ABSTRACT
zong non-coding RNAs (lncRNAs) have been identified as crucial effector in modulating the progression of assorted malignancies. In our study, the main aim was to unveil the role and the underlying regulatory mechanism of long intergenic non-protein coding RNA 1605 (LINC01605) in nasopharyngeal carcinoma (NPC). RT-qPCR analysis results suggested that LINC01605 was upregulated in NPC cells. According to the results of function experiments, LINC01605 promoted NPC cell proliferation and impeded cell apoptosis. The oncogenic role of LINC01605 in NPC was further validated by animal experiments. Additionally, we verified that LINC01605 regulated Ikbkb expression to promote the nuclear translocation of p65 and thereby activated the NF-κB pathway in NPC cells. Mechanism experiments further suggested that LINC01605 could regulate Ikbkb expression via sponging miR-942-5p. Moreover, LINC01605 recruited IGF2BP2 to stabilize ubiquitin-specific protease 3 (USP3) mRNA and thereby enhanced the stability of IkB subunit beta (IKKβ) protein. In addition, p65 acted as a transcription activator to upregulate LINC01605 in NPC cells. In conclusion, this study demonstrated a positive feedback loop between LINC01605 and the NF-κB signalling pathway that promoted NPC cell growth, thus providing new insights to better understand NPC.
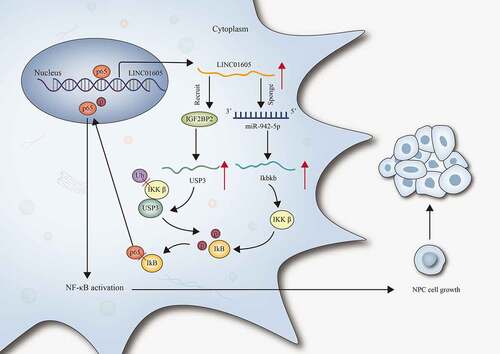
Introduction
Nasopharyngeal carcinoma (NPC), one of the most common malignant head and neck tumours, which originates from the nasopharynx epithelium, is prevalent in Southeast Asia and Southern China [Citation1]. From the recent literature, the tumourigenesis of NPC has been evidenced to be closely associated with genetic and environmental factorsas well as Epstein–Barr virus infection, but the precise molecular mechanism of its pathogenesis remains to be further investigated [Citation2]. With advances achieved in applied technology of radiotherapy and chemoradiotherapy, the prognosis of patients with NPC has been substantially improved [Citation3]. However, about 30% of NPC patients are very likely to suffer from distant metastasis, and the therapeutic outcome of those patients is unsatisfactory [Citation4]. Therefore, it is essential to make further investigation into the molecular mechanisms implicated in NPC progression for the discovery of novel and effective therapeutic targets for NPC.
Increasing evidence has demonstrated that more than 98% of human transcriptomes that lack protein-coding ability are identified as non-coding RNAs (ncRNAs) [Citation5]. Furthermore, ncRNAs, especially long ncRNAs (lncRNAs) and microRNAs (miRNAs), are suggested as crucial participants in epigenetic regulation [Citation6]. LncRNAs are defined with a length of transcripts of more than 200 nucleotides and lack the potential to encode proteins [Citation7]. LncRNAs have been verified to mediate gene expression via serving as nuclear transcriptional regulators, RNA-binding protein (RBP) sequestering agents and a sponge of a certain miRNA [Citation8]. Accumulating studies have unveiled the crucial involvement of lncRNAs in modulating malignant behaviours of NPC cells and in regulating NPC progression [Citation9]. Specifically, lncRNA UCA1 contributes to the epithelial–mesenchymal transition of NPC cells [Citation10]. Overexpression of SNHG12 regulates cell proliferation and metastasis and predicts a poor prognosis of NPC [Citation11].
The nuclear factor-κB (NF-κB) signalling pathway has been suggested as essential effectors in regulating the expression of genes, thereby influencing cell proliferation, apoptosis as well as inflammation and immune responses [Citation12]. There are five members of NF-κB transcription factors: p50, p52, p65 (RelA), c-Rel and RelB proteins [Citation13]. RelB, c-Rel and p65 contain transcription activation domains that are responsible for positively regulating the expression of downstream genes [Citation14]. Upon stimulation, I kappa B kinase (IKK) is activated. IKK phosphorylates the inhibitors of kappa B proteins (IκB), which are then degraded by the proteasome, allowing the translocation of the NF-κB dimers to the nucleus [Citation15]. Based on recent studies, the close association between NF-κB pathway activation and NPC progression has been ascertained [Citation16]. Therefore, we also intended to reveal the putative involvement of lncRNA and the NF-κB pathway in modulating biological behaviours of NPC cells.
Ubiquitination has been considered as an important process in the post-translational modification of cellular proteins in cancers [Citation17]. Ubiquitin-specific protease 3 (USP3) is a member of the ubiquitin-specific protease family, which can regulate the ubiquitination and stability of proteins [Citation18]. USP3 has been reported to function as an oncogenic factor in the development of some malignancies [Citation19], but its role in NPC remains largely unknown.
Aiming to elaborate the role of long intergenic non-protein coding RNA 1605 (LINC01605) in NPC, we investigated the expression of LINC01605 in NPC cells and explored the changes in the proliferative and apoptotic abilities of NPC cells under the influence of LINC01605 deficiency or overexpression. Moreover, we probed into the molecular mechanism of LINC01605 in activating the NF-κB pathway and discovered the relation between LINC01605 and USP3-mediated ubiquitination process. The influences of NF-κB/p65 on LINC01605 expression were also evaluated. Collectively, the validity of the feedback loop between LINC01605 and the NF-κB pathway was ascertained in the current study, which might provide a novel insight for in-depth NPC investigation and ultimately contribute to the discovery of novel, effective targets for NPC diagnosis and treatment.
Material and methods
Cell culture and treatments
NPC cells (SUNE1, CNE-2, 5–8 F and CNE-1), human normal nasopharyngeal epithelial cells NP69 and human embryonic kidney (HEK) 293 T cells were purchased from Shanghai Huzhen Biotechnology Co., Ltd (Shanghai, China). SUNE1 and HEK293T cells were cultured in DMEM (A4192101, Gibco, USA), while other cells were cultured in RPMI-1640 medium (A4192301, Gibco), added with 10% foetal bovine serum (FBS; 16140071, Thermo Fisher Scientific, USA). All cells were kept in a humid incubator with 5% CO2 at 37°C. For cell treatment, cycloheximide (CHX; 5 µM; 5087390001, Sigma-Aldrich, St. Louis, MO, USA), MG132 (10 Μm; M7449, Sigma-Aldrich), actinomycin D (4 μg/Ml; SBR00013, Sigma-Aldrich) and p65 inhibitor (parthenolide; PT; 5 μmol/L; HY-N0141, MedChemExpress, New Jersey, USA), were purchased.
