ABSTRACT
We aimed to investigate the beneficial effect of Celastrol on inner ear stem cells and potential therapeutic value for hearing loss. The inner ear stem cells were isolated and characterized from utricular sensory epithelium of adult mice. The stemness was evaluated by sphere formation assay. The relative expressions of Atoh1, MAP-2 and Myosin VI were measured by RT-PCR and immunoblotting. The up-regulation of MAP-2 was also analysed with immunofluorescence. The in vitro neuronal excitability was interrogated by calcium oscillation. The electrophysiological property was determined by inward current recorded on patch clamp. Our results demonstrated that Celastrol treatment significantly improved the viability and proliferation of mouse inner ear stem cells, and facilitated sphere formation. Moreover, Celastrol stimulated differentiation of mouse inner ear stem cells to neuronal-like cells and enhanced neural excitability. Celastrol also enhanced neuronal-like cell identity in the inner ear stem cell derived neurons, as well as their electrophysiological function. Most notably, these effects were apparently associated with the upregulation of Atoh1 in response to Celastrol treatment. Celastrol showed beneficial effect on inner ear stem cells and held therapeutic promise against hearing loss.
INTRODUCTION
Permanent hearing loss is one of the most common auditory disabilities worldwide and imposes severe compromise on health and life quality. The etiology of hearing loss intimately associates with loss and decreased regenerative capacity of mechanosensory hair cells within the sensory epithelium of the cochlea.Citation1 Currently, hearing aids are the most effective treatment for most patients suffering from moderate to severe hearing loss, but its use is very limited in the applicable patient population.Citation2 For those patients diagnosed with profound sensorineural hearing loss, cochlear implant is the sole available therapeutic option with favorable prognosis.Citation3 Therefore, the alternative therapeutics aiming for hearing rehabilitation is under intensive development.
Although the modest regenerative capacity has been observed in rodent vestibular sensory epithelia,Citation4 no hair cell regeneration occurred in adult mammalian cochlea at all. The endeavor to activate the regenerative capacity for medical purpose has been disappointing so far, which partially attributes to highly differentiated and specified cells in the delicate architecture of human auditory system.Citation5 Recently, the cells with high proliferative index and pluripotency has been characterized in mouse vestibular sensory epithelia.Citation6 Moreover, the progenitor population of hair cells has been derived from the cochlea of neonatal rats.Citation7 Despite of the well-characterized pluripotency and stem cell-like property of supporting cells, stimulation of its trans-differentiating potential via medical approach for the therapeutic purpose is still challenging. Likewise, the strategy to enhance the commitment of inner stem cells into hair cells is in urgent need.
Atoh1 (Atonal BHLH Transcription Factor 1) is the member of the basic helix-loop-helix (BHLH) transcription factor family. Through collaboration with TCF3/E47, Atoh1 activates E box-dependent transcription and plays critical roles in differentiation of several subsets of neural cells.Citation8 It was reported that the transcription activity of Atoh1 was readily antagonized by the negative regulator of neurogenesis HES1.Citation9 Loss-of-function of Atoh1 has been identified in the Goblet cell carcinoid and infratentorial cancer.Citation10 More importantly, Atoh1 is the first gene characterized to be involved in inner ear development, and especially plays a fundamental role in hair cell differentiation.Citation11 During inner ear formation, the Atoh1-positive cells are committed to generate sensory hair cells and indispensable for their development, survival, differentiation and maturation. The ectopic introduction of Atoh1 led to formation of supernumerary cochlear sensory hair cells,1Citation2 and aberrant overexpression of Atoh1 stimulated trans-differentiation of supporting cells to hair cells.Citation13 Although investigations into the underlying mechanism have identified Pou4f3, Gfi1 and Myosin 7a as potent downstream effectors,Citation14 the comprehensive understanding of Atoh1 in the biology of auditory system is still to be fulfilled.
Celastrol is a quinone methide triterpene and the effective composition isolated from Thunder God Vine root.Citation15 The traditional Chinese medicine Thunder God Vine has historically been used in treatment of inflammatory and auto-immune diseases such as rheumatoid arthritis.Citation15 The molecular investigations into the pharmacological properties of Celastrol have identified a variety of potential targets, most of which are attributed to the IKK-NF-κB signalling pathway. For example, Celastrol has been shown to directly inhibit the IKKα and β kinases via targeting the Cys-179 in the activation loop of IKKβ.Citation16 High-throughput screening for androgen signalling inhibitors has identified Celastrol in blocking the interactions between HSP90 and co-chaperone Cdc37/p23.Citation17 Yang et al. demonstrated the inhibitory effect of Celastrol on rabbit 20S proteasomes and elicited cell apoptosis in prostate cancer cells.Citation18 In addition, Celastrol treatment triggered heat shock response via inducing HSP70 expression.Citation19 Most importantly, Celastrol has been demonstrated to inhibit aminoglycoside-induced ototoxicity via heat shock protein 32 induction,Citation20 which prompted us to further hypothesize that Celastrol could confer beneficial effect on inner ear stem cells, and have the potential as adjuvant treatment for stem cell therapy against hearing loss.
