ABSTRACT
Singlet oxygen is generated by bacteriochlorophylls when light and oxygen are simultaneously present in Rhodobacter sphaeroides. Singlet oxygen triggers a specific response that is partly regulated by the alternative sigma factor RpoHI/HII. The sRNA RSs2461 has previously been identified as an RpoHI/HII-dependent sRNA and is derived from the 3′ UTR of the mRNA for an OmpR-type transcriptional regulator. Similar to the RpoHI/HII-dependent CcsR and SorY sRNAs, RSs2461 affects the resistance of R. sphaeroides against singlet oxygen and was therefore renamed here SorX. Furthermore, SorX has a strong impact on resistance against organic hydroperoxides that usually occur as secondary damages downstream of singlet oxygen. The 75-nt SorX 3′ fragment, which is generated by RNase E cleavage and highly conserved among related species, represents the functional entity. A target search identified potA mRNA, which encodes a subunit of a polyamine transporter, as a direct SorX target and stress resistance via SorX could be linked to potA. The PotABCD transporter is an uptake system for spermidine in E. coli. While spermidine is generally described as beneficial during oxidative stress, we observed significantly increased sensitivity of R. sphaeroides to organic hydroperoxides in the presence of spermidine. We therefore propose that the diminished import of spermidine, due to down-regulation of potA by SorX, counteracts oxidative stress. Together with results from other studies this underlines the importance of regulated transport to bacterial stress defense.
Abbreviations
aSD | = | anti-Shine-Dalgarno |
ATP | = | adenosine triphosphate |
BSA | = | bovine serum albumin |
C1 | = | one carbon |
EDTA | = | ethylenediaminetetraacetic acid |
GSH | = | glutathione |
mRNA | = | messenger ribonucleic acid |
NADH | = | nicotinamide adenine dinucleotide |
rRNA | = | ribosomal ribonucleic acid |
SD | = | Shine-Dalgarno |
SDS | = | sodium dodecyl sulfate |
sRNA | = | small ribonucleic acid |
tBOOH | = | tert-butyl hydroperoxide |
UTR | = | untranslated region |
Introduction
Most bacteria encounter changing conditions in their natural habitats and need to adapt to these changes. Since conditions are rarely optimal for growth and can be even harmful to the cells this adaptation often requires timely stress responses. Past investigations unraveled complex regulatory networks controlling bacterial stress responses that involve protein as well as RNA regulators.
The Alphaproteobacterium Rhodobacter sphaeroides has the ability to form photosynthetic complexes and to use light energy for the production of ATP by anoxygenic photosynthesis. However, the simultaneous presence of bacteriochlorophyll, light and oxygen leads to the generation of the harmful singlet oxygen. To avoid photooxidative stress the formation of photosynthetic complexes is strictly regulated by oxygen and light signals.Citation1 In addition R. sphaeroides mounts a protective response in the presence of singlet oxygenCitation2 which has been intensively studied in the past.Citation3,4 Transcriptome and proteome studies identified several mRNAs and proteins with increased abundance upon singlet oxygen stressCitation5,6 and the sigma factor RpoE was recognized as a main regulator of this response.Citation5,7,8 RpoE is inactivated by binding to its cognate anti-sigma factor ChrR under non-stress conditions.Citation9 Upon singlet oxygen stress ChrR is degraded by proteases homologous to DegS and RsePCitation10 rendering RpoE available to RNA polymerase. Among the few genes which are directly activated by RpoE is the gene for another alternative sigma factor, RpoHII, which in turn activates a much larger subset of genes.Citation11 The RpoHII regulon overlaps with the regulon of RpoHI that has a major role in the heat shock response.Citation12,13 The singlet oxygen stress response does not only induce many mRNAs but also several sRNAs in R. sphaeroides.Citation14 The RNA chaperon Hfq, which has an important role in sRNA-mRNA interactionCitation15 was shown to bind to several of those sRNAs and to contribute to photooxidative stress resistance.Citation16 To date 2 of the R. sphaeroides sRNAs transcribed from RpoHI/HII promoters and induced by singlet oxygen and other stresses have been investigated in more detail: SorY interacts with the takP mRNA for a TRAP-T transporter. The data supported a model in which SorY reduces the metabolic flux into the tricarboxylic acid cycle by reducing malate import through TakP.Citation17 It thus contributes to a metabolic switch, which reduces the production of the pro-oxidant NADH in favor of the production of the anti-oxidant NADPH. The RpoHI/HII-dependent, singlet oxygen induced sRNA CcsR is present in a repeat of 4 copies.Citation18 It directly interacts with the mRNA for the transcriptional regulator FlhR and as a consequence modulates the C1 metabolism and subsequently the level of the anti-oxidant glutathione (GSH). Furthermore it affects mRNAs for the pyruvate dehydrogenase complex.
In this study we address the function of the singlet oxygen induced sRNA RSs2461. It is co-transcribed with an upstream gene encoding an OmpR-type transcriptional regulator from an RpoHI/HII-dependent promoter Citation12,14 and interacts with the RNA chaperone Hfq.Citation16 Our data demonstrate a role of RSs2461 in the defense against singlet oxygen and it was therefore renamed here SorX (for singlet oxygen resistance RNA X). Reaction of singlet oxygen with proteins, lipids and photopigments results in both direct damage and the formation of long-lived reactive organic peroxides.Citation19 Notably, SorX has a strong impact on resistance against organic hydroperoxides, such as tert-butyl hydroperoxide (tBOOH). The 116-nt pre-SorX transcript is processed by RNase E into the fully functional 75-nt SorX small RNA that is highly conserved among related species within the Rhodobacteraceae family. The impact of SorX on stress resistance in R. sphaeroides could be linked to the polyamine transporter gene potA which was shown to be directly targeted at its Shine-Dalgarno (SD) sequence by an anti-Shine-Dalgarno (aSD) sequence located in the SorX 3′ fragment. SorX therefore represents the second sRNA in R. sphaeroides that counteracts oxidative stress by targeting the mRNA for a transporter.
Results
The conserved 3′ fragment of SorX is generated by RNase E cleavage
SorX was originally identified as RSs2461, an sRNA that is induced by singlet oxygen stress.Citation14 RSs2461 was renamed here SorX (for singlet oxygen resistance RNA X) due to its effect on singlet oxygen resistance (see below). SorX is transcribed together with the upstream gene RSP_0847 from an RpoHI/HII promoterCitation12 and the 116-nt long pre-SorX transcript is further processed into a more abundant 75-nt 3′ fragment ().Citation14 The 75-nt fragment is predicted to have 2 conserved stem-loops and harbors a putative aSD sequence in the single-stranded region between the stem-loops (). Total RNA was treated with tobacco acid pyrophosphatase (TAP) to distinguish between 5′-monophosphates (5′-P) and 5′-triphosphates (5′-PPP) using 5′ RACE. Adapters for RT-PCR can only be ligated to processed transcripts (contain 5′-P), while primary transcripts (contain 5′-PPP) have to be treated with TAP to gain 5′-P. Both pre-SorX and SorX were amplified without TAP treatment, suggesting that both are processed transcripts (). As a control we amplified the sRNA RSs0682, which is known to be produced from an upstream promoter and to undergo further processing. By expressing a temperature-sensitive RNase E variant from E. coliCitation20,21 in R. sphaeroides, we could show that the 75-nt 3′ fragment is not detectable at the non-permissive temperature (). The 116-nt pre-SorX transcript in contrast accumulates under these conditions. This argues for an RNase E-dependent generation of the 75-nt 3′ fragment. A homology search for SorX in species closely related to R. sphaeroides revealed that the SorX 3′ fragment is highly conserved in the Rhodobacteraceae family ().Citation14 These data indicate that the small fragment might represent the functional entity in a variety of species. It was predicted that SorX has an upstream FnrL binding site partly internal to RSP_0847 based on ChIP-seq experiments.Citation22 Even though an FnrL-dependent induction of SorX was observed in an oxygen-shift experiment (Fig. S1), neither pre-SorX nor SorX could be detected on Northern blots when the RSP_0847-sorX locus bared its RpoHI/HII promoter (data not shown). This argues against the existence of an internal promoter, which is able to drive strong transcription of SorX.
