ABSTRACT
Pathogens secrete effector proteins to suppress host immune responses. Recently, we showed that an aggressive plant fungal pathogen Botrytis cinerea can also deliver small RNA effectors into host cells to suppress host immunity. B. cinerea sRNAs (Bc-sRNAs) translocate into host plants and hijack the plant RNAi machinery to induce cross-kingdom RNAi of host immune responsive genes. Here, we functionally characterized another Bc-sRNA effector Bc-siR37 that is predicted to target at least 15 Arabidopsis genes, including WRKY transcription factors, receptor-like kinases, and cell wall-modifying enzymes. Upon B. cinerea infection, the expression level of Bc-siR37 was induced, and at least eight predicted Arabidopsis target genes were downregulated. These target genes were also suppressed in the transgenic Arabidopsis plants overexpressing Bc-siR37, which exhibited enhanced disease susceptibility to B. cinerea. Furthermore, the knockout mutants of the Bc-siR37 targets, At-WRKY7, At-PMR6, and At-FEI2, also exhibited enhanced disease susceptibility to B. cinerea, giving further support that these genes indeed play a positive role in plant defense against B. cinerea. Our study demonstrates that analysis of pathogen sRNA effectors can be a useful tool to help identify host immunity genes against the corresponding pathogen.
Introduction
In plants, pathogen-associated molecular patterns (PAMPs) are recognized by plant microbial pattern recognition receptors (PRRs), leading to PAMP-triggered immunity (PTI). Most PRRs in the model plant Arabidopsis are involved in plant immunity.Citation13,4,10,29,40,23 In the plant-pathogen arms race, successful pathogens secrete dozens of effector proteins to modulate host physiology and to undermine PTI, the pathways of which has been well characterized for bacterial and oomycete pathogens.Citation1,16,17 Bacterial pathogens evolved molecular secretion systems for effector delivery, such as the type-III and type-IV secretion systems,Citation31 while the delivery and entry of oomycete and fungal effectors into host cells was just started to be understood. As the second layer of the immune response, plant species have evolved many resistance (R) genes. Most R-genes encode nuclear-binding leucine-rich repeat (NB-LRR)-type receptor-like proteins, which recognize pathogen effectors and induce a robust immune response, named effector-triggered immunity (ETI).Citation8,16,5
Small RNAs (sRNAs), such as small interfering RNAs (siRNAs) and microRNAs (miRNAs) that trigger RNA interference (RNAi) are important in regulation and fine-tuning of plant immunity genes.Citation26,19,35 Several plant endogenous siRNAs and miRNAs contribute to the regulation of PTI, such as Arabidopsis miR393, miR160, and miR167;Citation22,37 ETI, such as natural antisense (nat)-siRNAATGB2,Citation20 Arabidopsis long-siRNA-1,Citation18 and miRNA863–3pCitation23; or both PTI and ETI, as in the case of miR393*.Citation38
Even though the regulatory role of endogenous plant sRNAs in plant immunity has been well characterized, little is known about the role of pathogen-derived sRNAs in microbial pathogenicity and host adaptation.Citation33 Remarkably, the fungal pathogen B. cinerea transmits Bc-sRNA effectors into the host cells to silence important host immunity genes by hijacking the host RNAi machinery.Citation2,30,36 Bc-sRNA effectors have been found to target important immune-related genes in two host plant species, Arabidopsis and Solanum lycopersicum (tomato). For example, Bc-siR3.2 targets two related genes, Arabidopsis Mitogen-activated protein kinase 1 (At-MPK1) and At-MPK2, as well as the tomato Sl-MPKKK4. MPKs are important proteins involved in signal transduction in the plant immune responses.Citation36 Most Bc-sRNAs that exhibit predicted host plant target genes are derived from long-terminal repeat (LTR) retrotransposon loci in the B. cinerea genome. LTR retrotransposons have been associated with fast-evolving genome regions that related to microbial virulence.Citation36,35,33 This may result in fast evolutionary turnover of Bc-sRNA effectors which would impact the molecular arms race during host and pathogen coevolution. Our recent study suggested that the fungal plant pathogen Verticillium dahliae, the causal agent of Verticillium wilt, also uses pathogen sRNAs (Vd-sRNAs) as effectors, as Vd-sRNAs were found to be loaded into Arabidopsis Argonaute1 (AGO1) during infection.Citation34 The majority of the predicted host targets of these Vd-sRNA effectors are putative factors of plant immunity. In addition, animal parasites, such as the gastrointestinal nematode Heligmosomoides polygyrus, also secrete sRNAs via extracellular vesicles. These parasite sRNAs can be taken up by mice epithelial cells and suppress host genes that regulate inflammation and immune responses.Citation7 Thus, these observations of pathogen- or parasite-induced host gene silencing indicate that such cross-kingdom RNAi - based virulence strategy has evolved in diverse pathogens and pests of both plant and animal systems.
Here, we characterized another Bc-sRNA effector, Bc-siR37, which is predicted to target at least 15 host plant genes in Arabidopsis under stringent prediction criteria.Citation6 We confirmed that several of these Arabidopsis target genes were silenced by Bc-siR37. We further verified that three target genes participate in plant defense against B. cinerea: At-WRKY7, At-PMR6 and At-FEI2, which encode an immune-related transcription factor, a pectin-lyase, and a leucine-rich repeat (LRR) receptor kinase, respectively. Thus, identification and characterization of pathogen sRNA effectors is an excellent approach to identify host immunity genes that are involved in plant defense against eukaryotic pathogens.