Here we presented evidences that Celastrol significantly improved inner ear stem cells viability and proliferation. Moreover, Celastrol stimulated neuronal-like cells differentiation and excitability, which might be mediated by up-regulation of the master transcription factor Atoh1. Our results warranted further investigations into the potential therapeutic value of Celastrol in disease model and clinic trial.
MATERIALS AND METHODS
Isolation and culturing of inner ear stem cells
The BALB/C6 mice, aged 4–7 weeks, were purchased from the Shanghai Laboratory Animal Center (SLAC) and maintained in the pathogen-free environment. All mice experiments were conducted in strict accordance with protocol approved by the institutional committee of animal care and use. The sphere-forming and self-renewing inner ear stem cells were isolated from the utricular sensory epithelium of adult BALB/C6 mice following previously established method.Citation21 Briefly, Mice were first euthanized by cervical dislocation, and the utricular maculae were carefully dissected from mice and rinsed with ice-cold PBS buffer. The sensory sheets were isolated by gentle treatment with thermolysin in DMEM/high glucose-F12 mixture (1:1) and followed by trypsin digestion in PBS. The excessive enzymatic activity was quenched by soybean trypsin inhibitor (Worthington). The cells were dispersed by repeated pipetting and resuspended in mixed culture medium (DMEM/high glucose 1:1 F12 with complete supplementation, Gibco, Grand Island, NY, USA). The single-cell suspension was prepared by passing through the 70 μm cell strainer (BD, Franklin Lakes, NJ, USA) and subjected to continuous culture in humidified incubator supplemented with 5% CO2 at 37°C.
MTT assay
Celastrol was first dissolved in DMSO at a stock concentration of 10 mg/mL, which was then diluted in medium at assay specific concentrations. Cells in log phase were seeded into 96-well plates in triplicate for 24 hours culture and subjected to Celastrol treatment for 24 h. MTT (working concentration 0.5 mg/ml) was added into each well and incubated at 37°C for 4 h in the dark. The medium was carefully aspirated and replaced by DMSO (100 μl per well) to resolve crystal. Absorbance at 570 nm was recorded using microplate reader (Molecular Devices, USA) and relative cell viability was calculated.
Proliferation assay with bromodeoxyuridine (BrdU)
The relative proliferative index was evaluated with BrdU incorporation assay using the commercial available kit (Proliferation Assay Kit, Millipore, USA) according to the manufacturer's instructions. Briefly, exponentially growing cells were cultured in the 96-well plates at density of 5,000 cells/well for 24 continuous hours and then subjected to Celastrol treatment for 48 hours, before BrdU was applied to each well for incorporation.
Sphere formation assay
The single cells isolated from utricular sensory epithelium were plated in 10-cm Petri dish at very low density to ensure all the spheres were originated from single cells. Total number of primary spheres was counted 7 days post-culture and portion was collected and dissociated with NeuroCult chemical dissociation kit (StemCell Technologies, Hangzhou, China) for propagative purpose.
Cell differentiation
For cell differentiation purpose, the spheres were first treated with 0.05% trypsin and allowed for adherent growth on poly-L-ornithine/fibronectin (Sigma, St. Louis, MO, USA) coated dishes. The third-generation of utricular maculae-derived sphere cells was stimulated to differentiate with 50 ng/ml NT-3 and 100 ng/ml BDNF (R & D Systems, Minneapolis, MN, USA). The success for differentiation inducing was validated by immunocytochemistry after 14 days.
RT-PCR
The total RNA was extracted from exponentially growing cells with Trizol reagent (Invitrogen, Carlsbad, CA, USA) following the manufacturer's instruction. The quality and quantity was determined by Bioanalyzer 2000 (Agilent, Santa Clara, CA) prior to any further processing. 1 μg RNA was reversely transcribed into cDNA using the PrimeScript First Strand cDNA Synthesis Kit (Clontech). The RT-PCR was performed with SYBR Green Master Kit (Promega, Madison, WI, USA) in accordance with the provider's recommendation. The relative expression of target genes was calculated by 2−ΔΔCt method and normalized to β-actin.
