ABSTRACT
Circular RNAs (circRNAs) are novel noncoding RNAs with covalently closed-loop structures that can regulate eukaryotic gene expression. Due to their stable structure, circRNAs are widely distributed in the cytoplasm and have important biological functions, including as microRNA sponges, RNA-binding protein conjugates, transcription regulators, and translation templates. Breast cancer is among the most common malignant cancers diagnosed in women worldwide. Despite the development of comprehensive treatments, breast cancer still has high mortality rates. Recent studies have unmasked critical roles for circRNAs in breast cancer as regulators of tumour initiation, progression, and metastasis. Further, research has revealed that some circRNAs have the potential for use as diagnostic and prognostic biomarkers in clinical practice. Herein, we review the biogenesis and biological functions of circRNAs, as well as their roles in different breast cancer subtypes. Moreover, we provide a comprehensive summary of the clinical significance of circRNAs in breast cancer. CircRNAs are believed to be a hot focus in basic and clinical research of breast cancer, and innovative future research directions of circRNAs could be used as biomarkers, therapeutic targets, or novel drugs.
Abbreviations: CeRNA: Competitive endogenous RNA; ciRNA: Circular intronic RNA; circRNA: Circular RNA; EIciRNA: Exon-intron circRNA; EMT: Epithelial–mesenchymal transition; IRES: Internal ribosome entry site; lncRNA: Long non-coding RNA; miRNA: MicroRNA; MRE: MiRNA response element; ncRNA: Non-coding RNA; RBP: RNA-binding protein; RNA-seq: RNA sequencing; RT-PCR: Reverse transcription-polymerase chain reaction
1. Introduction
Over recent years, breast cancer has become the most common malignant cancer in women worldwide, with high incidence and mortality rates [Citation1,Citation2]. Breast cancer is categorized into four major subtypes: Luminal A, Luminal B, HER2-positive, and triple-negative [Citation3]. The molecular mechanisms underlying breast cancer have yet to be fully elucidated [Citation4]. Further, although comprehensive treatments, including surgery, radiotherapy, chemotherapy, and targeted therapies, benefit a proportion of patients with breast cancer, many problems, such as individual treatment efficacy, drug resistance, prevention and management of recurrence, remain unsolved [Citation5,Citation6].
Circular RNAs (circRNAs), as newly discovered post-transcriptional regulators of gene expression, have been proven to play key roles in cancer progression [Citation7–10]. RNA sequencing (RNA-seq) techniques and sequence conservation analysis have revealed dysregulation of numerous circRNAs in cancer cells [Citation11,Citation12], which are not merely byproducts of alternative splicing [Citation13]. CircRNAs can be detected in peripheral blood plasma and exosomes [Citation14,Citation15], indicating their promise as potential cancer biomarkers. Public databases, such as CircInteractome, circBase, circRNADisease, MiOncoCirc, and Cancer-Specific CircRNA Databases (Supplement Table S1), are powerful tools for circRNA research, covering numerous circRNAs and predicting their functions, while exoRBase is a repository for circRNAs derived from human blood exosome RNA-seq data analyses [Citation33–35].
CircRNAs often present tissue-restricted and cancer-specific expression patterns. CircRNAs are abundant in breast cancer cells [Citation36], and high-throughput RNA sequencing has shown the significantly differentially expressed circRNAs in breast cancer and para-cancer tissues, tumours with different TNM stages or molecular subtypes, highlighting their clinical utility as a biomarker [Citation37]. Emerging studies that have reported the functions of circRNAs in breast cancer, which are involved in the tumour progression [Citation38,Citation39] and drug resistance [Citation16,Citation40]. CircRNAs are detectable in plasma, indicating their potential role as biomarkers for liquid biopsy. It is very encouraging that studies have found that cell-free circRNAs and extracellular vesicles circRNAs can both serve as circulating biomarkers for breast cancer [Citation41,Citation42]. In addition, circRNAs often play their important regulatory roles by circRNA-miRNA-mRNA axis, and the integration of detecting RNAs in circRNA-miRNA-mRNA axis may provide higher efficacy and accuracy for the development of breast cancer biomarkers.
Here, we review the biogenesis and biological functions of circRNAs, and discuss their broad clinical significance in breast cancer, including their potential for application in early diagnosis, prognosis evaluation, and therapeutic response assessment. CircRNAs that function in different breast cancer subtypes are also discussed in detail.
2. Discovery and biogenesis of circRnas
CircRNAs were first observed in the cytoplasm of eukaryotic cells in 1976 by electron microscopy and were considered virus analogs at that time [Citation43]. CircRNA transcripts from the SRY gene locus were first confirmed in 1993 [Citation44], although circRNAs were long considered the products of faulty splicing. Recent advances in high-throughput sequencing technologies and bioinformatic analyses have identified significant roles for circRNAs in regulating various biological processes [Citation45,Citation46]. Research on circRNA has faced stagnation for an extended period primarily due to its historical classification as a mere byproduct of RNA splicing. Nevertheless, circRNAs can now be precisely identified through RT-PCR following treatment with RNase R, which can digest linear RNAs [Citation47]. Another reason for the lack of attention on circRNAs may be that they were not mapped to the linear reference genome, leading to sequencing reads containing circRNAs being mistakenly deleted from sequencing data [Citation48]. Further, the lack of polyA tails on circRNA molecules means that they are generally removed during RNA sequencing library preparation [Citation49].
Unlike the standard splicing patterns of linear RNAs, circRNAs biogenesis occurs via a process referred to as back-splicing [Citation50,Citation51]. Competition between linear splicing and back-splicing of exons occurs in the transcription of human genes. Back-splicing is favoured by long flanking introns, inverted repeat elements like Alu elements and trans-acting RNA binding proteins (RBPs), such as RNA-binding protein FUS, protein quaking (HQK), NF90, and NF110 (both protein products of the interleukin enhancer-binding factor 3 gene) [Citation52,Citation53]. The base pairing between inverted repeat elements or RBPs’ dimerization brings a downstream splice-donor site (SD) close to an upstream splice-acceptor site (SA), facilitating back-splicing through the canonical splicing machinery.
In back-splicing, an upstream branch point (BP) attacks a downstream SD site, which then attacks an upstream SA site, leading to the formation of exon-intron circRNAs (EIcircRNAs) or exonic circRNAs where the internal intron is spliced out. These RBPs disrupt base pairing between inverted repeat elements, allowing the splicing machinery to produce linear mRNA [Citation54].
CircRNAs mainly localize to the cytoplasm [Citation55] and cannot easily be degraded by RNA exonucleases [Citation56]. Due to their high copy numbers and stable structures, circRNAs have been widely detected in various eukaryotic organisms [Citation34,Citation57,Citation58]. CircRNAs can be divided into three categories, based on their biogenesis patterns: exonic circRNAs (circRNAs in which the internal intron is spliced out), intronic circRNAs (circRNAs in which the exon is spliced out) [Citation59], and exon-intron circRNAs (EIciRNAs, comprising circRNAs containing both exon and intron sequences) [Citation60] (). (PMID: 34912049)
Figure 1. Classification of circRNAs and their biological functions in eukaryotic cells. three types of circRnas based on their mode of biosynthesis (1. EIciRNAs, 2. exonic circRNAs, 3. intronic circRNAs (CiRNA)) and their functions in the nucleus and cytoplasm of eukaryotic cells: I. circRnas act as sponges of miRnas; II. circRNAs as RBP conjugates; III. circRNAs as transcription regulators; IV. circRNAs as translation templates. CircRNA, circular RNA; ciRNA, circular intronic RNA; EIciRNA, exon-intron circRNA; EMT, epithelial to mesenchymal transition; miRNA, microRNA; RBP, RNA binding protein; pol II, RNA polymerase II.
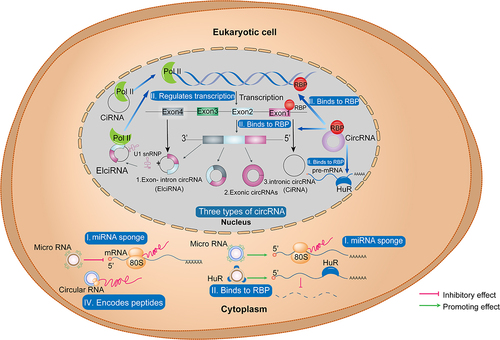
3. Biological functions of circRnas
The main ways by which circRNAs exert their biological functions in breast cancer cells include 1) by acting as microRNA (miRNA) sponges, thereby regulating downstream protein translation [Citation61] 2) binding to RNA-binding proteins (RBPs) in the nucleus, to regulate transcription, alternative splicing, and polyadenylation of pre-mRNAs; circRNAs also bind to RBPs (e.g. HuR) in the cytoplasm, where they induce mRNA degradation [Citation62,Citation63] 3) by interacting with RNA polymerase II and regulating transcription [Citation64], in which EIciRNAs can interact with U1 snRNP and promote transcription of their parental genes [Citation60]. Further, although circRNAs are non-coding RNAs, recent evidence shows that 4) some circRNAs have small open reading frames (ORFs), which can encode short peptides [Citation65,Citation66] (). Moreover, circRNAs can also form circRNP complexes, thereby influencing signalling pathways. In addition, circRNAs have been reported to serve as regulators of innate immunity [Citation37,Citation40]. Previous research has already confirmed the physiological functions of circRNA in various tumours, including regulating the cell cycle [Citation67], modulating autophagy and apoptosis [Citation68]. CircRNA is also closely related to cellular energy metabolism [Citation69] and is involved in the invasion and metastasis of tumour cells [Citation37,Citation69,Citation70]. Although research on circRNAs in breast cancer is still relatively limited, our review focused on discussing the biological roles that circRNAs play in breast cancer.