Results
B. cinerea sRNA Bc-siR37 is predicted to target a large number of host plant genes
Next generation sequencing (NGS) profiling of sRNAs from B. cinerea-infected Arabidopsis leaves, tomato leaves, and tomato fruits led to the identification of Bc-sRNAs, some of which were confirmed to be transported into host cells and silence host immunity genes during the infection process.Citation36 From this data set, we identified another Bc-sRNA effector, Bc-siR37, which was mapped to the Bcin12G_06020 open read frame (ORF) that putatively encodes an ABC-type plasma membrane Bc-ATPase (). Bc-siR37 was identified as a sRNA effector in our previous study based on its multiple predicted host target genes. It was not included in table S3 ofCitation36 because we only listed predicted Bc-sRNA effectors with 100 or more reads per million (RPM) of total Bc-sRNAs. Here, we chose to further study Bc-siR37 because it has 15 predicted plant targets when using the same stringent prediction criteria as inCitation36 (), and several of these targets have already been shown to play a role in host immune responses. Moreover, Bc-siR37 was more enriched in the B. cinerea-infected Arabidopsis libraries as well as the conidiospore fraction library, compared with other sRNAs derived from the Bcin12G_06020 ORF locus (), suggesting that Bc-siR37 is likely functional during host infection.
Figure 1. Bc-siR37 accumulates in B. cinerea-infected Arabidopsis. Bc-sRNA expression profiles at the locus of the Bc-ATPase gene Bcin12G_06020 in B. cinerea mycelium, conidiospore samples, or in the B. cinerea-infected Arabidopsis at 0, 24, 48, and 72 hpi. Bc-siR37 was enriched in infected Arabidopsis at 24 hpi. sRNA reads were normalized to reads per million (RPM) in mycelium and condiospore samples and reads per 10 million (RP10M) in B. cinerea-infected plant samples. The size range of small RNAs that mapped to the Bc-ATPase (Bcin12G_06020) gene locus was 20–35 nt with predominately 21–22-nt, and 24–26-nt classes.
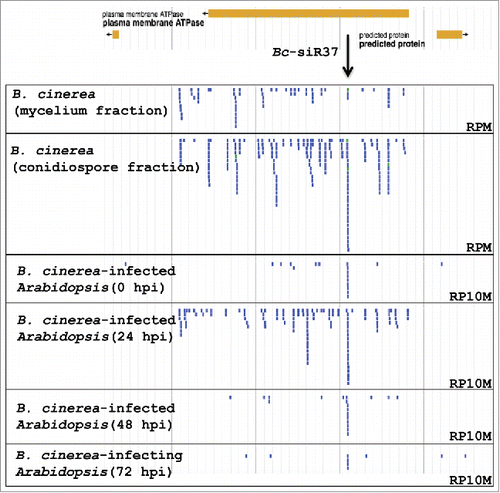
Table 1. Bc-siR37 host targets in Arabidopsis.
Arabidopsis genes targeted by Bc-siR37 have lower transcript levels upon B. cinerea infection
We first aimed to confirm the accumulation of Bc-siR37 in B. cinerea-infected host samples. Infected Arabidopsis leaf materials were collected at 0, 24, 48, and 72 hours post infection (hpi) for RNA extraction and adaptor ligation-based PCR to amplify Bc-siR37. Bc-siR37 was clearly detected at 0, 24, 48, and 72 hpi and enriched at 24 hpi (). This observation was consistent with the NGS deep sequencing data (). As a positive control for B. cinerea sRNA production during infection, we detected Bc-siR3.1, a previously characterized Bc-sRNA effector ().Citation36 Next, if Bc-siR37 has a suppressive effect on predicted host target genes during infection, a lower transcriptional level of these targets would be expected upon B. cinerea infection. Therefore, we performed real-time RT-PCR to quantify transcript levels of At-WRKY7, At-FEI2, At-PMR6, At-ATG5, At-PK, At-TOM7–2, At-DUF538, and At-RING/U-box at 0, 24, 48, and 72 hpi, according to the time points that we had detected the accumulation of the Bc-siR37 by PCR () and by NGS data analysis of sRNAs (). Indeed, transcript levels of predicted target genes evaluated here were reduced at 24, 48, and 72 hpi compared with 0 hpi (). At-PDF1.2 and At-BIK1 were chosen as Botrytis-induced marker genes, which indeed showed strong induction during infection ().Citation21,32 Therefore, we assumed that the reduced transcript levels of predicted Bc-siR37 host targets were due to gene silencing rather than plant cell death caused by the pathogen. Nevertheless, comprehensive transcriptional re-programing is common during stress response in plants, which could explain the reduced transcription levels of the predicted target genes during infection. Therefore, we performed transient co-expression assays with Bc-siR37 and two of its target genes, At-WRKY7 and At-FEI2, to confirm that Bc-siR37 can indeed cause gene silencing of the host target genes.
