ABSTRACT
Growth hormone (GH), whose synthesis and release are mainly regulated by intracellular signals mediated by growth hormone-releasing hormone receptor (GHRHR), is one of the major pituitary hormones and critical regulators of organism growth, metabolism, and immunoregulation. Pig GHRHR splice variants (SVs) may activate different signalling pathways via the variable C-terminal by alternative splicing, and SVs have the potential to change microRNA (miRNA) binding sites. In this study, we first confirmed the existence of pig GHRHR SVs (i.e., GHRHR, GHRHR SV1 and SV2) and demonstrated the inhibitory effects of critical pituitary miRNAs (i.e., let-7e and miR-328-5p) on GH synthesis and cell proliferation of primary pituitary cells. The SVs of GHRHR targeted by let-7e and miR-328-5p were predicted via bioinformatics analysis and verified by performing dual-luciferase reporter assays and detecting the expression of target transcripts. The differential responses of let-7e, and miR-328-5p to GH-releasing hormone and the changes in signalling pathways mediated by GHRHR suggested that let-7e and miR-328-5p were involved in GH synthesis mediated by GHRHR SVs, indicating that the two miRNAs played different roles by different ways. Finally, results showed that the protein coded by the GHRHR transcript regulated GH through the NO/NOS signalling pathway, whereas that coded by SV1 and SV2 regulated GH through the PKA/CREB signalling pathway, which was confirmed by the changes in signalling pathways after transfecting the expression vectors of GHRHR SVs to GH3 cells. To the best of our knowledge, this paper is the first to report pituitary miRNAs regulate GH synthesis by targeting the different SVs of GHRHR.
Introduction
Growth performance is particularly sensitive to treatment with growth hormone (GH), which increases the average daily weight gain [Citation1–4], enhances protein accretion, and reduces fat deposition [Citation5]. Previous research reported that the collaborations between the neuroendocrine, growth, and immune axes could effectively combat infection [Citation6], and the circulating GH levels would be enhanced during infection or inflammation. Previous studies demonstrated that lipopolysaccharide(LPS) can directly stimulate GH secretion from somatotroph cells in sheep and human [Citation7–10], the results were confirmed in a subpopulation of somatotrophs [Citation6]. A possible explanation for this phenomenon may reside in the response of the pituitary to specific cytokines. In addition, studies on the effects of LPS on pituitary hormone release in prepubertal pigs indicated that systemic levels of GH were increased within minutes after challenge [Citation11,Citation12].
Hypothalamus-derived GH-releasing hormone (GHRH) stimulates pituitary GH synthesis and secretion as well as somatotroph proliferation in mammals [Citation13–15], and these biological actions are mediated by its receptor (i.e., GHRHR) [Citation16–21]. GHRHR and its splice variants (SVs) exist in pituitary and peripheral tissues, implying a physiological or pathophysiological role besides its normal endocrine function [Citation22]. Study indicated that the identical SV of GHRHRβ was present in pituitaries from dwarf rats, which was different with GHRHRα in carboxyl terminal, and the two isoforms maybe involved in multiple intracellular signalling pathways [Citation23]. Chicken cGHRHR-SV1 was characterized by an N-terminal deletion, cGHRHR-SV2 was a carboxyl-terminal truncated variant, while of the two SVs only cGHRHR-SV1 was shown to be capable of transmitting signal upon GHRH stimulation [Citation24]. These findings suggested that the biological actions of target cells were regulated by GHRH response, and the modulation of response may be through receptor isoform interactions and multiple intracellular signalling pathways [Citation25], such as cAMP/PKA [Citation26,Citation27] and NOS/NO/cGMP signalling pathways [Citation28–31]. GHRHR and its SVs respond differently to LPS, for example, GHRHR is increased in the pituitary, whereas its SV (SV1) is downregulated after LPS stimulation [Citation32].
Alternative splicing in eukaryotes involves the removal of introns or the joining of exons in primary transcripts to generate multiple mRNA transcripts from a single gene, thereby increasing transcriptome and proteome diversity. Thus, SVs have the potential to lose or gain microRNA (miRNA) binding sites [Citation33], whereas miRNAs regulate gene expression at the post-transcription level by suppressing translation or degrading their target mRNA [Citation34,Citation35]. Pig GHRHR has complex SVs according to the NCBI and Ensembl databases, and different SVs contain different binding sites for the pituitary miRNAs (i.e., let-7e and miR-328-5p) according to bioinformatics analysis.
Therefore, the aim of this study was to explore how the SVs of GHRHR respond to LPS and ascertain the effect of pituitary miRNAs on the GH synthesis by targeting different SVs of GHRHR, then clarify the molecular mechanism by detecting the signalling pathways changes that the miRNAs involved downstream of GHRHR.
Materials and methods
Animal and cell culture
The animal experiments were conducted according to the Review of Welfare and Ethics of Laboratory Animals guidelines approved by the Guangdong Province Administration Office of Laboratory Animals, and all experiments were conducted as described in the animal protocol (SCAU-AEC-2010-0416) approved by the Institutional Animal Care and Use Committee of South China Agricultural University. Three 7-day-old female Landrace pigs were collected to isolate primary pituitary cells according to the previous reports [Citation33,Citation34]. Briefly, the collected pituitaries were washed in PBS three times. On a clean bench, anterior pituitaries were minced into small pieces and digested at 37°C for 30 min in the following digestive reagent: 0.25% trypsin-EDTA (Gibco, USA), 0.1 mg/mL collagenase type II (Sigma, Japan). The digestion was terminated with F12K medium (Gibco, USA) containing 10% (v/v) foetal bovine serum (FBS) (Gibco, USA). To remove cell debris, cells were filtrated through 75-mm nylon filters (200 meshes) and harvested by centrifugation at 1, 000 rpm for 5 min. After removal of the supernatant, cells were washed in F12K medium twice and re-suspended with F12K medium containing 2.5% FBS, 15% horse serum (Solarbio, China) and 1% penicillin/streptomycin (Gibco, USA). HEK293T cells used in this study were cultured in DMEM (Hyclone, China) supplemented with 10% FBS and 1% penicillin/streptomycin.