3.1 CircRnas as miRNA sponges
miRNAs are small non-coding RNAs that function in association with AGO proteins, which are major components of miRNA-induced silencing complexes (miRISCs) [Citation71,Citation72]. MiRISCs interact with and modulate the expression of target mRNAs via 3′-untranslated region (UTR) sequence complementarity [Citation73]. Further, miRNAs can regulate expression of tumour-associated genes in cancer cells [Citation74–76]. Many circRNAs participate in post-transcriptional gene regulation as miRNAs sponges, by blocking mRNA–miRNA interactions and regulating initiation of protein translation [Citation67,Citation77].
CircRNAs in tumour tissues are closely related to post-transcriptional regulation of miRNAs [Citation78,Citation79], thereby controlling the expression of cancer-related genes. As illustrated in , there is extensive evidence that circRNAs function as miRNA sponges in breast cancer. Hsa_circ_0000199 can interact with miR-613 and miR-206, leading to the inhibition of the PI3K/Akt/mTOR pathway [Citation31]. CircFBXW7, which acts as a sponge for miR-197-3p, was found to upregulate FBXW7 expression in triple negative breast cancer (TNBC) cells, thereby inducing c-MYC degradation [Citation27], while circNR3C2, as a sponge for miR-513a-3p, can promote HRD1 expression and inhibit epithelial to mesenchymal transition (EMT) in TNBC cells [Citation29]. Moreover, circ_0001667 attenuates Adriamycin resistance in MCF-7 and MDA-MB-231 cells by acting as a sponge for miR-4458, leading to upregulation of the NCOA3 expression [Citation80].
Figure 2. CircRnas as miRNA sponges in breast cancer. CircRNAs (circSEPT9, hsa_circ_0000199, circFBXW7, circNR3C2, circ_0001667) function as miRNA sponges in breast cancer. LIF, leukaemia inhibitory factor; Stat3, signal transducer and activator of transcription 3; PI3K, phosphoinositide 3-kinase; AKT, protein kinase B; mTOR, mammalian target of rapamycin; FBXW7, F-Box and WD repeat domain containing 7; HRD1, HMG-CoA reductase degradation 1; EMT, epidermal mesenchymal transition; NCOA3, nuclear receptor coactivator 3.
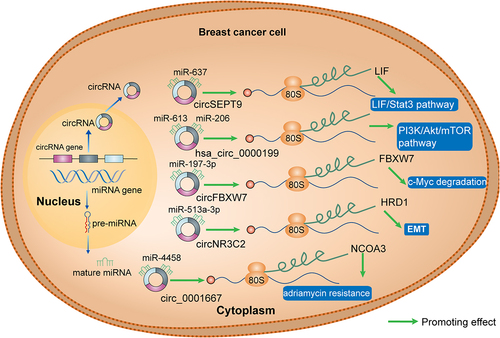
3.2 CircRnas as RBP conjugates
RBPs are proteins that bind to RNA through RNA-binding domains and are involved in RNA transcription, splicing, and polyadenylation of pre-mRNAs, as well as RNA degradation [Citation81–83]. Recent studies have shown that circRNA-RBP interactions play a critical role in breast cancer [Citation84–86]. HuR, a type of RBPs, is known to stabilize many mRNAs and modulate their translation [Citation87]. Yi et al. [Citation88] reported that circ-1073 can bind with HuR to act as a tumour suppressor in breast cancer, and circ-1073 increases cleaved-caspase 3/9 levels to facilitate breast cancer cell apoptosis [Citation88]. Further, circ-1073 influences EMT by upregulating E-cadherin and downregulating Vimentin expression. Through bioinformatics analysis and investigation of the underlying mechanisms, Wang et al. verified that hsa_circ_0068631 maintains c-MYC mRNA stability and upregulates c-MYC by binding to eukaryotic translation initiation factor 4A3 (EIF4A3) [Citation86], indicating that circRNAs can be critical regulators of c-MYC-mediated cell proliferation and glycolysis in breast cancer ().
Figure 3. CircRNAs as conjugates of RBPs in breast cancer. Has_circ_1073 binds with HuR to upregulate c-caspase 3/9 and inhibits EMT through regulating E-cadherin and vimentin expression; has_circ_0068631 binds to EIFA3, resulting in c-MYC upregulation. C-caspase, cleaved-caspase; EIFA3, eukaryotic translation initiation factor 3 subunit A; EMT, epithelial to mesenchymal transition; RBP, RNA binding protein.
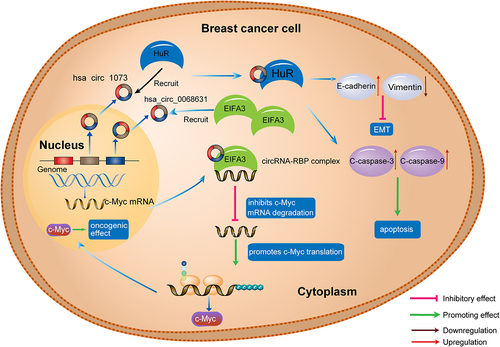
3.3 CircRnas as transcription regulators
CircRNAs can interact directly with RNA polymerase II to regulate gene transcription [Citation89,Citation90], while EIciRNA can interact with U1 snRNP and bind to RNA polymerase II (). CircScrib570, an antisense circRNA, suppresses pre-mRNA splicing and mRNA translation and upregulates E-cadherin, while downregulating N-cadherin and vimentin, thereby promoting cancer cell proliferation, migration, and invasion [Citation91]. Wang et al. illustrated that circACTN4 upregulates MYC mRNA transcription, which facilitates expression of the cell cycle-related proteins, CDK4 and CCND2 [Citation92], suggesting that circACTN4 can promote breast cancer development and metastasis by activating the proto-oncogene, MYC ().
Figure 4. CircRNAs as transcription regulators in breast cancer. by upregulating MYC mRNA transcription, circACTN4 facilitates CDK4 and CCND2 expression; circScrib570 regulates expression of E-cadherin, N-cadherin, and vimentin, resulting in elevated cancer cell proliferation, migration, and invasion. CDK4, cyclin dependent kinase 4; CCND2, cyclin D2; E-cad, E-cadherin; FIR, FBP-interacting repressor; FUSE, far upstream element; SCRIB, scribble planar cell polarity protein; USF2, upstream stimulatory factor 2.
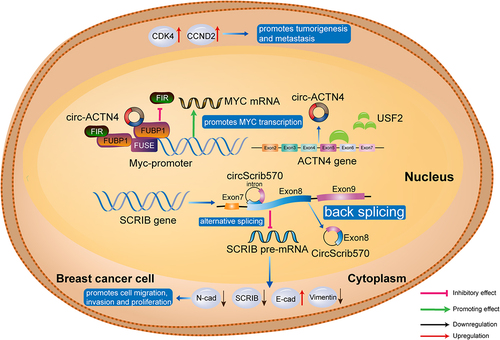
3.4 CircRNAs as templates for translation
In eukaryotic cells, the 5’ and 3’ UTRs of genes are fundamental to translation initiation [Citation93–95], and circRNAs are generally considered non-coding RNAs, due to their lack of 5’and 3’ ends [Citation96]. Recently, increasing evidence has supported the binding of some circRNAs to ribosomes [Citation8,Citation66,Citation97] and has also shown that they can contain initiation codons (AUG) and putative ORFs [Citation98]. These findings suggest that circRNAs are potential translation templates, and accumulating evidence indicates that circRNAs can encode peptides relevant in breast cancer. Ye et al. found that circFBXW77 encoded a 185 amino acid polypeptide (FBXW7-185aa), which inhibits TNBC cell proliferation and migration by increasing FBXW7 abundance and inducing c-MYC degradation [Citation27]. Li et al. reported that circ-HER2 encodes the HER2–103 peptide in TNBC cells, in which HER2–103 facilitates EGFR/EGFR homodimer and EGFR/HER3 heterodimer formation and contributes to PI3K-AKT pathway activation [Citation21]. Interestingly, the oncogenic phenotype induced by HER2–103 can be inhibited by pertuzumab, which exerts its anti-tumour effects by recognizing the CR I domain of HER2, suggesting that HER2–103 translated from circ-HER2 can promote pertuzumab resistance in TNBC cells [Citation21] ().
Figure 5. CircRNAs as translation templates in TNBC. circ-HER2 is translated to HER2–103 peptide in TNBC cells, and HER2–103 facilitates EGFR/EGFR homodimer and EGFR/HER3 heterodimer formation, resulting in PI3K-AKT pathway activation. Another circRNA, circFBXW7, encodes the protein, FBXW7–185aa, which interacts competitively with USP28, preventing USP28 from binding to FBXW7 and antagonizing USP28-induced c-MYC stabilization. AKT, protein kinase B; BC, breast cancer; EGFR, epidermal growth factor receptor; FBXW7, F-box and WD repeat domain containing 7; HER2, human epidermal growth factor receptor 2; USP28, ubiquitin specific peptidase 28.