Quantitative real-time polymerase chain reaction (RT-qPCR)
After total RNA extraction with TRIzol reagent (15596018, Thermo Fisher Scientific), total RNA was reversely transcribed into cDNA with PrimeScript RT master mix (RR058A, Takara, Japan) and qPCR was performed with the SYBR Premix Ex Taq™ kit (RR390W, Takara). GAPDH and U6 were used as internal controls. The calculation of gene expression was based on the 2−ΔΔCT method. Primer sequences used in RT-qPCR are provided in Supplementary file 1.
Fluorescence in situ hybridization (FISH)
FISH kit (C10910, RiboBio, Guangzhou, China) was applied for verifying the cellular localization of LINC01605. Cells were subjected to the washing process with PBS, fixation with 4% formaldehyde and permeabilization with 0.5% Triton X-100 (HFH10, Invitrogen, USA). Then, the cells were incubated with a FISH probe targeting LINC01605 in the hybridization solution. After the nuclear staining with DAPI (D9542, Sigma-Aldrich), the images were analysed under confocal microscopy. The sequence of the FISH probe targeting LINC01605 is listed in Supplementary file 1.
Subcellular fractionation
The PARIS kit (AM1921, Thermo Fisher Scientific) was applied for isolating the nuclear and cytoplasmic fraction based on the supplier’s protocol. The nuclear and cytoplasmic RNAs were analysed by RT-qPCR. U6 and GAPDH were severally used for the nuclear and cytoplasmic reference. Relevant CT values are provided in Supplementary file 2.
Cell transfection
The LINC01605-, USP3- or IGF2BP2-specific shRNAs and sh-NC, pcDNA/LINC01605, pcDNA/Ikbkb and pcDNA, p65 expression vector and empty vector were provided by RiboBio (China). Moreover, miR-942-5p mimics, NC mimics, miR-942-5p inhibitor and NC inhibitor were purchased from GenePharma Cells Company. Lipofectamine 3000 transfection (L3000015, Thermo Fisher Scientific) was used for transfection for 48 h. The transfection efficiency of indicated plasmids was determined via RT-qPCR. Relevant sequences are listed in Supplementary file 1.
Cell counting kit-8 (CCK-8)
Cell viability was measured with a CCK-8 kit (HY-K0301, MedChemExpress, USA) in line with the supplier’s instructions. Cells were inoculated in a 96-well plate (3000 cells per well), and 10 μl of CCK-8 solutions were added into each well for 24-h incubation. A microplate reader was applied for the measurement of the absorbance at 450 nm in each well.
5-Ethynyl-2’-deoxyuridine (EdU)
Cell proliferation was determined with an EdU kit (C10310, RiboBio) in the light of the manufacturer’s suggestions. Briefly, cells were seeded into 96-well plates and incubated with 100 μl of 50 μM EdU at 37°C, followed by fixation with 4% paraformaldehyde. After nuclear staining with DAPI, EdU positive cells were observed under fluorescence microscopy and analysed to reflect the changes in cell proliferation.
Colony formation
Cells were seeded in 6-well plates with a density of 500 cells in each well for 2-week culture. Subsequent to the PBS washing process, cells were fixed with 4% paraformaldehyde and stained with 0.5% crystal violet (V5265, Sigma-Aldrich). The number of colonies (diameter ≥100 μm) was counted manually to reflect the proliferative abilities in indicated groups.
JC-1
Cells were centrifuged and resuspended, followed by incubation in 500 μl JC-1 staining working solution (C2006, Beyotime, Guangzhou, China) for 30 min at 37°C. The fluorescence labelled cells were then rinsed with PBS and analysed with an EnSpire Reader. The fluorescence rate at 590 nm versus 530 nm emission was employed for the measurement of the mitochondrial membrane potential. GraphPad Prism 5 statistical software was applied for recording data and images. The data obtained from JC-1 assays indicated healthy mitochondria in red fluorescence and unhealthy ones in green fluorescence, and the JC-1 ratio was defined as the arithmetic mean (AM) of red fluorescence/AM of green fluorescence.
Terminal deoxynucleotidyl transferase dUTP nick end labelling (TUNEL)
After being planted in 96-well plates, cells were fixed with 4% paraformaldehyde and permeabilized with 0.5% Triton X-100, followed by cultivation with 50 μL TdT reaction mix (16314015, Thermo Fisher Scientific). After nuclear staining with DAPI, fluorescence microscopy was applied to obtain images for further calculation of cell apoptotic rates.
Flow cytometry
Flow cytometry and double Annexin V/PI staining kit (C1062S, Beyotime) were applied for assessing the apoptosis of the transfected cancer cells as previously described [Citation20]. CNE-1 and 5–8 F cells in 6-well plates were finally analysed by a flow cytometer (92821250S, Beckman Coulter, Kraemer Boulevard Brea, CA, USA). IgG isotype control (11–4888-81, Invitrogen, Carlsbad, CA, USA) was utilized.
Luciferase reporter assay
Cignal Finder Reporter Array (336841, QIAGEN, Dusseldorf, Germany) was applied to ravel out the specific signalling pathway involved in the LINC01605 modulation mechanism in NPC cells. Moreover, wild-type or mutant LINC01605 or Ikbkb 3ʹUTR fragment covering miR-942-5p binding sites was inserted into pmirGLO luciferase reporter vectors (E1330, Promega, Madison, WI, USA). In addition, pGL3-basic reporter vectors (E1751, Promega) containing USP3 promoter were transfected with sh-LINC01605#1. Also, pGL3-basic reporter vectors containing LINC01605 promoter were transfected into cells with co-transfection of p65 inhibitor or p65 expression vector or into cells before and after IL-1α (NF-κB activator) treatment. After 48-h transfection, luciferase activities in indicated groups were examined with a luciferase reporter assay kit (16164, Thermo Fisher Scientific). All the sequences of reporter constructs are listed in Supplementary file 1.