Lipopolysaccharide (LPS) and growth hormone-releasing hormone (GHRH) treatments
Pig primary pituitary cells at 80%–90% confluence were serum starved for 6 h in F12K medium supplemented with antibiotics and incubated for 48 h in fresh starvation medium supplemented with 0.5 μg/mL LPS [Citation36–38] and 10 nmol/L GHRH [Citation39,Citation40]. The cells were then collected for GH, GHRHR SVs, and miRNA expression detection.
miRNA transfection
All RNA oligonucleotides used in this study, including let-7e mimic, miR-328-5p mimic, let-7e inhibitor, miR-328-5p inhibitor, and negative controls, were obtained from Genepharma Co., Ltd. (Suzhou, China), and their sequences are shown in . The oligonucleotides were transfected using LipoPlus™ Reagent (Sage Creation, China) according to the manufacturer’s protocol with at least three replications. Primary pituitary cells were seeded in a 6 cm cell culture dish and serum starved for 6 h in F12K medium to confirm that the response of GHRHR SVs and GH to GHRH are indeed linked to let-7e and miR-328-5p. Then, 400 pM let-7e and miR-328-5p mimics or inhibitors were transfected, and the culture medium was replaced by serum-free F12K medium containing 10 nmol/L GHRH 6 h after transfection. The cells were collected for RNA and protein extraction 48 h later.
Table 1. Sequences of the miRNA oligonucleotides
Cell viability assay
Cell proliferation was measured by the Cell Counting Kit-8 (Apexbio, USA) assay according to the introduction of the kit. Briefly, the primary pituitary cells were plated into 96-well plates at a density of 5, 000 cells/well with 100 μL culture medium. Then the cells were incubated for 30 min with 10 μL CCK-8 reagent at 37 °C after transfected with let-7e mimic, miR-328-5p mimic, let-7e inhibitor, miR-328-5p inhibitor and negative controls for 0, 24, 48, 72 and 96 h. The number of cells in triplicate wells was measured using absorbance at 450 nm by a 96-well microplate reader (TECAN, Switzerland).
Bioinformatics analysis
The target transcripts prediction of the let-7e and miR-328-5p were conducted through TargetScan (http://www.targetscan.org) and miRBase (http://mirbase.org). According to NCBI annotation, the pig GHRHR gene contains 14 exons that form three transcripts by variable splicing (). The three transcripts share the 5´-end 12 exons and exhibit differences at the 3´-end. In detail, the main transcript (GHRHR) is formed by the connection of exons 12 and 13, GHRHR SV1 is formed by the connection of exons 12 and 14, and GHRHR SV2 contains all 14 exons. The predicted secondary structures of let-7e and miR-328-5p binding to the 3´UTR of different GHRHR SVs were generated using mfold (http://unafold.rna.albany.edu), and the MFE values were predicted by RNAhybrid.
Plasmid construction and transfection
In order to confirm the miRNAs targeting the predicted transcripts, the 3´UTR sequences of GHRHR transcripts were synthesized and inserted into a psiCHECKTM-2 vector (Promega, USA) to construct the GHRHR-3´UTR and GHRHR-SV1/2-3´UTR vectors, respectively.
To confirm which of the intracellular signalling pathway that the multiple GHRHR SVs involved, the three transcripts of GHRHR gene were inserted to the pcDNA3.1(+) vector to construct the pcDNA3.1-GHRHR, pcDNA3.1-SV1 and pcDNA3.1-SV2 vectors. Then 6 μg of each recombinant vector was transfected to a 6-cm-dish of GH3 cells. 48 h later, the cells were collected for RNA and protein extraction.
Dual-luciferase reporter assay
HEK-293T cells (1 × 104 cells per well) were seeded in 96-well culture plates and cotransfected with 200 ng GHRHR-3´UTR or GHRHR-SV1/2-3´UTR vectors and miRNA mimics or NC (10 pM) using LipoPlus™ Reagent (Sage creation, China) for 48 h. The activities of the Renilla and firefly luciferases were determined using a Dual-Luciferase Reporter Assay System (TECAN, Switzerland), and the activity of renilla luciferase was normalized to the activity of firefly luciferase according to the manufacturer’s recommendations.
RNA isolation, cDNA synthesis, RT-PCR and qRT-PCR analysis
Total RNA was isolated from primary pituitary cells after transfecting let-7e, miR-328-5p mimics or inhibitors and GH3 cells after transfecting pcDNA3.1 constructed vectors using RNAiso Plus (Takara, Japan). The cDNAs were obtained by PrimeScript RT Reagent Kit (Takara, Japan) with gDNA Eraser from 500 ng of total RNA. The reverse transcription reaction was performed under the introductions of the kit, oligodT primer was used for mRNA reverse transcription and the specific reverse primers of let-7e (GTCGTATCCAGTGCGTGTCGTGGAGTCGGCAATTGCACTGGATACGACAACTA
TAC), miR-328-5p (GTCGTATCCAGTGCGTGTCGTGGAGTCGGCAATTGCACTGG
ATACGACCCCTGAGC) and U6 (AACGCTTCACGAATTTGCG) were used for miRNA reverse transcription. RT-PCR reactions were used to detect the expression of GHRHR in GH3 cells transfected with pcDNA3.1 constructed vectors and qRT-PCR reactions were carried out in a Real-Time PCR Systems ABI PRISM 7900 (Applied Biosystems, USA) with SYBR Green Supermix Kit (Takara, Japan). The 2−ΔCt method was employed to quantify and normalize the expression data. The primers used for qRT-PCR were also listed in .
Table 2. Primer pairs used for RT-PCR and qRT-PCR reactions
Western blot analysis
The protein contents were quantified under the guidance of the Bradford Protein Assay Kit (Beyotime, China). The total protein (40 μg for each) of the cell lysates was first separated on 12% or 15% (for GH and GHRHR detection) SDS-PAGE gels and transferred to polyvinylidene difluoride membranes, then blocked in 10% non-fat dry milk for 1.5 h at 37°C followed by the incubation of primary antibodies at 4°C for 12 h. The primary antibodies used in this study were as follows: GH monoclonal antibody (sc-374266, Santa Cruz, USA), GHRHR polyclonal antibody (BS5732, Bioworld, USA), PKA polyclonal antibody (YT3749, Immunoway, USA), CREB polyclonal antibody (YT1097, Immunoway, USA), phospho-CREB (Ser133) polyclonal antibody (YP0075, Immunoway, USA), nNOS monoclonal antibody (BS90953, Bioworld, USA), and β-actin polyclonal antibody (D110001, BBI, China). The HRP-conjugated goat anti-rabbit IgG (BS13278; Bioworld, USA) and HRP-conjugated goat anti-mouse IgG (ab6789, Abcam, England) were used as secondary antibodies. Supersignal HRP Chemiluminescent Substrates (Thermo Fisher Scientific, USA) was used for protein detection according to the manufacturer’s instructions. All samples were performed in triplicate, and each protein quantification represents an average of at least three measurements. The expression amount of each target gene was obtained by GenoSens Analysis (Clinx Science Instruments, China), the relative amounts were corrected by the corresponding β-actin amount.