The primers used in this study were listed below:
Atoh1 sense: 5′- ACTTGCCTCATCCGAGTCAC-3′
anti-sense: 5
′- GCAGGAGGAAAACAGCAAAA-3′;
MAP-2 sense: 5′- TTCGTTGTGTCGTGTTCTCA -3′
anti-sense: 5
′- AACCGAGGAAGCATTGATTG -3′;
Myosin VI sense: 5′- CCTCCATTTTTGAAGGAGGA-3′
anti-sense: 5
′- CTTCACCCGTACAGGTAGCC-3′;
β-actin sense: 5′- GTTGTCGACGACGAGCG-3′
anti-sense: 5
′- GCACAGAGCCTCGCCTT-3′;
Western blot
The cell lysates were prepared from indicated cells in log phase in RIPA lysis buffer. The protein concentrations were determined with commercial BCA Protein Assay (ThermoFisher, Waltham, MA, USA). The equal amount of proteins was resolved by SDS-PAGE gel and transferred to PVDF membrane. After brief blocking with 5% skim milk in TBST buffer, the membrane was hybridized with indicated primary antibodies (Atoh1, SAB2100177, Sigma, 1 μg/ml; MAP-2 ab5392, Abcam, 1:500; Myosin VI, ab11098, Abcam, 1:500; β-actin, ab8227, Abcam, 1:1,000) in refrigerator overnight. The unbound antibodies were completely washed off with TBST for 30 min at room temperature. The PVDF membrane was then incubated with respective secondary antibodies (CST#7075, Cell Signaling Technology, 1:5,000) for one hour at room temperature, and followed by the second round of rigorous wash with TBST. The blots were visualized by the Enhanced Chemiluminescence Kit (Millipore, Billerica, MA, USA) following the manufacturer's instruction. The relative intensity of target bands was determined by densitometry scanning and normalized to internal reference protein for loading control.
Immunofluorescence
The exponentially growing cells were cultured onto coverslips for 24 hours prior to the further processing. The culture medium was aspirated and cells were fixed in 4% formaldehyde solution for 15 min at room temperature. The fixed cells were rinsed three times in PBS buffer for 5 min each and blocked with 5% normal serum for 60 min at room temperature. After aspiration of blocking buffer, the coverslips were incubated with indicated primary antibody overnight at 4°C. The cells were rinsed three times with PBS for 5 min each and incubated with fluorochrome-conjugated secondary antibody for one hour at room temperature in the dark. The unbound antibodies were washed off with PBS. The coverslips were mounted with Prolong Gold Antifade Reagent with DAPI overnight at room temperature and sealed with nail polish for long-term preservation.
Electrophysiology
The inner ear stem cell derived neuronal-like cells were plated onto coverslips in the standard artificial perilymph solution (144 mM NaCl, 0.7 mM NaH2PO4, 5.8 mM KCl, 1.3 mM CaCl2, 0.9 mM MgCl2, 5.6 mM D-glucose, 10 mM HEPES-NaOH, pH7.4, 320 mmole/kg osmolality). The recording pipettes were prepared by borosilicate capillary glass with resistance range between 2∼4 MΩ and preserved in pipette solution (135 mM KCl, 4 mM K4-EGTA, 0.1 mM CaCl2, 3 mM Na2ATP, 10 mM HEPES-NaOH, pH7.4). The inward current was recorded at a holding potential of -60 mV and amplified with an Axopatch 700B amplifier (Molecular Devices, Sunnyvale, CA, USA). Data were collected and analyzed with included software.
Calcium assays
The indicated cells were first washed twice with standard solution (150 mM NaCl, 2 mM CaCl2, 5 mM KCl, 1 MgCl2, 10 mM D-glucose, 10 mM HEPES, pH 7.3, and then incubated with Fluo-4-AM (2.5 μM, Dojindo Laboratories) and pluronic F-127 (Sigma, USA) for 45 min at 37°C. The reaction was terminated by replacement with standard solution and incubated for another 30 min. The fluorescence signal was recorded at 510 nm on Carl Zeiss confocal microscope. The oscillation frequency was recorded as the number of spikes per minute.
Statistical analysis
The statistical analysis in this study was performed with SPSS 23.0 software. All data presented in this study were repeated independently at least three times unless otherwise stated. All the results were expressed as Mean + standard deviation (SD). The statistical significances were analyzed by one-way ANOVA, and p < 0.05 was considered statistically different. Sample size of animal experiments was determined using statistical power analysis. Differences between means of each group were divided by the standard deviation to determine the standardized effect size (> 2.0). Using 5% as significance level in Student t-test and 90% power, the minimum required sample size was calculated to be 6.
RESULTS
Celastrol improves viability and proliferation of mouse inner ear stem cells
Celastrol is the effective ingredient from the traditional Chinese herbal medicine. Previous study demonstrated that Celastrol ameliorated the ototoxicity elicited by aminoglycoside, which prompted us to investigate the potential beneficial effect of Celastrol on differentiation of inner ear stem cells and associated molecular mechanism.
In this study, we first isolated the mouse inner ear stem cells following the well-established protocol. The success of in vitro isolation and culturing of the inner stem cells were evaluated by sphere formation assay. We set out to determine the impact on inner ear stem cell growth by Celastrol in vitro. The relative cell viability and proliferation were measured by MTT assay and BrdU incorporation assay, respectively. As shown in , the cell viability was significantly increased by treatment with up to 2 μM Celastrol in a dose-dependent manner. However, high concentration of Celastrol (10 μM) displayed remarkable suppressive effect on cell growth, which might be due to ensued cytotoxicity. Consistent with the influence imposed on cell viability, the incorporation of BrdU was dramatically increased by 2 μM Celastrol treatment, which indicated the pro-proliferative effect of Celastrol (). Our data clearly demonstrated the beneficial effect of Celastrol on growth of inner ear stem cells in vitro. In addition, based on the above dose-dependent results, 2 μM Celastrol was determined to be the optimal concentration, which was therefore chosen as the working concentration in the rest of our experiments.