Figure 1. The SorX 3′ fragment is generated by RNase E cleavage and conserved among Rhodobacteraceae. (A) The genetic context of the sorX gene in R. sphaeroides 2.4.1. SorX is transcribed together with the upstream gene RSP_0847 from an RpoHI/HII promoter and the 116-nt long pre-SorX is further processed into a more abundant 75-nt SorX fragment. The lollipop indicates a Rho-independent terminator. (B) Secondary structure prediction of SorX using RNAfold from the ViennaRNA web server.Citation58 (C) 5′ RACE analysis of SorX. Total RNA, treated with TAP (+) or untreated (−), was subjected to 5′-adapter ligation and RT-PCR, using one gene-specific and one adapter-specific primer. As a negative control, the RT step was omitted (-RT). The pre-SorX and SorX transcripts produce RT-PCR products of 115 (asterisk) and 74 bp, respectively. RT-PCR products were analyzed on 10% polyacrylamide gels. (D) Northern blot analysis of SorX in R. sphaeroides wild type 2.4.1 and strain 2.4.1rneE.c.(ts) that expresses a temperature-sensitive RNase E variant (rne-3071ts mutation) from E. coli. The strains were analyzed at different temperatures (32°C to 42°C). The upper band is an unspecific signal (see Fig. S2). 5.8S rRNA signals are displayed as a loading control. (E) Synteny analysis of the sorX locus within the Rhodobacteraceae family. Light gray arrows represent the gene for a 2-component transcriptional regulator that is present upstream of sorX. The dashed line indicates the RNase E-dependent processing site of SorX in R. sphaeroides 2.4.1. The gene for an Exodeoxyribonuclease III (dark gray arrows) can be found downstream of sorX in several cases.
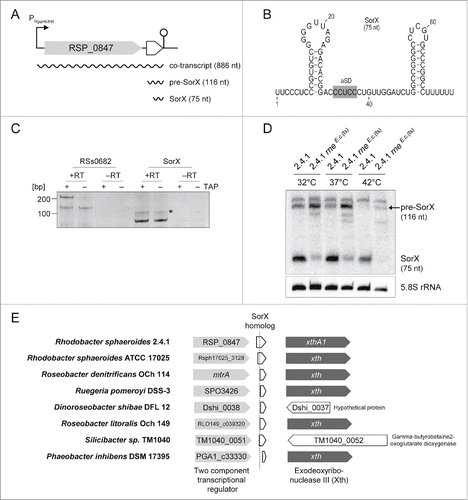
SorX is induced by various stresses and affects the resistance to singlet oxygen and organic hydroperoxides
The RSP_0847 gene product encodes a putative OmpR-type transcriptional regulator, which is strongly induced by singlet oxygen.Citation5 Previous work revealed that RpoHI/HII-dependent genes also respond to other stresses like hydrogen peroxide, superoxide, organic peroxides, cadmium chloride, and heat stress.Citation18 shows moderate induction of the 75-nt SorX fragment by singlet oxygen, organic hydroperoxides (tBOOH), heat stress, and diamide. In contrast, superoxide, hydrogen peroxide, and cadmium chloride caused a negligible increase of SorX. There was no effect by SDS/EDTA treatment.
Figure 2. Various stress conditions induce SorX expression. R. sphaeroides wild type 2.4.1 was grown under aerobic conditions to apply singlet oxygen (1O2), superoxide (O2–), organic hydroperoxide (tBOOH), and hydrogen peroxide (H2O2) stress. Growth under microaerobic condition was conducted to induce heat stress (42°C) or to treat the cells with CdCl2, SDS/EDTA, and diamide. Total RNA isolated at the indicated time points was analyzed on Northern blots. Results for the 75-nt SorX 3′ fragment are shown. Band intensities for SorX were normalized to the 5S rRNA. Fold changes and standard deviations were calculated from biological triplicates.
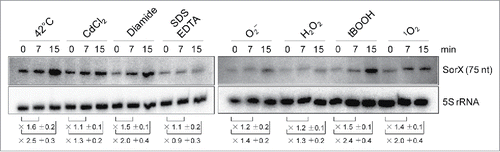
In order to elucidate the role of SorX in stress responses of R. sphaeroides, a strain lacking this sRNA (2.4.1ΔSorX) and a strain, which overexpresses full-length SorX from a strong and constitutive 16S rRNA promoter (2.4.1pRKSorX144), were constructed (Fig. S2). Deletion of SorX as well as overexpression resulted in increased doubling time (5 h ± 1.1 h and 4.1 h ± 0.3 h, respectively) compared to the wild type (3.1 h ± 0.1 h) under aerobic conditions. Interestingly, the SorX deletion strain showed significantly higher sensitivity to tert-butyl hydroperoxide (tBOOH) and singlet oxygen stress compared to the wild type, while overexpression resulted in increased resistance (). This was true for overexpression of full-length SorX as well as for overexpression of a 76-nt 3′ fragment of SorX ().
Figure 3. Effects of SorX deletion or overexpression on resistance to singlet oxygen and organic hydroperoxides monitored by zone inhibition assays. Zones of inhibition for each strain were calculated relative to their respective control strain (100%). 1 M tBOOH or 50 mM methylene blue with light were used to generate organic hydroperoxide or singlet oxygen stress, respectively. (A) SorX deletion strain (2.4.1ΔSorXpRK4352), SorX overexpression strain (2.4.1pRKSorX144) and complemented SorX deletion strain (2.4.1ΔSorXpRKSorX144) were compared to wild type 2.4.1 carrying an empty vector control (2.4.1pRK4352). (B) The SorX deletion was complemented either with full-length SorX (2.4.1ΔSorXpBBRSorX144) or the 76-nt 3′ part of SorX (2.4.1ΔSorXpBBRSorX76). The corresponding strains were compared to wild type 2.4.1 (2.4.1pBBR4352) and the SorX deletion strain (2.4.1ΔSorXpBBR4352), each carrying an empty vector control. The error bars indicate the standard deviation from the mean of biological triplicates.
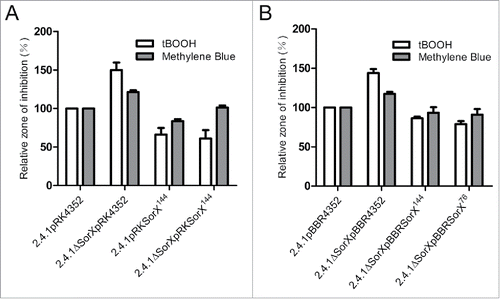
Effect of SorX on global gene expression
We compared the singlet oxygen transcriptomes of the SorX overexpression strain 2.4.1pRKSorX144 and control strain 2.4.1pRK415 by microarray analysis to identify RNAs that are regulated by SorX. In total, 37 mRNAs were identified as differentially regulated (log2 ratios ≥ 0.6 or ≤ −0.6; p-value < 0.05; ). Albeit not statistically significant, we found the CcsR and SorY sRNAs to be induced upon SorX overexpression (). In addition, many differentially regulated mRNAs in the SorX microarray were previously identified as CcsR or SorY targets ().Citation17,18 This motivated us to test whether SorX has an influence on CcsR and SorY expression. Northern blot analysis with the SorX overexpression strain verified the microarray results for CcsR1 and SorY (). Most strikingly, both sRNAs could barely be detected in a SorX deletion mutant (). On the contrary, SorX was clearly detectable and even slightly induced in a SorY deletion mutant (Fig. S3). The CcsR sRNAs are known to have a pronounced impact on cellular GSH levelsCitation18 and we therefore assumed that SorX might impact GSH through CcsR. Since a CcsR deletion strain is not available, it is difficult to prove this hypothesis directly. However, overexpression as well as deletion of SorX had a significant effect on the cellular GSH levels (), which might be a consequence of CcsR regulation. We reasoned that RpoHI/HII-dependent transcription of CcsR and SorY is influenced by SorX. We therefore constructed transcriptional reporter gene fusions to both RpoHI/HII promoters. This experiment clearly validated a positive effect of SorX on expression from these promoters (). The underlying mechanisms however need further elucidation.