Quantification of secretory GH by ELISA
The concentration of GH in cell medium of the primary pituitary cells transfected with let-7e and miR-328-5p mimics and inhibitors or GHRH treatment were determined using the Growth Hormone ELISA kit (KRC5311, Invitrogen, USA) according to the ultra-sensitive assay procedure. The medium and standards were incubated in antibody-coated plates for 2 h at room temperature, then incubated the washed wells with GH Biotin Conjugate for 1 h and the HRP conjugates were subsequently added to all wells, followed by incubation for 30 min at room temperature on the shaker. The reaction was terminated by the addition of 50 μL of stop solution. Colour alterations in the wells were read using the 96-well microplate reader (TECAN, Switzerland) at 450 nm.
Measurement of NO production
Briefly, the primary pituitary cells were plated into 96-well plates at a density of 5, 000 cells/well with 100 μL culture medium. Then the supernatant was collected and the cells were splitted by RIPA lysis buffer after transfecting with let-7e mimic, miR-328-5p mimic, let-7e inhibitor, miR-328-5p inhibitor and negative controls. NO levels of the cell lysis and supernatant were estimated using a commercially available kit according to the manufacturer’s instructions (Beyotime, China). Briefly, 50 µL of supernatant or cell lysis were mixed with 100 µL of Griess reagent in a 96-well plate. Optical density was determined in a 96-well microplate reader (TECAN, Switzerland) at 540 nm to form a standard curve (0–100 µM) derived from NaNO2. Each experiment was performed in triplicate.
Statistics analysis
All experimental results are presented as mean ± S.E.M, with at least three independent replications. Statistical analysis was performed by SPSS 17.0 software. The statistically differences among groups were tested by one-way analysis of variance (ANOVA). p < 0.05 was considered to be statistically significant. *p < 0.05, ** p < 0.01.
Results
Effect of LPS onGHRHR SVs and GH expression
GH plays an important role in cell immunity, whereas cytokines involved in inflammatory responses to LPS also promote GH synthesis [Citation11,Citation12]. GHRHR, an important receptor in GH synthesis, is also reported to be stimulated by LPS. According to the NCBI database, pig GHRHR gene has three potential transcripts (i.e., GHRHR, GHRHR SV1, and GHRHR SV2) formed by alternative splicing. In this study, the three potential transcripts of GHRHR were first identified by RT–PCR using specific primers (). The response of GHRHR SVs and GH to LPS showed that GH1 and the transcripts of GHRHR were significantly enhanced (p < 0.05; ), as well as the protein levels of GH and GHRHR(p < 0.05; and ). Moreover, GHRHR SV1 and SV2 were reduced in the mRNA level under the stimulation of LPS (), and the coding protein level of GHRHR SV2 was sharply reduced in the LPS group compared with that in the NC group (p < 0.05; and ). The level of the coding protein of GHRHR SV1 was too low to detect. In addition, the expression levels of let-7e and miR-328-5p both increased under the stimulation of LPS ().
Figure 1. GH and GHRHR SVs expression analysis in primary pituitary cells after LPS treatment. (A) Relative GHRHR SVs and GH1 mRNA expression ratio of LPS-treated cells versus the corresponding control cells by qRT-PCR. (B) Identification of GHRHR SVs in pig primary pituitary cells. M represents Marker DL2000; 1, 2, 3 represent the transcripts of GHRHR, GHRHR SV2 and GHRHR SV1, respectively. (C) The protein expression ratio of GHRHR SVs and GH in LPS-treated cells versus the control cells by western blot detection. (D) The relative intensity ratios obtained from (C). (E) let-7e and miR-328-5p expression ratio in LPS-treated cells versus the control cells. * p < 0.05; ** p < 0.01
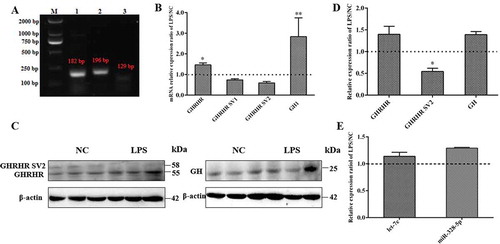
Let-7e and miR-328-5p inhibited pituitary cell proliferation and reduced GH expression
Based on our previous study, the expression levels of let-7e and miR-328-5p were significantly higher in the pituitary of 20-day-old female Bama Xiang miniature pigs than those in Landrace pigs (p < 0.01; ) [Citation41]. Through bioinformatics analysis, another study suggested that they may participate in the regulation of pituitary function and GH [Citation40]. Thus, let-7e and miR-328-5p were first overexpressed in the primary pituitary cells and then cell proliferation and GH synthesis were detected. Results demonstrated that let-7e and miR-328-5p were successfully transfected into the primary pituitary cells (), and cell proliferation was significantly inhibited by both let-7e and miR-328-5p (p < 0.05; ). In addition, GH1 expression was reduced by let-7e and miR-328-5p in mRNA level (p < 0.05; ) as well as the GH protein level (), but it was counteracted by the inhibitors of let-7e and miR-328-5p (). Moreover, the release of GH was significantly reduced by let-7e and miR-328-5p (p < 0.05; ).
Figure 2. Differentially expressed pituitary miRNAs inhibited proliferation and GH synthesis of primary pituitary cells. (A) let-7e and miR-328-5p were highly expressed and differently expressed between the pig breeds with different growth performance. (B) Detection of the transfection efficiency of miRNAs in primary pituitary cells by fluorescence microscopy. (C) Detection of the transfection efficiency of let-7e and miR-328-5p mimics or inhibitors in primary pituitary cells by qRT-PCR. (D, E) The proliferation of primary pituitary cells transfected with let-7e and miR-328-5p mimics (D) or inhibitors (E). (F) Relative GH1 mRNA expression in primary pituitary cells transfected with let-7e and miR-328-5p mimics or inhibitors. (G) GH protein expression in primary pituitary cells transfected with let-7e and miR-328-5p mimics or inhibitors detected by western blot. (H) The released GH determined by ELISA in the cell medium after the transfection of let-7e and miR-328-5p mimics and inhibitors. * p < 0.05; ** p < 0.01
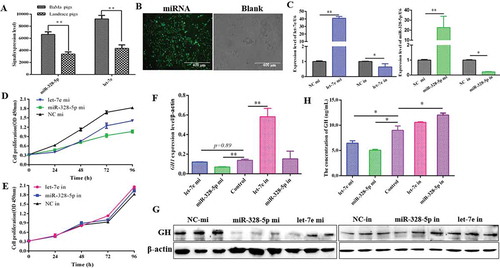
Let-7e and miR-328-5p downregulated the expression levels of different GHRHR SVs
According to the NCBI database, 3ʹUTR has two different types because GHRHR and GHRHR SV1/SV2 possess different 3ʹ-end exons that may have the potential to change miRNA binding sites for GHRHR transcripts to regulate GHRHR expression. let-7e was predicted to target GHRHR, whereas miR-328-5p may target GHRHR, GHRHR SV1, and GHRHR SV2 (). The effects of let-7e and miR-328-5p on GHRHR SVs were further tested. Results demonstrated that let-7e and miR-328-5p both significantly reduced the total GHRHR expression in mRNA () and protein levels (p < 0.05; and ), but it was counteracted by the inhibitors of let-7e and miR-328-5p (, and ). let-7e only contributed to the GHRHR transcript and its coding protein (), whereas miR-328-5p contributed to all three SVs (–) and their coding proteins ( and ). The protein level of GHRHR coded by GHRHR SV1 was too low to be detected.