Figure 2. Arabidopsis target genes of Bc-siR37 are suppressed upon infection. (A) Total RNA from B. cinerea and B. cinerea-infected Arabidopsis collected 0, 24, 48, and 72 hpi was isolated for sRNA library construction. Expression of Bc-siR37 was determined by liagation-based sRNA RT-PCR. Bc-siR3.1 was used as a positive control.Citation36 Expression levels of At-ACTIN and Bc-ACTIN were measured as further control for RNA integrity and B. cinerea infection. Both Bc-siR37 and Bc-siR3.1 are 21-nt long, while the sizes of PCR products are 74- and 75-nt, respectively. (B) Relative mRNA levels of the Bc-siR37 host target genes, At-WRKY7, At-FEI2, At-PMR6, At-ATG5, At-PK, At-TOM7–2, At-DUF538, and At-RING/U-box, as well as B. cinerea-induced At-BIK1 and At-PDF1.2 marker genes, were examined by real-time RT-PCR. The error bars indicate the standard deviation (s.d.) of 3 technical replicates. Similar results were obtained in three independent biological replicates.
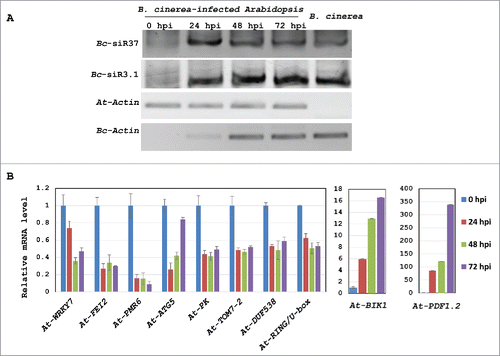
Bc-siR37 efficiently silences Arabidopsis At-WRKY7 and At-FEI2 genes in transient expression assay
Bc-siR37 targets At-WRKY7 and At-FEI2 at their 5′ UTR region with a nearly perfect complementary sequence alignment ( and ). To demonstrate that gene silencing was target site-specific, we conducted an A. tumefaciens-mediated transient co-expression assay of Bc-siR37 with its host target genes using N. benthamiana leaves. We cloned the Bc-siR37 sequence into a plant artificial microRNA vector (amiR-Bc-siR37),Citation28 and the At-WRKY7 or At-FEI2 cDNA sequences into the pEarlygate101 (pE101) binary expression vector that carried a hemagglutinin (HA) and a YFP tag at the C-terminus. We also cloned the synonymous mutations at the sRNA target sites of At-WRKY7 (At-WRKY7m) and At-FEI2 (At-FEI2m) that should be no longer targeted by Bc-siR37 ( and ). Silencing efficiencies of Bc-siR37 on At-WRKY7 and At-FEI2 were analyzed at 48 hours post Agrobacterium-infiltration by Western blot using anti-HA antibody. At-WRKY7 and At-FEI2 were highly expressed without Bc-siR37 co-expression ( and ). In contrast, when co-expressed with Bc-siR37, there was a clear reduction of At-WRKY7 and At-FEI2 expression ( and ), demonstrating sequence-specific gene silencing. Moreover, when we co-expressed the Arabidopsis miRNA At-miR395 instead of Bc-siR37, which had neither sequence homology to At-WRKY7 nor to At-FEI2, no target gene suppression was observed ( and ). Finally, when we co-expressed Bc-siR37 with At-WRKY7m or At-FEI2m, the silencing effect was completely abolished ( and ).
Figure 3. Bc-siR37 silences the host target genes as revealed by co-expression assays in N. benthamiana. Co-expression of Bc-siR37 and its host targets (HA and YFP-tagged) At-WRKY7 (A) and At-FEI2 (B) in N. benthamiana revealed suppression of the host targets by Bc-siR37. The top panel showes the protein levels of At-WRKY7 and its target site-mutated version At-WRKY7m (A), as well as At-FEI2 and At-FEI2m (B), by Western blot analysis. The bottom panel shows the sequence alignment of Bc-siR37 with its target sites in At-WRKY7/At-WRKY7m (A), and At-FEI2/At-FEI2m (B). (C) Expression of At-WRKY7 and At-WRKY7m after infection with B. cinerea was quantified by confocal microscopy. Error bars indicate the s.d. of 5 pictures, and asterisk indicates the statistical significance of P<0.01 (One-way Anova). Similar results were obtained in 2 independent biological replicates (A-C).
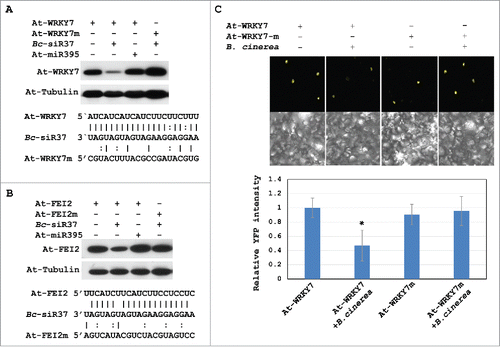
To verify the sequence specificity of YFP-tagged At-WRKY7 silencing during B. cinerea infection of N. benthamiana leaves, we used confocal microscopy coupled with quantitative image analysis. We infected N. benthamiana leaves transiently expressing YFP-At-WRKY7 or YFP-At-WRKY7m with B. cinerea and found that only At-WRKY7 was suppressed at fungal infection sites but not At-WRKY7m (). Based on these results, we believe that Bc-siR37 indeed silences Arabidopsis host genes At-WRKY7 and At-FEI2.