Figure 4. SorX affects transcription of 2 other sRNAs. (A) Analysis of the SorX overexpression strain 2.4.1pRKSorX144 by microarrays. The Volcano plot depicts log2 ratios and p-values (negative log10) for the analyzed genes. Vertical lines indicate log2 ratios of ≥ 0.6 and ≤ −0.6. The horizontal line represents a p-value threshold of 0.05. Genes discussed in this study are marked. (B) Expression of CcsR1 and SorY depends on SorX. Total RNA was isolated from each strain before and 7 min after adding 100 mM tBOOH and used for Northern blot analysis. 5.8S rRNA was hybridized as a loading control. (C) Effect of SorX on intracellular glutathione (GSH) level. The error bars indicate the standard deviation from the mean of 2 biological replicates with 3 technical replicates. Asterisks indicate a statistically significant change (p ≤ 0.05) compared to the wild type harboring empty plasmid pRK4352 as control. (D, E) Effect of SorX on the promoter activity of RSP_6037 (D) and SorY (E). The promoter regions were transcriptionally fused to ecfp to determine promoter activities. Fluorescence intensities were calculated relative to wild type 2.4.1 carrying the empty vector pRK4352 (set to 100%). The error bars indicate the standard deviation from the mean of biological triplicates with 3 technical replicates.
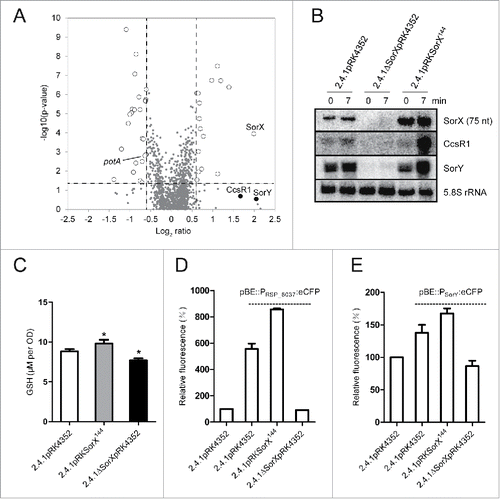
Table 1. Differentially regulated genes after SorX overexpression.
We compared the transcriptome data to proteome data that have been generated for the overexpression strain 2.4.1pRKSorX116 and control strain 2.4.1pRK415 using 2D gel electrophoresis. Labeling of proteins with L-[35S]-methionine allows to relatively quantify protein synthesis rather than absolute protein levels. The periplasmic substrate-binding protein of a putative polyamine transporter (encoded by potD or RSP_1883) was decreased in synthesis when SorX was overexpressed (Fig. S4). Interestingly, potA (RSP_1882) showed decreased mRNA levels upon overexpression of SorX in the microarray data (). In E. coli the pot genes form an operon and dRNA-seq data indicate that potA (RSP_1882), potD (RSP_1883), potB (RSP_1884), and potC (RSP_1886) are co-transcribed in R. sphaeroides (data not shown). Since an interaction of SorX with potA was predicted by IntaRNA (tool for prediction of RNA-RNA interactions),Citation23 we considered potA as a putative direct target of SorX.
SorX targets the potA mRNA within the Shine-Dalgarno sequence
In the wild-type strain we observed a log2 ratio of −1.7 for the potA mRNA 7 min after addition of tBOOH (). The SorX deletion strain showed however slightly increased potA mRNA level under the same condition. Furthermore, the level of potA mRNA was higher in the SorX deletion strain than in the wild type after tBOOH treatment (). This confirmed an effect of SorX on potA mRNA levels in the presence of organic hydroperoxides, a situation where SorX-mediated resistance was most pronounced. A similar observation was made for potD (RSP_1883) (Fig. S5). To test for a direct interaction between SorX and potA mRNA, we applied a lacZ-based in vivo reporter system previously established for Alphaproteobacteria.Citation24 A potA fragment, which contains the predicted interaction site () and the first 8 codons, was translationally fused to the lacZ gene on plasmid pPHU4352 to generate the reporter plasmid pPHUpotA. The ß-galactosidase activity measured in a SorX deletion strain harboring the control vector pBBR4352 in addition to the reporter plasmid was set to 100%. When the overexpression plasmid pBBRSorX144 was present, the ß-galactosidase activity dropped to ∼50% (). The same was true when the 76-nt SorX fragment was overexpressed (), indicating that the small SorX fragment has the ability to target potA mRNA. Overexpression of 76-nt SorX had no effect in a strain lacking the RNA chaperon Hfq (), indicating that Hfq is required for SorX-potA interaction. Overexpression of SorX had no effect on a potD-lacZ reporter, suggesting that the effect of SorX on potD is indirect through base pairing with potA (Fig. S5). When three nucleotides in the proposed seed region of SorX were exchanged to weaken the predicted interaction with potA, there was only a slight effect of SorX overexpression on the activity of the potA-lacZ fusion (). When compensatory mutations were introduced into potA, overexpression of mutated SorX resulted in a strong reduction of ß-galactosidase activity, while no effect was observed when the mutated potA sequence was present together with wild-type SorX ().
Figure 5. SorX affects mRNA level and stability of potA. (A) Effect of SorX on potA mRNA levels as quantified by real time RT-PCR. Total RNA was isolated from wild type 2.4.1 and the SorX deletion strain (2.4.1ΔSorX) before and 7 min after adding 100 mM tBOOH. The relative transcript level (RTL) is given as log2 fold change. (B) Effect of SorX on potA mRNA stability. The levels of mRNA at different time points after addition of rifampicin were quantified by real time RT-PCR (expression values were normalized to 16S rRNA) and the half-life was calculated. The error bars indicate the standard deviation from the mean of biological triplicates with 2 technical replicates.
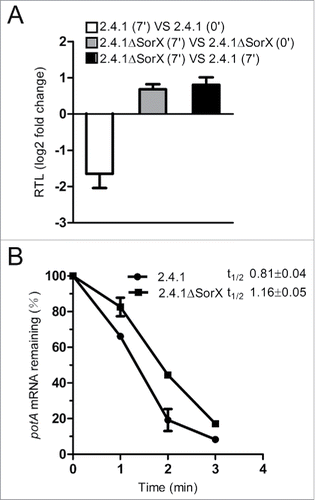
Figure 6. Direct interaction between SorX and potA mRNA. (A) Interaction of SorX-potA as predicted by IntaRNA. Position +1 of the mRNA represents the translational start site. The seed region in potA is from −21 to −5 with respect to the start codon. A triple mutation (M3) in SorX (CUC → GAG) and a compensatory mutation in potA (GAG → CUC) are marked by asterisks. (B) Relative ß-galactosidase activities for lacZ fusions with wild-type potA or mutated (M3) potA. Wild-type SorX or mutated SorX were overexpressed in the SorX deletion background (2.4.1ΔSorXpBBRSorX144, 2.4.1ΔSorXpBBRSorX144-M3). A SorX deletion strain harboring the empty vector pBBR4352 served as a control. ß-galactosidase activities were calculated relative to the corresponding control (set to 100%). (C, D) Relative ß-galactosidase activities for a lacZ fusion with potA. Full-length SorX (2.4.1ΔSorXpBBRSorX144) and the 76-nt SorX 3′ fragment (2.4.1ΔSorXpBBRSorX76) were overexpressed in the SorX deletion strain (C) or in an hfq deletion strain (D). ß-galactosidase activities in an empty vector control strain were set to 100%. The error bars indicate the standard deviation from the mean of biological triplicates with 2 technical replicates.