Figure 3. Let-7e and miR-328-5p reduced GHRHR SVs and GH expression. (A) The exons composition models of GHRHR SVs and the potential targets of let-7e and miR-328-5p. (B) Total GHRHR mRNA expression level in primary pituitary cells transfected with let-7e and miR-328-5p mimics or inhibitors. (C) GHRHR mRNA expression level. (D) Relative GHRHR SV1 mRNA expression level. (E) Relative GHRHR SV2 mRNA expression level. (F) GHRHR SVs protein expression in primary pituitary cells transfected with let-7e and miR-328-5p mimics or inhibitors. (G, H) Quantification results of (F). * p < 0.05; ** p < 0.01
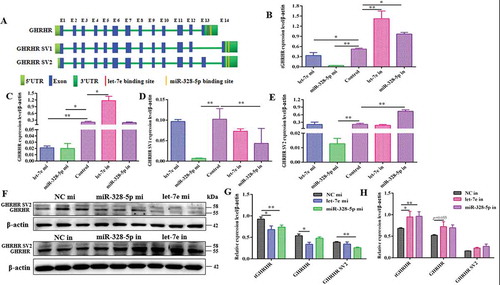
Let-7e targeted GHRHR, whereas miR-328-5p targeted GHRHR and GHRHRSVs
RNAHybrid database was used to predict the binding sites between the 3ʹUTRs of GHRHR SVs and seed sequences of let-7e or miR-328-5p () by offering minimum free energy of hybridization to predict whether the GHRHR SVs are the direct target transcripts of let-7e and miR-328-5p. The corresponding minimum free energy of GHRHR with let-7e was 22.7 kcal/mol, and that of GHRHR with miR-328-5p was 40.7 kcal/mol, besides, two binding sites were present between SV1/SV2 and miR-328-5p. The minimum free energies were 37.8 and 34.5 kcal/mol, respectively (). In order to confirm whether the GHRHR SVs are the direct target transcripts of let-7e and miR-328-5p, we cloned the putative 3ʹUTR target sites to the downstream of a luciferase reporter gene of the psiCHECKTM-2 vector () to construct GHRHR 3ʹUTR-WT/GHRHR3ʹUTR-MUT/GHRHR 3ʹUTR-DEL and GHRHR SV1/2 3ʹUTR-WT/GHRHR SV1/2 3ʹUTR-MUT/GHRHR SV1/2 3ʹUTR-DEL vectors. We transfected the recombinant vectors and let-7e or miR-328-5p (mimics or NC) into HEK-293 T cells. Results showed that the relative luciferase activity of the cells cotransfected with let-7e and GHRHR 3´UTR-WT vector significantly decreased compared with that of the cells cotransfected with NC and GHRHR 3ʹUTR-WT vector (p < 0.05; ). The luciferase activity of the cells cotransfected with miR-328-5p and GHRHR 3ʹUTR-WT vector obtained the same result. The relative luciferase activity of the cell cotransfected with miR-328-5p and GHRHR SV1/2-WT vector significantly decreased (p < 0.05; ). These results demonstrated that GHRHR was the direct target transcript of let-7e and miR-328-5p. Moreover, miR-328-5p also targeted GHRHR SV1 and SV2.
Figure 4. Confirmation of the target transcripts for let-7e and miR-328-5p. (A) The seed regions of let-7e or miR-328-5p and the seed-recognizing sites in the 3ʹUTR (WT, MT and DEL) of the potential target transcripts and the seed-recognizing sites were marked in red. (B) Predicted secondary structure and minimum free energy of let-7e or miR-328-5p for binding to the 3ʹUTR of the potential target transcripts generated by mfold and RNAHybrid. (C) Schematic of inserted GHRHR SVs 3´UTR sequences into the psiCHECKTM-2 vector. (D) Detection of the transfection efficiency of miRNAs in HEK-293T cells by fluorescence microscopy. (E) Relative luciferase activities detection after the HEK-293T cells were cotransfected with constructed psiCHECKTM-2 plasmids and let-7e/miR-328-5p mimics or negative control (NC). ** p < 0.01
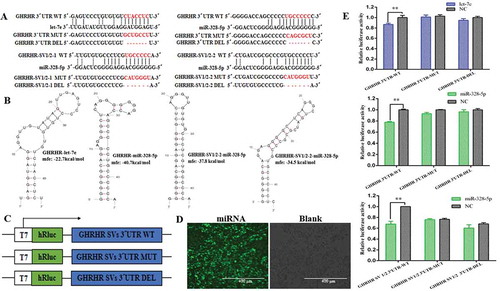
Let-7e and miR-328-5p were both involved in GHRH-induced GH synthesis
GHRH reportedly stimulate GH release by binding to GHRHR. The response of let-7e and miR-328-5p to GHRH was detected to further confirm that let-7e and miR-328-5p inhibit pituitary GH synthesis by targeting GHRHR SVs. Briefly, 10 nmol/L GHRH was treated to the primary pituitary cells. Results showed that the GHRH treatment significantly increased GH1 and GHRHR SVs mRNA levels (p < 0.05; ), along with the coding protein (p < 0.05; and ) and GH secretion levels (p < 0.05; ). GHRH significantly increased let-7e expression (p < 0.05) and significantly decreased miR-328-5p expression (p < 0.05; ).