Transgenic Arabidopsis plants expressing Bc-siR37 exhibited attenuated plant defense against B. cinerea
To further confirm that Bc-siR37 indeed silences host target genes in Arabidopsis, we stably transformed the amiR-Bc-siR37 expression vector into Arabidopsis Col-0 wild type (WT) plants. We obtained in total 13 transformed lines that expressed Bc-siR37 at various levels (Bc-siR37ox), as shown by Northern blot analysis (). None of these transformed lines exhibited any obvious morphological or developmental defects. We collected F2 progenies from lines with high expression level of Bc-siR37. To study the silencing effect of constitutively expressed Bc-siR37 on predicted Arabidopsis target genes, we measured transcriptional levels of At-WRKY7, At-FEI2, At-PMR6, At-ATG5, At-PK, At-TOM7–2, At-DUF538, and At-RING/U-box in the Bc-siR37ox transgenic lines in comparison to WT Arabidopsis plants using real-time RT-PCR. In agreement with previous results that showed lower transcript levels of these genes upon B. cinerea inoculation (), all tested Arabidopsis target genes showed lower transcript levels in uninfected Bc-siR37ox lines (). We thus confirmed that Bc-siR37 alone is capable of suppressing these host target genes in Arabidopsis. Association of Bc-siR37 and plant AGO1 protein was also examined after infection by AGO1 co-immunoprecipitation, and Bc-siR37 was clearly detected in the AGO1-pull down fraction, supporting that Bc-siR37 was loaded into Arabidopsis AGO1 (Fig. S1).
Figure 4. Bc-siR37-expressing Arabidopsis exhibits reduced expression of host target genes and enhanced susceptibility toward B. cinerea. (A) The expression levels of Bc-siR37 in the transgenic Arabidopsis Bc-siR37ox lines were shown by Northern blot analysis. (B) The mRNA levels of the predicted Bc-siR37 host target genes At-WRKY7, At-FEI2, At-PMR6, At-ATG5, At-PK, At-TOM7–2, At-DUF538, and At-RING/U-box were reduced in Bc-siR37ox plants. The error bars indicate the s.d. of 3 technical replicates. (C) Arabidopsis Bc-siR37ox plants were more susceptible to B. cinerea than WT Arabidopsis plants. The lesion size was measured 4 d post infection (dpi) using ImageJ software. Error bars indicate s.d. of 10 leaves. The relative biomass was quantified by real-time PCR, and error bars indicate the s.d. of 3 technical replicates. Asterisks indicate statistical significance (t-test, P<0.01). Similar results were obtained in 3 biological replicates for (B)-(C).
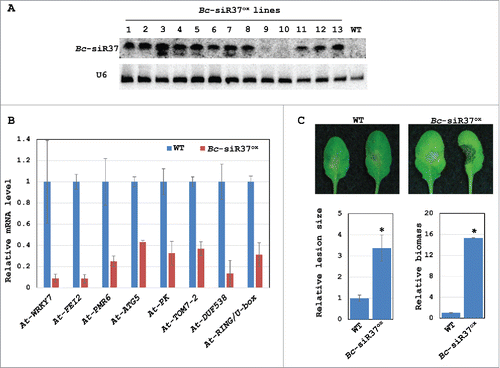
To examine if Bc-siR37ox transgenic plants are impaired in plant defense against B. cinerea infection, we inoculated Bc-siR37ox plants and WT plants with a B. cinerea spore suspension. Bc-siR37ox lines were more susceptible to B. cinerea compared with WT plants (). Consistent with our previous result,Citation36 the virulence of B. cinerea dcl1 dcl2 was partially restored when infected on Bc-siR37ox plants (Fig. S2). These results support that host Bc-siR37 target genes have functional roles in plant immune responses against B. cinerea. This notion was further supported by the fact that T-DNA insertion lines of At-WRKY7, At-FEI2, and At-PRM6 showed enhanced disease susceptibility toward B. cinerea infection ( and Fig. S3A). Moreover, the Arabidopsis transgenic At-WRKY7ox lines, which overexpress At-WRKY7, showed enhanced disease resistance against B. cinerea when compared with WT plants ( and Fig. S3B).
Figure 5. The host target genes of Bc-siR37 are involved in plant immunity against B. cinerea. Arabidopsis at-wrky7, at-fei2, and at-pmr6 mutants were more susceptible to B. cinerea (A), while Arabidopsis At-WRKY7ox lines were more resistant to B. cinerea (B). The lesion sizes were calculated 4 dpi using ImageJ, and error bars represent the s.d. of 10 leaves. The relative biomass was quantified by real-time PCR, and error bars indicate the s.d. of 3 technical replicates. Asterisks indicate statistical significance (One-way Anova for (A), and student's t-test for (B), P<0.01). Similar results were obtained in 3 independent biological replicates.