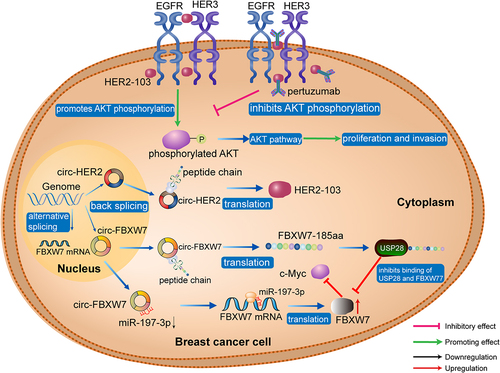
4. CircRnas in different breast cancer subtypes
4.1 CircRnas in ER+ breast cancer
The ER+ subtype accounts for three-quarters of all cases of breast cancer [Citation99,Citation100]. CircRNAs involved in ER+ breast cancer are listed in . Yuan et al. analysed the circRNA expression profile of ER+ breast cancer and adjacent non-tumour tissues using bioinformatics, and found that hsa_circ_0087378 was downregulated in ER+ breast cancer [Citation18]. Anti-hormonal therapy is the standard treatment for ER+ breast cancer, where tamoxifen has been the first-line drug for the treatment of premenopausal women for decades [Citation101,Citation102]. In a substantial number of patients, breast cancers develop resistance to tamoxifen, and noncoding RNAs, including circRNAs, contribute to this phenomenon [Citation103–105]. Sang et al. found that hsa_circ_0025202 expression was downregulated in ER+ breast cancer tissues and was negatively correlated with lymphatic metastasis and histological grade [Citation16]. Hsa_circ_0025202 acts as a sponge for miR-182-5p to regulate FOXO3a expression and activity, resulting in elevated tumour suppression and tamoxifen sensitivity [Citation16,Citation106]. In addition to endocrine therapy, CDK inhibitors are upcoming drugs for the treatment of ER+ breast cancer. CircPGR is involved in the cyclin pathway in ER+ breast cancer cells as a sponge of miR-301a-5p, thereby regulating the expression of multiple cell cycle genes [Citation17]. CircPGR is transcribed from the oestrogen-induced gene, PGR, and RNA-seq analysis showed that it can upregulate the expression of cyclins, including CDK1, CDK6, and CHEK2, to promote breast cancer cell proliferation [Citation17,Citation107]. Although accumulating evidence supports important roles for circRNAs in ER+ breast cancer, in vivo experiments and clinical studies remain very limited, and further exploration is warranted.
Table 1. CircRNAs in different breast cancer subtypes (from 2017 to 2022).
4.2 CircRnas in HER2+ breast cancer
HER2+ breast cancer is a highly aggressive breast cancer subtype [Citation108,Citation109]. Currently, HER2-directed antibody drugs (e.g. trastuzumab and pertuzumab) and HER2 tyrosine kinase inhibitors (e.g. lapatinib and others) are widely used as therapies for patients with HER2+ breast cancer [Citation109–111]. The potential involvement of circRNAs in anti-HER2 drug resistance has attracted considerable attention [Citation112–114] (). Ling et al. conducted high-throughput sequencing and RT-qPCR experiments demonstrating that circCDYL2 is overexpressed in trastuzumab-resistant HER2+ breast cancer [Citation40]. CircCDYL2 can stabilize GRB7 by preventing its ubiquitination-mediated degradation and enhancing its interaction with FAK, thus sustaining the downstream activities of AKT and ERK1/2. Wu et al. reported that circ-MMP11 is highly upregulated in HER2+ breast cancer cell lines [Citation19]. Mechanistically, circ-MMP11 regulates ANLN expression through sponging miR-153-3p, and overexpression of ANLN promotes HER2+ breast cancer cell viability and migration, as well as their resistance to lapatinib. Consistently, ANLN is upregulated in 1,085 breast cancer tissues, compared with 291 normal adjacent tissues, and is particularly strongly overexpressed in lapatinib-resistant breast cancer tissues, where its levels are negatively correlated with those of miR-153-3p [Citation19,Citation115].
Most of the circRNAs mentioned in this section act as miRNA sponges or RBP binding molecules in HER2+ breast cancer; however, Liang et al. found that circCDYL was negatively regulated by miR-92b-3p and that overexpression of circCDYL promoted HER2+ breast tumour progression in vivo [Citation20]. The underlying mechanisms may involve circCDYL promotion of HER2 positive breast cancer cell proliferation by activating the PI3K/AKT signalling pathway [Citation20].
4.3 CircRnas in TNBC
TNBC is recognized as the most malignant breast cancer subtype [Citation116,Citation117]. Clinically, TNBC has higher rates of metastasis and recurrence and is associated with a poor prognosis [Citation118,Citation119]. Further, TNBC tumours usually respond poorly to conventional chemotherapies and exhibit very limited response to targeted therapies [Citation120,Citation121]. Many studies have demonstrated that circRNAs participate in TNBC progression and drug resistance [Citation39,Citation122] ().
Some circRNAs act as regulators to promote TNBC. Zheng et al. found that circSEPT9 is expressed at significantly higher levels in the TNBC cell lines and tissues than in normal breast epithelial cells and adjacent normal tissues, respectively [Citation22]. CircEPSTI1 functions as a sponge for miR-4753 and miR-6809 to upregulate the expression of BCL11A, a novel gene identified to promote breast cancer development [Citation123], while circEPSTI1 knockdown can significantly inhibit TNBC cell proliferation [Citation23]. BCL11A is overexpressed in TNBC tissues relative to other breast cancer subtypes, which promotes tumorigenesis in vivo [Citation123]. The proto-oncogene, kinesin family member 4A (KIF4A), is highly expressed in various malignant tumours, including breast cancer [Citation25,Citation124,Citation125]. Circ-KIF4A regulates KIF4A expression as competing endogenous RNA [Citation25], in which circ-KIF4A sponges miRNA-375 to upregulate KIF4A levels, thereby promoting TNBC cell proliferation and metastasis. Zeng et al. found that circANKS1B expression is higher in TNBC tumours compared with adjacent normal tissues and is positively related to lymph node metastasis and later clinical stage [Citation32]. CircANKS1B acts as a sponge for miR-148a-3p and miR-152-3p, to increase the expression of the transcription factor, USF1, which can upregulate TGF-β1, thereby activating TGF-β1/Smad signalling and promoting EMT [Citation32,Citation126,Citation127].
Some circRNAs have functions in TNBC beyond their roles as miRNA sponges. CircHIF1A modulates NFIB expression and translocation at the transcription and translation levels in TNBC cells, leading to AKT/STAT3 activation and inhibition of P21 signalling, resulting in cancer cell EMT [Citation14]. Li et al. detected circ-HER2 expression in more than 30% of TNBC tumour tissue samples and found that it acts as a direct template for HER2–103 translation to promote TNBC cell proliferation and invasion [Citation21]. Interestingly, the HER2–103 amino acid sequence translated from circ-HER2 is highly homologous to the HER2 CR1 domain, which can be recognized by pertuzumab.
Some circRNAs are downregulated in patients with TNBC, and act as tumour inhibiting factors. Ya et al. proved that circNR3C2 is significantly downregulated in TNBC tissues relative to adjacent normal tissues and sponges miR-513a-3p to promote HRD1 expression [Citation29]. As an inhibitory factor, HRD1 was significantly downregulated in TNBC tissues, thus promoting TNBC cell proliferation, migration, invasion, and EMT [Citation128] (). Likewise, circ -0,044,234 is significantly downregulated in TNBC compared with non-TNBC tissue samples [Citation28]. Circ -0,044,234 suppresses TNBC progression through the circ_0044234/miR-135b-5p/GATA3-axis, in which GATA3 is a transcription factor that inhibits the TNBC progression and metastasis [Citation129,Citation130]. Xu et al. demonstrated that circTADA2A-E6 is downregulated in TNBC patient samples relative to normal mammary gland tissues and preferentially acts as an miR-203a-3p sponge to restore SOCS3 expression, leading to a more aggressive carcinogenic phenotype in TNBC [Citation131].
TNBC usually develops drug resistance to chemotherapy [Citation132], and circRNAs are proven to be involved in drug resistance in TNBC [Citation26]. Dou et al. found that high expression of circUBE2D2 in patients with TNBC is related to advanced TNM stage and a higher rate of lymph node metastases [Citation26]. CircUBE2D2 acts as an miR-512-3p sponge and upregulates CDCA3 expression, which is a trigger factor that initiates mitosis, while silencing of circUBE2D2 reduced Adriamycin resistance of TNBC cells [Citation133]. Another circRNA, circ_0001667, is upregulated in Adriamycin resistant tumour tissues compared to non-tumour tissues [Citation80], and targets miR-4458 to restore NCOA3 expression, thereby enhancing Adriamycin resistance [Citation80]. Wang et al. found that circWAC was highly expressed in TNBC tumours relative to adjacent tissues and was associated with worse overall survival of patients with TNBC [Citation30]. Further, downregulation of circWAC increased the sensitivity of TNBC cells to paclitaxel, as circWAC acts as an miR-142 sponge to upregulate WWP1 expression, which activates the PI3K/AKT pathway [Citation30,Citation134,Citation135].