Figure 5. Responses of GHRHR SVs, GH, let-7e and miR-328-5p to GHRH. (A) The mRNA level of GH1 and GHRHR SVs in primary pituitary cells after being treated with GHRH for 48 h. (B, C) The protein level of GH and GHRHR SVs after being treated with GHRH for 48 h. (D) The released GH was determined by ELISA in the cell medium after being treated with GHRH. (E) The expression level of let-7e and miR-328-5p in primary pituitary cells after being treated with GHRH. * p < 0.05; ** p < 0.01
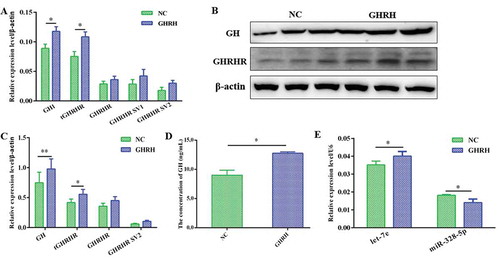
The recovery effect of GHRH on the response of GH and GHRHR SVs to let-7e and miR-328-5p was further detected to confirm that the expressions of GHRHR SVs and GH response to GHRH are indeed linked to let-7e and miR-328-5p. Results indicated that let-7e was significantly induced in the let-7e mi+GHRH group compared with the let-7e mi group (p < 0.05; ), whereas the expression levels of GHRHR (GHRHR) and GH (GH1) decreased in the let-7e mi+GHRH group ( and ). On the contrary, miR-328-5p significantly decreased in the miR-328-5p mi+GHRH group compared with the miR-328-5p mi group (p < 0.05; ), whereas the expression levels of GHRHR SVs (GHRHR SVs) and GH (GH1) increased in the miR-328-5p mi+GHRH group ( and ).
Figure 6. Expression of GH and GHRHR SVs response to GHRH indeed related to let-7e and miR-328-5p. (A) let-7e and miR-328-5p expression level under the transfection of let-7e and miR-328-5p mimics or inhibitors followed by the stimulation of GHRH. (B) The mRNA level of GH1 and GHRHR SVs in cells transfected with let-7e mimic or inhibitor followed by the stimulation of GHRH. (C) The mRNA level of GH1 and GHRHR SVs in cells transfected with miR-328-5p mimic or inhibitor followed by the stimulation of GHRH. (D) The protein level of GH and GHRHR SVs in cells transfected with let-7e and miR-328-5p mimics or inhibitors followed by the stimulation of GHRH. * * p < 0.05
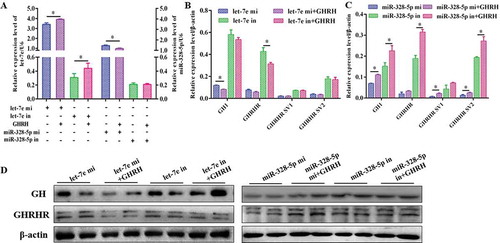
Let-7e and miR-328-5p were involved in the different signalling pathways that GHRHR mediated
The possible signalling pathways (i.e., PKA/CREB and NOS/NO signalling pathways) were investigated to investigate how let-7e and miR-328-5p mediate GH synthesis and secretion after inhibiting different GHRHR SVs. Results demonstrated that let-7e overexpression obviously reduced nNOS expression () and NO production () compared with the NC group (p < 0.05), whereas miR-328-5p sharply decreased PKA and phosphorylated-CREB expression compared with the NC group (p < 0.05; and ), which could be counteracted by the inhibitors ( and ).
Figure 7. Effect of let-7e and miR-328-5p on the related signal pathways. (A) Western blot evaluation results of PKA and p-CREB protein expression following transfection with let-7e and miR-328-5p mimics or inhibitors, respectively. (B) Western blot evaluation results of nNOS protein expression following transfection with let-7e and miR-328-5p mimics or inhibitors, respectively. (C, D) Quantification of the western blot results for (A) and (B), which were shown as mean ± S.E.M. and the data were representative of at least three independent assays. (E) NO production of the primary pituitary cells after being transfected with let-7e and miR-328-5p mimics or inhibitors, respectively. * p < 0.05; ** p < 0.01
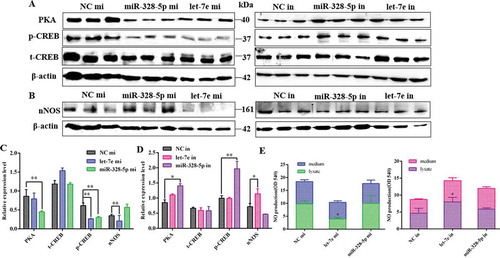
GHRHR SVs activated different signalling pathways in regulating GH synthesis
On the basis of the influence of let-7e and miR-328-5p on the downstream pathway mediated by GHRHR SVs, we assumed that the protein coded by different GHRHR SVs may regulate GH through different signalling pathways. We first compared the amino acid sequences of GHRHR encoded by the three transcripts and found significant differences at the C-terminal, especially the potential phosphorylation sites (). The expression vectors of the three GHRHR transcripts were constructed () and transfected to GHRHR-free GH3 cells. Preliminary results demonstrated that GHRHR encoded by the three GHRHR SVs were all expressed in the GH3 cells, and GH significantly increased in the GH3 cells transfected with the expression vectors of the three GHRHR transcripts (–).
Figure 8. Construction of GHRHR SVs expression vectors and expression detection of GHRHR SVs after being transfected with constructed vectors. (A) Amino acid sequences alignment of protein coded by GHRHR SVs, and the phosphorylation sites at the differential C-terminals have been marked with asterisks. (B) The GHRHR SVs fragmentswere inserted into the pcDNA3.1 vector between EcoRI site and XhoI site. (C) The RT-PCR results of the GH3 cells transfected with pcDNA3.1-GHRHR, pcDNA3.1-SV1, pcDNA3.1-SV2 and pcDNA3.1 vectors. (D) The relative mRNA expression of GHRHR and GH1 in GH3 cells transfected with pcDNA3.1-GHRHR, pcDNA3.1-SV1, pcDNA3.1-SV2 and pcDNA3.1 vectors. (E) GHRHR and GH protein level detection of GH3 cells transfected with pcDNA3.1-GHRHR, pcDNA3.1-SV1, pcDNA3.1-SV2 and pcDNA3.1 vectors. * p < 0.05; ** p < 0.01
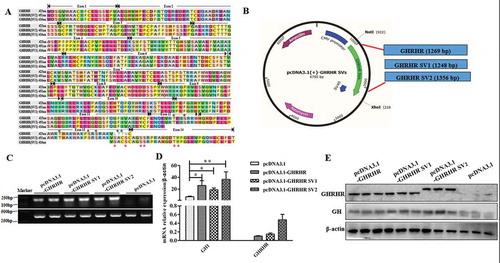
Furthermore, the results of signal pathway detection showed that the production of nNOS ( and ) and NO () in the GH3 cells transfected with the GHRHR expression vectors was higher than that in the control group (p < 0.05), whereas the contents of PKA and phosphorylated-CREB in the cells transfected with GHRHR SV1 and SV2 significantly increased (p < 0.05; and ). These results confirmed our conjecture.