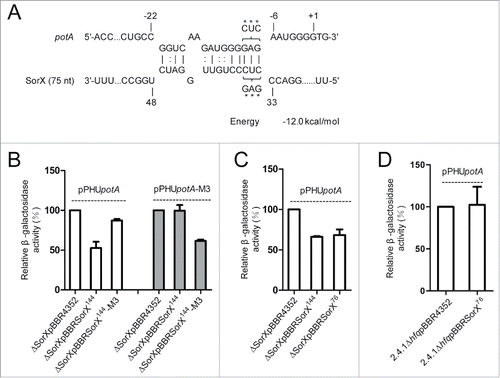
We provide very strong evidence for a direct interaction of an anti-Shine-Dalgarno (aSD) sequence in SorX with the Shine-Dalgarno (SD) sequence of potA mRNA (). The aSD sequence is located between 2 stem-loops in the SorX 3′ fragment and was shown to be conserved among close relatives of R. sphaeroides.Citation14 sRNAs that directly bind to the ribosome binding site of a target mRNA mostly affect the rate of translation.Citation25,26 However, translational repression is frequently paralleled by destabilization of target mRNAs. We determined the half-life of potA mRNA and found that it was increased by ∼30% in the SorX deletion strain compared to the wild type ().
Overexpression of mutated SorX also failed to affect resistance toward tBOOH (). The same was true for overexpression of wild-type SorX in a strain lacking Hfq (). A strain lacking the potA gene showed increased resistance to both tBOOH and singlet oxygen when compared to its parental wild type (). Overexpression of SorX in the potA deletion background was not able to trigger resistance to tBOOH (). Taken together our data strongly support the assumption that SorX-mediated resistance to organic hydroperoxides depends on potA as a direct target of regulation.
Figure 7. Effect of SorX overexpression in the SorX deletion background (A) or the hfq deletion strain (B) on resistance to organic hydroperoxides as determined by zone inhibition assays. Strains harboring the empty vector pBBR4352 served as controls (set to 100%). 1 M tBOOH was applied for the assay. Asterisks indicate a statistically significant change in inhibition zones (p ≤ 0.05) compared to their respective control strains (C) Effect of potA deletion on resistance to singlet oxygen and organic hydroperoxides monitored by zone inhibition assays. 1 M tBOOH or 50 mM methylene blue with light were used. Asterisks indicate a statistically significant change in inhibition zones (p ≤ 0.05) compared to wild type 2.4.1 (D) Effect of SorX overexpression in the potA deletion strain on resistance to singlet oxygen and organic hydroperoxides. The error bars indicate the standard deviation from the mean of biological triplicates.
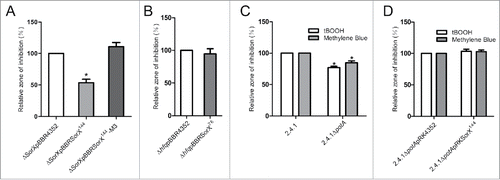
Addition of the polyamine spermidine increases the sensitivity to organic hydroperoxides in a potA-dependent manner
The PotABCD transporter is an uptake system for spermidine in E. coliCitation27 and most likely serves a similar function in R. sphaeroides. Various effects of polyamines on bacterial cells have been described.Citation28 The addition of spermidine to the growth medium led to higher levels of reactive oxygen species in all tested Rhodobacter strains () and significantly increased the sensitivity of R. sphaeroides wild type to tBOOH (). The same observation was made for the SorX deletion strain. In contrast, the potA deletion strain was less susceptible to the harmful effect of spermidine and showed significantly higher resistance than its parental wild type (). Spermidine together with GSH is a substrate for the synthesis of glutathionylspermidine, which is used for modification of proteins. demonstrates that in presence of spermidine the GSH level is significantly decreased in the wild type supporting an effect of spermidine on the GSH concentration. As a main cellular reductant GSH has a pivotal role in the defense against oxidative stress. The difference in GSH levels in presence or absence of spermidine in the medium was not significant in the potA mutant.
Figure 8. (A) Effect of spermidine on cellular reactive oxygen species levels. Cultures were grown under aerobic condition and harvested at an OD660 of 0.2 to quantify cellular reactive oxygen species (ROS) levels using the oxidation-sensitive fluorescent probe 2,7-dihydrodichlorofluorescein diacetate. The ROS level in wild type 2.4.1 without spermidine served as a control and was set to 100%. All other ROS levels were calculated relative to the control. (B) Effect of spermidine on resistance to organic hydroperoxides monitored by zone inhibition assays. The inhibition zone diameter from wild type 2.4.1 without spermidine served as a control and was set to 100%. (C) Effect of spermidine on intracellular GSH levels. The GSH level in wild type 2.4.1 without spermidine served as a control and was set to 100%. The error bars indicate the standard deviation from the mean of biological triplicates with technical replicates. Asterisks indicate a statistically significant change (p ≤ 0.05). Cultures were untreated (white bars) or treated with 10 mM spermidine (gray bars).
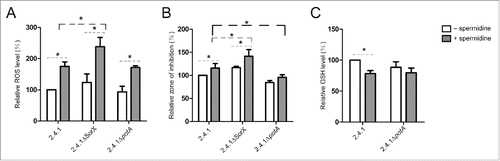
Discussion
Previous reports have demonstrated the involvement of protein and RNA regulators in the response of R. sphaeroides to photooxidative stress.Citation5,11,12,17,18 Here we demonstrate a role for another sRNA, previously known as RSs2461 and renamed here SorX, in the photooxidative stress response. As already observed for the SorY and CcsR sRNAs, different stress factors can induce SorX expression, which seems to be a common feature of RpoHI/HII-dependent sRNAs. Upon deletion or overexpression of SorX, resistance to organic hydroperoxides and singlet oxygen was only slightly affected. This is in agreement with the view that most sRNAs have a modulating function in regulatory networksCitation29 and phenotypes of deletion or overexpression strains are rarely pronounced. The conserved 3′ fragment of SorX represents the functional entity in R. sphaeroides and is highly conserved within the Rhodobacteraceae family. Synteny analysis further revealed that sorX is generally preceded by a gene encoding a 2-component transcriptional regulator (e.g., the OmpR-type regulator RSP_0847 in R. sphaeroides). In Roseobacter denitrificans, a 71-nt SorX homolog was shown to be induced upon photooxidative stress and to be co-transcribed with the RSP_0847 homolog mtrA.Citation30 This suggests a conserved function of the RSP_0847-sorX locus in Alphaproteobacteria. It is tempting to speculate that SorX and the OmpR-type regulator represent the non-coding and coding arm of the same stress response, as was e.g. shown for the 3′ UTR-derived sRNA CpxQ in the Cpx response in enterobacteria.Citation31 Preliminary data on OmpR-dependent regulation in R. sphaeroides are not conclusive enough to answer this question without ambiguity.
