ABSTRACT
MicroRNAs (miRNAs) are small non-coding RNAs with a wide distribution in nature among the living things. They play a key role both in normal signaling pathways and in pathological ones. Bovine leukemia virus (BLV) is an oncogenic retrovirus of Deltaretrovirus genus causing persistent infection in its natural hosts – cattle, zebu and water buffalo with diverse clinical manifestations through the defeat of B-lymphocytes (B-cells). Ten BLV encoded miRNAs (further miRs-B) transcribed from five different pre-miRNA (further pre-miR-B) genes are abundantly detected in BLV infected B-cells. Here we report about several alleles of each of pre-miRs-B’ genes, some of which have a highly significant association with an increase or a decrease of the number of leukocytes (WBCs – white blood cells) in BLV-infected cows.
Summary
MicroRNAs (miRNAs) are small non-coding RNAs with a wide distribution in nature among the living things. They play a key role both in normal signaling pathways and in pathological ones, through the regulation of protein-coding genes by targeting mRNAs [Citation1–Citation5]. More than 30 000 miRNA have been discovered so far [Citation6]. In pathological pathways, miRNAs can play a significant role in various infectious processes caused by different microorganisms including viruses [Citation7–Citation9]. In the host-virus interaction, we should distinguish host and viral miRNAs, both of which can modulate viral life cycle phases, and in different modes of actions can destroy the normal cell functioning: host miRNAs can promote infection process and viral miRNAs can participate in apoptosis of cells and in evasion of the immune response or even can subvert host immune defenses.
Bovine leukemia virus (BLV) is an oncogenic retrovirus of Deltaretrovirus [Citation10] genus causing persistent infection in its natural hosts – cattle, zebu and water buffalo (Bos taurus, Bos indicus, and Bubalus bubalis, respectively) with diverse clinical manifestations through the defeat of B-lymphocytes (B-cells) [Citation11–Citation13].
Ten miRs-B transcribe from five different pre-miR-B genes and abundantly detected in BLV infected B-cells of both asymptomatic and leukemic stages of BLV infection [Citation14–Citation18]. However, there is no information about a polymorphism of pre-miR-B genes and its connectivity with various stages of BLV caused clinical symptoms.
Here we report about several alleles of each of pre-miRs-B genes, some of which have a highly significant association with an increase or a decrease of WBC counts in BLV-infected cows. We show that the polymorphism of pre-miR-B genes could be responsible for the rise of the WBC counts in BLV-infected cows.
According to the International Committee on Taxonomy of Viruses (ICTV) (2017 Release) BLV have been classified as belonged to Ortervirales order, Retroviridae family, Orthoretrovirinae subfamily, Deltaretrovirus genus with three other species: Primate T-lymphotropic virus 1, Primate T-lymphotropic virus 2 and Primate T-lymphotropic virus 3 (PTLV-1, PTLV-2 and PTLV-3, respectively) (the ICTV taxonomy database website: http://ictv.global/virus Taxonomy.asp) [Citation10]. The two of them, PTLV-1 and PTLV-2, are well known as HTLV-1 and HTLV-2 (Human T-cell lymphotropic virus 1 and Human T-cell lymphotropic virus 2, respectively), which have been renamed. The third, PTLV-3, has been innovated to the classification on ICTV 7th Report in the year 2000 [Citation19].
A paramount strategy of all retrovirus is an integration of viral DNA into the host genome with subsequent tasks of transforming host cells to abnormal ones. BLV has no reason to avoid this strategy. So, it is not surprising that BLV replicates with a relatively low level of viral mRNA in blood of infected animals, and as a consequence of this, there are a small number of viral particles in the blood plasma (the viremia is absent) [Citation20–Citation22]. The consequence of the absence of viremia is a short lifetime of BLV antigens in the blood of infected animals. This situation, in turn, leads to problems with BLV vaccines, which have not been successful enough for a long time, although some authors have been declared that they have received an effective vaccine strain by deletions of presumably harmful genes (tax, G4, and miRs-B) [Citation23].
In contrast to the low level of viral mRNAs, a huge amount of miR-Bs (up to about 40% of total host miRNAs) are expressed in host BLV-infected B-cells [Citation14].