Figure 9. Effect of the GHRHR SVs on the signal pathways downstream of GHRHR. (A) Western blot evaluation results of PKA, phosphorylated-CREB and nNOS protein expression following transfection with pcDNA3.1-GHRHR, pcDNA3.1-SV1, pcDNA3.1-SV2 and pcDNA3.1 vectors in GH3 cells. (B) Quantification of the expression levels of PKA, phosphorylated-CREB and nNOS corrected by GHRHR. (C) NO production of GH3 cells after being transfected with pcDNA3.1-GHRHR, pcDNA3.1-SV1, pcDNA3.1-SV2 and pcDNA3.1 vectors. * p < 0.05; ** p < 0.01
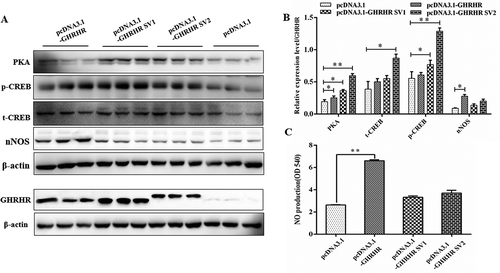
Discussion
GHRHR is a G protein-coupled receptor (GPCR) whose signalling has been reviewed extensively [Citation42–44]. Briefly, GPCRs undergo a conformational change that triggers their association with the heterotrimeric (i.e., subunit α, β, and γ) G proteins located in the plasma membraneupon binding to an external ligand, thereby activating the intracellular signalling pathways. The reported types of G protein are presently Gs, Gi, Gt, Go, and Gq, of which the Go-type G protein coupled to GHRHR can mediate cGMP phototransduction [Citation45]. Therefore, we suspected that different pig GHRHR SVs may activate different signalling pathways when coupling to different types of G proteins due to thevariable C-terminal of GHRHR SVs by alternative splicing, which in turn expose different phosphorylation sites.
GH is one of the major pituitary hormones and critical regulators of organism growth and metabolism, and it is also needed for optimal immune cell function. Circulating GH levels are enhanced during infection or inflammation. LPS and LPS-induced cytokines can directly stimulate GH secretion from somatotroph cells [Citation44–46]. GHRHR, as the most important GPCR in regulating GH release, is reportedly stimulated by LPS. In addition, the SVs of GHRHR are regulated by LPS [Citation32]. In several preliminary studies, we first examined the response of GHRHR SVs and GH to LPS. We confirmed that LPS induced GH and GHRHR expression but reduced GHRHR SV1 and SV2 expressions. These results were basically consistent with those of a previous study [Citation32]. The findings indicated that the different GHRHR SVs would display distinct expression levels under the stimulation of certain factors. In this case, whether the other factors in the cell, such as miRNA, will mediate GH synthesis by regulating GHRHR SVs is the next issue we are concerned with [Citation47,Citation48].
miRNAs have recently emerged as key regulators that participate in pituitary and GH regulation [Citation49–51]. Through bioinformatics analysis, we compared in a previous research the abundance of miRNAs in the pituitary of Landrace and Bama Xiang miniature pigs and enriched the miRNAs involved in regulating the synthesis of pituitary GH. We found that let-7e and miR-328-5p were highly and differently expressed in the pituitary of Landrace (large pigs) and Bama Xiang miniature pigs (miniature pigs) [Citation40]. The two breeds have different growth rates and body size. Bama Xiang miniature pigs are an excellent Chinese miniature pig breed with a slower growth rate and a smaller body size [Citation52] than Landrace pigs [Citation53]. let-7e and miR-328-5p significantly inhibited GH expression and proliferation of the primary pituitary cells. As we continued to trace the mechanism of GH inhibition, we found that let-7e and miR-328-5p inhibited the expression of GHRHR SVs by targeting different GHRHR SVs. Our conclusion was verified via dual-luciferase reporter assays. A previous study suggested that let-7 miRNA members in the pituitary play an important role in regulating the growth of fish by negatively regulating the expression of their target genes [Citation54]. However, the function of pituitary miR-328-5p has not been elucidated yet.
GH regulation mediated by GHRHR in pituitary cells mainly depends on the binding of the ligand, GHRH, which is secreted by the hypothalamus. We further demonstrated the changes in GHRHR SVs and GH under the action of GHRH, as well as the response of let-7e and miR-328-5p to GHRH, to further confirm whether let-7e and miR-328-5p are involved in GH synthesis mediated by GHRHR. Consistent with the expected results, GHRH promoted the expression of GH and GHRHR SVs. However, the two miRNAs exhibited an opposite response. let-7e significantly increased under the action of GHRH, whereas miR-328-5p significantly decreased at high GH expression. This result was confirmed by the recovery effect of GHRH on the response of GH and GHRHR SVs to let-7e and miR-328-5p. We propose two hypotheses to explain these results. First, the response of miRNAs to the regulators may depend on acting time and dose [Citation55]. Second, let-7e plays a major inhibitory role in regulating GH synthesis, whereas miR-328-5p maintains the dynamic balance.
let-7e and miR-328-5p both inhibited GH expression and regulated GHRHR by targeting different GHRHR SVs, which own the distinct C-terminaland may lead to different activations of downstream pathways. GHRHR, as a GPCR, how to activate intracellular signal transduction is determined by coupled G proteins and their activated effectors [Citation56]. GHRHR with different C-terminals binds and activates different types of G proteins, thereby activating different intracellular signaling pathways. For example, GHRHR can mediate the cAMP/PKA/CREB signalling pathway when it couples to Gs-type G protein [Citation28], whereas it can activate the NOS/NO/cGMP signalling pathway when it couples to Go-type G protein [Citation31]. According to our results, let-7e was involved in the NOS/NO signalling pathway, and miR-328-5p contributed more to the PKA/CREB signalling pathway. Therefore, we conjectured that the protein coded by the GHRHR transcript regulates GH through the NOS/NO signalling pathway, whereas the GHRHR coded by GHRHR SV1 and SV2 regulates GH through the PKA/CREB signalling pathway.
We constructed the expression vectors of three transcripts and detected the changes in the signalling pathways in GHRHR-free GH3 cells, and our preliminary results confirmed our conjecture. Meanwhile, we detected the difference in molecular weights between GHRHR and GHRHR SV2 proteins but failed to separate GHRHR and GHRHR SV1 proteins because of the minute molecular weight difference and the low expression amount of GHRHR SV1. To the best of our knowledge, this study is the first to report on the regulation mode of GH by pig GHRHR SVs.
In summary, this study addressed how GHRHR SVs respond to LPS, ascertained the effect of critical pituitary miRNAs (i.e., let-7e and miR-328-5p) on GH synthesis by targeting different SVs of GHRHR, and further clarified the molecular mechanism by detecting the changes in signalling pathways that the miRNAs involved undergo downstream of GHRHR and by exploring the different signalling pathways mediated by the coding protein of GHRHR SVs.