FIGURE 1. Celastrol improves viability and proliferation of mouse inner ear stem cells in a dose-dependent manner. Cell viability (A) and proliferation (B) of the mouse inner ear stem cells were measured by MTT and BrdU incorporation assay, respectively, after 48 hr treatments of increasing Celastrol doses (0, 1, 2, 5 and 10 μM). Values were shown as mean + SD. *p < 0.05, **p < 0.01, n not significant, compared to 0 μM Celastrol.
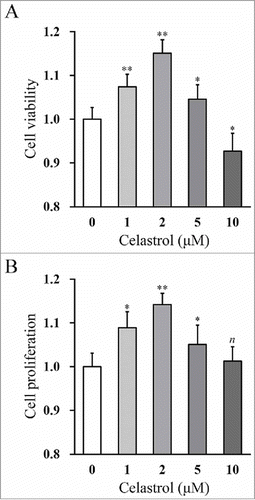
Celastrol improves the capability of sphere formation
Our above data supported the favourable role of Celastrol on inner ear stem cell growth in vitro. Next, we attempted to evaluate the impact of Celastrol on sphere formation capacity of the inner ear stem cells. Both the number and size of the formed spheres were recorded during 2 μM Celastrol treatment, with fresh Celastrol replenished each day to the cell culture. Our results demonstrated that the spheres in Celastrol-treated group were significantly bigger than the ones in control group (). Similarly, the total amount of spheres formed was much higher in Celastrol-treated group (). The representative images were shown in . Our data suggested that, in addition to the pro-growth effect, Celastrol treatment significantly improved the sphere formation capacity of inner ear stem cells.
FIGURE 2. Celastrol improves the capability of sphere formation. Isolated inner ear stem cells were incubated in the presence or absence of 2 μM Celastrol, and sphere diameter (A) and number of spheres/104 cells (B) were measured at indicated time points. Values were shown as mean + SD. *p < 0.05, **p < 0.01, compared to 0 μM Celastrol control.
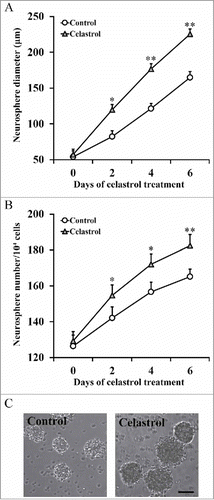
Celastrol upregulates Atoh1 expressions in inner ear stem cells and formed spheres
Atoh1 has been characterized as the master gene in coordinating the sensory hair cell development and regeneration in the cochlea. Therefore, here we sought to investigate further whether Atoh1 was involved in Celastrol-stimulated cell growth and sphere formation of inner ear stem cells.
Both the transcript and protein levels of Atoh1 were determined in primary inner ear stem cell culture and the subsequently formed sphere culture. In comparison to control treatment, Celastrol group showed significant up-regulation of Atoh1 mRNA and even more remarkable increase in the protein level (). Moreover, Celastrol was capable of inducing Atoh1 expression in the subsequently formed spheres (). In agreement with the notion that Atoh1 played critical role in biology of the sensory hair cells, our data showed that Celastrol treatment significantly induced Atoh1 expression at both transcriptional and translational levels.
FIGURE 3. Celastrol upregulates Atoh1 expressions in inner ear stem cells and formed spheres. (A) Atoh1 mRNA and protein expressions in the mouse inner ear stem cells were measured by RT-PCR and Western blot analyses, respectively, in the presence or absence of 2 μM Celastrol. (B) Atoh1 mRNA and protein expressions in the spheres, formed by the inner stem cell culture after 7 days, were measured by RT-PCR and Western blot analyses, respectively, in the presence or absence of 2 μM Celastrol. Values were shown as mean + SD. *p < 0.05, **p < 0.01, compared to 0 μM Celastrol control.
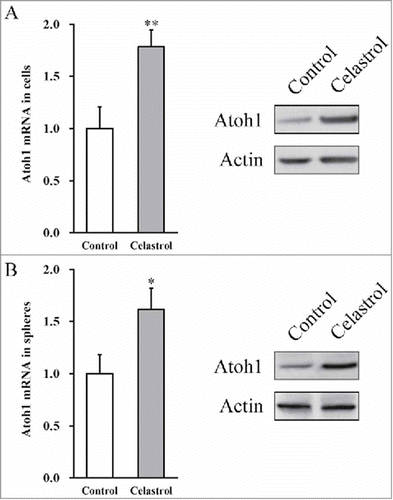
Celastrol improves differentiation of mouse inner ear stem cells to neurons
Our aforementioned results indicated that Celastrol treatment improved cell growth and sphere formation of inner ear stem cells. Along this direction, we next investigated the potential impact of Celastrol on stem cell differentiation, using undifferentiated inner ear stem cells as negative control and hippocampal neurons as positive control. In response to Celastrol treatment, the density of differentiated neurons was significantly higher than both undifferentiated inner ear stem cells and differentiated cells without Celastrol treatment (). We further characterized the expression of neuronal marker MAP-2. As shown in , our RT-PCR and immunoblotting results demonstrated that Celastrol treatment stimulated expression of MAP-2 at both transcriptional and translational levels. The induced MAP-2 was further validated by immunofluorescence assay. As shown in , the representative image displayed more intensive MAP-2 staining signal in Celastrol treated neuronal culture, similar to hippocampal neuronal culture, and much higher than either undifferentiated inner ear stem cells or differentiated cells without Celastrol treatment. Our data suggested that Celastrol prompted inner ear stem cells to differentiate into mature neuronal-like cells.
