Zhang AL, Wang Y, Molina MJ. 2020. Identifying airborne transmission as the dominant route for the spread of COVID-19. Proc Natl Acad Sci USA. 117(26):14857–14863. doi:https://doi.org/10.1073/pnas.2009637117