Western blot
Proteins were extracted from cells using RIPA lysis buffer (R0278, Sigma-Aldrich). Subsequent to protein concentration calculation, the protein was separated with the utilization of SDS-PAGE (1610174, Bio-Rad Laboratories, USA) and then transferred to PVDF membranes (IPVH00010, Millipore, USA). Subsequent to blocking with 5% skim milk, the membranes were incubated with the primary antibodies procured from Abcam (USA), including anti-p65 (0.5 µg/ml; ab32536), anti-Histone H3 (1/1000; ab1791anti-IKKβ (1/10000; ab124957), anti-p-IKBα (Ser36) (1/10000; ab133462), anti-p-IKBβ (Ser23) (1/10000; ab75746), anti-GAPDH (1/1000; ab8245), anti-USP3 (1/1000; ab229348), anti-IGF2BP2 (1/2000; ab128175), anti-FUS (1/1000; ab124923), anti-EIF4A3 (1/1000; ab180573), anti-ELAVL1 (1/1000; ab200342), anti-IKBα (1/1000; ab32518), anti-IKBβ (1/1000; ab76429) and anti-HNRNPC (1/10000; ab133607) at 4°C overnight. Subsequently, horseradish peroxidase-conjugated secondary antibodies were added for further incubation. Clarity Max Western ECL Substrate (32134, Pierce Biotechnology, Rockford, IL, USA) was applied for visualizing immunoblots. The original blots for Western blot results are provided in Supplementary file 3.
Immunofluorescence (IF) staining
IF staining was conducted as previously described [Citation21]. After being fixed with 4% paraformaldehyde, cells were permeabilized with 0.5% Triton X-100 and then incubated with primary antibody (1/100), followed by incubation with fluorescently labelled secondary antibody (1/200) conjugated with magnetic beads. After the staining of nuclei with DAPI, a fluorescence microscope was applied for observation.
RNA-binding protein immunoprecipitation (RIP)
RIP assay was conducted with reference to a previous study [Citation22]. Magna RIP™ RNA-Binding Protein Immunoprecipitation kit (17–700, Millipore) was applied for performing RIP assay, with the manufacturer instructions strictly followed. After the washing process with PBS and the acquisition of cell lysates with lysis buffer, Turbo DNase was applied to treat the lysate, followed by30-min incubation at 4°C with rotation. NT2 buffer was then added for the dilution of cell lysate, and 50 μL of the diluted mixtures were spared as the input for further PCR analysis. After washing twice in cold NT2 buffer, 50 μL of protein G magnetic beads were pre-blocked by 1× PBS plus 5 mg/mL BSA, followed by incubation with 5 μg of Ago2 antibody (ab186733, Abcam), IGF2BP2 antibody and IgG antibody (401455–2ML-M, Millipore), with 1-h rotation at room temperature. Next, 500 μL of supernatant was incubated with antibody-binding beads at 4℃ overnight . Subsequently, after washing the RNA/antibody/protein complex six times (5 min for each wash) in NT2 buffer supplemented with protease inhibitor cocktail and protector RNase inhibitor, the RNA was extracted with acid phenol to chloroform, pH 4.5 (with IAA, 125:24:1). RT-qPCR analysis was applied for accessing the enrichment of target genes.
Immunoprecipitation (IP) and ubiquitination
Firstly, cold PBS was used to wash the cells three times. Total protein was extracted with lysis buffer, and the target protein was immunoprecipitated from cell lysate after one-night incubation with anti-IKKβ antibody and protein A/G beads. After washing and boiling, the immunoprecipitated protein complex was analysed by Western blot. To test IKKβ ubiquitylation, the lysis buffer was treated with ubiquitin reaction buffer (U6253, Sigma-Aldrich).
RNA pull-down assay
In line with the method described previously, RNA pull-down assay was done to explore the putative affinity among genes [Citation23]. LINC01605, USP3 and their respective antisense RNAs were transcribed in vitro and biotin-labelled by Roche. Then protein lysates from cells were subjected to 1-h incubation with the biotin-labelled RNA. Streptavidin agarose beads (100 ml; Invitrogen) were added for further 1-h incubation at room temperature. The beads were boiled in SDS buffer after being washed three times, and finally, Western blot was performed for the detection of the retrieved protein.
Chromatin immunoprecipitation (ChIP)
The EZ ChIP kit (17–295, Millipore) was used to perform a ChIP assay as described previously [Citation24]. Cells were collected and cross-linked with 4% paraformaldehyde at room temperature. After the addition of glycine, the cells were lysed, incubated, sheared by sonication and then centrifuged. Next, cell lysates were incubated with anti-p65 or anti-IgG, followed by the addition of protein A/G magnetic beads (Roche, USA) overnight. After immunoprecipitation, protein–DNA crosslinks were reversed and the chromatin proteins were removed after DNA purification. The immunoprecipitated DNA complex was finally analysed by AGE. The binding region of p65 on the LINC01605 promoter was at site 440–449 (TSS upstream): GGGAAGTTGC. The primer sequence specific for the LINC01605 promoter is listed in Supplementary file 1.
Xenograft model
Female BALB/c nude mice of 4–6 weeks old were acquired from Guangdong Medical Laboratory Animal Center. NPC cells transfected with sh-LINC01605 and sh-NC were implanted into the mice via subcutaneous injection. Tumour volumes were measured every 4 days and calculated with the following formula: volume = (length × width2)/2. After 4 weeks, all mice were sacrificed, and the tumours were excised and collected for the measurement of tumour size and weight. The animal experiments were approved by Zhongshan People’s Hospital.
Statistical analysis
Data are presented as mean ± SD and analysed using GraphPad Prism software. All experiments were conducted in triplicate. Student’s t-test was applied for comparison between two groups, and one-way analysis of variance (ANOVA) or two-way ANOVA was used to compare the differences of more than two groups. P values less than 0.05 were statistically significance.
Results
LINC01605 is upregulated in NPC cells
To search for lncRNAs related to NPC development, we applied GEPIA (http://gepia.cancer-pku.cn) database to find all upregulated lncRNAs in head and neck squamous cell carcinoma (HNSC), and the result showed there were 11 candidates (Figure S1A). Then, their expressions in four of NPC cells (SUNE1, CNE-2, 5–8 F and CNE-1) and in human normal nasopharyngeal epithelial cells NP69 were detected using RT-qPCR. The data revealed that only the expression of LINC01605 was higher in all NPC cell lines than in NP69 cells (Figure S1B-K & )). Statistics from GEPIA also indicated that LINC01605 was obviously upregulated in HNSC tissues compared with normal tissues ()). It is well acknowledged that the subcellular localization of lncRNAs is closely associated with their functions and mechanisms [Citation25]. Thus, we performed FISH and subcellular fractionation assays to determine the cellular location of LINC01605 in NPC cells. As revealed in , LINC01605 principally existed in the cytoplasm, suggesting the post-transcriptional role of LINC01605 in NPC cells. To sum up, LINC01605 was upregulated in NPC.