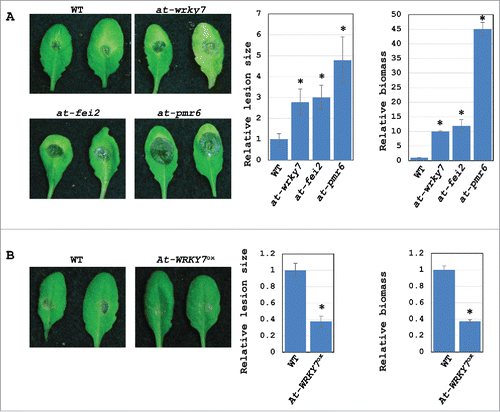
Discussion
Our previous study only listed 73 Bc-sRNA effectors with at least 100 RPM of total Bc-sRNAs, but there are more Bc-sRNA effector candidates with lower reads in the data set. Most of those potential Bc-sRNA sequences were mapped to retrotransposon regions in the B. cinerea genome.Citation36 In this paper, we characterized a new Bc-sRNA effector, Bc-siR37, which is transcribed from the sense strand of a gene, Bcin12G_06020. Bc-siR37 caught our attention because it was predicted to target many host plant genes, some of which were known to be involved in plant immunity. One of the Arabidopsis target genes, At-ATG5, has already been demonstrated to participate in plant defense against B. cinerea.Citation24 In addition, we confirmed that 3 additional Arabidopsis genes, At-WRKY7, At-FEI2, and At-PMR6, are involved in the plant defense responses against B. cinerea.
Unlike most predicted Bc-sRNA effectors, which are derived from retrotransposon regions, Bc-siR37 is derived from a gene encoding a Bc-ATPase, Bcin12G_06020. Interestingly, two more B. cinerea ATPases, Bc-CCC2 and Bc-PMR1, were proven to be involved in pathogenicity.Citation27,25 It needs to be clarified whether the Bc-ATPase from Bcin12G_06020 itself is also required for virulence. At least, sRNA Bc-siR37 from this locus is a bona fide virulence factor.
At-WRKY7 is a negative regulator of salicylic acid (SA)-dependent PR1 gene expression in plant defense response to the bacterial pathogen Pseudomonas syringae.Citation21 This suggests that At-WRKY7 might positively regulate jasmonate (JA)-dependent defense response due to the antagonism of SA and JA hormone signaling pathways.Citation12 Indeed, our results indicated a positive role for At-WRKY7 in defense against the necrotrophic pathogen B. cinerea. However, how At-WRKY7 activates JA-responsive defense genes, either directly as a transcriptional activator, or indirectly through inhibition of SA signaling, is still not understood. Yet, another WRKY transcription factor, At-WRKY33, is a positive regulator of plant immunity against B. cinerea, and it has been shown to indirectly regulate the JA pathway signaling.Citation39,3 Interestingly, another WRKY transcription factor At-WRKY57 is also a target of Bc-siR37. At-WRKY57 was first identified as a transcriptional mediator in response to plant drought tolerance.Citation15 Moreover, At-WRKY57 participates in activation of JA-responsive genes and actively binds to JASMONATE ZIM-DOMAIN4/8 (JAZ4/8),Citation14 which suggests that At-WRKY57 is involved in JA-dependent activation of plant defense against B. cinerea. Taken together, Bc-siR37 targets multiple members of the WRKY transcription factor family in Arabidopsis. Similarly, we had found earlier that Bc-siR3.2 targeted the plant MPK family member At-MPK1 and At-MPK2 in Arabidopsis.Citation36 Therefore, we believe that distinct Bc-sRNA effectors target host plant genes that are key factors of the innate immune responses. These Bc-sRNA effectors seem to target either a single pathway through silencing homologous genes to eliminate functional redundancy or by silencing different target genes for the sake of strengthening suppression efficiency.
Many predicted host targets of Bc-sRNA effectors were demonstrated to be involved in plant immune responses against B. cinerea, including At-MPK1, At-MPK2, At-WAK, At-PRXIIF, At-ATG5, At-WRKY7, At-FEI2, At-PMR6, and Sl-MPKKK4.Citation36 Thus, we propose that predicting target genes of pathogen sRNA effectors is a suitable way to identify new host immunity factors. Here, we found that Bc-siR37 has several host target genes with unknown functions () but are very likely to be involved in host immunity against B. cinerea. Currently, more and more sRNA data sets from diverse pathogen species are becoming available in public databases. Data mining of pathogen sRNA information provides a rich resource to identify novel immunity gene candidates in many host-pathogen systems, providing a short-cut to laborious forward genetic screens.
Materials and methods
Plasmid construction
The amiR-Bc-siR37 construct was designed and generated according to the website WMD3 (http://wmd3.weigelworld.org/cgi-bin/webapp.cgi). The plasmid RS300 was used as template to amplify three fragments with primer pairs Oligo A and Bc-siR37 I miR (a), Bc-siR37 II miR-a and Bc-siR37 III miR*s (b), Bc-siR37 IV miR*a and Oligo B (c). The a, b, c fragments were used as templates, and together with primers Oligo A and Oligo B to amplify fragment d, which exchanged Bc-siR37 into At-miR319a backbone. Fragment d was cloned into pENTR/D-TOPO (Invitrogen, Carlsbad, CA), and next into the destination vector pEG100 by LR reactions (Invitrogen, Carlsbad, CA). Primer sequences are listed in supplementary materials.
At-WRKY7, At-WRKY7m, At-FEI2, and At-FEI2m overexpression vectors: Arabidopsis cDNAs were used as template with primers At-WRKY7-FOR and At-WRKY7-REV, At-WRKY7m-FOR and At-WRKY7-REV, At-FEI2-FOR and At-FEI2-REV, At-FEI2m-FOR and At-FEI2-REV to amplify the cDNA sequences of At-WRKY7, At-WRKY7m, At-FEI2, and At-FEI2m, respectively. At-WRKY7, At-WRKY7m, At-FEI2, and At-FEI2m cDNA fragments were cloned into pENTR/D-TOPO (Invitrogen, Carlsbad, CA), and finally into the destination vector pEG101 by LR reactions (Invitrogen, Carlsbad, CA). All primer sequences are listed in supplementary materials.