5. Clinical significance of circRnas in breast cancer
Emerging studies have reported that circRNAs could serve as promising biomarkers in numerous types of cancer, including breast cancer. Below, we review recent studies reporting circRNAs as biomarkers for early diagnosis, prognosis evaluation, and therapeutic response assessment in patients with breast cancer. Only studies with sample sizes ≥ 50 were included in our review.
5.1 CircRnas in early diagnosis of breast cancer
The high morbidity associated with breast cancer makes early diagnosis an urgent requirement. Noninvasive liquid biopsies are increasingly being applied in early diagnosis of cancer, and cell-free circRNAs are one type of biomarker analysed using this approach. CircRNAs are stable with long half-lives (>48 h) and abundantly distributed in blood and other body fluids (e.g. urine), making them suitable as liquid biopsy biomarkers. Yin et al. screened hsa_circ_0001785 as significantly dysregulated in plasma specimens from five patients with breast cancer and paired healthy volunteers and showed that it had good diagnostic value in an extended study cohort comprising 20 patients with breast cancer [Citation136]. Further, they found that plasma hsa_circ_0001785 had better diagnostic accuracy (area under the receiver operating characteristic curve (AUC) value = 0.784) than CEA and CA153 and that plasma hsa_circ_0001785 level was strongly associated with histological grade, TNM stage, and distant metastasis [Citation136]. Hu et al. screened plasma from 378 patients with breast cancer and 102 healthy controls and validated hsa_circ_0008673 as the most significantly upregulated circRNAs in breast cancer, with good diagnostic value (AUC = 0.833) [Citation137]. Plasma hsa_circ_0008673 was remarkably downregulated after breast mastectomy, and its expression was positively associated with tumour size and distant metastasis [Citation137]. Li et al. screened hsa_circ_0104824 as significantly downregulated in breast cancer tissue and further validated its downregulation in peripheral blood samples from 83 patients with breast cancer, compared with levels in 49 healthy controls [Citation138]. ROC curve analysis revealed that hsa_circ_0104824 had an AUC value of 0.849, indicating its potential diagnostic value in breast cancer [Citation138]. Liu et al. collected blood samples from 95 patients with breast cancer and matched healthy controls, and found that plasma hsa_circ_0000615 was expressed at significantly higher levels in patients with breast cancer [Citation139]. The AUC value of plasma hsa_circ_0000615 for diagnosis was 0.904, and its high expression was closely associated with poor prognostic factors, including advanced tumour stage, lymph node metastasis, and higher recurrence risk [Citation139]. Wang et al. detected expression levels of hsa_circ_0000745, hsa_circ_0001531, and hsa_circ_0001640 in whole blood samples from 129, 19 and 13 patients with breast cancer, patients with benign breast tumours, and healthy controls, respectively, and found that the circRNAs were upregulated and had good diagnostic potential for breast cancer and that the combination of all three circRNAs had superior diagnostic potential, with an AUC value of 0.913 [Citation140].
Although circRNAs exhibited good diagnostic value for breast cancer with high AUC values in all the studies described above and were even better than classic biomarkers, such as CA153 and CEA in some reports, prospective investigations are needed to further assess their effectiveness in screening for early-stage breast cancer.
Several circRNAs have been found to be ectopically expressed in breast cancer tissue, of which some were identified as tumour promoters (e.g. circSEPT9 [Citation22] and hsa_circ_0005046 [Citation141]), while others were tumour suppressors (e.g. circVRK1 [Citation142], hsa_circ_0006220 [Citation143], hsa_circ_0043278 [Citation144], hsa_circ_0001073 [Citation88], hsa_circ_006054 [Citation145], hsa_circ_100219 [Citation145], and hsa_circ_406697 [Citation145]). All circRNAs reported to have value for early diagnosis of breast cancer, from studies with breast cancer sample size ≥ 50, published from 2017 to 2022, are listed in . Expression levels of circRNAs identified from breast cancer tissue samples require further investigation in blood or other body fluids, to validate whether they have value as non-invasive biomarkers for early diagnosis of breast cancer.
Table 2. CircRNAs for early diagnosis in patients with breast cancer (from 2017 to 2022).
5.2 CircRnas for evaluation of breast cancer prognosis
Survival statistics from the SEER database show that the 5-year relative survival rate for patients with breast cancer of all SEER stages combined is 90%, while that for patients with distant metastasis is only 29% [Citation147]. Good prognostic biomarkers can help identify patients with worse prognoses, such as those who may develop distant metastasis, for whom strict monitoring or intensive anti-cancer treatment should be recommended at an early stage. Many circRNAs have been identified as significantly related to the survival of patients with breast cancer and represent potentially attractive prognostic biomarkers. CircRNAs highlighted as having the potential for evaluating prognosis in patients with breast cancer in recent studies, including total breast cancer sample size ≥ 100, published from 2017 to 2022, are summarized in .
Table 3. CircRNAs for evaluating prognosis in patients with breast cancer (from 2017 to 2022).
Some circRNAs promote cancer progression and have been identified as unfavourable prognostic factors in patients with breast cancer (). Wang et al. assessed circZNF609 expression in 143 patients with breast cancer, and found that high levels of circZNF609 were significantly associated with lymph node metastasis, advanced TNM stage, and poor overall survival [Citation148]. Based on tumour samples from 136 patients with breast cancer, high hsa_circ_001783 levels were associated with poor clinical outcomes [Citation38]. Disease-free survival was significantly shorter in patients with high circ_001783 expression, and hsa_circ_001783 expression was significantly associated with tumour size, lymph node status, TNM stage, and TNBC subtype [Citation38]. In a cohort of 145 patients with breast cancer, hsa_circ_001569 was validated as an independent prognostic factor for 5-year overall survival, and its overexpression was associated with lymph node metastasis, advanced clinical stage, and shorter overall survival [Citation149]. Zeng et al. found that increased circANKS1B expression was significantly associated with advanced clinical stage, lymph node metastasis and shorter overall survival time [Citation32]. In a recent study conducted by Cai et al., lower hsa_circ_0000515 expression was associated with longer overall survival in a cohort of 340 patients with breast cancer [Citation152]. TNBC is an aggressive breast cancer subtype, with very unfavourable outcomes. Hence, identification of efficient biomarkers for TNBC monitoring is an urgent priority, and circRNAs may serve as novel biomarkers in this context. He et al. explored the clinical implications of circGFRA1 in 222 patients with TNBC, and found that its expression was positively associated with heavier tumour burden and significantly associated with shorter overall survival and disease-free survival [Citation24]. Chen et al. found that circEPSTI1 mediates TNBC progression and that high levels of circEPSTI1 were correlated with reduced survival in a cohort of 240 patients with TNBC [Citation23]. CircRAD18 has been proven to promote TNBC progression, and its expression is significantly upregulated in patients with TNBC [Citation150]. Further, analysis of the clinical relevance of circRAD18 revealed that high levels are significantly associated with later clinical stage, higher pathological grade, and poor overall survival in patients with TNBC [Citation150].
In addition, some circRNAs can suppress cancer progression and have been identified as favourable prognostic factors in breast cancer (). Based on a cohort of 283 patients with breast cancer, circ_ LARP4 was identified as significantly correlated with reduced tumour size, TNM stage, and better prognosis [Citation151]. Interestingly, circ_VRK1, which has a diagnostic value in breast cancer, is also an independent predictor of better overall survival in patients with breast cancer [Citation142]. In a cohort of 350 patients with breast cancer, the mean overall survival of patients with high circ_VRK1 expression was 4.8 months longer than those with low expression [Citation142]. Another circRNA, hsa_circ_0001073, has an AUC value as a biomarker for breast cancer reaching 0.99, and has a strong prognostic value in this context [Citation88]. Investigation of the clinical relevance of hsa_circ_0001073 in 132 patients with breast cancer showed that high expression was strongly associated with reduced tumour burden and longer relapse-free survival [Citation88]. Exosomes are 30–100 nm microvesicles released by most cells with a wide range of functional molecules including proteins and RNAs. As a liquid biopsy and a novel source of biomarkers, exosomes containing circRNAs have the potential for specificity and sensitivity in predictive, diagnostic, and prognostic analysis. For example, tumour exosomal cSERPINE2 can suppress breast cancer progression by modulating MALT1-NF-KB-IL-6 axis of tumour-associated macrophages, which may serve as a promising nanotherapeutic strategy for the treatment of breast cancer [Citation153].
CircRNAs which are favourable prognostic indicators in patients with TNBC have also been reported. Ye et al. recruited a cohort of 473 patients with breast cancer, including 275 TNBC cases, and demonstrated poor overall survival and disease-free survival of those with low circFBXW7 levels, showing that circFBXW7 was an independent prognostic factor in patients with TNBC [Citation27]. Cai et al. conducted a study of 107 patients with TNBC and found that circ-NOL10 was a promising prognostic indicator, with low circ-NOL10 expression associated with aggressive disease characteristics and shorter survival time [Citation84].