Figure 1. LINC01605 is upregulated in NPC cells.
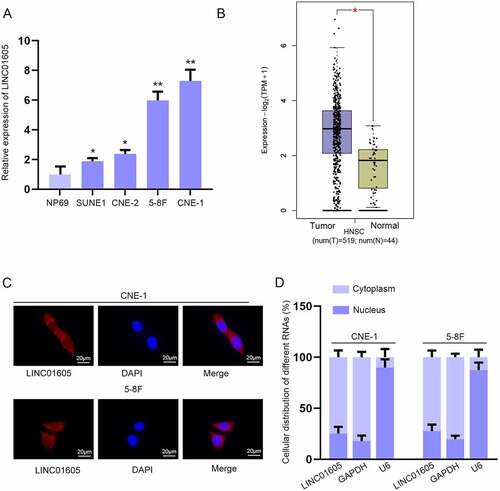
LINC01605 silence inhibits cell malignant phenotype in NPC
Among the selected four NPC cells, relative LINC01605 expression was the highest in 5–8 F and CNE-1 cells, so 5–8 F and CNE-1 cells were chosen as subjects to ravel out the biological function of LINC01605. Then, sh-LINC01605 was transfected to stably knock down LINC01605 expression in cells, and RT-qPCR proved that LINC01605 expression was downregulated by nearly 70% ()). We also transfected pcDNA/LINC01605 into NPC cells to overexpress LINC01605. As a result, LINC01605 expression was much upregulated by pcDNA/LINC01605 (Figure S2A). Then, functional assays were conducted with sh-LINC01605 or pcDNA/LINC01605 transfected cells. It was suggested in the CCK-8 assay that LINC01605 depletion could obviously weaken the viability of CNE-1 and 5–8 F cells ()), and contrarily, LINC01605 overexpression was discovered to notably strengthen cell viability (Figure S2B). EdU and colony formation assays also revealed that LINC01605 depletion repressed the proliferation of 5–8 F and CNE-1 cells () while LINC01605 upregulation resulted in enhanced NPC cell proliferation (Figure S2C-D). Moreover, the influences of LINC01605 expression on cell apoptosis were investigated. A decrease in the JC-1 ratio was discovered as a result of LINC01605 knockdown ()), while LINC01605 overexpression overtly augmented the JC-1 ratio (Figure S2E), which reflected that LINC01605 silence could strengthen the apoptotic ability of NCP cells and LINC01605 overexpression led to opposite consequences. TUNEL and flow cytometry assays further confirmed the results (); S2F-S2G; S3A). Meanwhile, tumourigenesis experiments were carried out for evaluating the influence of LINC01605 on tumour development. After measuring the tumour size, volume and weight, it was indicated that downregulated LINC01605 repressed tumour growth (Figure S3B-D). In addition, through IHC assay, it was found that Ki67 expression reduced, while cleaved caspase-3 expression increased after LINC01605 depletion (Figure S3E). To conclude, LINC01605 functioned as an oncogenic effector in NPC.
Figure 2. LINC01605 silence inhibits cell proliferation and enhances cell apoptosis in NPC. (a) Efficiency of sh-LINC01605#1/2 in CNE-1 and 5–8 F cells was evaluated by RT-qPCR. (b–d) The changes in cell viability and proliferation under the influence of sh-LINC01605 transfection were evaluated by CCK-8, EdU and colony formation assays. (e–f) Apoptosis of sh-LINC01605-transfected CNE-1 and 5–8 F cells was measured by JC-1 and TUNEL assays. One-way ANOVA was applied for statistical analysis. **P < 0.01.
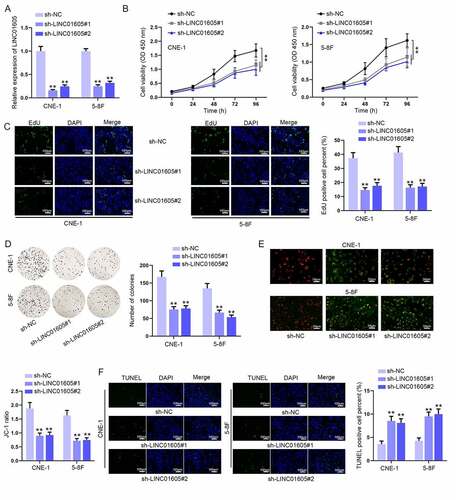
LINC01605 positively regulates Ikbkb expression to promote the nuclear translocation of p65 and thereby activates the NF-κB pathway
LncRNAs are involved in cancer progression via various signalling pathways. Aiming to fathom out the specific signalling pathway implicated in the LINC01605 modulation mechanism in NPC, we conducted a luciferase reporter assay with Cignal Finder Reporter Array in HEK293T and CNE-1 cells. As illustrated in ), the absence of LINC01605 significantly weakened the luciferase activity of the NF-κB pathway but barely affected that of other pathways. To confirm the link between LINC01605 expression and the activation of the NF-κB pathway, Western blot assay was carried out to detect the influences of LINC01605 depletion on key proteins of the NF-κB pathway. The results evidenced that LINC01605 silence decreased the level of p-p65 in the nucleus when total p65 expression in cell lysates remained unchanged, and the protein levels of IKKβ, p-IKBα (Ser36) and p-IKBβ (Ser23) in whole cells were decreased (), left). LINC01605 augment caused the opposite results (), right). Then, the cells were treated with parthenolide (PT), which was able to induce p65 retention in the cell cytoplasm. We found that the phosphorylation of IKBα and IKBβ decreased with time after PT treatment, reflecting the inactivation of the NF-κB pathway (Figure S3F). Subsequently, it was discovered that the mRNA level of Ikbkb was dramatically downregulated when LINC01605 was knocked down, while that of other effector molecules was not changed ()). It was proved by IF assay that LINC0605 could induce nuclear entry of p-p65 as the intensity of p-p65 in the nucleus was obviously reduced after the silence of LINC01605 ()). Hence, it was suggested that LINC01605 could positively regulate Ikbkb expression to promote the nuclear translocation of p65 and thereby activate the NF-κB pathway.
Figure 3. LINC01605 regulates Ikbkb expression to promote nuclear translocation of p65 and thereby activates the NF-κB pathway.