FIGURE 4. Celastrol improves differentiation of mouse inner ear stem cells to neurons. Inner ear stem cells were subjected to neuronal differentiation for 2 weeks, in the presence (Celastrol) or absence (No Celastrol) of 2 μM Celastrol, using undifferentiated inner ear stem cells (SCs) as negative control, and hippocampal neurons as positive control (HNs). (A) Density of neurons in culture. (B) MAP-2 mRNA and protein expressions in the culture were measured by RT-PCR and Western blot analyses, respectively. Values were shown as mean + SD. *p < 0.05, **p < 0.01, compared to no Celastrol control. # p < 0.05, compared to SCs. (C) Representative fluorescence images of the differentiated neuronal culture, stained for MAP-2 in green and DAPI in blue. Scale bar 200 µm.
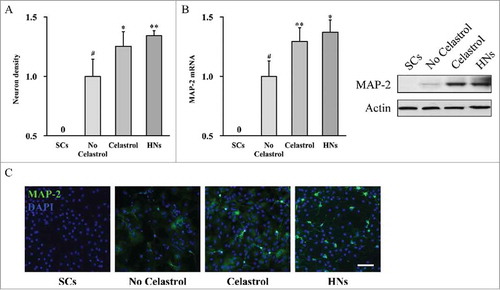
Celastrol enhances calcium fluctuation
The above results showed remarkable pro-differentiating effects of Celastrol on inner ear stem cells. Next, we sought to determine its impact on mature neural excitability, in terms of calcium fluctuation. The intracellular Ca2+ concentration, percentage of the cells with Ca2+ oscillations and spike frequency were measured in response to Celastrol treatment. Celastrol stimulated remarkable fluctuation in intracellular Ca2+ content (). Moreover, approximate 26% neurons showed Ca2+ oscillations in Celastrol treatment group in comparison with 15% in control group (). Simultaneously, the spike frequency was dramatically increased in response to Celastrol treatment (). These results unambiguously demonstrated that Celastrol treatment enhanced calcium fluctuation in our in vitro system.
FIGURE 5. Celastrol enhances calcium fluctuation. Following 2 weeks of neuronal differentiation of mouse inner ear stem cells in the presence or absence of 2 μM Celastrol, changes in Ca2+ concentration (A), % cells with Ca2+ oscillations (B) and spike frequency (C) were measured. Values were shown as mean + SD. **p < 0.01, compared to 0 μM Celastrol control.
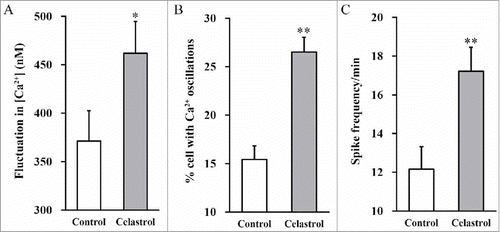
Celastrol enhances neuronal-like cell identity in the inner ear stem cell derived neurons, as well as their electrophysiological function
As mentioned above, Celastrol treatment induced up-regulation of Atoh1 in inner ear stem cells, which has been increasingly recognized to play master role in hair cell differentiation. To explore the potential involvement of Celastrol treatment in induction of neuronal-like cell differentiation, here we sought to interrogate the emergence of neuronal-like cell identity during Celastrol treatment and characterize its physiological functions.
The relative expression of hair cell marker gene myosin VI was determined by both RT-PCR and immunoblotting. As shown in , Celastrol significantly stimulated expression of myosin VI in cultured inner ear stem cells, which indicated the enhance neuronal-like cell identity during Celastrol treatment. In addition, the electrophysiological function of derived neuronal-like cells was determined by in vitro electrophysiological measurement, which was much higher in treatment group in comparison with control group (). Our data indicated that Celastrol stimulated differentiation of inner ear stem cells into neuronal-like cell and their functioning.
FIGURE 6. Celastrol enhances neuronal-like cell identity in the inner ear stem cell derived neurons, as well as their electrophysiological function. (A) Myosin VI mRNA and protein expressions in the culture were measured by RT-PCR and Western blot analyses, respectively. Values were shown as mean + SD. *p < 0.05, **p < 0.01, compared to 0 μM Celastrol control. (B) Voltage-dependent currents were recorded from the derived neuronal-like cells bathed in 1.3 mM Ca2+, in the presence or absence of 2 μM Celastrol.