MiRNAs have 20–23 nucleotides in lengths, and can be produced by cellular genes and by genes of cellular pathogens (including viral or proviral ones). Their sequences and their genomic locations are collected into a miRBase catalog (http://www.mirbase.org/) [Citation6]. Cellular miRNAs (or host miRNAs) are the part of the RNA interference (RNAi) pathway. At the beginning of host canonical miRNA biogenesis, a long primary miRNA (pri-miRNA, looked as series of RNA hairpins) are transcribed by RNA polymerase II (RNAP II). Then, pri-miRNA is processed by a nuclear protein complex, which consists of RNase II endonuclease (Drosha) and RNA-binding protein (DCGR8). After the cleavage by Drosha, a stem and loop RNA-structure called pre-miRNA forms. Via a protein Exportin 5, this pre-miRNA is transfered into a cytoplasm from a nucleus with the subsequent processing by RNase III enzyme, Dicer, which cleaves the hairpin into a short miRNA duplex. The last one is loaded into the RNA-Induced Silencing complex (RISC) composed of Ago2 and TRBP (TAR RNA-binding) proteins. In the RISC, one strand becomes a guide strand and further involved in the regulation of the translation and/or degradation of a target mRNA, but the other passenger strand is discarded [Citation24,Citation25].
The biogenesis of viral miRNAs often can occur by a non-canonical way, as it had been described for some viral miRNA, as well for some mammalian ones [Citation26–Citation29].
The evidence of the non-canonical biogenesis pathway for miRs-B has been discovered. Pre-miRs-B are transcribed by RNA polymerase III (RNAP III) from a compact RNAP III type II genes, individually and directly: each pre-miR-B from own pre-miR-B gene. So, there are five genes for ten mature miRs-B in proviral BLV DNA in the intron region () [Citation17,Citation18]. Further, pre-miRs-B are processed by Dicer, so that Drosha processing of its maturation have been skipped [Citation15].
Figure 1. The schematic map of the proviral BLV DNA (GeneBank: KT122858.1) and locations of BLV-MIRS-F and BLV-MIRS-B oligonucleotides. Genes encoding proteins are shown in green, and genes encoding RNAs are shown in blue. Thick lines indicate exons, and thin lines represent introns.
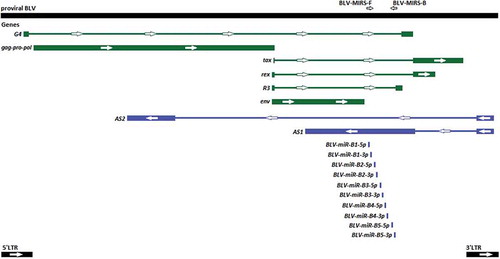
Features of non-canonical biogenesis of viral miRNAs may be a cause of a dysregulation of host miRNA pathways. This has been found for some viruses, including HTLV-1 (PTLV-1) [Citation30], closely related to BLV, with the same homologous genes (tax and rex), which inherent for this Deltaretrovirus genus. Moreover, a direct interaction has been observed between the Tax oncoprotein and Drosha with subsequent degradation mediated by proteasome complex, thereby enabling Drosha’s downregulation [Citation31]. The efficiency of the normal host miRNA production has been decreased, as a consequence of this, but viral miRNA production can be increased. It has been shown for miRs-B, their production can be increased so much, that even BLV proviral DNA has been called as ‘a prodigious producer of viral microRNAs’ with their expression in both asymptomatic and leukemic stages of BLV infection [Citation16]. Furthermore, it has been found, that miR-B4-3p is a functional analog of host miRNA miR-29 and the participation of it in tumorigenesis is debated [Citation15]. MiRs-B also directly or indirectly target some biologically relevant genes, which are related to oncogenesis, or participated in apoptosis, immunity, and cell signaling [Citation32].
Such features of miRs-B may lead us to an assumption that they could play a significant role in a conquest of B-cells cellular resources by BLV. Furthermore, all these data reflect the fact that BLV-infected animals can be divided into three groups with different symptoms of the manifestation: mostly asymptomatic (about 70% animals) or with the disease called Enzootic Bovine Leukosis (EBL). The disease can be mainly split into two types: Persistent Lymphocytosis (PL) (about 30% ones) and B-cell leukemia/lymphoma (lymphosarcoma) (< 5% ones). PL is a usually stable benign form of the disease, feature of which is an increase in absolute WBC (leukocyte) count (leukocytosis) due to abnormal accumulation of immature B-lymphocytes in the peripheral blood. PL may develop into the tumor stage of the disease (B-cell leukemia/lymphoma) or maybe not [Citation11–Citation13].