Abbreviations
GH, growth hormone; SV, splice variants; GHRHR, growth hormone releasing hormone receptor; miRNA, microRNA; LPS, lipopolysaccharide; FBS, foetal bovine serum; NC, negative control; cAMP, cyclic adenosine monophosphate; CREB, cAMP response element binding protein; PKA, protein kinase A; NCBI, National Coalition Building Institute; MFE, minimum free energy; PCR, polymerase chain reaction; qRT-PCR, quantificational real-time polymerase chain reaction; 3´UTR, 3´untranslated regions. GPCR, G-protein coupled receptor; cGMP, cyclic guanosine monophosphate; NOS, nitric oxide synthase.
Acknowledgments
This work was supported by National Key Research and Development Program of China (2016YFD0500503, 2016YFD0501205), the Natural Science Foundation of China Program (No. 31672514, 31772699, 31802156, 31872435), and Project funded by China Postdoctoral Science Foundation (2018M643106).
Disclosure statement
We declare that we have no financial and personal relationships with other people or organizations that can inappropriately influence our work, there is no professional or other personal interest of any nature or kind in any product, service and/or company that could be construed as influencing the position presented in the manuscript entitled.
Additional information
Funding
References
- Etherton TD, Wiggins JP, Chung CS, et al. Stimulation of pig growth performance by porcine growth hormone and growth hormone-releasing factor. J Anim Sci. 1986;63(5):1389–1399.
- Etherton TD, Wiggins JP, Evock CM, et al. Stimulation of pig growth performance by porcine growth hormone: determination of the dose-response relationship. J Anim Sci. 1987;64(2):433–443.
- Campbell RG, Steele NC, Caperna TJ, et al. Interrelationships between energy intake and endogenous porcine growth hormone administration on the performance, body composition and protein and energy metabolism of growing pigs weighing 25 to 55 kilograms live weight. J Anim Sci. 1988;66(7):1643–1655.
- Klindt J, Buonomo FC, Yen JT, et al. Administration of porcine somatotropin by daily injection: growth and endocrine responses in genetically lean and obese barrows and gilts. J Anim Sci. 1995;73(11):3294.
- Campbell RG, Steele NC, Caperna TJ, et al. Interrelationships between sex and exogenous growth hormone administration on performance, body composition and protein and fat accretion of growing pigs. J Anim Sci. 1989;67(1):177–186.
- Renner U, Sapochnik M, Lucia K, et al. Intrahypophyseal immune-endocrine interactions: endocrine integration of the inflammatory inputs. Front Horm Res. 2017;48:37–47.
- Coleman ES, Elsasser TH, Kemppainen RJ, et al. Effect of endotoxin on pituitary hormone secretion in sheep. Neuroendocrinology. 1993;58(1):111–122.
- Elin RJ, Wolff SM, Mcadam KP, et al. Properties of reference Escherichia coli endotoxin and its phthalylated derivative in humans. J Infect Dis. 1981;144(4):329–336.
- Lang CH, Pollard V, Fan J, et al. Acute alterations in growth hormone-insulin-like growth factor axis in humans injected with endotoxin. Am J Physiol. 1997;273(1 Pt 2):R371.
- Fry C, Gunter DR, Mcmahon CD, et al. Cytokine-mediated growth hormone release from cultured ovine pituitary cells. Neuroendocrinology. 1998;68(3):192–200.
- Parrott RF, Vellucci SV, Goode JA, et al. Cyclo-oxygenase mediation of endotoxin-induced fever, anterior and posterior pituitary hormone release, and hypothalamic c-Fos expression in the prepubertal pig. Exp Physiol. 1995;80(4):663.
- Wright KJ, Balaji R, Hill CM, et al. Integrated adrenal, somatotropic, and immune responses of growing pigs to treatment with lipopolysaccharide. J Anim Sci. 2000;78(7):1892.
- Harvey S, Scanes CG, Phillips JG. Growth hormone secretion in anaesthetized fowl 1. Refractoriness to repeated stimulation by human pancreatic growth hormone-releasing factor (hpGRF) or thyrotrophin releasing hormone (TRH). Gen Comp Endocrinol. 1985;59(1):1–9.
- Giustina A, Veldhuis JD. Pathophysiology of the neuroregulation of growth hormone secretion in experimental animals and the human. Endocr Rev. 1998;19(6):717–797.
- Frohman LA, Kineman RD. Growth hormone-releasing hormone and pituitary development, hyperplasia and tumorigenesis. Trends Endocrinol Metab. 2002;13(7):299–303.
- Mayo KE. Molecular cloning and expression of a pituitary-specific receptor for growth hormone-releasing hormone. Mol Endocrinol. 1992;6(10):1734–1744.
- Petersenn S, Schulte HM. Structure and function of the growth-hormone-releasing hormone receptor. Vitamins Hormones-adv Res Appl. 2000;59:35–69.
- Gaylinn BD. Growth hormone releasing hormone receptor. Recept Channels. 2002;8(3–4):155.
- Porter TE, Ellestad LE, Allison F, et al. Identification of the chicken growth hormone-releasing hormone receptor (GHRH-R) mRNA and gene: regulation of anterior pituitary GHRH-R mRNA levels by homologous and heterologous hormones. Endocrinology. 2006;147(5):2535–2543.
- Toogood AA, Stephen H, Thorner MO, et al. Cloning of the chicken pituitary receptor for growth hormone-releasing hormone. Endocrinology. 2006;147(4):1838–1846.
- Yajun W, Juan L, Crystal Ying W, et al. Identification of the endogenous ligands for chicken growth hormone-releasing hormone (GHRH) receptor: evidence for a separate gene encoding GHRH in submammalian vertebrates. Endocrinology. 2007;148(5):2405–2416.
- Kiaris H, Chatzistamou I, Papavassiliou AG, et al. Growth hormone-releasing hormone: not only a neurohormone. Trends Endocrinol Metab. 2011;22(8):311–317.
- Zeitler P, Stevens P, Siriwardana G. Functional GHRH receptor carboxyl terminal isoforms in normal and dwarf (dw) rats. J Mol Endocrinol. 1998;21(3):363–371.
- Wang CY, Wang Y, Kwok AH, et al. Identification of two novel chicken GHRH receptor splice variants: implications for the roles of aspartate 56 in the receptor activation and direct ligand receptor interaction. J Endocrinol. 2007;195(3):525–536.
- Miller TL, Godfrey PA, Dealmeida VI, et al. The rat growth hormone-releasing hormone receptor gene: structure, regulation, and generation of receptor isoforms with different signaling properties. Endocrinology. 1999;140(9):4152–4165.
- Mayo KE, Miller T, Dealmeida V, et al. Regulation of the pituitary somatotroph cell by GHRH and its receptor. Recent Prog Horm Res. 2000;55(55):237–266.