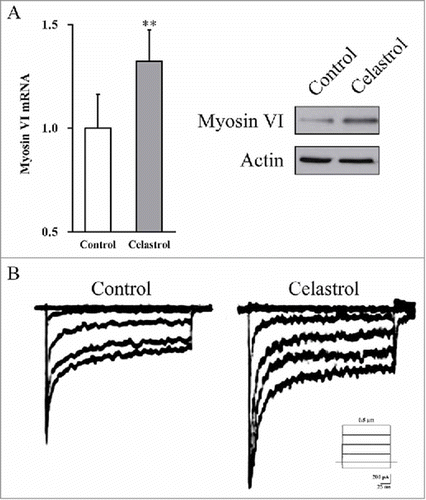
DISCUSSION
Celastrol is the pharmacological component extracted from the traditional Chinese medicine Thunder God Vine, which has been widely used as active ingredient in medicine formula for treatment of variety of inflammatory and auto-immune related human diseases including rheumatoid arthritis. Notably, the anti-ototoxicity property of Celastrol has been revealed recently through induction of heat shock protein 32 to suppress both aminoglycoside- and cisplatin-induced hair cell death.Citation20 Moreover, Celastrol administration significantly rescued the hearing loss in mice receiving systematic aminoglycoside treatment. Mechanistically, Celastrol induced HSP32 expression, which in turn inhibited pro-apoptotic c-Jun N-terminal kinase (JNK) activation and hair cell death.Citation20 In view of the protective effect of Celastrol on aminoglycoside antibiotics and anti-neoplastic agent caused hair cell death,Citation20 here we sought to investigate the potential benefit of Celastrol on inner ear stem cells, which is believed to be permanently dormant in adult mammalian auditory system. In line with the protective effect against ototoxicity, here we demonstrated that 2 μM Celastrol treatment remarkably improved the viability and proliferation of isolated inner ear stem cells in vitro. Other than the sensory hair cells in the previous report, here we focused on utricular sensory epithelium derived stem cells. Our in vitro sphere formation assay clearly showed that Celastrol significantly increased both the size and number of the formed spheres. Until now, the clinical exploitations to activate the regeneration capacity to replace impaired hair cell are frustrating, the ability to preserve and improve the stemness of inner ear stem cells makes Celastrol the highly potent candidate for alternative therapeutics to stimulate neuronal-like cell regeneration.
The hearing function heavily depends on properly differentiated hair cells. In this study, we demonstrated that Celastrol promoted inner ear stem cell differentiation into physiologically functional neurons. More importantly, our data suggested the neuronal-like cell identity, as well as their electrophysiological functioning, was significantly enhanced by Celastrol treatment. In summary, here we revealed a multifaced protective effect of Celastrol on inner ear stem cells in addition to neuronal-like cells, follow-up experiments are needed validate the current findings.
Atoh1 has been identified as the first gene involved in auditory system development.Citation13 Consistent with its critical role in coordinating sensory hair cell development and regeneration in the cochlea,Citation11 here we showed Atoh1 was significantly up-regulated at both mRNA and protein levels in response to Celastrol treatment, which might mechanistically underlie the beneficial effect of Celastrol on inner ear stem cells. Noteworthily, we have not examined thoroughly on the signaling pathways related to Atoh1 activation, since intensive researches have been invested into this subject. For instance, the Notch signaling has been reported to produce inhibitory bHLH proteins (HES1 and HES5) that blocked the effect of Atoh1 and led to inhibition of the hair cell fate.Citation9 Upon activation of fibroblast growth factor (FGF) signaling by FGF2, several neurotropic factors such as NGF, BDNF and NT-3 could significantly increase Atoh1 expression, which suggested that FGF signaling played a fundamental role in modulating the specification of progenitor cells and their subsequent differentiation.Citation9 Accumulating evidences suggest that Wnt/β-catenin signaling is indispensable for pro-sensory cell proliferation as well as sensory hair cell differentiation.Citation22 Through direct interaction with and Atoh1 3′ enhancer, β-catenin stimulated Atoh1 expression in neural progenitor cells.Citation23
The essential step in cochlea development is the commitment of pro-sensory cells to hair cell identity. Assembling evidences support the hypothesis that Atoh1 is the determinant factor that drive stem cell differentiation and development. All the auditory and vestibular hair cells were absent in Atoh1 deficient mice,2Citation4 and ectopic re-introduction of wild-type Atoh1 restored the hair cell fate within both the pro-sensory domain and non-sensory regions of the cochlea.Citation25 In line with these observations, our data demonstrated that Celastrol enhanced neuronal-like cell identity in the inner ear stem cell population as well as their electrophysiological function, which was highly likely to be mediated by the up-regulation of Atoh1. In addition to preservation and stimulation of the regeneration capacity, here we provided evidences that Celastrol treatment also enhanced the differentiation of inner ear stem cells into neuronal-like cells, and their electrophysiological functioning.