Herein, we have paid attention to these facts. Briefly, firstly, asymptomatic BLV-infected animals can abundantly express miRs-B, as well as leukemic BLV-infected ones; Secondly, miRs-B can be involved not only in oncogenesis, but also in cell signaling, apoptosis, and immunity; Thirdly, the most BLV-infected animals remain asymptomatic; Fourthly, BLV produces the prodigious amount of miRs-B. Thus, the question has been raised: may the reason for such situation be hidden in a polymorphism of pre-miRs-B’ genes? This question has been reinforced by the fact that for another well-known oncogenic retrovirus HIV, the considerable structural polymorphism of one of the miRNA (miR-H1) isolated from different tissues of different patients with different clinical outcomes of different AIDS-related diseases have been found. Moreover, the potential disease association between a less stable option of miR-H1 and the development of AIDS-related lymphoma (ARL) has been discovered [Citation33]. The polymorphism of host miRNAs in the context of viral diseases also have been intensively reviewed [Citation34].
For investigation of the probability of the polymorphism of pre-miRs-B’ genes we use DNA from 16 samples of the peripheral blood of Black and White Holsteinized cows (farming in Moscow region, Russia). By the time of sampling, cows were from 2 to 5 years old. All 16 samples have been tested for proviral DNA host genome insertion (BLV-positive) and for the presence of antibodies to gp51 BLV antigen by AGID (BLV-seropositive) (further: BLV-positive or BLV+ group). 26 samples from the proviral BLV-negative and the BLV-seronegative group of cows have been taken as the control group (further: BLV-negative or BLV- group). All peripheral blood samples and its WBC counts have been the same as in the previous article [Citation35].
Two primers BLV-MIRS-F and BLV-MIRS-B (5ʹ-GCGCCAGAGATCTACTCTC-3ʹ and 5ʹ-CTGAGCACCCCCTAGTAGAG-3ʹ, respectively) have been designed on the basis of the alignment of 37 complete genomic sequences of BLV from GeneBank [Citation36–Citation44], for one of which (the accession number: KT122858.1) miRs-B had been sequenced and mapped earlier [Citation16] (). Using these primers, ~ 600 bp PCR fragment of five pre-miR-B genes has been cloned with help of CloneJET PCR Cloning Kit (Thermo Scientific™), and further total 86 clones (5–6 clones for each of 16 BLV-positive samples) have been sequenced by Sanger’s sequencing method with subsequent alignment with GeneRunner program.
As the result, several different pre-miRs-B’ alleles have been revealed. We have found that all samples can be divided into two subgroups with different representations of pre-miRs-B’ alleles (().
Table 1. (a). Nucleotide sequences of BLV pre-miR-B alleles. (b). Nucleotide sequences of pre-miR-B alleles and their frequencies of the occurrence in each subgroup (I-st and II-nd) of BLV-positive cows. The significance level of P values is indicated by color: purple – not significant (P pre-miR-B1 = 0.21), pink – highly significant (Ppre-miR-B2 = 0.15 × 10−12, Ppre-miR-B3 = 0.50 × 10−10, Ppre-miR-B4 = 0.59 × 10−15, Ppre-miR-B5 = 0.78 × 10−9). Pre-miR-B alleles highlighted in green have been considered as contributed to a decrease of WBC counts. Pre-miR-B alleles do not highlight have been considered as contributed to increasing of WBC counts. Pre-miR-B alleles identified in both subgroups have been highlighted in yellow. Loop sequences of pre-miRs-B have been underlined.
Firstly, we will focus on the difference in WBC counts between BLV-negative and BLV-positive groups of cows. Gaussian distributions of both groups have been tested, and the difference of WBC counts between BLV-negative and BLV-positive groups has been considered to be extremely significant (P ≤ 0.0001), unpaired t-test results have revealed this (http://www.graphpad.com/quickcalcs/). WBC count reference intervals (i.e., mean ± 2 SD) for BLV-negative and BLV-positive groups have been ranged from 5,906 to 16,609 WBCs/µL and from 4,710 to 29,322 WBCs/µL, respectively (). Our results are consistent with the data of other authors, wherein the correlation of total WBC counts and BLV proviral loads in BLV-infected animals has been investigated [Citation45,Citation46]. It is despite the fact, that apart from BLV infection, the increase of the number of WBCs can be the consequence of inflammation, stress, injury, leukemia, and other infections [Citation47]. In the other research the reference interval for BLV-negative Holstein dairy cows has been a little bit different than ours (2,153 to 11,493 WBCs/µL) [Citation45]. It had been shown earlier that healthy animals from different decades and locations and of different breeds may have markedly different WBC reference intervals, so that WBC counts should be updated for more correct conclusions to which they could lead [Citation47].