Although a number of circRNAs have been identified to have prognostic value in breast cancer, the possibility of their clinical application for evaluation of breast cancer prognosis requires further investigation. Most studies have focused on and evaluated the prognostic value of single circRNA molecules, while the development of a panel of valuable circRNAs may further improve the sensitivity and specificity of prognosis evaluation.
5.3 Predicting therapeutic response
Drug resistance is a major obstacle to effective treatment of patients with breast cancer, and chemotherapy is a standard treatment approach. Current first-line chemotherapies, including anthracycline-based regimens and taxanes, have response rates of 30%–70%, while response rates to second- or third-line chemotherapies (e.g. paclitaxel, gemcitabine, and capecitabine, among others) tend to be much lower (20%–30%) [Citation154]. Chemotherapy resistance can arise through several different mechanisms, and circRNAs have been identified as involved in these processes. Interestingly, some circRNAs are ectopically expressed in chemotherapy-resistant tumour tissue, which may indicate their role in therapeutic responses (). Circ-RNF111 [Citation157], circ-ABCB10 [Citation156], and hsa_circ_0006528 [Citation155] are reported to contribute to paclitaxel resistance, and their expression is upregulated in paclitaxel-resistant breast cancer tissues relative to paclitaxel-sensitive tissues. Further, hsa_circ_0006528 is also expressed at higher levels in Adriamycin-resistant breast cancer tissues, and high hsa_circ_0006528 is associated with worse patient outcomes [Citation158]. Another circRNA, hsa_circ_0001667, is expressed at significantly higher levels in Adriamycin-resistant breast cancer tissues relative to those in Adriamycin-sensitive tissues [Citation80]. Anti-HER2 therapy is the most important targeted treatment for HER2+ breast cancer, although resistance to anti-HER2 therapy is also common [Citation161]. Trastuzumab is the most commonly used anti-HER2 agent, and Hsa_circ_0001598 [Citation159] and circ-BGN [Citation160] were observed to be clearly upregulated in trastuzumab-resistant breast cancer tissues and related to poor patient survival (). Interestingly, exosome-transmitted circHIPK3 was found to induce trastuzumab chemoresistance in drug-sensitive breast cancer cells [Citation162].
Table 4. CircRNAs indicating therapeutic response in patients with breast cancer (from 2017 to 2022).
Current research into the relationships between circRNAs and therapeutic response is very limited and superficial. Studies to date have been limited to demonstrating that expression of some circRNAs is dysregulated in drug-resistant tumour tissues relative to drug-sensitive tissues but have not reported accurate sensitivity or specificity values of these circRNA as monitoring biomarkers for therapeutic response. Further, discovery of circRNAs suitable for assessment by liquid biopsy to monitor therapeutic response would be preferable and improve the feasibility of their application.
6. Conclusions and future perspectives
With the rapid development of RNA-Seq and bioinformatics analytic tools, the key roles of circRNAs in regulating cellular biology in breast cancer have been partially elucidated [Citation146,Citation163,Citation164]. These abundant and stable noncoding RNAs play vital roles in the circRNA-miRNA-protein axis. As circRNAs can also be located in the nucleus, where they bind to RBPs, some studies have reported their roles in regulating alternative splicing and mRNA degradation. Furthermore, circRNAs have functions involving their roles as transcription regulators and protein translation templates.
In recent years, studies have demonstrated the clinical prospects for the use of circRNAs as tumour biomarkers. In this review, we summarize the biological functions of circRNAs in different breast cancer subtypes, including their regulatory functions in breast cancer cell proliferation, migration, invasion, EMT, and drug resistance. As circRNAs are stable in exosomes and blood plasma, they have promising advantages as potential biomarkers for early diagnosis, prognosis evaluation, and monitoring therapeutic response in patients with breast cancer[Citation165].
Nevertheless, research on circRNAs in breast cancer remains insufficient and the application of circRNAs in clinical practice for diagnosing, treating, and monitoring breast cancer will require considerable further development. We suggest the following approaches to study circRNAs in breast cancer in the future. First, more in vivo experiments to provide solid evidence explaining the roles of circRNAs in breast cancer. Second, given the heterogeneity of breast cancer, more attention needs to be paid to the mechanisms of actions and biological functions of key circRNAs involved in the four breast cancer subtypes; for example, different subtypes may have distinct driver circRNAs, and one circRNA may have totally different functions in different breast cancer subtypes. Third, as regulators, the functions of circRNAs depend on numerous other molecules, such as miRNAs and mRNAs, and the interplay among circRNAs and these molecules should be considered in exploration of circRNA regulatory networks; for example, sequencing of circRNAs, miRNAs, and mRNAs could be conducted synchronously in the same batch of specimens. Fourth, the emerging role of exosomal circRNAs warrants close attention; in particular, their unique biological functions in breast cancer metastasis and potential value as liquid biopsy biomarkers. Finally, numerous clinical studies and multi-centre clinical trials are required, as the ultimate goal of circRNA research in breast cancer is to apply circRNAs in clinical practice; however, with the development of techniques for screening circRNAs and updating of experimental tools for researching into circRNAs, we believe that the roles of circRNAs in breast cancer can be clearly determined, and ultimately have potential to benefit patients with breast cancer in clinical practice.
Author’s contributions
JJ Zhou and L Wang planned and designed this review. JJ Zhou and L Wang drew the outline of the review. JJ Zhou, ZW Wang, H Deng and Y Jin wrote the manuscript. H Deng drew the figure. ZW Wang, H Deng and Y Jin drew the tables. Meng L, J Huang, J Wang and K Zhang participated in searching clinical studies for circRNAs in breast cancer. All authors have read and approved the final manuscript.
Consent for publication
All authors have read and approved the final manuscript.
Supplemental Material
Download MS Word (18.7 KB)Acknowledgments
This study was supported by grants from the Natural Science Foundation of Zhejiang Province (Grant No. LY21H160039 to JJ Zhou), the National Natural Science Foundation of China (Grant No. 82172344 to JJ Zhou), the funding of Medical Science and Technology Project of Zhejiang Province (Grant No. 2022RC174 to JJ Zhou).
Disclosure statement
No potential conflict of interest was reported by the authors.
Supplementary material
Supplemental data for this article can be accessed online at https://doi.org/10.1080/15476286.2023.2272468
Additional information
Funding
References
- Heer E, Harper A, Escandor N, et al. Global burden and trends in premenopausal and postmenopausal breast cancer: a population-based study. Lancet Glob Health. 2020;8(8):e1027–e1037. doi: 10.1016/s2214-109x(20)30215-1
- Sung H, Ferlay J, Siegel RL, et al. Global cancer statistics 2020: GLOBOCAN estimates of incidence and mortality worldwide for 36 cancers in 185 countries. CA Cancer J Clin. 2021;71(3):209–249. doi: 10.3322/caac.21660
- Holm J, Eriksson L, Ploner A, et al. Assessment of breast cancer risk factors reveals subtype heterogeneity. Cancer Res. 2017;77(13):3708–3717. doi: 10.1158/0008-5472.Can-16-2574