Although we have characterized up-regulation of Atoh1 in inner ear stem cells in response to Celastrol treatment, the detailed molecular mechanism underlying this modulation is still to be elucidated. Previous study suggested that Atoh1 was subjected to ubiquitin-proteasome degradation via E3 ligase Huwe1 and Casein kinase 1 during normal sensory hair cell development,Citation26 which was protected by Shh signaling in neural precursors.Citation27 On the other hand, accumulating evidences indicated that Celastrol possessed inhibitory effect on proteasomal activity. For example, Celastrol has been demonstrated to suppress androgen-independent prostate cancer progression by modulating apoptotic proteins and NF-κB pathway via proteasome inhibition.Citation28 Walcott SE et al. reported that Celastrol could inhibit proteasome activity and up-regulate the expression of heat shock protein genes including Hsp30 and Hsp70 in Xenopus laevis A6 cells.Citation29 Yang et al. suggested that Celastrol suppressed human prostate cancer growth in nude mice model via potent proteasome inhibition.Citation18 It is therefore reasonable to speculate that Celastrol-induced Atoh1 expression could be mediated by its potent proteasome inhibitory effect, however, this hypothesis requires further experimental verification.
In summary, here we demonstrated that Celastrol treatment stimulated expression of the master transcription factor Atoh1 in inner ear stem cells and subsequently derived spheres. Moreover, Celastrol facilitated the commitment of inner ear stem cells to neuronal-like cells, which might hold great therapeutic promise for further exploitations.
CONFLICTS OF INTEREST
The authors declare that they have no conflict of interest.
ACKNOWLEDGMENTS
None.
Additional information
Funding
REFERENCES
- Korver AM, Smith RJ, Van Camp G, Schleiss MR, Bitner-Glindzicz MA, Lustig LR, Usami SI, Boudewyns AN. Congenital hearing loss. Nat Rev Dis Primers. 2017;3:16094. doi:10.1038/nrdp.2016.94. PMID:28079113.
- Tharpe AM, Gustafson S. Management of children with mild, moderate, and moderately severe sensorineural hearing loss. Otolaryngol Clin North Am. 2015;48:983–94. doi:10.1016/j.otc.2015.07.005. PMID:26433444.
- Liu JF, Dai JS, Wang NY. [Effect of cochlear implantation on sound localization for patients with unilateral sensorineural hearing loss]. Zhonghua Er Bi Yan Hou Tou Jing Wai Ke Za Zhi. 2016;51:623–30. PMID:27625137.
- Warchol ME, Lambert PR, Goldstein BJ, Forge A, Corwin JT. Regenerative proliferation in inner ear sensory epithelia from adult guinea pigs and humans. Science. 1993;259:1619–22. doi:10.1126/science.8456285. PMID:8456285.
- Forge A, Wright T. The molecular architecture of the inner ear. Br Med Bull. 2002;63:5–24. doi:10.1093/bmb/63.1.5. PMID:12324381.
- Li H, Liu H, Heller S. Pluripotent stem cells from the adult mouse inner ear. Nat Med. 2003;9:1293–9. doi:10.1038/nm925. PMID:12949502.
- Zhai S, Shi L, Wang BE, Zheng G, Song W, Hu Y, Gao WQ. Isolation and culture of hair cell progenitors from postnatal rat cochleae. J Neurobiol. 2005;65:282–93. doi:10.1002/neu.20190. PMID:16155904.
- Masuda M, Pak K, Chavez E, Ryan AF. TFE2 and GATA3 enhance induction of POU4F3 and myosin VIIa positive cells in nonsensory cochlear epithelium by ATOH1. Dev Biol. 2012;372:68–80. doi:10.1016/j.ydbio.2012.09.002. PMID:22985730.
- Su YX, Hou CC, Yang WX. Control of hair cell development by molecular pathways involving Atoh1, Hes1 and Hes5. Gene. 2015;558:6–24. doi:10.1016/j.gene.2014.12.054. PMID:25550047.
- Flora A, Klisch TJ, Schuster G, Zoghbi HY. Deletion of Atoh1 disrupts Sonic Hedgehog signaling in the developing cerebellum and prevents medulloblastoma. Science. 2009;326:1424–7. doi:10.1126/science.1181453. PMID:19965762.
- Izumikawa M, Minoda R, Kawamoto K, Abrashkin KA, Swiderski DL, Dolan DF, Brough DE, Raphael Y. Auditory hair cell replacement and hearing improvement by Atoh1 gene therapy in deaf mammals. Nat Med. 2005;11:271–6. doi:10.1038/nm1193. PMID:15711559.
- Gubbels SP, Woessner DW, Mitchell JC, Ricci AJ, Brigande JV. Functional auditory hair cells produced in the mammalian cochlea by in utero gene transfer. Nature. 2008;455:537–41. doi:10.1038/nature07265. PMID:18754012.
- Kelly MC, Chang Q, Pan A, Lin X, Chen P. Atoh1 directs the formation of sensory mosaics and induces cell proliferation in the postnatal mammalian cochlea in vivo. J Neurosci. 2012;32:6699–710. doi:10.1523/JNEUROSCI.5420-11.2012. PMID:22573692.