Table 2. Unpaired t-test results for groups and subgroups of cows.
Further, the difference of WBC counts between I-st and II-nd BLV-positive subgroups have been also considered to be extremely significant (P ≤ 0.0001) by the unpaired t-test calculation. WBC count reference intervals for I-st and II-nd BLV-positive subgroups have been ranged from 7,948 to 16,196 WBCs/µL and from 12,791 to 31,128 WBCs/µL, respectively (). ANOVA test has been done using GraphPad Prism V.7.04 (1992–2017 GraphPad Software, Inc.) (). We can see from and , that the WBC count for both the I-st BLV-positive subgroup and the BLV-negative group are almost the same: the difference has been considered to be not significant (P = 0.4359). It means that this I-st BLV-positive subgroup of cows remains asymptomatic.
Figure 2. Two-way ANOVA test results for BLV-negative, BLV-, (N = 26) and all BLV-positive, BLV+, (N = 16) groups of cows, and for BLV-positive, I-st BLV+, (N = 8) and II-nd BLV+ (N = 8) subgroups of cows. The number of WBCs (y ˣ 103cells/µL) is on the y-axis.
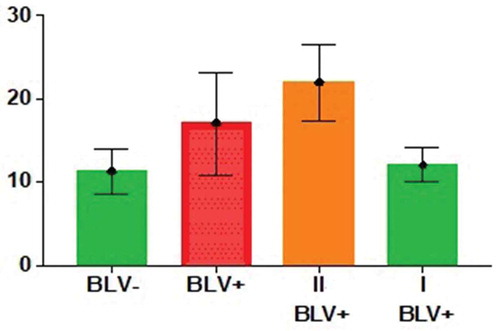
The dividing of BLV-infected animals into two subgroups by WBC values had been noted also in the previous article [Citation35]. The I-st BLV+ subgroup has relatively low WBC counts and the II-nd BLV+ subgroup has relatively high WBC counts. Fisher’s exact test [Citation48] has shown the highly significant (P < 0.01) association of such division of BLV-positive cows into two subgroups and different representations of alleles in each of them for pre-miR-B2, pre-miR-B3, pre-miR-B4 and pre-miR-B5 genes; And not significant for pre-miR-B1 gene (P ≥ 0.05) ((). In the other words, increased or decreased number of WBCs may depend on the presence of those or other pre-miRs-B’ alleles in proviral BLV genome for four from five pre-miR-B (pre-miR-B2, pre-miR-B3, pre-miR-B4 and pre-miR-B5) genes. Namely, pre-miR-B2e, pre-miR-B3d, pre-miR-B4a, pre-miR-B5g, and pre-miR-B5h alleles have been considered contributors to the decrease of the WBC count, but the opposite situation has been observed for pre-miR-B2a and pre-miR-B2d, and for pre-miR-B4j and pre-miR-B4m alleles: their presence may lead to increase of the WBC number. These results allow us to conclude that some alleles of pre-miR-B2, pre-miR-B3, pre-miR-B4, and pre-miR-B5 genes may be the cause of high WBC counts, but some vice versa.
We have received in this work evidences for the polymorphism of pre-miRs-B’ genes, which, moreover, may be related to the change of the WBC counts in BLV-infected cows. Furthermore, summarizing facts reviewed above, we may suppose a significant value of miRs-B as a key to the understanding of precise mechanisms of BLV pathogenicity.
Acknowledgments
We thank I.А. Andreichenko for help in the testing of blood samples for the presence of BLV proviral DNA in cows.
Disclosure statement
No potential conflict of interest was reported by the authors.
References
- Catalonotto C, Cogoni C, Zardo G. MicroRNA in control of gene expression: an overview of nuclear functions. Int J Mol Sci. 2016;17(10):pii: E1712.
- Lenkala D, Gamazon ER, LaCroix B, et al. MicroRNA biogenesis and cellular proliferation. Transl Res. 2015;166(2):145–151.
- Chandra S, Vimal D, Sharma D, et al. Role of miRNAs in development and disease: lessons learned from small organisms. Life Sci. 2017;185:8–14.
- Lin S, Gregory RI. MicroRNA biogenesis pathways in cancer. Nat Rev Cancer. 2017;15(6):321–333.