SorX is a 3′ UTR-derived sRNA that is co-transcribed with RSP_0847 in an RpoHI/HII-dependent fashion.Citation12,14 sRNAs that are derived from the 3′ region of known mRNAs are an emerging class of sRNAs in bacteria.Citation32 Our data demonstrate that the functional 75-nt SorX fragment is generated from its precursor by RNase E cleavage. It is however not known how the 116-nt pre-SorX fragment is generated and whether RNase E cleavage occurs on the full-length co-transcript or on the 116-nt transcript. It was predicted by ChIP-seq experiments that SorX has an upstream FnrL binding site partly internal to RSP_0847.Citation22 SorX was however not detected in a strain baring the RpoHI/HII promoter upstream of RSP_0847, which argues for co-transcription together with RNase E-dependent processing to be the primary pathway for SorX biogenesis.
Transcriptome analysis revealed a strong effect of SorX on the levels of other RpoHI/HII-dependent sRNAs with a role in singlet oxygen and other stress responses: SorY and CcsR.Citation17,18 Regulatory networks that contain several sRNAs, which indirectly affect each other, are commonly found in bacteria.Citation25,29,33,34 In Salmonella, deletion of the 2 small RNAs RybB and MicA leads to upregulation of the alternative sigma factor σE due to chronic envelope stress.Citation35 Similarly, deletion of SorX was expected to increase oxidative stress levels and consequently to trigger RpoHII. Also, deletion of SorX might affect other sRNAs due to altered competition for Hfq.Citation36-38 In both scenarios further RpoHI/HII- and Hfq-dependent sRNAs (e.g., CcsR and SorY) would increase in transcript level. Our data however reveal that deletion of SorX results in a strong decline of CcsR and SorY, which could be contributed to a diminished transcription. Since rpoHII mRNA levels, as measured by real time RT-PCR, were not changed (data not shown), it is conceivable that SorX acts on another regulatory factor, which still needs identification.
One aim of this study was the target identification of SorX. Based on the transcriptome and proteome data together with bioinformatic predictions for sRNA-mRNA interactions, we selected potA (RSP_1882) for further analysis and could verify it as a direct target of SorX. SorX targets the SD sequence of potA mRNA via an aSD sequence located between the 2 stem-loops in the SorX 3′ fragment. Targeting the SD sequence to inhibit translation is a common mechanism of bacterial sRNAs.Citation39 A very similar regulation was also shown for takP (gene for a TRAP-T transporter) in R. sphaeroides: the SD sequence of takP mRNA is targeted by an aSD sequence located between 2 stem-loops in the SorY RNA.Citation17 On that note, SorX and SorY share some distinct homologous features and might regulate an overlapping regulon. Their aSD sequences and some neighboring nucleotides are almost identical (Fig. S6). Notably, takP was down-regulated after SorX overexpression and it appears possible that takP is regulated by both SorY and SorX. In contrast, potA was not regulated after SorY overexpression, indicating that the 2 sRNAs have both shared and individual targets. This situation is reminiscent of regulation of outer membrane proteins by sRNAs in enterobacteria.Citation26 It is conceivable that transporters in Rhodobacter species are regulated by sRNAs in a dense overlapping regulon under oxidative stress conditions.
The CcsR sRNAs of R. sphaeroides, which are also involved in defense against oxidative stress, represent another example of how to regulate with an aSD sequence.Citation18 CcsR sRNAs harbor 2 stem-loops each with a CCUCCUCCC sequence in the loop and belong to the “cuckoo family” of RNAs that are highly conserved among Alphaproteobacteria.Citation40 Like SorX, the CcsR sRNAs are co-transcribed from an RpoHI/HII promoter together with an upstream open reading frame. It was evidenced that the CcsR sRNAs use their cuckoo motives to target an AG-rich sequence within the flhR mRNA slightly upstream of the actual SD sequence. Although the exact mechanism of regulation remains elusive, it is obvious that several RpoHI/HII-dependent sRNAs in R. sphaeroides exploit aSD sequences to regulate their targets.
Here we verified potA to be a direct SorX target. potA is the first gene in the potADBC operon that encodes the subunits of a putative polyamine transporter. A mutant strain lacking potA showed increased resistance to tBOOH when compared to the parental strain, proving a role of this transporter in stress resistance. In E. coli the proteins encoded by the pot operon are involved in the import of polyamines. Unfortunately, the physiological role of polyamines in living cells is not clear. They are implicated in a wide variety of biological reactions,Citation41 are considered as simple primordial stress molecules, which are induced by diverse stresses, and are beneficial for survival.Citation28 Polyamines can function as ROS scavengers, acid tolerance factor and chemical chaperone, and can regulate stress response genes.Citation28 Polyamines were shown to protect E. coli cells from the toxic effect of oxygen or the superoxide generating paraquat.Citation42 Polyamines were reported to stimulate translation of various mRNAs with weak or inefficient SD sequences, which encode proteins with a role in stress responses in E. coli.Citation43 Furthermore, the polyamine spermidine together with GSH is a substrate for the synthesis of glutathionylspermidine (Gsp), which is used for modification of proteins. The Level of Gsp-modified proteins increased in E. coli in response to oxidative stress.Citation44 Gsp is also used by glyoxalase I and II as thiol cofactor to convert toxic methylglyoxal to non-toxic D-lactate in presence of Ni2+ ions.Citation45 Methylglyoxal is generated when catabolic and anabolic reactions are imbalanced, which occurs under oxidative stress.Citation46 When cells of Acinetobacter baumannii were grown with polyamines, intracellular GSH levels were significantly decreased.Citation47 In R. sphaeroides an effect of spermidine on binding of the global transcriptional regulator PrrA to DNA was observed.Citation48 This study described induction of the photosynthetic apparatus under aerobic conditions in presence of spermidine as a consequence of altered PrrA affinity to DNA. In contrast to this report we could not detect an effect of spermidine on the formation of photosynthetic complexes in R. sphaeroides (data not shown). However, we observed significantly increased ROS levels when spermidine was added to aerobically grown R. sphaeroides cultures implying that high spermidine levels can cause stress. Considering this, decreased import of spermidine under stress conditions due to downregulation of the Pot system by SorX would help to decrease ROS levels.
SorX is the second singlet oxygen induced sRNA of R. sphaeroides that targets the mRNA for a transporter. While the role of spermidine in stress responses is not clear to date, SorY affects malate import and thereby reduces the metabolic flux into the tricarboxylic acid cycle. Under oxidative stress bacteria switch from glycolysis to the pentose phosphate pathway and reduce activity of the TCA cycle.Citation49 As a consequence the production of the prooxidant NADH is reduced and production of the protective NADPH is enhanced. Together with the modulation of C1 metabolism by the CcsR sRNAs this demonstrates, how the same stress signal acts on multiple RNA regulators, each affecting different metabolic processes in order to defend the stress situation.
Materials and methods
Bacterial strains and cultivation
Bacterial strains and plasmids used in this study are listed in Table S1 and construction procedures are submitted as Supplementary Methods. R. sphaeroides strains were grown in minimal salt medium at 32°C either under aerobic (180 µM dissolved oxygen) or microaerobic (5 µM dissolved oxygen) conditions as described before.Citation18 All stress experiments were performed when an OD660 of 0.4 was reached (exponential growth phase). For oxidative stress experiments, R. sphaeroides strains were cultured under aerobic conditions and treated with 250 µM paraquat (Sigma-Aldrich; 856177), 100 µM tBOOH (tert-butyl hydroperoxide; Sigma-Aldrich; B2633) or 1 mM H2O2 (Carl Roth; 8070.2). Photooxidative stress experiments were performed under aerobic conditions as described (0.2 µM methylene blue [Sigma-Aldrich; M9140] and 800 W/m2 white light).Citation2 Microaerobic cultures were treated with 10 µM CdCl2, 1.5 mM diamide (Sigma-Aldrich; D3648) For heat shock experiments, cells were grown at 32°C under microaerobic conditions and shifted to 42°C in a water bath. Cells were harvested on ice and collected by centrifugation at 10,000 g and 4°C. Escherichia coli strains were grown at 37°C in Luria-Bertani broth with continuous shaking at 180 rpm. When needed, antibiotics were used at concentrations given in Table S3.