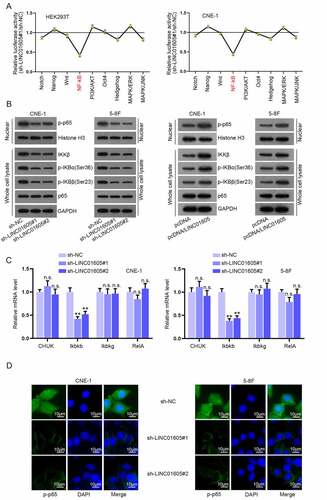
LINC01605 sequesters miR-942-5p to regulate Ikbkb mRNA
Given that LINC01605 had been proven to mainly accumulate in the cytoplasm, we hypothesized that LINC01605 may participate in the ceRNA mechanism and sponge certain miRNAs to regulate Ikbkb expression. Therefore, we used DIANA (http://carolina.imis.athena-innovation.gr) website to search for miRNAs with the potential to combine with LINC01605 and used starBase (http://starbase.sysu.edu.cn) database to predict miRNAs that were able to bind with Ikbkb. As illustrated in ), 9 miRNAs of LINC01605 (threshold> 0.9) and 161 miRNAs of Ikbkb are displayed in the Venn diagram. Notably, only one miRNA (miR-942-5p) is in the intersection. Moreover, the respective binding sites between LINC01605 and miR-942-5p or Ikbkb and miR-942-5p are shown in ). Subsequently, we analysed miR-942-5p expression in NPC cells via RT-qPCR analysis and observed that miR-942-5p expression was relatively low in NPC cells (5–8 F and CNE-1 in particular) ()). Moreover, an increment in the miR-942-5p level was observed after miR-942-5p mimics transfection into cells ()). For the verification of the combination between LINC01605/Ikbkb and miR-942-5p, we subcloned LINC01605 or Ikbkb with wild-type and mutated miR-942-5p binding sites into dual-luciferase reporters and then co-transfected the constructed reporters with indicated plasmids (NC mimics or miR-942-5p mimics) into 5–8 F and CNE-1 cells. We observed an overt decline in the luciferase activity of LINC01605-WT and Ikbkb-WT after miR-942-5p mimics transfection, while no obvious alterations in that of LINC01605-MUT and Ikbkb-MUT were observed under the same conditions ()). More importantly, the inhibitory impacts of miR-942-5p overexpression on luciferase activity of Ikbkb-WT were offset after co-transfection of pcDNA/LINC01605 ()). The Ago2 protein is a core component of the RNA-induced silencing complex (RISC). To confirm the existence of a ceRNA network involving LINC01605, miR-942-5p and Ikbkb, an RIP assay was carried out. The results showed that these three RNAs were preferentially precipitated in the Ago2 antibody rather than the IgG antibody ()). Additionally, the Ikbkb mRNA level reduced after LINC01605 depletion could almost be recovered as a result of miR-942-5p inhibition, but the decreased IKKβ protein level caused by LINC01605 silence was only partly recovered by miR-942-5p inhibitor co-transfection ()).
Figure 4. LINC01605 sequesters miR-942-5p to regulate Ikbkb mRNA.
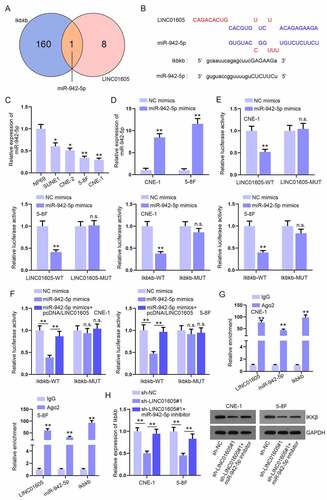
To further determine the impacts of the LINC01605/miR-942-5p/Ikbkb axis on NPC cell proliferation and apoptosis, functional experiments with CNE-1 cells were carried out in a rescue way. Ikbkb overexpression was accomplished by transfecting pcDNA/Ikbkb into CNE-1 cells (Figure S4A). It was observed in the CCK-8 assay that the lessened cell proliferation due to the transfection of sh-LINC01605#1 was partly reversed as a result of miR-942-5p inhibitor co-transfection and was totally restored after the co-transfection of pcDNA/Ikbkb (Figure S4B). Similar results were discovered in EdU and colony formation assays (Figure S4C-D). In parallel, the data from JC-1, TUNEL and flow cytometry assays suggested that the promoting influence of LINC01605 downregulation on apoptosis ability of cells were moderately countervailed by miR-942-5p inhibition and were fully counteracted after co-transfection of pcDNA/Ikbkb (Figure S4E-G). Thus, LINC01605/miR-942-5p/Ikbkb was confirmed to influence NPC cell malignant behaviours.
LINC01605 regulates USP3 to stabilize IKKβ protein
Since miR-942-5p inhibition could partly recover the reduction in the IKKβ protein level caused by LINC01605 silence, we further investigated the molecular mechanism, of which LINC01605 could further regulate IKKβ protein. We treated 5–8 F and CNE-1 cells with CHX (a protein synthesis inhibitor) before and after LINC01605 knockdown to explore the influence of LINC01605 on IKKβ protein stability. The IKKβ protein degraded obviously more quickly when LINC01605 was silenced, indicating that LINC01605 could facilitate the stability of the IKKβ protein ()). To further determine whether LINC01605 could inhibit IKKβ protein degradation through ubiquitination, we treated 5–8 F and CNE-1 cells with proteasome inhibitor MG132 upon LINC01605 silence and found that MG132 could attenuate the suppressive effects of LINC01605 silence on IKKβ protein stability ()). As expected, the IP assay verified that the ubiquitination of IKKβ protein was increased when LINC01605 was depleted in 5–8 F and CNE-1 cells ()). Thus, we conjectured that LINC01605 might regulate certain proteins to mediate the deubiquitination of IKKβ protein and enhance the stability of IKKβ protein.
Figure 5. LINC01605 regulates USP3 to stabilize IKKβ protein.
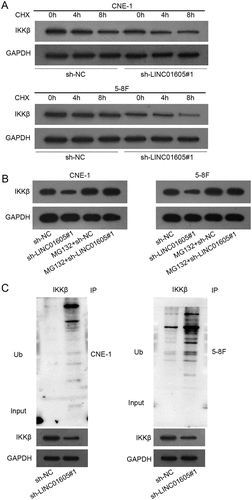
Ubiquitin-specific peptidase (USP) is the main member of the deubiquitinating enzyme (DUB) family [Citation26]. RT-qPCR analysis was done to measure the expression of USP family members in CNE-1 cells before and after LINC01605 depletion. As revealed in Figure S5A, the expression levels of three USP family members including USP3, USP5 and USP9X were all significantly reduced after LINC01605 depletion. To confirm which DUB was responsible for regulating the level of IKKβ protein, we silenced the three DUBs, respectively (Figure S5B). Then, Western blot was performed for the measurement of the IKKβ protein level before and after the inhibition of these three DUBs, separately. The outcome displayed that the IKKβ protein level was significantly decreased only when USP3 was silenced (Figure S5C). To summarize, LINC01605 regulated USP3 to mediate IKKβ deubiquitination, thus stabilizing the IKKβ protein.