- Saeidimehr S, Ebrahimi A, Saki N, et al. MicroRNA-based linkage between aging and cancer: from epigenetics view point. Cell J. 2016;18(2):117–126.
- Kozomara A, Griffiths-Jones S. miRBase: annotating high confidence microRNAs using deep sequencing data. Nucleic Acids Res. 2014;42:D68–D73.
- Das K, Garnica O, Dhandayuthapani S. Modulation of host miRNAs by intracellular bacterial pathogens. Front Cell Infect Microbiol. 2016;6:79.
- Bruscella P, Bottini S, Baudesson C, et al. Viruses, and miRNAs: more friends than foes. Front Microbiol. 2017;8:824.
- Tycowski KT, Guo YE, Lee N, et al. Viral noncoding RNAs: more surprises. Genes Dev. 2015;29(6):567–584.
- Lefkowitz EJ, Dempsey DM, Hendrickson RC, et al. Virus taxonomy: the database of the International Committee on Taxonomy of Viruses (ICTV). Nucleic Acids Res. 2018;46(D1):D708–D717.
- Gillet N, Florins A, Boxus M, et al. Mechanisms of leukemogenesis induced by bovine leukemia virus: prospects for novel anti-retroviral therapies in human. Retrovirology. 2007;4:18.
- EFSA Panel on Animal Health and Welfare (AHAW). Enzootic bovine leukosis. Efsa J. 2015;3(7):4188, 63.
- Barez P-Y, de Brogniez A, Carpentier A, et al. Recent advances in BLV research. Viruses. 2015;7(11):6080–6088.
- Rosewick N, Momont M, Durkin K, et al. Deep sequencing reveals abundant noncanonical retroviral microRNAs in B-cell leukemia/lymphoma. Proc Natl Acad Sci USA. 2013;110(6):2306–2311.
- Kincaid RP, Burke JM, Sullivan CS. RNA virus microRNA that mimics a B-cell oncomiR. Proc Natl Acad Sci USA. 2012;109(8):3077–3082.
- Durkin K, Rosewick N, Artesi M, et al. Characterization of novel Bovine Leukemia Virus (BLV) antisense transcripts by deep sequencing reveals constitutive expression in tumors and transcriptional interaction with viral microRNAs. Retrovirology. 2016;13(1):33.
- Burke JM, Bass CR, Kincaid RP, et al. Identification of tri-phosphatase activity in the biogenesis of retroviral microRNAs and RNAP III-gene rated shRNAs. Nucleic Acids Res. 2014;42(22):13949–13962.
- Van Driessche B, Rodari A, Delacourt N, et al. Characterization of new RNA polymerase III and RNA polymerase II transcriptional promoters in the Bovine Leukemia Virus genome. Sci Rep. 2016;6:31125.
- van Regenmortel MHV, Fauquet CM, Bishop DHL, et al. Virus taxonomy. Seventh report of the international committee on taxonomy of viruses. San Diego: Academic Press; 2000. p. 1162.
- Lagarias DM, Radke K. Transcriptional activation of bovine leukemia virus in blood cells from experimentally infected, asymptomatic sheep with latent infections. J Virol. 1989;63:2099–2107.
- Jensen WA, Rovnak J, Cockerell GL. In vivo transcription of the bovine leukemia virus tax/rex region in normal and neoplastic lymphocytes of cattle and sheep. J Virol. 1991;65:2484–2490.
- Radke K, Sigala TJ, Grossman D. Transcription of bovine leukemia virus in peripheral blood cells obtained during early infection in vivo. Microb Pathog. 1992;12:319–331.
- Gutiérrez G, Rodríguez SM, De Brogniez A, et al. Vaccination against δ-Retroviruses: the bovine δ leukemia virus paradigm. Viruses. 2014;6(6):2416–2427.
- O’Brien J, Hayder H, Zayed Y, et al. Overview of MicroRNA biogenesis, mechanisms of actions, and circulation. Front Endocrinol. 2018;9:402.
- Bhat SS, Jarmolowski A, Szweykowska-Kulińska Z. MicroRNA biogenesis: epigenetic modifications as another layer of complexity in the micro RNA expression regulation. Acta Biochim Pol. 2016;63(4):717–723.
- Holley CL, Topkara VK. An introduction to small non-coding RNAs: miRNA and snoRNA. Cardiovasc Drugs Ther. 2011;25(2):151–159.
- Havens MA, Reich AA, Duelli DM, et al. Biogenesis of mammalian microRNAs by a non-canonical processing pathway. Nucleic Acids Res. 2012;40(10):4626–4640.