- Costa A, Henrique D. Transcriptome profiling of induced hair cells (iHCs) generated by combined expression of Gfi1, Pou4f3 and Atoh1 during embryonic stem cell differentiation. Genom Data. 2015;6:77–80. doi:10.1016/j.gdata.2015.08.017. PMID:26697340.
- Lipsky PE, Tao XL. A potential new treatment for rheumatoid arthritis: thunder god vine. Semin Arthritis Rheum. 1997;26:713–23. doi:10.1016/S0049-0172(97)80040-6. PMID:9144848.
- Lee JH, Koo TH, Yoon H, Jung HS, Jin HZ, Lee K, Hong YS, Lee JJ. Inhibition of NF-kappa B activation through targeting I kappa B kinase by celastrol, a quinone methide triterpenoid. Biochem Pharmacol. 2006;72:1311–21. doi:10.1016/j.bcp.2006.08.014. PMID:16984800.
- Hieronymus H, Lamb J, Ross KN, Peng XP, Clement C, Rodina A, Nieto M, Du J, Stegmaier K, Raj SM, et al. Gene expression signature-based chemical genomic prediction identifies a novel class of HSP90 pathway modulators. Cancer Cell. 2006;10:321–30. doi:10.1016/j.ccr.2006.09.005. PMID:17010675.
- Yang H, Chen D, Cui QC, Yuan X, Dou QP. Celastrol, a triterpene extracted from the Chinese "Thunder of God Vine," is a potent proteasome inhibitor and suppresses human prostate cancer growth in nude mice. Cancer Res. 2006;66:4758–65. doi:10.1158/0008-5472.CAN-05-4529. PMID:16651429.
- Westerheide SD, Bosman JD, Mbadugha BN, Kawahara TL, Matsumoto G, Kim S, Gu W, Devlin JP, Silverman RB, Morimoto RI. Celastrols as inducers of the heat shock response and cytoprotection. J Biol Chem. 2004;279:56053–60. doi:10.1074/jbc.M409267200. PMID:15509580.
- Francis SP, Kramarenko, II, Brandon CS, Lee FS, Baker TG, Cunningham LL. Celastrol inhibits aminoglycoside-induced ototoxicity via heat shock protein 32. Cell Death Dis. 2011;2:e195. doi:10.1038/cddis.2011.76. PMID:21866174.
- Oshima K, Senn P, Heller S. Isolation of sphere-forming stem cells from the mouse inner ear. Methods Mol Biol. 2009;493:141–62. doi:10.1007/978-1-59745-523-7_9. PMID:18839346.
- Kuo BR, Baldwin EM, Layman WS, Taketo MM, Zuo J. In vivo cochlear hair cell generation and survival by coactivation of beta-catenin and atoh1. J Neurosci. 2015;35:10786–98. doi:10.1523/JNEUROSCI.0967-15.2015. PMID:26224861.
- Shi F, Cheng YF, Wang XL, Edge AS. Beta-catenin up-regulates Atoh1 expression in neural progenitor cells by interaction with an Atoh1 3' enhancer. J Biol Chem. 2010;285:392–400. doi:10.1074/jbc.M109.059055. PMID:19864427.
- Woods C, Montcouquiol M, Kelley MW. Math1 regulates development of the sensory epithelium in the mammalian cochlea. Nat Neurosci. 2004;7:1310–8. doi:10.1038/nn1349. PMID:15543141.
- Jones JM, Montcouquiol M, Dabdoub A, Woods C, Kelley MW. Inhibitors of differentiation and DNA binding (Ids) regulate Math1 and hair cell formation during the development of the organ of Corti. J Neurosci. 2006;26:550–8. doi:10.1523/JNEUROSCI.3859-05.2006. PMID:16407553.
- Cheng YF, Tong M, Edge AS. Destabilization of atoh1 by E3 ubiquitin ligase huwe1 and casein kinase 1 Is essential for normal sensory hair cell development. J Biol Chem. 2016;291:21096–109. doi:10.1074/jbc.M116.722124. PMID:27542412.
- Forget A, Bihannic L, Cigna SM, Lefevre C, Remke M, Barnat M, Dodier S, Shirvani H, Mercier A, Mensah A, et al. Shh signaling protects Atoh1 from degradation mediated by the E3 ubiquitin ligase Huwe1 in neural precursors. Dev Cell. 2014;29:649–61. doi:10.1016/j.devcel.2014.05.014. PMID:24960692.
- Dai Y, Desano J, Tang W, Meng X, Meng Y, Burstein E, Lawrence TS, Xu L, et al. Natural proteasome inhibitor celastrol suppresses androgen-independent prostate cancer progression by modulating apoptotic proteins and NF-kappaB. PLoS One. 2010;5:e14153. doi:10.1371/journal.pone.0014153. PMID:21170316.
- Walcott SE, Heikkila JJ. Celastrol can inhibit proteasome activity and upregulate the expression of heat shock protein genes, hsp30 and hsp70, in Xenopus laevis A6 cells. Comp Biochem Physiol A Mol Integr Physiol. 2010;156:285–93. doi:10.1016/j.cbpa.2010.02.015. PMID:20188206.