LINC01605 recruits IGF2BP2 to stabilize USP3 mRNA
To investigate the potential mechanism through which LINC01605 could regulate USP3 expression, we first performed a luciferase reporter assay to determine whether LINC01605 regulated USP3 expression at the transcriptional level. As revealed in ), no significant alterations in the luciferase activity of pGL3-basic reporter vectors containing USP3 promoter were observed as a result of sh-LINC01605#1 transfection, which excluded the transcriptional regulation possibility. 5–8 F and CNE-1 cells were then treated with actinomycin D to inhibit USP3 mRNA synthesis, and it was found that the stability of USP3 mRNA obviously declined after LINC01605 depletion ()). Therefore, we assumed that LINC01605 might recruit some RNA-binding proteins (RBPs) to stabilize USP3 mRNA.
Figure 6. LINC01605 recruits IGF2BP2 to stabilize USP3 mRNA.
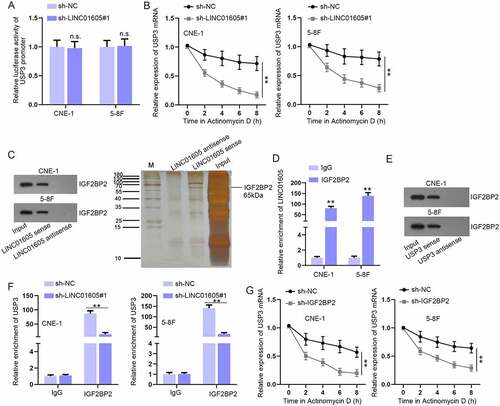
After utilizing the starBase website, we predicted 34 common RBPs of LINC01605 and USP3 (Figure S6A), and only five RBPs have been reported to promote the stability of mRNAs [Citation27–31]. For further screening, we inhibited these five RBPs, respectively, and the inhibition efficiency was determined via RT-qPCR and Western blot (Figure S6B-C). Then, USP3 expression in response to the knockdown of respective RBP was evaluated. According to the experimental results, only IGF2BP2 inhibition resulted in an overt reduction in the both mRNA and protein levels of USP3 (Figure S6D-E). Then, the IF-FISH assay was performed, the result of which indicated that LINC01605 and IGF2BP2 co-existed in the cell cytoplasm (Figure S6F). Additionally, RNA pull-down and RIP assays further confirmed the combination between IGF2BP2 and LINC01605 (). The combination between IGF2BP2 and USP3 was certified by the RNA pull-down assay ()). More importantly, we found that the combination between USP3 and IGF2BP2 was significantly lessened when LINC01605 was depleted in 5–8 F and CNE-1 cells ()). Afterwards, we confirmed that IGF2BP2 reduction decreased the stability of USP3 mRNA ()). Therefore, the conclusion could be drawn that LINC01605 recruited IGF2BP2 to enhance the stability of USP3 mRNA.
Overexpression of p65 enhances LINC01605 expression
It has been reported that p65 overexpression can enhance the expression of lncRNA [Citation32]. Herein, we also wondered whether p65 could affect the expression of LINC01605, thus forming a positive feedback loop between LINC01605 and the NF-κB pathway. Through ChIP assay, we found that the enrichment of the LINC01605 promoter was abundant in p65 antibody, indicating that p65 might affect LINC01605 expression at the transcriptional level ()). Next, p65 expression vectors were procured and transfected into cells to overexpress p65 (Figure S6G). In the luciferase reporter assay, the luciferase activity of the LINC01605 promoter was obviously weakened with p65 inhibition and enhanced with p65 overexpression ()). Moreover, RT-qPCR analysis further confirmed that LINC01605 was positively regulated by p65 ()). In addition, we tested the luciferase activity of the LINC01605 promoter and LINC01605 expression after treating cells with IL-1α (NF-κB activator). The results manifested that the luciferase activity of pGL3-basic reporter vectors containing the LINC01605 promoter was strengthened, and LINC01605 expression was elevated after the addition of IL-1α (Figure S6H-I). In sum, NF-κB pathway activation could also facilitate the transcription of LINC01605.
Figure 7. Overexpression of p65 enhances LINC01605 expression.
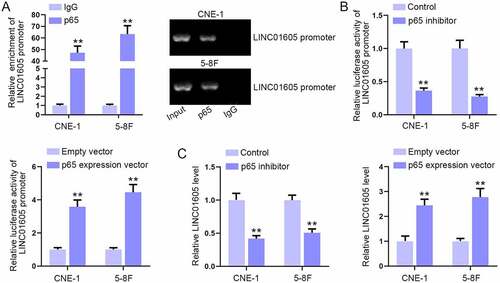
Discussion
In our study, it was confirmed that LINC01605 was significantly upregulated in NPC cells. LINC01605 exacerbated NPC cell malignant behaviours in vitro and aggravated NPC tumour growth in vivo. LINC01605 functioned as an miR-942-5p sponge to increase Ikbkb expression and thereby promoted the nuclear translocation of p65. Meanwhile, LINC01605 recruited IGF2BP2 to stabilize USP3 and thereby enhanced the deubiquitination of IKKβ to stabilize IKKβ protein. In addition, p65 acted as a transcription activator to upregulate LINC01605 in NPC cells. In conclusion, a positive feedback loop between LINC01605 and the NF-κB signalling pathway was discovered to promote NPC.
Increasing more evidence has indicated that lncRNAs are important regulators in NPC progression [Citation33,Citation34]. Herein, we probed into the role of LINC01605 in NPC. Based on bioinformatics analysis and RT-qPCR analysis, LINC01605, upregulated in HNSC tissues and the four NPC cell lines, was selected as our research target. A previous study has indicated that high expression of LINC01605 is related to poor prognosis and facilitates tumour progression in bladder cancer [Citation35]. Consistently, our study found that LINC01605 silence curbed cell proliferation and elevated cell apoptosis in NPC, while LINC01605 overexpression led to the opposite consequences. Moreover, the promoting influences of LINC01605 on NPC progression were validated by animal experiments. Therefore, the oncogenic role of LINC01605 in NPC was ascertained. However, the clinical significance of LINC01605 in NPC remains to be further investigated with the involvement of clinical samples.