Plant materials
Arabidopsis thaliana ecotype Columbia (Col-0) and N. benthamiana were grown at 22°C under 12 h light per day. All mutants used in this study were in the Col-0 background. The at-wrky7 mutant line and At-WRKY7ox line were provided by Dr. Zhixiang Chen's laboratory, and at-pmr6 (CS6354) and at-fei2 (SALK_083958) T-DNA insertion lines were purchased from the Arabidopsis Biological Resource Center (Ohio State Universide, USA).
To generate the Bc-siR37ox lines, the amiR-Bc-siR37 construct was transformed into A. tumefaciens GV3101, which was then used to transform Arabidopsis by the flower dipping method as described previously.Citation9
Adaptor ligation-based PCR method to amplify sRNAs
Total RNA from different tissues was extracted using TRIzol (Invitrogen, Carlsbad, CA). RNA extracts were size-separated on a 14% denatured (8 M Urea) polyacrylamide gel, and the size fractions of 18–30 nt in length were cut out of the gel and purified. The purified sRNA was ligated to 3′ RNA adaptors using truncated T4 RNA ligase 2 (NEB, Ipswich, MA). The ligation products were separated on a 14% denatured polyacrylamide gel and RNA size fractions from 30–50 nt were cut out of the gel and purified. The 3′ adaptor ligated RNA products were ligated with a 5′ RNA adaptor by T4 RNA ligase 1 (NEB, Ipswich, MA). Upon gel size fractionation and purification of the 60–80 nt, the final RNA ligation product was converted into first strand cDNA. The cDNA was amplified using adaptor specific primers SBS5′ and SBS3′. The 116 nt amplification product was purified and used as template for Bc-siR37 amplification using the Bc-siR37 lib forward primer and 3′ adaptor reverse primer SBS3'. All primer sequences are listed in supplementary materials.
Measure mRNA levels of target genes by real-time RT-PCR
Total RNA was extracted from plant tissues using TRIzol (Invitrogen, Carlsbad, CA), and treated with DNase I enzyme (Roche, Basel, Switzerland). The purified DNase I-treated RNA was converted into first strand cDNA using SuperScript III (Invitrogen, Carlsbad, CA). The cDNA was used as template for real-time RT-PCR using the Bio-Rad IQ5 thermocycler (Bio-rad) and the iQ SYBR Green Supermix (Bio-Rad, Hercules, CA), and gene-specific RT-PCR primers (Table S1). The PCR program performed as followed: 95°C at 2 min and 45 cycles of 95°C at 30 s, 55°C at 30 s, 72°C at 30 s, and fluorescence was measured at the end of extension step for 30 s.
Co-expression assay
A. tumefaciens carrying amiR-Bc-siR37 or amiR395 was co-infiltrated with At-WRKY7, At-WRKY7m, At-FEI2, or At-FEI2m into leaves of four-week-old N. benthamiana plants. Two days after infiltration, leaf samples were collected for Western blot analysis or confocal microscopy. Quantitative microscopy analysis was performed as described previously.Citation36
B. cinerea infection assay
B. cinerea spores were collected from 10 d old B. cinerea cultured on malt extract agar plates and diluted to 2 × 105 spores per milliliter in inoculation medium (10 mM sucrose, 10 mM KH2PO4, Tween-20 0.025%) for infection. A 15 µl spore solution drop was placed onto the center of four-week-old Arabidopsis leaves. Photos were taken with a digital camera and analyzed using ImageJ software to measure the lesion size. The calculation of relative DNA contents of B. cinerea to Arabidopsis (or relative biomass) was described previously.Citation11 For infection of N. benthamiana leaves, B. cinerea spores were drop inoculated at the abaxial (bottom) side of Agrobacterium-infiltrated leaves.
Disclosure of potential conflicts of interest
No potential conflicts of interest were disclosed.
Supplemental_Data.docx
Download MS Word (567.7 KB)Acknowledgments
We thank Dr. Zhixiang Chen for at-wrky7 mutant and At-WRKY7ox line seeds. We thank Yifan Lii for editing the paper.
Funding
This work was supported by grants from the National Institute of Health (R01 GM093008) and National Science Foundation (IOS-1257576, IOS-1557812) awarded to HJ.