Zone inhibition assay
To verify the sensitivity of R. sphaeroides to singlet oxygen and organic hydroperoxides, zone inhibition assays were performed as described before.Citation18 Over-night cultures were diluted to an OD660 of 0.2 and cultured under microaerobic conditions at 32°C. At an OD660 of 0.4, 500 µl of the culture were mixed with 5 ml of soft agar (0.8% agarose in minimal salt medium) and poured on top of an agar plate (1.6% agarose in minimal salt medium) without antibiotics. A filter soaked with 7 µl of 1 M tBOOH was placed on top of the plates. After incubation at 32°C for 2 to 3 days, the diameter of inhibition zones was measured. For zone inhibition assays with spermidine, cultures were grown under aerobic condition until an OD660 of 0.2 was reached. One ml culture was mixed with 5 ml of soft agar containing 10 mM spermidine (Sigma-Aldrich; S2501) and poured on top of an agar plate as described. Filters were soaked with 7 µl of 700 mM tBOOH.
Intracellular GSH measurement
Cultures were grown under microaerobic conditions to an OD660 of 0.4. Two ml of culture were harvested by centrifugation at 10,000 g. Cells were resuspended in 3.75% 5-sulfosalicylic acid for lysis by vigorous shaking. After centrifugation at 10,000 g, the total GSH was measured in the supernatant. GSH measurements were performed as described before.Citation18 Total GSH concentrations were normalized to OD660 values.
RNA isolation
For Northern blot analysis, total RNA was isolated by the hot phenol method.Citation50 For Real Time RT-PCR, the peqGOLD TriFast™ Kit (PEQLAB; 30-2010) was used to isolate total RNA as described in the manufacturer′s manual. Samples were treated with 12 U of DNaseI (Invitrogen; #18047019) per 1 µg of RNA at 37°C for 1 hour to remove DNA contaminations. Afterwards, RNA was purified by a mixture of phenol/chloroform/isoamyl alcohol and the quality was confirmed on 10% polyacrylamide gels.
Northern blot analysis
Northern blots were performed as described before.Citation14 For detection of SorX, a PCR product spanning the RSP_0847-sorX locus was labeled with [α-32P]-dCTP (Hartmann Analytic; SRP-205) using the Prime-a-Gene® Labeling System (Promega; U1100) as described in the manufacturer's manual. CcsR1 and SorY sRNAs were detected as described elsewhere.Citation18 Oligodeoxynucleotides used for detection are listed in Table S2. Membranes were hybridized over night at 42°C in low stringency Church buffer (7% SDS, 10 mg/ml BSA, 0.5 M Na2HPO4 (pH 7.2), 1 mM EDTA).Citation51 A buffer containing 5x SSC and 0.01% SDS was used to wash the membranes. Afterwards, membranes were exposed on phosphoimaging screens (Bio-Rad) and images were analyzed using 1D-Quantity One software (Bio-Rad).
Real time RT-PCR
The Brilliant III Ultra-Fast SYBR® Green QRT-PCR Master Mix (Agilent; #600886) was used for Real Time RT-PCR. A final concentration of 4 ng DNaseI-digested RNA per μl was applied to 10 µl reactions. Reactions were run in a C1000™ Thermal Cycler (Bio-Rad) to calculate cycle threshold (Ct) values for relative quantification. Primers used for amplification are listed in Table S2 and their efficiencies can be found in Table S4. Expression levels of mRNAs were calculated relative to control samples and normalized to the reference gene rpoZ according to the formula given by Pfaffl.Citation52 Half-life determination of mRNAs by Real Time RT-PCR has been described previously.Citation17
5′ RACE
Total RNA was isolated using the hot phenol method and applied to 5′ RACE as described.Citation14 The RNA adapter and primers are listed in Table S2. RT-PCR products were separated on 10% polyacrylamide gels.
Microarray analysis
Transcriptomes of R. sphaeroides 2.4.1pRKSorX144 and 2.4.1pRK415 were compared by microarray analysis as described.Citation6 In short, RNA was isolated by the hot phenol methodCitation50 and hybridized to Custom Gene Expression Microarrays from Agilent Technologies (8 × 15K; ID: 027061) after labeling with either Cy3 or Cy5 (Kreatech; EA-022/EA-023) (2-color microarrays). Raw median fluorescence values were extracted using an Agilent DNA microarray scanner and the Feature Extraction Software (Agilent). Within-array normalization according to LOESS was accomplished with the Bioconductor package Limma for R.Citation53 Probes with fluorescence intensities above background were retained and subsequently used to calculate fold changes as log2 ratios (Cy3/Cy5). Probe-specific p-values were combined to gene-specific p-values using the sumlog function (Fisher's method) in package Metap for R. Gene-specific p-values were adjusted for multiple comparison using the method of HolmCitation54 in function p.adjust. Data shown in this study represent the results from 2 biological replicates and one technical replicate of one of the biological replicates to give a total of 3 microarrays. The microarray results can be found in Table S5.
The data discussed in this publication have been deposited in NCBI's Gene Expression OmnibusCitation55 and are accessible through GEO Series accession number GSE79421.
Two-dimensional gel electrophoresis
Radioactive labeling of proteins and proteome analysis by 2D gel electrophoresis were carried out as described elsewhere.Citation5,11
In vivo reporter assay
In order to confirm possible interactions between SorX and its predicted target gene potA, a lacZ-based in vivo reporter assay was applied.Citation24 Construction of reporter plasmids is described in Supplementary Methods. Reporter plasmids were transferred to R. sphaeroides strains by conjugation. Conjugants of each strain were grown in 3 independent liquid cultures at 32°C. Three samples from each culture were withdrawn at an OD660 of 0.4. Measurements of β-galactosidase activity were carried out as described before.Citation56
Quantification of reactive oxygen species
Reactive oxygen species (ROS) were measured as described beforeCitation57 using the oxidation-sensitive fluorescent probe 2,7-dihydrodichlorofluorescein diacetate (DCFH-DA; Molecular Probes/Thermo Fisher Scientific; D399). 95 µl of culture were incubated with 5 µl DCFH-DA (100 μM) for 1 hour at 32°C in the dark. Fluorescence intensities (excitation: 492 nm, emission: 525 nm) were measured in an Infinite® M200 microplate reader (Tecan).
Disclosure of potential confllicts of interest
No potential conflicts of interest were disclosed.
Supplementary_Data.zip
Download Zip (805.2 KB)Funding
T. Peng was supported by a fellowship of the Chinese Scholarship Council (CSC), J.I. Oh by the Financial Supporting Project of Long-term Overseas Dispatch of PNU's Tenure-track faculty. B.A. Berghoff wants to acknowledge the DFG for a Research Fellowship (BE5210/1-1; BE5210/2-1). The project was supported by DFG (Kl563/20-2).