- Gong Y, Ji P, Yang Y-S, et al. Metabolic-pathway-based subtyping of triple-negative breast cancer reveals potential therapeutic targets. Cell Metab. 2021;33(1):51–64 e59. doi: 10.1016/j.cmet.2020.10.012
- Giuliano AE, Connolly JL, Edge SB, et al. Breast cancer—major changes in the American joint committee on cancer eighth edition cancer staging manual. CA Cancer J Clin. 2017;67(4):290–303. doi: 10.3322/caac.21393
- Jin X, Demere Z, Nair K, et al. A metastasis map of human cancer cell lines. Nature. 2020;588(7837):331–336. doi: 10.1038/s41586-020-2969-2
- Burgess DJ. Epigenetics: therapy-induced transcription is cryptically widespread. Nat Rev Cancer. 2017;17(8):456. doi: 10.1038/nrc.2017.57
- Zhang M, Zhao K, Xu X, et al. A peptide encoded by circular form of LINC-PINT suppresses oncogenic transcriptional elongation in glioblastoma. Nat Commun. 2018;9(1):4475. doi: 10.1038/s41467-018-06862-2
- Jiang T, Xia Y, Lv J, et al. A novel protein encoded by circMAPK1 inhibits progression of gastric cancer by suppressing activation of MAPK signaling. Mol Cancer. 2021;20(1):66. doi: 10.1186/s12943-021-01358-y
- Grosselin K, Durand A, Marsolier J, et al. High-throughput single-cell ChIP-seq identifies heterogeneity of chromatin states in breast cancer. Nature Genet. 2019;51(6):1060–1066. doi: 10.1038/s41588-019-0424-9
- Memczak S, Jens M, Elefsinioti A, et al. Circular RNAs are a large class of animal RNAs with regulatory potency. Nature. 2013;495(7441):333–338. doi: 10.1038/nature11928
- Dong R, Ma XK, Li GW, et al. Circpedia v2: an updated database for comprehensive circular RNA annotation and expression comparison. Int J Genomics Proteomics. 2018;16(4):226–233. doi: 10.1016/j.gpb.2018.08.001
- Conn SJ, Pillman K, Toubia J, et al. The RNA binding protein quaking regulates formation of circRnas. Cell. 2015;160(6):1125–1134. doi: 10.1016/j.cell.2015.02.014
- Chen T, Wang X, Li C, et al. CircHIF1A regulated by FUS accelerates triple-negative breast cancer progression by modulating NFIB expression and translocation. Oncogene. 2021;40(15):2756–2771. doi: 10.1038/s41388-021-01739-z
- Du WW, Li X, Ma J, et al. Promotion of tumor progression by exosome transmission of circular RNA circSKA3. Mol Ther Nucleic Acids. 2022;27:276–292. doi: 10.1016/j.omtn.2021.11.027
- Sang Y, Chen B, Song X, et al. circRNA_0025202 regulates tamoxifen sensitivity and tumor progression via regulating the miR-182-5p/FOXO3a axis in breast cancer. Mol Ther. 2019;27(9):1638–1652. doi: 10.1016/j.ymthe.2019.05.011
- Wang L, Yi J, Lu L-Y, et al. Estrogen-induced circRNA, circPGR, functions as a ceRNA to promote estrogen receptor-positive breast cancer cell growth by regulating cell cycle-related genes. Theranostics. 2021;11(4):1732–1752. doi: 10.7150/thno.45302
- Yuan C, Zhou L, Zhang L, et al. Identification and integrated analysis of key differentially expressed circular RNAs in ER-positive subtype breast cancer. Epigenomics. 2019;11(3):297–321. doi: 10.2217/epi-2018-0147
- Wu X, Ren Y, Yao R, et al. Circular RNA circ-MMP11 contributes to lapatinib resistance of breast cancer cells by regulating the miR-153-3p/ANLN axis. Front Oncol. 2021;11:639961. doi: 10.3389/fonc.2021.639961
- Liang G, Ling Y, Lin Q, et al. MiR-92b-3p inhibits proliferation of HER2-positive breast cancer cell by targeting circCDYL. Front Cell Dev Biol. 2021;9:707049. doi: 10.3389/fcell.2021.707049
- Li J, Ma M, Yang X, et al. Circular HER2 RNA positive triple negative breast cancer is sensitive to pertuzumab. Mol Cancer. 2020;19(1):142. doi: 10.1186/s12943-020-01259-6
- Zheng X, Huang M, Xing L, et al. The circRNA circSEPT9 mediated by E2F1 and EIF4A3 facilitates the carcinogenesis and development of triple-negative breast cancer. Mol Cancer. 2020;19(1):73. doi: 10.1186/s12943-020-01183-9
- Chen B, Wei W, Huang X, et al. circEPSTI1 as a prognostic Marker and Mediator of triple-negative breast cancer progression. Theranostics. 2018;8(14):4003–4015. doi: 10.7150/thno.24106
- He R, Liu P, Xie X, et al. circGFRA1 and GFRA1 act as ceRnas in triple negative breast cancer by regulating miR-34a. J exper cli can res : CR. 2017;36(1):145. doi: 10.1186/s13046-017-0614-1
- Tang H, Huang X, Wang J, et al. circKIF4A acts as a prognostic factor and mediator to regulate the progression of triple-negative breast cancer. Mol Cancer. 2019;18(1):23. doi: 10.1186/s12943-019-0946-x
- Dou D, Ren X, Han M, et al. CircUBE2D2 (hsa_circ_0005728) promotes cell proliferation, metastasis and chemoresistance in triple-negative breast cancer by regulating miR-512-3p/CDCA3 axis. Cancer Cell Int. 2020;20(1):454. doi: 10.1186/s12935-020-01547-7
- Ye F, Gao G, Zou Y, et al. circFBXW7 inhibits malignant progression by sponging miR-197-3p and encoding a 185-aa protein in triple-negative breast cancer. Mol Ther Nucl Acids. 2019;18:88–98. doi: 10.1016/j.omtn.2019.07.023
- Darbeheshti F, Zokaei E, Mansoori Y, et al. Circular RNA hsa_circ_0044234 as distinct molecular signature of triple negative breast cancer: a potential regulator of GATA3. Cancer Cell Int. 2021;21(1):312. doi: 10.1186/s12935-021-02015-6
- Fan Y, Wang J, Jin W, et al. CircNR3C2 promotes HRD1-mediated tumor-suppressive effect via sponging miR-513a-3p in triple-negative breast cancer. Mol Cancer. 2021;20(1):25. doi: 10.1186/s12943-021-01321-x
- Wang L, Zhou Y, Jiang L, et al. CircWAC induces chemotherapeutic resistance in triple-negative breast cancer by targeting miR-142, upregulating WWP1 and activating the PI3K/AKT pathway. Mol Cancer. 2021;20(1):43. doi: 10.1186/s12943-021-01332-8
- Li H, Xu W, Xia Z, et al. Hsa_circ_0000199 facilitates chemo-tolerance of triple-negative breast cancer by interfering with miR-206/613-led PI3K/Akt/mTOR signaling. Aging. 2021;13(3):4522–4551. doi: 10.18632/aging.202415
- Zeng K, He B, Yang BB, et al. The pro-metastasis effect of circANKS1B in breast cancer. Mol Cancer. 2018;17(1):160. doi: 10.1186/s12943-018-0914-x
- Li JH, Liu S, Zhou H, et al. starBase v2.0: decoding miRNA-ceRNA, miRNA-ncRNA and protein–RNA interaction networks from large-scale CLIP-Seq data. Nucleic Acids Res. 2014;42(D1):D92–97. doi: 10.1093/nar/gkt1248
- Vo JN, Cieslik M, Zhang Y, et al. The landscape of circular RNA in cancer. Cell. 2019;176(4):869–881.e813. doi: 10.1016/j.cell.2018.12.021
- Li S, Li Y, Chen B, et al. exoRbase: a database of circRNA, lncRNA and mRNA in human blood exosomes. Nucleic Acids Res. 2018;46(D1):D106–d112. doi: 10.1093/nar/gkx891
- Ruan H, Xiang Y, Ko J, et al. Comprehensive characterization of circular RNAs in ~ 1000 human cancer cell lines. Genome Med. 2019;11(1):55. doi: 10.1186/s13073-019-0663-5
- Kristensen LS, Jakobsen T, Hager H, et al. The emerging roles of circRnas in cancer and oncology. Nat Rev Clin Oncol. 2022;19(3):188–206. doi: 10.1038/s41571-021-00585-y
- Liu Z, Zhou Y, Liang G, et al. Circular RNA hsa_circ_001783 regulates breast cancer progression via sponging miR-200c-3p. Cell Death Dis. 2019;10(2):55. doi: 10.1038/s41419-018-1287-1
- Li J, Gao X, Zhang Z, et al. CircCD44 plays oncogenic roles in triple-negative breast cancer by modulating the miR-502–5p/KRAS and IGF2BP2/Myc axes. Mol Cancer. 2021;20(1):138. doi: 10.1186/s12943-021-01444-1
- Ling Y, Liang G, Lin Q, et al. circCDYL2 promotes trastuzumab resistance via sustaining HER2 downstream signaling in breast cancer. Mol Cancer. 2022;21(1):8. doi: 10.1186/s12943-021-01476-7
- Yu Y, Zheng W, Ji C, et al. Tumor-derived circRnas as circulating biomarkers for breast cancer. Front Pharmacol. 2022;13:811856. doi: 10.3389/fphar.2022.811856
- Lin L, Cai G-X, Zhai X-M, et al. Plasma-derived extracellular vesicles circular RNAs serve as biomarkers for breast cancer diagnosis. Front Oncol. 2021;11:752651. doi: 10.3389/fonc.2021.752651
- Sanger HL, Klotz G, Riesner D, et al. Viroids are single-stranded covalently closed circular RNA molecules existing as highly base-paired rod-like structures. Proc Natl Acad Sci U S A. 1976;73(11):3852–3856. doi: 10.1073/pnas.73.11.3852