References
- Alfano JR, Collmer A. Type III secretion system effector proteins: double agents in bacterial disease and plant defense. Ann Rev Phytopathol 2004; 42:385-414; PMID:15283671; http://dx.doi.org/10.1146/annurev.phyto.42.040103.110731
- Baulcombe D. Plant science. Small RNA–the secret of noble rot. Science 2013; 342:45-6; PMID:24092716; http://dx.doi.org/10.1126/science.1245010
- Birkenbihl RP, Diezel C, Somssich IE. Arabidopsis WRKY33 is a key transcriptional regulator of hormonal and metabolic responses toward Botrytis cinerea infection. Plant Physiol 2012; 159:266-85; PMID:22392279; http://dx.doi.org/10.1104/pp.111.192641
- Boller T, Felix G. A renaissance of elicitors: perception of microbe-associated molecular patterns and danger signals by pattern-recognition receptors. Annu Rev Plant Biol 2009; 60:379-406; PMID:19400727; http://dx.doi.org/10.1146/annurev.arplant.57.032905.105346
- Boller T, He SY. Innate immunity in plants: an arms race between pattern recognition receptors in plants and effectors in microbial pathogens. Science 2009; 324:742-4; PMID:19423812; http://dx.doi.org/10.1126/science.1171647
- Bonnet E, He Y, Billiau K, Van de Peer Y. TAPIR, a web server for the prediction of plant microRNA targets, including target mimics. Bioinformatics 2010; 26:1566-8; PMID:20430753; http://dx.doi.org/10.1093/bioinformatics/btq233
- Buck AH, Coakley G, Simbari F, McSorley HJ, Quintana JF, Le Bihan T, Kumar S, Abreu-Goodger C, Lear M, Harcus Y, et al. Exosomes secreted by nematode parasites transfer small RNAs to mammalian cells and modulate innate immunity. Nat Commun 2014; 5:5488; PMID:25421927; http://dx.doi.org/10.1038/ncomms6488
- Chisholm ST, Coaker G, Day B, Staskawicz BJ. Host-microbe interactions: shaping the evolution of the plant immune response. Cell 2006; 124:803-14; PMID:16497589; http://dx.doi.org/10.1016/j.cell.2006.02.008
- Clough SJ, Bent AF. Floral dip: a simplified method for Agrobacterium-mediated transformation of Arabidopsis thaliana. Plant J 1998; 16:735-43; PMID:10069079; http://dx.doi.org/10.1046/j.1365-313x.1998.00343.x
- Dodds PN, Rathjen JP. Plant immunity: towards an integrated view of plant-pathogen interactions. Nat Rev Genet 2010; 11:539-48; PMID:20585331; http://dx.doi.org/10.1038/nrg2812
- Gachon C, Saindrenan P. Real-time PCR monitoring of fungal development in Arabidopsis thaliana infected by Alternaria brassicicola and Botrytis cinerea. Plant Physiol Biochem 2004; 42:367-71; PMID:15191738; http://dx.doi.org/10.1016/j.plaphy.2004.04.001
- Glazebrook J. Contrasting mechanisms of defense against biotrophic and necrotrophic pathogens. Annu Rev Phytopathol 2005; 43:205-27; PMID:16078883; http://dx.doi.org/10.1146/annurev.phyto.43.040204.135923
- He P, Shan L, Sheen J. Elicitation and suppression of microbe-associated molecular pattern-triggered immunity in plant-microbe interactions. Cell Microbiol 2007; 9:1385-96; PMID:17451411; http://dx.doi.org/10.1111/j.1462-5822.2007.00944.x
- Jiang Y, Liang G, Yang S, Yu D. Arabidopsis WRKY57 functions as a node of convergence for jasmonic acid- and auxin-mediated signaling in jasmonic acid-induced leaf senescence. Plant Cell 2014; 26:230-45; PMID:24424094; http://dx.doi.org/10.1105/tpc.113.117838
- Jiang Y, Liang G, Yu D. Activated expression of WRKY57 confers drought tolerance in Arabidopsis. Mol Plant 2012; 5:1375-88; PMID:22930734; http://dx.doi.org/10.1093/mp/sss080
- Jones JD, Dangl JL. The plant immune system. Nature 2006; 444:323-9; PMID:17108957; http://dx.doi.org/10.1038/nature05286
- Kamoun S. A catalogue of the effector secretome of plant pathogenic oomycetes. Annu Rev Phytopathol 2006; 44:41-60; PMID:16448329; http://dx.doi.org/10.1146/annurev.phyto.44.070505.143436
- Katiyar-Agarwal S, Gao S, Vivian-Smith A, Jin H. A novel class of `bacteria-induced small RNAs in Arabidopsis. Genes Dev 2007; 21:3123-34; PMID:18003861; http://dx.doi.org/10.1101/gad.1595107
- Katiyar-Agarwal S, Jin H. Role of small RNAs in host-microbe interactions. Ann Rev Phytopathol 2010; 48:225-46; PMID:20687832; http://dx.doi.org/10.1146/annurev-phyto-073009-114457
- Katiyar-Agarwal S, Morgan R, Dahlbeck D, Borsani O, Villegas A Jr, Zhu JK, Staskawicz BJ, Jin H. A pathogen-inducible endogenous siRNA in plant immunity. Proc Natl Acad Sci U S A 2006; 103:18002-7; PMID:17071740; http://dx.doi.org/10.1073/pnas.0608258103
- Kim KC, Fan B, Chen Z. Pathogen-induced Arabidopsis WRKY7 is a transcriptional repressor and enhances plant susceptibility to Pseudomonas syringae. Plant physiology 2006; 142:1180-92; PMID:16963526; http://dx.doi.org/10.1104/pp.106.082487