References
- Bauer C, Elsen S, Swem LR, Swem DL, Masuda S. Redox and light regulation of gene expression in photosynthetic prokaryotes. Philos Trans R Soc Lond B Biol Sci 2003; 358:147-53; PMID:12594923; http://dx.doi.org/10.1098/rstb.2002.1189
- Glaeser J, Klug G. Photo-oxidative stress in Rhodobacter sphaeroides: protective role of carotenoids and expression of selected genes. Microbiol 2005; 151:1927-38; PMID:15942000; http://dx.doi.org/10.1099/mic.0.27789-0
- Dufour YS, Landick R, Donohue TJ. Organization and evolution of the biological response to singlet oxygen stress. J Mol Biol 2008; 383:713-30; PMID:18723027; http://dx.doi.org/10.1016/j.jmb.2008.08.017
- Glaeser J, Nuss AM, Berghoff BA, Klug G. Singlet oxygen stress in microorganisms. Adv Microb Physiol 2011; 58:141-73; PMID:21722793; http://dx.doi.org/10.1016/B978-0-12-381043-4.00004-0
- Glaeser J, Zobawa M, Lottspeich F, Klug G. Protein synthesis patterns reveal a complex regulatory response to singlet oxygen in Rhodobacter. J Proteome Res 2007; 6:2460-71; PMID:17536848; http://dx.doi.org/10.1021/pr060624p
- Berghoff BA, Konzer A, Mank NN, Looso M, Rische T, Förstner KU, Krüger M, Klug G. Integrative “omics”-approach discovers dynamic and regulatory features of bacterial stress responses. PLoS Genet 2013; 9:e1003576; PMID:23818867; http://dx.doi.org/10.1371/journal.pgen.1003576
- Braatsch S, Moskvin OV, Klug G, Gomelsky M. Responses of the Rhodobacter sphaeroides transcriptome to blue light under semiaerobic conditions. J Bacteriol 2004; 186:7726-35; PMID:15516587; http://dx.doi.org/10.1128/JB.186.22.7726-7735.2004
- Anthony JR, Warczak KL, Donohue TJ. A transcriptional response to singlet oxygen, a toxic byproduct of photosynthesis. Proc Natl Acad Sci USA 2005; 102:6502-7; PMID:15855269; http://dx.doi.org/10.1073/pnas.0502225102
- Newman JD, Falkowski MJ, Schilke BA, Anthony LC, Donohue TJ. The Rhodobacter sphaeroides ECF Sigma Factor, aE, and the Target promoters cycA P3 and rpoE P1. J Mol Biol 1999; 294:307-20; PMID:10610760; http://dx.doi.org/10.1006/jmbi.1999.3263
- Nuss AM, Adnan F, Weber L, Berghoff BA, Glaeser J, Klug G. DegS and RseP homologous proteases are involved in singlet oxygen dependent activation of RpoE in Rhodobacter sphaeroides. PLoS One 2013; 8:e79520; PMID:24223961; http://dx.doi.org/10.1371/journal.pone.0079520
- Nuss AM, Glaeser J, Klug G. RpoH(II) activates oxidative-stress defense systems and is controlled by RpoE in the singlet oxygen-dependent response in Rhodobacter sphaeroides. J Bacteriol 2009; 191:220-30; PMID:18978062; http://dx.doi.org/10.1128/JB.00925-08
- Nuss AM, Glaeser J, Berghoff BA, Klug G. Overlapping alternative sigma factor regulons in the response to singlet oxygen in Rhodobacter sphaeroides. J Bacteriol 2010; 192:2613-23; PMID:20304993; http://dx.doi.org/10.1128/JB.01605-09
- Dufour YS, Imam S, Koo BM, Green HA, Donohue TJ. Convergence of the transcriptional responses to heat shock and singlet oxygen stresses. PLoS Genet 2012; 8:e1002929; PMID:23028346; http://dx.doi.org/10.1371/journal.pgen.1002929
- Berghoff BA, Glaeser J, Sharma CM, Vogel J, Klug G. Photooxidative stress-induced and abundant small RNAs in Rhodobacter sphaeroides. Mol Microbiol 2009; 74:1497-512; PMID:19906181; http://dx.doi.org/10.1111/j.1365-2958.2009.06949.x
- De Lay N, Schu DJ, Gottesman S. Bacterial small RNA-based negative regulation: Hfq and its accomplices. J Biol Chem 2013; 288:7996-8003; PMID:23362267; http://dx.doi.org/10.1074/jbc.R112.441386
- Berghoff BA, Glaeser J, Sharma CM, Zobawa M, Lottspeich F, Vogel J, Klug G. Contribution of Hfq to photooxidative stress resistance and global regulation in Rhodobacter sphaeroides. Mol Microbiol 2011; 80:1479-95; PMID:21535243; http://dx.doi.org/10.1111/j.1365-2958.2011.07658.x
- Adnan F, Weber L, Klug G. The sRNA SorY confers resistance during photooxidative stress by affecting a metabolite transporter in Rhodobacter sphaeroides. RNA Biol 2015; 12:569-77; PMID:25833751; http://dx.doi.org/10.1080/15476286.2015.1031948
- Billenkamp F, Peng T, Berghoff BA, Klug G. A cluster of four homologous small RNAs modulates C1 metabolism and the pyruvate dehydrogenase complex in Rhodobacter sphaeroides under various stress conditions. J Bacteriol 2015; 197:1839-52; PMID:25777678; http://dx.doi.org/10.1128/JB.02475-14
- Ryter SW, Tyrrell RM. Singlet molecular oxygen (1O2): A possible effector of eukaryotic gene expression. Free Radic Biol Med 1998; 24:1520-34; PMID:9641271; http://dx.doi.org/10.1016/S0891-5849(97)00461-9
- Apirion D. Isolation, genetic mapping and some characterization of a mutation in Escherichia coli that affects the processing of ribonucleic acid. Genetics 1978; 90:659-71; PMID:369943
- Goldblum K, Apirion D. Inactivation of the ribonucleic acid-processing enzyme ribonuclease E blocks cell division. J Bacteriol 1981; 81:128-32; PMID:6163761
- Imam S, Noguera DR, Donohue TJ. Global analysis of photosynthesis transcriptional regulatory networks. PLoS Genet 2014; 10:e1004837; PMID:25503406; http://dx.doi.org/10.1371/journal.pgen.1004837
- Smith C, Heyne S, Richter AS, Will S, Backofen R. Freiburg RNA Tools: a web server integrating INTARNA, EXPARNA and LOCARNA. Nucleic Acids Res 2010; 38:373-7; PMID:20444875; http://dx.doi.org/10.1093/nar/gkq316
- Mank NN, Berghoff BA, Hermanns YN, Klug G. Regulation of bacterial photosynthesis genesby the small noncoding RNA PcrZ. Proc Natl Acad Sci USA 2012; 109:16306-11; PMID:22988125; http://dx.doi.org/10.1073/pnas.1207067109
- Storz G, Vogel J, Wassarman KM. Regulation by small RNAs in bacteria: expanding frontiers. Mol Cell 2011; 43:880-91; PMID:21925377; http://dx.doi.org/10.1016/j.molcel.2011.08.022
- Beisel CL, Storz G. Base pairing small RNAs and their roles in global regulatory networks. FEMS Microbiol Rev 2010; 34:866-82; PMID:20662934; http://dx.doi.org/10.1111/j.1574-6976.2010.00241.x
- Igarashi K, Kashiwagi K. Polyamine transport in bacteria and yeast. Biochem J 1999; 344:633-42; PMID:10585849; http://dx.doi.org/10.1042/bj3440633
- Rhee HJ, Kim EJ, Lee JK. Physiological polyamines: simple primordial stress molecules. J Cell Mol Med 2007; 11:685-703; PMID:17760833; http://dx.doi.org/10.1111/j.1582-4934.2007.00077.x