LncRNAs can regulate a variety of signalling pathways to impact cancer progression, including NPC [Citation11]. Through a series of experiments, LINC01605 was suggested as an NF-κB pathway activator in NPC cells. The NF-κB signalling pathway plays a critical role in immune response and cancer progression [Citation36]. However, the regulation of lncRNA on the NF-κB signalling pathway in NPC has been rarely reported. It was further confirmed that LINC01605 positively regulated Ikbkb expression. Ikbkb (IKKβ) is a catalytic component of the IκB kinase (IKK) complex [Citation37]. Upon stimulation, IκBs can be phosphorylated by IKKβ, which causes its rapid degradation and releases p65 for nuclear translocation and subsequently regulates gene transcription [Citation38]. In line with this evidence, our study also confirmed that knockdown of LINC01605 inhibited the nuclear translocation of p65. Taken together, LINC01605 regulated Ikbkb expression to promote the nuclear translocation of p65 and thereby activated the NF-κB pathway in NPC cells.
Accumulating evidence has suggested that lncRNAs, as miRNAs sponges or decoys, can regulate the expression of mRNAs [Citation39], and our research was also a good example on the relation furthermore. The role of LINC01605 as an miRNA sponge has been reported in laryngeal squamous cell carcinoma [Citation40]. Consistent with the above studies, our research further found that LINC01605 regulated Ikbkb expression via acting as an miR-942-5p sponge. In a previous study, miR-942-5p has been discovered to display aberrantly high expression in many cancers, such as melanoma [Citation41], non-small-cell lung cancer [Citation42] and hepatocellular carcinoma [Citation43]. However, our study confirmed that miR-942-5p was downregulated in NPC cells, which was different from the tumour-promoting role of miR-942-5p in other cancers as reported.
Functional assays in a rescue way reflected that the influences of LINC01605 on NPC cell proliferation and apoptosis could be partly countervailed by miR-942-5p inhibition but completely neutralized by Ikbkb overexpression. Another finding of our study implied that miR-942-5p inhibition could partly reverse the decline in the IKKβ protein level resulting from LINC01605 silence. Therefore, we speculated that there was another regulatory network through which LINC01605 could modulate IKKβ protein. On this basis, our research further confirmed that LINC01605 regulated USP3 to promote IKKβ deubiquitination and stabilize IKKβ protein. Mounting studies have uncovered that USP3 regulates cancer progression via deubiquitinating proteins [Citation44,Citation45], which are consistent with our study. In addition, our study found that LINC01605 recruited IGF2BP2 to stabilize USP3 mRNA. The upregulated expression of IGF2BP2 has been demonstrated to exert a tumourigenic role in several cancer types, often with a poor prognosis. For instance, it has been reported that IGF2BP2 depletion would significantly inhibit non-small-cell lung cancer cell growth [Citation46]. Overexpressed IGF2BP2 in human colon cancer LoVo cells could promote cell invasion and migration [Citation47]. In this study, IGF2BP2 was discovered to act as the RBP to facilitate USP3 mRNA stability, but the expression and role of IGF2BP2 in NPC remain to be further explored.
Moreover, our study further confirmed that p65 could act as a transcription factor to enhance the expression of LINC01605. p65 has been reported to function as an oncogene in NPC progression [Citation48]. Therefore, a conclusion may be drawn that a positive feedback loop between LINC01605 and the NF-κB signalling pathway could promote NPC progression.
Supplemental Material
Download Zip (40.1 MB)Acknowledgments
Appreciate for all supports.
Disclosure statement
No potential conflict of interest was reported by the author(s).
Supplementary material
Supplemental data for this article can be accessed here.
Additional information
Funding
References
- Chen YP, Chan ATC, Le QT, et al. Nasopharyngeal carcinoma. Lancet. 2019;394:64–80.
- Chua MLK, Wee JTS, Hui EP, et al. Nasopharyngeal carcinoma. Lancet. 2016;387:1012–1024.
- Lam WKJ, Chan JYK. Recent advances in the management of nasopharyngeal carcinoma. F1000Res. 2018;7. DOI:10.12688/f1000research.15066.1
- Liu MT, Chen MK, Huang CC, et al. Prognostic value of molecular markers and implication for molecular targeted therapies in nasopharyngeal carcinoma: an update in an era of new targeted molecules development. World J Oncol. 2015;6:243–261.
- Wang J, Zhu S, Meng N, et al. ncRNA-encoded peptides or proteins and cancer. Mol Ther. 2019;27:1718–1725.
- Esteller M. Non-coding RNAs in human disease. Nat Rev Genet. 2011;12:861–874.
- Ma Y, Zhang J, Wen L, et al. Membrane-lipid associated lncRNA: a new regulator in cancer signaling. Cancer Lett. 2018;419:27–29.
- Kopp F, Mendell JT. Functional classification and experimental dissection of long noncoding RNAs. Cell. 2018;172:393–407.
- Zhang Y, Zhu R, Wang J, et al. Upregulation of lncRNA H19 promotes nasopharyngeal carcinoma proliferation and metastasis in let-7 dependent manner. Artif Cells Nanomed Biotechnol. 2019;47:3854–3861.
- Han R, Chen S, Wang J, et al. LncRNA UCA1 affects epithelial-mesenchymal transition, invasion, migration and apoptosis of nasopharyngeal carcinoma cells. Cell Cycle (Georgetown, Tex). 2019;18:3044–3053.
- Liu ZB, Tang C, Jin X, et al. Increased expression of lncRNA SNHG12 predicts a poor prognosis of nasopharyngeal carcinoma and regulates cell proliferation and metastasis by modulating Notch signal pathway. Cancer Biomarkers. 2018;23:603–613.
- DiDonato JA, Mercurio F, Karin M. NF-κB and the link between inflammation and cancer. Immunol Rev. 2012;246:379–400.
- Oeckinghaus A, Hayden MS, Ghosh S. Crosstalk in NF-κB signaling pathways. Nat Immunol. 2011;12:695–708.
- Perkins ND. The diverse and complex roles of NF-κB subunits in cancer. Nat Rev Cancer. 2012;12:121–132.
- Gilmore TD. Introduction to NF-kappaB: players, pathways, perspectives. Oncogene. 2006;25:6680–6684.
- Zhang W, Guo Q, Liu G, et al. NKILA represses nasopharyngeal carcinoma carcinogenesis and metastasis by NF-κB pathway inhibition. PLoS Genet. 2019;15:e1008325.
- Popovic D, Vucic D, Dikic I. Ubiquitination in disease pathogenesis and treatment. Nat Med. 2014;20:1242–1253.