- Capel B, Swain A, Nicolis S, et al. Circular transcripts of the testis-determining gene sry in adult mouse testis. Cell. 1993;73(5):1019–1030. doi: 10.1016/0092-8674(93)90279-y
- Zheng Q, Bao C, Guo W, et al. Circular RNA profiling reveals an abundant circHIPK3 that regulates cell growth by sponging multiple miRnas. Nat Commun. 2016;7(1):11215. doi: 10.1038/ncomms11215
- Chen L, Wang C, Sun H, et al. The bioinformatics toolbox for circRNA discovery and analysis. Brief Bioinform. 2021;22(2):1706–1728. doi: 10.1093/bib/bbaa001
- Panda AC, De S, Grammatikakis I, et al. High-purity circular RNA isolation method (RPAD) reveals vast collection of intronic circRnas. Nucleic Acids Res. 2017;45(12):e116. doi: 10.1093/nar/gkx297
- Venø MT, Hansen TB, Venø ST, et al. Spatio-temporal regulation of circular RNA expression during porcine embryonic brain development. Genome Biol. 2015;16(1):245. doi: 10.1186/s13059-015-0801-3
- Guo JU, Agarwal V, Guo H, et al. Expanded identification and characterization of mammalian circular RNAs. Genome Biol. 2014;15(7):409. doi: 10.1186/s13059-014-0409-z
- Zhang XO, Dong R, Zhang Y, et al. Diverse alternative back-splicing and alternative splicing landscape of circular RNAs. Genome Res. 2016;26(9):1277–1287. doi: 10.1101/gr.202895.115
- Feng J, Chen K, Dong X, et al. Genome-wide identification of cancer-specific alternative splicing in circRNA. Mol Cancer. 2019;18(1):35. doi: 10.1186/s12943-019-0996-0
- Errichelli L, Dini Modigliani S, Laneve P, et al. FUS affects circular RNA expression in murine embryonic stem cell-derived motor neurons. Nat Commun. 2017;8(1):14741. doi: 10.1038/ncomms14741
- Li X, Liu C-X, Xue W, et al. Coordinated circRNA biogenesis and function with NF90/NF110 in viral infection. Mol Cell. 2017;67(2):214–227.e217. doi: 10.1016/j.molcel.2017.05.023
- Kristensen LS, Andersen MS, Stagsted LVW, et al. The biogenesis, biology and characterization of circular RNAs. Nat Rev Genet. 2019;20(11):675–691. doi: 10.1038/s41576-019-0158-7
- Wu G, Zhou W, Pan X, et al. RETRACTED: circular RNA profiling reveals exosomal circ_0006156 as a novel biomarker in papillary thyroid cancer. Mol Ther Nucleic Acids. 2020;19:1134–1144. doi: 10.1016/j.omtn.2019.12.025
- Jeck WR, Sharpless NE. Detecting and characterizing circular RNAs. Nat Biotechnol. 2014;32(5):453–461. doi: 10.1038/nbt.2890
- Starke S, Jost I, Rossbach O, et al. Exon circularization requires canonical splice signals. Cell Rep. 2015;10(1):103–111. doi: 10.1016/j.celrep.2014.12.002
- Wang PL, Bao Y, Yee M-C, et al. Circular RNA is expressed across the eukaryotic tree of life. PLoS One. 2014;9(3):e90859. doi: 10.1371/journal.pone.0090859
- Ivanov A, Memczak S, Wyler E, et al. Analysis of intron sequences reveals hallmarks of circular RNA biogenesis in animals. Cell Rep. 2015;10(2):170–177. doi: 10.1016/j.celrep.2014.12.019
- Li Z, Huang C, Bao C, et al. Exon-intron circular RNAs regulate transcription in the nucleus. Nat Struct Mol Biol. 2015;22(3):256–264. doi: 10.1038/nsmb.2959
- Bartel DP. MicroRNAs: target recognition and regulatory functions. Cell. 2009;136(2):215–233. doi: 10.1016/j.cell.2009.01.002
- Chen J, Wu Y, Luo X, et al. Circular RNA circRHOBTB3 represses metastasis by regulating the HuR-mediated mRNA stability of PTBP1 in colorectal cancer. Theranostics. 2021;11(15):7507–7526. doi: 10.7150/thno.59546
- Okholm TLH, Sathe S, Park SS, et al. Transcriptome-wide profiles of circular RNA and RNA-binding protein interactions reveal effects on circular RNA biogenesis and cancer pathway expression. Genome Med. 2020;12(1):112. doi: 10.1186/s13073-020-00812-8
- Zhang Y, Zhang X-O, Chen T, et al. Circular intronic long noncoding RNAs. Mol Cell. 2013;51(6):792–806. doi: 10.1016/j.molcel.2013.08.017
- Chen CK, Cheng R, Demeter J, et al. Structured elements drive extensive circular RNA translation. Mol Cell. 2021;81(20):4300–4318.e4313. doi: 10.1016/j.molcel.2021.07.042
- Yang Y, Fan X, Mao M, et al. Extensive translation of circular RNAs driven by N(6)-methyladenosine. Cell Res. 2017;27(5):626–641. doi: 10.1038/cr.2017.31
- Cheng Z, Yu C, Cui S, et al. circTP63 functions as a ceRNA to promote lung squamous cell carcinoma progression by upregulating FOXM1. Nat Commun. 2019;10(1):3200. doi: 10.1038/s41467-019-11162-4
- Geng Y, Bao Y, Deng L, et al. Circular RNA hsa_circ_0014130 inhibits apoptosis in non–small cell lung cancer by sponging miR-136-5p and upregulating BCL2. Mol Cancer Res. 2020;18(5):748–756. doi: 10.1158/1541-7786.Mcr-19-0998
- Yu L, Shi J, Cheng S, et al. Estrogen promotes prostate cancer cell migration via paracrine release of ENO1 from stromal cells. Mol Endocrinol. 2012;26(9):1521–1530. doi: 10.1210/me.2012-1006
- Shen P, Yang T, Chen Q, et al. CircNEIL3 regulatory loop promotes pancreatic ductal adenocarcinoma progression via miRNA sponging and A-to-I RNA-editing. Mol Cancer. 2021;20(1):51. doi: 10.1186/s12943-021-01333-7
- Eystathioy T, Chan EKL, Tenenbaum SA, et al. A phosphorylated cytoplasmic autoantigen, GW182, associates with a unique population of human mRnas within novel cytoplasmic speckles. Mol Biol Cell. 2002;13(4):1338–1351. doi: 10.1091/mbc.01-11-0544
- Yang Z, Jakymiw A, Wood MR, et al. GW182 is critical for the stability of GW bodies expressed during the cell cycle and cell proliferation. J Cell Sci. 2004;117(23):5567–5578. doi: 10.1242/jcs.01477
- Zhang L, Ding L, Cheung TH, et al. Systematic identification of C. elegans miRISC proteins, miRnas, and mRNA targets by their interactions with GW182 proteins AIN-1 and AIN-2. Mol Cell. 2007;28(4):598–613. doi: 10.1016/j.molcel.2007.09.014
- Rupaimoole R, Slack FJ. MicroRNA therapeutics: towards a new era for the management of cancer and other diseases. Nat Rev Drug Discov. 2017;16(3):203–222. doi: 10.1038/nrd.2016.246
- Bonci D, Coppola V, Musumeci M, et al. The miR-15a–miR-16-1 cluster controls prostate cancer by targeting multiple oncogenic activities. Nat Med. 2008;14(11):1271–1277. doi: 10.1038/nm.1880
- Callegari E, Elamin BK, Giannone F, et al. Liver tumorigenicity promoted by microRNA-221 in a mouse transgenic model. Hepatology. 2012;56(3):1025–1033. doi: 10.1002/hep.25747
- Xia S, Feng J, Chen K, et al. CSCD: a database for cancer-specific circular RNAs. Nucleic Acids Res. 2018;46(D1):D925–d929. doi: 10.1093/nar/gkx863
- Hansen TB, Jensen TI, Clausen BH, et al. Natural RNA circles function as efficient microRNA sponges. Nature. 2013;495(7441):384–388. doi: 10.1038/nature11993
- Tay Y, Rinn J, Pandolfi PP. The multilayered complexity of ceRNA crosstalk and competition. Nature. 2014;505(7483):344–352. doi: 10.1038/nature12986
- Cui Y, Fan J, Shi W, et al. Circ_0001667 knockdown blocks cancer progression and attenuates adriamycin resistance by depleting NCOA3 via releasing miR-4458 in breast cancer. Drug Dev Res. 2022;83(1):75–87. doi: 10.1002/ddr.21845
- Akira S, Maeda K. Control of RNA stability in immunity. Annu Rev Immunol. 2021;39(1):481–509. doi: 10.1146/annurev-immunol-101819-075147
- Nyati KK, Zaman MM, Sharma P, et al. Arid5a, an RNA-Binding protein in Immune regulation: RNA stability, inflammation, and autoimmunity. Trends Immunol. 2020;41(3):255–268. doi: 10.1016/j.it.2020.01.004
- Xia Z, Donehower LA, Cooper TA, et al. Dynamic analyses of alternative polyadenylation from RNA-seq reveal a 3′-UTR landscape across seven tumour types. Nat Commun. 2014;5(1). doi: 10.1038/ncomms6274
- Cai Y, Zhao X, Chen D, et al. Circ-NOL10 regulated by MTDH/CASC3 inhibits breast cancer progression and metastasis via multiple miRnas and PDCD4. Mol Ther Nucl Acids. 2021;26:773–786. doi: 10.1016/j.omtn.2021.09.013
- Ng WL, Mohd Mohidin TB, Shukla K. Functional role of circular RNAs in cancer development and progression. RNA Biol. 2018;15:995–1005. doi: 10.1080/15476286.2018.1486659