- De Lay N, Gottesman S. A complex network of small non-coding RNAs regulate motility in Escherichia coli. Mol Microbiol 2012; 86:524-38; PMID:22925049; http://dx.doi.org/10.1111/j.1365-2958.2012.08209.x
- Berghoff BA, Glaeser J, Nuss AM, Zobawa M, Lottspeich F, Klug G. Anoxygenic photosynthesis and photooxidative stress: a particular challenge for Roseobacter. Environ Microbiol 2011; 13:775-91; PMID:21108722; http://dx.doi.org/10.1111/j.1462-2920.2010.02381.x
- Chao Y, Vogel J. A 3′ UTR-derived small RNA provides the regulatory noncoding arm of the inner membrane stress response. Mol Cell 2016; 61:352-63; PMID:26805574; http://dx.doi.org/10.1016/j.molcel.2015.12.023
- Miyakoshi M, Chao Y, Vogel J. Regulatory small RNAs from the 3′ regions of bacterial mRNAs. Curr Opin Microbiol 2015; 24:132-9; PMID:25677420; http://dx.doi.org/10.1016/j.mib.2015.01.013
- Hussein R, Lim HN. Disruption of small RNA signaling caused by competition for Hfq. Proc Natl Acad Sci USA 2011; 108:1110-5; PMID:21189298; http://dx.doi.org/10.1073/pnas.1010082108
- Vogel J, Papenfort K. Small non-coding RNAs and the bacterial outer membrane. Curr Opin Microbiol 2006; 9:605-11; PMID:17055775; http://dx.doi.org/10.1016/j.mib.2006.10.006
- Papenfort K, Pfeiffer V, Mika F, Lucchini S, Hinton JC, Vogel J. aE-dependent small RNAs of Salmonella respond to membrane stress by accelerating global omp mRNA decay. Mol Microbiol 2006; 62:1674-88; PMID:17427289; http://dx.doi.org/10.1111/j.1365-2958.2006.05524.x
- Papenfort K, Said N, Welsink T, Lucchini S, Hinton JC, Vogel J. Specific and pleiotropic patterns of mRNA regulation by ArcZ, a conserved, Hfq-dependent small RNA. Mol Microbiol 2009; 74:139-58; PMID:19732340; http://dx.doi.org/10.1111/j.1365-2958.2009.06857.x
- Fender A, Elf J, Hampel K, Zimmermann B, Wagner EG. RNAs actively cycle on the Sm-like protein Hfq. Genes Dev 2010; 24:2621-6; PMID:21123649; http://dx.doi.org/10.1101/gad.591310
- Moon K, Gottesman S. Competition among Hfq-binding small RNAs in Escherichia coli. Mol Microbiol 2011; 82:1545-62; PMID:22040174; http://dx.doi.org/10.1111/j.1365-2958.2011.07907.x
- Storz G, Opdyke JA, Zhang A. Controlling mRNA stability and translation with small, noncoding RNAs. Curr Opin Microbiol 2004; 7:140-4; PMID:15063850; http://dx.doi.org/10.1016/j.mib.2004.02.015
- Reinkensmeier J, Giegerich R. Thermodynamic matchers for the construction of the cuckoo RNA family. RNA Biol 2015; 12:197-207; PMID:25779873; http://dx.doi.org/10.1080/15476286.2015.1017206
- Tabor CW, Tabor H. Polyamines in microorganisms. Microbiol Rev 1985; 49:81-9; PMID:3157043
- Minton KW, Tabor H, Tabor CW. Paraquat toxicity is increased in Esherichia coli defective in the synthesis of polyamines. Proc Natl Acad Sci USA 1990; 87:2851-5; PMID:2181453; http://dx.doi.org/10.1073/pnas.87.7.2851
- Yoshida M, Kashiwagi K, Shigemasa A, Taniguchi S, Yamamoto K, Makinoshima H, Ishihama A, Igarashi K. A unifying model for the role of polyamines in bacterial cell growth, the polyamine modulon. J Biol Chem 2004; 279:46008-13; PMID:15326188; http://dx.doi.org/10.1074/jbc.M404393200
- Chiang BY, Chen TC, Pai CH, Chou CC, Chen HH, Ko TP, Hsu WH, Chang CY, Wu WF, Wang AH, et al. Protein S-thiolation by Glutathionylspermidine (Gsp): the role of Escherichia coli Gsp synthetASE/amidase in redox regulation. J Biol Chem 2010; 285:25345-53; PMID:20530482; http://dx.doi.org/10.1074/jbc.M110.133363
- Mullings KY, Sukdeo N, Suttisansanee U, Ran Y, Honek JF. Ni2+-activated glyoxalase I from Escherichia coli: substrate specificity, kinetic isotope effects and evolution within the betaalphabetabetabeta superfamily. J Inorg Biochem 2012; 108:133-40; PMID:22173092; http://dx.doi.org/10.1016/j.jinorgbio.2011.11.008
- Booth IR, Ferguson GP, Miller S, Li C, Gunasekera B, Kinghorn S. Bacterial production of methylglyoxal: a survival strategy or death by misadventure? Biochem Soc T 2003; 31:1406-8; PMID:14641075; http://dx.doi.org/10.1042/bst0311406
- Kwon DH, Hekmaty S, Seecoomar G. Homeostasis of glutathione is associated with polyamine-mediated β-lactam susceptibility in Acinetobacter baumannii ATCC 19606. Antimicrob Agents Chemother 2013; 57:5457-61; PMID:23979736; http://dx.doi.org/10.1128/AAC.00692-13
- Eraso JM, Kaplan S. Regulation of gene expression by PrrA in Rhodobacter sphaeroides 2.4.1: role of polyamines and DNA topology. J Bacteriol 2009; 191:4341-52; PMID:19411327; http://dx.doi.org/10.1128/JB.00243-09
- Rui B, Shen T, Zhou H, Liu J, Chen J, Pan X, et al. A systematic investigation of Escherichia coli central carbon metabolism in response to superoxide stress. BMC Syst Biol 2010; 4:122; PMID:20809933; http://dx.doi.org/10.1186/1752-0509-4-122
- Janzon L, Löfdahl S, Arvidson S. Evidence for a coordinate transcriptional control of α-toxin and protein A synthesis in Staphylococcus aureus. FEMS Microbiol Lett 1986; 33:193-8; http://dx.doi.org/10.1111/j.1574-6968.1986.tb01270.x
- Church GM, Gilbert W. Genomic sequencing. Proc Natl Acad Sci USA 1984; 81:1991-5; PMID:6326095; http://dx.doi.org/10.1073/pnas.81.7.1991
- Pfaffl MW. A new mathematical model for relative quantification in real-time RT-PCR. Nucleic Acids Res 2001; 29:900; PMID:11328886; http://dx.doi.org/10.1093/nar/29.9.e45
- Smyth GK, Speed T. Normalization of cDNA microarray data. Methods 2003; 31:265-73; PMID:14597310; http://dx.doi.org/10.1016/S1046-2023(03)00155-5
- Holm S. A simple sequentially rejective multiple test procedure. Scand J Statist 1979; 6:65-70
- Edgar R, Domrachev M, Lash AE. Gene Expression Omnibus: NCBI gene expression and hybridization array data repository. Nucleic Acids Res 2002; 30:207-10; PMID:11752295; http://dx.doi.org/10.1093/nar/30.1.207
- Hübner P, Willison JC, Vignais PM, Bickle TA. Expression of Regulatory nif Genes in Rhodobacter capsulatus. J Bacteriol 1991; 173:2993-9; PMID:1902215
- Remes B, Berghoff BA, Förstner KU, Klug G. Role of oxygen and the OxyR protein in the response to iron limitation in Rhodobacter sphaeroides. BMC Genomics 2014; 15:794; PMID:25220182; http://dx.doi.org/10.1186/1471-2164-15-794
- Hofacker IL. Vienna RNA secondary structure server. Nucleic Acids Res 2003; 31:3429-31; PMID:12824340; http://dx.doi.org/10.1093/nar/gkg599