- Zhou Q, Xiao Z, Zhou R, et al. Ubiquitin-specific protease 3 targets TRAF6 for deubiquitination and suppresses IL-1β induced chondrocyte apoptosis. Biochem Biophys Res Commun. 2019;514:482–489.
- Fan L, Chen Z, Wu X, et al. Ubiquitin-specific protease 3 promotes glioblastoma cell invasion and epithelial-mesenchymal transition via stabilizing snail. Mol Cancer Res. 2019;17:1975–1984.
- Parashar D, Geethadevi A, Aure MR, et al. miRNA551b-3p activates an oncostatin signaling module for the progression of triple-negative breast cancer. Cell Rep. 2019;29:4389–406.e10.
- Im K, Mareninov S, Diaz MFP, et al. An introduction to performing immunofluorescence staining. Methods Mol Biol. 2019;1897:299–311.
- Sun CC, Zhu W, Li SJ, et al. FOXC1-mediated LINC00301 facilitates tumor progression and triggers an immune-suppressing microenvironment in non-small cell lung cancer by regulating the HIF1α pathway. Genome Med. 2020;12:77.
- Wang SH, Ma F, Tang ZH, et al. Long non-coding RNA H19 regulates FOXM1 expression by competitively binding endogenous miR-342-3p in gallbladder cancer. J Exp Clin Cancer Res. 2016;35:160.
- Kim J, Piao HL, Kim BJ, et al. Long noncoding RNA MALAT1 suppresses breast cancer metastasis. Nat Genet. 2018;50:1705–1715.
- Qi X, Zhang DH, Wu N, et al. ceRNA in cancer: possible functions and clinical implications. J Med Genet. 2015;52:710–718.
- Young MJ, Hsu KC, Lin TE, et al. The role of ubiquitin-specific peptidases in cancer progression. J Biomed Sci. 2019;26:42.
- Udagawa T, Fujioka Y, Tanaka M, et al. FUS regulates AMPA receptor function and FTLD/ALS-associated behaviour via GluA1 mRNA stabilization. Nat Commun. 2015;6:7098.
- Yang H, Yang W, Dai W, et al. LINC00667 promotes the proliferation, migration, and pathological angiogenesis in non-small cell lung cancer through stabilizing VEGFA by EIF4A3. Cell Biol Int. 2020;44:1671–1680.
- Dhir T, Schultz CW, Jain A, et al. Abemaciclib is effective against pancreatic cancer cells and synergizes with HuR and YAP1 Inhibition. Mol Cancer Res. 2019;17:2029–2041.
- Shetty S. Regulation of urokinase receptor mRNA stability by hnRNP C in lung epithelial cells. Mol Cell Biochem. 2005;272:107–118.
- Ye S, Song W, Xu X, et al. IGF2BP2 promotes colorectal cancer cell proliferation and survival through interfering with RAF-1 degradation by miR-195. FEBS Lett. 2016;590:1641–1650.
- Zhu W, Men X. Negative feedback of NF-κB signaling by long noncoding RNA MALAT1 controls lipopolysaccharide-induced inflammation injury in human lung fibroblasts WI-38. J Cell Biochem. 2020;121:1945–1952.
- Wang Y, Chen W, Lian J, et al. The lncRNA PVT1 regulates nasopharyngeal carcinoma cell proliferation via activating the KAT2A acetyltransferase and stabilizing HIF-1α. Cell Death Differ. 2020;27:695–710.
- Li W, Wu X, She W. LncRNA POU3F3 promotes cancer cell migration and invasion in nasopharyngeal carcinoma by up-regulating TGF-β1. Biosci Rep. 2019;39. DOI:10.1042/BSR20181632
- Qin Z, Wang Y, Tang J, et al. High LINC01605 expression predicts poor prognosis and promotes tumor progression via up-regulation of MMP9 in bladder cancer. Biosci Rep. 2018;38. DOI:10.1042/BSR20180562
- Rinkenbaugh AL, Baldwin AS. The NF-κB pathway and cancer stem cells. Cells. 2016;5. DOI:10.3390/cells5020016
- Scheidereit C. IkappaB kinase complexes: gateways to NF-kappaB activation and transcription. Oncogene. 2006;25:6685–6705.
- Hayden MS, Ghosh S. Signaling to NF-kappaB. Genes Dev. 2004;18:2195–2224.
- Tay Y, Rinn J, Pandolfi PP. The multilayered complexity of ceRNA crosstalk and competition. Nature. 2014;505:344–352.
- Wang XY, Wang L, Xu PC, et al. LINC01605 promotes the proliferation of laryngeal squamous cell carcinoma through targeting miR-493-3p. Eur Rev Med Pharmacol Sci. 2019;23:10379–10386.
- Zhang W, Mao K, Liu S, et al. miR-942-5p promotes the proliferation and invasion of human melanoma cells by targeting DKK3. J Recept Signal Transduct Res. 2020;1–8:180–187.
- Wang Q, Wu J, Huang H, et al. lncRNA LIFR-AS1 suppresses invasion and metastasis of non-small cell lung cancer via the miR-942-5p/ZNF471 axis. Cancer Cell Int. 2020;20:180.
- Xu Q, Zhou L, Yang G, et al. Overexpression of circ_0001445 decelerates hepatocellular carcinoma progression by regulating miR-942-5p/ALX4 axis. Biotechnol Lett. 2020;42:2735–2747.
- Wu X, Liu M, Zhu H, et al. Ubiquitin-specific protease 3 promotes cell migration and invasion by interacting with and deubiquitinating SUZ12 in gastric cancer. J Exp Clin Cancer Res. 2019;38:277.
- Wu Y, Qin J, Li F, et al. USP3 promotes breast cancer cell proliferation by deubiquitinating KLF5. J Biol Chem. 2019;294:17837–17847.
- Huang RS, Zheng YL, Li C, et al. MicroRNA-485-5p suppresses growth and metastasis in non-small cell lung cancer cells by targeting IGF2BP2. Life Sci. 2018;199:104–111.
- Wu XL, Lu RY, Wang LK, et al. Long noncoding RNA HOTAIR silencing inhibits invasion and proliferation of human colon cancer LoVo cells via regulating IGF2BP2. J Cell Biochem. 2018. DOI:10.1016/j.biocel.2018.04.001.
- Lung HL, Kan R, Chau WY, et al. The anti-tumor function of the IKK inhibitor PS1145 and high levels of p65 and KLF4 are associated with the drug resistance in nasopharyngeal carcinoma cells. Sci Rep. 2019;9:12064.