- Xia J, Zhang W. Noncanonical microRNAs and endogenous siRNAs in lytic infection of murine gammaherpesvirus. PLoS One. 2012;7(10):e47863.
- Cheloufi S, Dos Santos CO, Chong MM, et al. A Dicer-independent miRNA biogenesis pathway that requires Ago catalysis. Nature. 2010;465(7298):584–589.
- Moles R, Nicot R. The emerging role of miRNAs in HTLV-1 infection and ATLL pathogenesis. Viruses. 2015;7(7):4047–4074.
- Van Duyne R, Guendel I, Klase Z, et al. Localization and sub-cellular shuttling of HTLV-1 tax with the miRNA machinery. PLoS One. 2012;7(7):e40662.
- Gillet NA, Hamaidia M, de Brogniez A, et al. Bovine leukemia virus small noncoding RNAs are functional elements that regulate replication and contribute to oncogenesis in vivo. PloS Pathog. 2016;12(4):e1005588.
- Lamers LS, Fogel GB, McGrath MS. HIV-miR-H1 evolvability during HIV pathogenesis. Biosystems. 2010;101(2):88–96.
- Ellwanger JH, Zambra FMB, Guimarães RL, et al. MicroRNA-related polymorphisms in infectious diseases-tiny changes with a huge impact on viral infections and potential clinical applications. Front Immunol. 2018;9:1316.
- Kosovskii GY, Glazko VI, Andreichenko IА, et al. THE INFECTION HAZARD OF CARRIERS OF PROVIRAL BOVINE LEUKEMIA VIRUS AND ITS EVALUATION WITH REGARD TO LEUKOCYTOSIS. Sel’skokhozyaistvennaya biologiya [Agric Biol]. 2016;51(4):475–482.
- Polat M, Takeshima SN, Aida Y. Epidemiology and genetic diversity of bovine leukemia virus. Virol J. 2017;14(1):209.
- Polat M, Takeshima SN, Hosomichi K, et al. A new genotype of bovine leukemia virus in South America identified by NGS-based whole genome sequencing and molecular evolutionary genetic analysis. Retrovirology. 2016;13:4.
- Dube S, Abbott L, Dube DK, et al. The complete genomic sequence of an in vivo low replicating BLV strain. Virol J. 2009;6:120.
- Dube S, Dolcini G, Abbott L, et al. The complete genomic sequence of a BLV strain from a Holstein cow from Argentina. Virology. 2000;277(2):379–386.
- Moratorio G, Fischer S, Bianchi S, et al. A detailed molecular analysis of complete Bovine Leukemia Virus genomes isolated from B-cell lymphosarcomas. Vet Res. 2013;44(1):19.
- Petropoulos C. Retroviral taxonomy, protein structures, sequences, and genetic maps. In: Coffin JM, Hughes SH, Varmus HE, editors. Retroviruses. Cold Spring Harbor (NY): Cold Spring Harbor Laboratory Press; 1997. p. 843.
- Sagata N, Yasunaga T, Tsuzuku-Kawamura J, et al. Complete nucleotide sequence of the genome of bovine leukemia virus: its evolutionary relationship to other retroviruses. Proc Natl Acad Sci USA. 1985;82(3):677–681.
- Derse D, Diniak AJ, Casey JW, et al. Nucleotide sequence and structure of integrated bovine leukemia virus long terminal repeats. Virology. 1985;141(1):162–166.
- Rovnak J, Boyd AL, Casey JW, et al. Pathogenicity of molecularly cloned bovine leukemia virus. J Virol. 1993;67(12):7096–7105.
- Alvarez I, Gutiérrez G, Gammella M, et al. Evaluation of total white blood cell count as a marker for proviral load of bovine leukemia virus in dairy cattle from herds with a high seroprevalence of antibodies against bovine leukemia virus. Am J Vet Res. 2013;74(5):744–749.
- Nishiike M, Haoka M, Doi T, et al. Development of a preliminary diagnostic measure for bovine leukosis in dairy cows using peripheral white blood cell and lymphocyte counts. J Vet Med Sci. 2016;78(7):1145–1151.
- George JW, Snipes J, Lane VM. Comparison of bovine hematology reference intervals from 1957 to 2006. Vet Clin Pathol. 2010;39(2):138–148.
- Weisstein EW. Fisher’s exact test. From MathWorld – A Wolfram Web Resource. Available from: http://mathworld.wolfram.com/FishersExactTest.html