- Navarro L, Dunoyer P, Jay F, Arnold B, Dharmasiri N, Estelle M, Voinnet O, Jones JD. A plant miRNA contributes to antibacterial resistance by repressing auxin signaling. Science 2006; 312:436-9; PMID:16627744; http://dx.doi.org/10.1126/science.1126088
- Niu D, Lii YE, Chellappan P, Lei L, Peralta K, Jiang C, Guo J, Coaker G, Jin H. miRNA863-3p sequentially targets negative immune regulator ARLPKs and positive regulator SERRATE upon bacterial infection. Nat Commun 2016; 7:11324; PMID:27108563; http://dx.doi.org/10.1038/ncomms11324
- Penninckx IA, Eggermont K, Terras FR, Thomma BP, De Samblanx GW, Buchala A, Metraux JP, Manners JM, Broekaert WF. Pathogen-induced systemic activation of a plant defensin gene in Arabidopsis follows a salicylic acid-independent pathway. Plant Cell 1996; 8:2309-23; PMID:8989885; http://dx.doi.org/10.1105/tpc.8.12.2309
- Plaza V, Lagues Y, Carvajal M, Perez-Garcia LA, Mora-Montes HM, Canessa P, Larrondo LF, Castillo L. bcpmr1 encodes a P-type Ca2+/Mn2+-ATPase mediating cell-wall integrity and virulence in the phytopathogen Botrytis cinerea. Fungal Genet Biol 2015; 76:36-46; PMID:25677379; http://dx.doi.org/10.1016/j.fgb.2015.01.012
- Ruiz-Ferrer V, Voinnet O. Roles of plant small RNAs in biotic stress responses. Ann Rev Plant Biol 2009; 60:485-510; PMID:19519217; http://dx.doi.org/10.1146/annurev.arplant.043008.092111
- Saitoh Y, Izumitsu K, Morita A, Tanaka C. A copper-transporting ATPase BcCCC2 is necessary for pathogenicity of Botrytis cinerea. Mol Genet Genomics 2010; 284:33-43; PMID:20526618; http://dx.doi.org/10.1007/s00438-010-0545-4
- Schwab R, Ossowski S, Riester M, Warthmann N, Weigel D. Highly specific gene silencing by artificial microRNAs in Arabidopsis. Plant Cell 2006; 18:1121-33; PMID:16531494; http://dx.doi.org/10.1105/tpc.105.039834
- Schwessinger B, Ronald PC. Plant innate immunity: perception of conserved microbial signatures. Annu Rev Plant Biol 2012; 63:451-82; PMID:22404464; http://dx.doi.org/10.1146/annurev-arplant-042811-105518
- Stower H. Small RNAs: RNAs attack! Nat Rev Genet 2013; 14:748-9; PMID:24136504; http://dx.doi.org/10.1038/nrg3600
- Tseng TT, Tyler BM, Setubal JC. Protein secretion systems in bacterial-host associations, and their description in the Gene Ontology. BMC Microbiol 2009; 9(Suppl 1):S2; PMID:19278550; http://dx.doi.org/10.1186/1471-2180-9-S1-S2
- Veronese P, Nakagami H, Bluhm B, Abuqamar S, Chen X, Salmeron J, Dietrich RA, Hirt H, Mengiste T. The membrane-anchored BOTRYTIS-INDUCED KINASE1 plays distinct roles in Arabidopsis resistance to necrotrophic and biotrophic pathogens. Plant Cell 2006; 18:257-73; PMID:16339855; http://dx.doi.org/10.1105/tpc.105.035576
- Wang M, Weiberg A, Jin HL. Pathogen small RNAs: a new class of effectors for pathogen attacks. Mol Plant Pathol 2015; 16:219-23; PMID:25764211; http://dx.doi.org/10.1111/mpp.12233
- Wang M, Weiberg A, Lin FM, Thomma BP, Huang HD, Jin H. Bidirectional cross-kingdom RNAi and fungal uptake of external RNAs confer plant protection. Nat Plants 2016; 2:16151; PMID:27643635; http://dx.doi.org/10.1038/nplants.2016.151
- Weiberg A, Wang M, Bellinger M, Jin H. Small RNAs: a new paradigm in plant-microbe interactions. Ann Rev Phytopathol 2014; 52:495-516; PMID:25090478; http://dx.doi.org/10.1146/annurev-phyto-102313-045933
- Weiberg A, Wang M, Lin FM, Zhao H, Zhang Z, Kaloshian I, Huang HD, Jin H. Fungal small RNAs suppress plant immunity by hijacking host RNA interference pathways. Science 2013; 342:118-23; PMID:24092744; http://dx.doi.org/10.1126/science.1239705
- Zhang W, Gao S, Zhou X, Chellappan P, Chen Z, Zhou X, Zhang X, Fromuth N, Coutino G, Coffey M, et al. Bacteria-responsive microRNAs regulate plant innate immunity by modulating plant hormone networks. Plant Mol Biol 2011a; 75:93-105; PMID:21153682; http://dx.doi.org/10.1007/s11103-010-9710-8
- Zhang X, Zhao H, Gao S, Wang WC, Katiyar-Agarwal S, Huang HD, Raikhel N, Jin H. Arabidopsis Argonaute 2 regulates innate immunity via miRNA393( *)-mediated silencing of a Golgi-localized SNARE gene, MEMB12. Mol Cell 2011b; 42:356-66; PMID:21549312; http://dx.doi.org/10.1016/j.molcel.2011.04.010
- Zheng Z, Qamar SA, Chen Z, Mengiste T. Arabidopsis WRKY33 transcription factor is required for resistance to necrotrophic fungal pathogens. Plant J 2006; 48:592-605; PMID:17059405; http://dx.doi.org/10.1111/j.1365-313X.2006.02901.x
- Zipfel C. Plant pattern-recognition receptors. Trends Immunol 2014; 35:345-51; PMID:24946686; http://dx.doi.org/10.1016/j.it.2014.05.004