- Karen LS, Wajnrajch MP. Growth Hormone Releasing Hormone (GHRH) and the GHRH Receptor. Rev Endocr Metab Disord. 2002;3(4):313.
- Bin W, Chaobin Q, Cong Z, et al. Differential involvement of signaling pathways in the regulation of growth hormone release by somatostatin and growth hormone-releasing hormone in orange-spotted grouper (Epinephelus coioides). Mol Cell Endocrinol. 2014;382(2):851–859.
- Kineman RD, Luque RM. Evidence that ghrelin is as potent as growth hormone (GH)-releasing hormone (GHRH) in releasing GH from primary pituitary cell cultures of a nonhuman primate (Papio anubis), acting through intracellular signaling pathways distinct from GHRH. Endocrinology. 2007;148(9):4440–4449.
- Olinto SCF, Adrião MG, Castro-Barbosa T, et al. Arginine induces GH gene expression by activating NOS/NO signaling in rat isolated hemi-pituitaries. Braz J Med Biol Res. 2012;45(11):1066–1073.
- Luque RM, Rodriguez PFSM, Gracia NF, et al. Differential Contribution of Nitric Oxide and cGMP to the Stimulatory Effects of Growth Hormone-Releasing Hormone and Low-Concentration Somatostatin on Growth Hormone Release from Somatotrophs. J Neuroendocrinol. 2010;17(9):577–582.
- Qin YJ, Chan SO, Chong KK, et al. Antagonist of GH-releasing hormone receptors alleviates experimental ocular inflammation. Proc Natl Acad Sci U S A. 2014;111(51):18303–18308.
- Yang X, Zhang H, Li L. Alternative mRNA processing increases the complexity of microRNA-based gene regulation in Arabidopsis. Plant J. 2012;70(3):421–431.
- Victor A. The functions of animal microRNAs. Nature. 2004;431(7006):350–355.
- Karginov FV, Cheloufi S, Chong MM, et al. Diverse endonucleolytic cleavage sites in the mammalian transcriptome depend upon microRNAs, Drosha, and additional nucleases. Mol Cell. 2010;38(6):781–788.
- Iwasaki Y, Taguchi T, Nishiyama M, et al. Lipopolysaccharide stimulates proopiomelanocortin gene expression in AtT20 corticotroph cells. Endocr J. 2008;55(2):285.
- Theas MS, Laurentiis AD, Lasaga M, et al. Effect of Lipopolysaccharide on Tumor Necrosis Factor and Prolactin Release from Rat Anterior Pituitary Cells. Endocrine. 1998;8(3):241–245.
- Lohrer P, Gloddek J, Carbia NA, et al. Lipopolysaccharide directly stimulates the intrapituitary interleukin-6 production by folliculostellate cells via specific receptors and the p38a mitogen-activated protein kinase/nuclear factor-κB pathway. Endocrinology. 2000; 141(12):4457–4465.
- Hemmer A, Hyde JF. Regulation of galanin secretion from pituitary cells in vitro by estradiol and GHRH. Peptides. 1992;13(6):1201–1206.
- Qi QE, Xi QY, Ye RS, et al. Alteration of the miRNA expression profile in male porcine anterior pituitary cells in response to GHRH and CST and analysis of the potential roles for miRNAs in regulating GH. Growth Hormone IGF Res. 2015;25(2):66–74.
- Ye RS, Meng L, Qi-En Q, et al. Comparative anterior pituitary miRNA and mRNA expression profiles of bama minipigs and landrace pigs reveal potential molecular network involved in animal postnatal growth. Plos One. 2015;10(7):e0131987.
- Park JY, Lee SY, Kim HR, et al. Structural mechanism of GPCR-arrestin interaction: recent breakthroughs. Arch Pharm Res. 2016;39(3):293–301.
- Gurevich VV, Gurevich EV. Molecular mechanisms of GPCR signaling: a structural perspective. Int J Mol Sci. 2017;18(12):2519.
- Pavlos NJ, Friedman PA. GPCR Signaling and Trafficking: the Long and Short of It. Trends Endocrinol Metab. 2017;28(3):213–226.
- Gotow T, Nishi T. Involvement of a Go-type G-protein coupled to guanylate cyclase in the phototransduction cGMP cascade of molluscan simple photoreceptors. Brain Res. 2007;1144:42–51.
- Mainardi GL, Saleri R, Tamanini C, et al. Effects of interleukin-1-beta, interleukin-6 and tumor necrosis factor-alpha, alone or in association with hexarelin or galanin, on growth hormone gene expression and growth hormone release from pig pituitary cells. Horm Res. 2002;58(4):180–186.
- Gong FY, Deng JY, Shi YF. Stimulatory effect of interleukin-1beta on growth hormone gene expression and growth hormone release from rat GH3 cells. Neuroendocrinology. 2005;81(4):217–228.
- Daniel JA, Elsasser TH, Martínez A, et al. Interleukin-1beta and tumor necrosis factor-alpha mediation of endotoxin action on growth hormone. Ajp Endocrinol Metab. 2005;289(4):650–657.
- Leone V, Langella C, Angelo DD, et al. Mir-23b and miR-130b expression is downregulated in pituitary adenomas. Mol Cell Endocrinol. 2014;390:1–7.
- Lee YJ, Cho JM, Moon JH, et al. Increased miR-338-3p expression correlates with invasiveness of GH-producing pituitary adenomas. Endocrine. 2017;58(1):184–189.
- Qiang J, Bao JW, Li HX, et al. miR-1338-5p modulates growth hormone secretion and glucose utilization by regulating ghitm in genetically improved farmed tilapia (GIFT, Oreochromis niloticus). Front Physiol. 2017;8. DOI:10.3389/fphys.2017.00998
- Cheng Y, Liu S, Zhang X, et al. Expression profiles of IGF-1R gene and polymorphisms of its regulatory regions in different pig breeds. Protein J. 2016;35:231–236.
- Komatsu Y, Sukegawa S, Yamashita M, et al. Identification of genes showing differential expression profile associated with growth rate in skeletal muscle tissue of Landrace weanling pig. J Genet. 2016;95(2):341–347.
- Zhao BW, Zhou LF, Liu YL, et al. Evolution of fish let-7 MicroRNAs and their expression correlated to growth development in blunt snout bream. Int J Mol Sci. 2017;18(3). DOI:10.3390/ijms18030646
- He J, Zhang J, Wang Y, et al. MiR-7 mediates the zearalenone signaling pathway regulating fsh synthesis and secretion by targeting FOS in female pigs. Endocrinology. 2018;159(8):2993–3006.
- Sikarwar AS, Bhagirath AY, Dakshinamurti S. Effects of post-translational modifications on membrane localization and signaling of prostanoid GPCR-G protein complexes and the role of hypoxia. J Membr Biol. 2019;252(4–5):509–526.