- Wang X, Chen M, Fang L. hsa_circ_0068631 promotes breast cancer progression through c-myc by binding to EIF4A3. Mol Ther Nucleic Acids. 2021;26:122–134. doi: 10.1016/j.omtn.2021.07.003
- Lebedeva S, Jens M, Theil K, et al. Transcriptome-wide analysis of regulatory interactions of the RNA-binding protein HuR. Mol Cell. 2011;43(3):340–352. doi: 10.1016/j.molcel.2011.06.008
- Yi Z, Li Y, Wu Y, et al. Circular RNA 0001073 attenuates malignant biological behaviours in breast cancer cell and is delivered by Nanoparticles to inhibit mice tumour growth. Onco Targets Ther. 2020;13:6157–6169. doi: 10.2147/ott.S248822
- Ashwal-Fluss R, Meyer M, Pamudurti N, et al. circRNA biogenesis competes with pre-mRNA splicing. Mol Cell. 2014;56(1):55–66. doi: 10.1016/j.molcel.2014.08.019
- Modahl LE, Macnaughton TB, Zhu N, et al. RNA-Dependent replication and transcription of hepatitis delta virus RNA involve distinct cellular RNA polymerases. Mol Cell Biol. 2000;20(16):6030–6039. doi: 10.1128/mcb.20.16.6030-6039.2000
- Ma J, Du WW, Zeng K, et al. An antisense circular RNA circSCRIB enhances cancer progression by suppressing parental gene splicing and translation. Mol Ther. 2021;29(9):2754–2768. doi: 10.1016/j.ymthe.2021.08.002
- Wang X, Xing L, Yang R, et al. The circACTN4 interacts with FUBP1 to promote tumorigenesis and progression of breast cancer by regulating the expression of proto-oncogene MYC. Mol Cancer. 2021;20(1):91. doi: 10.1186/s12943-021-01383-x
- Meyer KD, Patil D, Zhou J, et al. 5′ UTR m6A promotes cap-independent translation. Cell. 2015;163(4):999–1010. doi: 10.1016/j.cell.2015.10.012
- Wagner S, Herrmannová A, Hronová V, et al. Selective translation complex profiling reveals staged initiation and co-translational assembly of initiation factor complexes. Mol Cell. 2020;79(4):546–560.e547. doi: 10.1016/j.molcel.2020.06.004
- Yan X, Hoek TA, Vale RD, et al. Dynamics of translation of single mRNA molecules in vivo. Cell. 2016;165(4):976–989. doi: 10.1016/j.cell.2016.04.034
- Gozmanova M, Denti MA, Minkov IN, et al. Characterization of the RNA motif responsible for the specific interaction of potato spindle tuber viroid RNA (PSTVd) and the tomato protein Virp1. Nucleic Acids Res. 2003;31(19):5534–5543. doi: 10.1093/nar/gkg777
- Pamudurti NR, Bartok O, Jens M, et al. Translation of CircRNAs. Molecular Cell. 2017;66(1):9–21 e27. doi: 10.1016/j.molcel.2017.02.021
- Bazzini AA, Johnstone TG, Christiano R, et al. Identification of small ORFs in vertebrates using ribosome footprinting and evolutionary conservation. EMBO J. 2014;33(9):981–993. doi: 10.1002/embj.201488411
- Paik S, Tang G, Shak S, et al. Gene expression and benefit of chemotherapy in women with node-negative, estrogen receptor–positive breast cancer. J Clin Oncol. 2006;24(23):3726–3734. doi: 10.1200/jco.2005.04.7985
- Niemeier LA, Dabbs DJ, Beriwal S, et al. Androgen receptor in breast cancer: expression in estrogen receptor-positive tumors and in estrogen receptor-negative tumors with apocrine differentiation. Mod Pathol. 2010;23(2):205–212. doi: 10.1038/modpathol.2009.159
- Osborne CK, Wood AJJ. Tamoxifen in the treatment of breast cancer. N Engl J Med. 1998;339(22):1609–1618. doi: 10.1056/NEJM199811263392207
- Powles TJ, Ashley S, Tidy A, et al. Twenty-year follow-up of the Royal Marsden randomized, double-blinded tamoxifen breast cancer prevention trial. J Natl Cancer Inst. 2007;99(4):283–290. doi: 10.1093/jnci/djk050
- Liu B, Wang T, Wang H, et al. Oncoprotein HBXIP enhances HOXB13 acetylation and co-activates HOXB13 to confer tamoxifen resistance in breast cancer. J Hematol Oncol. 2018;11(1):26. doi: 10.1186/s13045-018-0577-5
- Shi Q, Li Y, Li S, et al. LncRNA DILA1 inhibits cyclin D1 degradation and contributes to tamoxifen resistance in breast cancer. Nat Commun. 2020;11(1):5513. doi: 10.1038/s41467-020-19349-w
- Wang J, Xie S, Yang J, et al. The long noncoding RNA H19 promotes tamoxifen resistance in breast cancer via autophagy. J Hematol Oncol. 2019;12(1):81. doi: 10.1186/s13045-019-0747-0
- Liu H, Song Y, Qiu H, et al. Downregulation of FOXO3a by DNMT1 promotes breast cancer stem cell properties and tumorigenesis. Cell Death Differ. 2020;27(3):966–983. doi: 10.1038/s41418-019-0389-3
- Otto T, Sicinski P. Cell cycle proteins as promising targets in cancer therapy. Nat Rev Cancer. 2017;17(2):93–115. doi: 10.1038/nrc.2016.138
- Burstein HJ. The distinctive nature of HER2-positive breast cancers. N Engl J Med. 2005;353(16):1652–1654. doi: 10.1056/NEJMp058197
- Piccart-Gebhart MJ, Procter M, Leyland-Jones B, et al. Trastuzumab after adjuvant chemotherapy in HER2-positive breast cancer. N Engl J Med. 2005;353(16):1659–1672. doi: 10.1056/NEJMoa052306
- von Minckwitz G, Procter M, de Azambuja E, et al. Adjuvant pertuzumab and trastuzumab in early HER2-positive breast cancer. N Engl J Med. 2017;377(2):122–131. doi: 10.1056/NEJMoa1703643
- Swain SM, Baselga J, Kim S-B, et al. Pertuzumab, trastuzumab, and docetaxel in HER2-positive metastatic breast cancer. N Engl J Med. 2015;372(8):724–734. doi: 10.1056/NEJMoa1413513
- Luo L, Zhang Z, Qiu N, et al. Disruption of FOXO3a-miRNA feedback inhibition of IGF2/IGF-1R/IRS1 signaling confers Herceptin resistance in HER2-positive breast cancer. Nat Commun. 2021;12(1):2699. doi: 10.1038/s41467-021-23052-9
- Zheng G, Guo Z, Li W, et al. Interaction between HLA-G and NK cell receptor KIR2DL4 orchestrates HER2-positive breast cancer resistance to trastuzumab. Signal Transduct Target Ther. 2021;6(1):236. doi: 10.1038/s41392-021-00629-w
- Deblois G, Smith HW, Tam IS, et al. ERRα mediates metabolic adaptations driving lapatinib resistance in breast cancer. Nat Commun. 2016;7(1):12156. doi: 10.1038/ncomms12156
- Zhou W, Wang Z, Shen N, et al. Knockdown of ANLN by lentivirus inhibits cell growth and migration in human breast cancer. Mol Cell Biochem. 2015;398(1–2):11–19. doi: 10.1007/s11010-014-2200-6
- Foulkes WD, Smith IE, Reis-Filho JS. Triple-negative breast cancer. N Engl J Med. 2010;363(20):1938–1948. doi: 10.1056/NEJMra1001389
- Dent R, Trudeau M, Pritchard KI, et al. Triple-negative breast cancer: clinical features and patterns of recurrence. Clin Cancer Res. 2007;13(15):4429–4434. doi: 10.1158/1078-0432.CCR-06-3045
- Asad S, Barcenas CH, Bleicher RJ, et al. Sociodemographic factors associated with rapid relapse in triple-negative breast cancer: a multi-institution study. J Natl Compr Canc Netw. 2021;19(7):797–804. doi: 10.6004/jnccn.2020.7659
- Wang X, Wang S-S, Huang H, et al. Effect of capecitabine maintenance therapy using lower dosage and higher frequency vs observation on disease-free survival among patients with early-stage triple-negative breast cancer who had received standard treatment: the SYSUCC-001 randomized clinical trial. JAMA. 2021;325(1):50–58. doi: 10.1001/jama.2020.23370
- Poggio F, Bruzzone M, Ceppi M, et al. Platinum-based neoadjuvant chemotherapy in triple-negative breast cancer: a systematic review and meta-analysis. Ann Oncol. 2018;29(7):1497–1508. doi: 10.1093/annonc/mdy127
- Yu KD, Ye F-G, He M, et al. Effect of adjuvant paclitaxel and carboplatin on survival in women with triple-negative breast cancer: a phase 3 randomized clinical trial. JAMA Oncol. 2020;6(9):1390–1396. doi: 10.1001/jamaoncol.2020.2965
- Li Y, Wang Z, Su P, et al. Circ-EIF6 encodes EIF6-224aa to promote TNBC progression via stabilizing MYH9 and activating the Wnt/beta-catenin pathway. Mol Ther. 2022;30(1):415–430. doi: 10.1016/j.ymthe.2021.08.026
- Khaled WT, Choon Lee S, Stingl J, et al. BCL11A is a triple-negative breast cancer gene with critical functions in stem and progenitor cells. Nat Commun. 2015;6(1):5987. doi: 10.1038/ncomms6987
- Cao Q, Song Z, Ruan H, et al. Targeting the KIF4A/AR axis to reverse endocrine therapy resistance in castration-resistant prostate cancer. Clin Cancer Res. 2020;26(6):1516–1528. doi: 10.1158/1078-0432.Ccr-19-0396
- Hu G, Yan Z, Zhang C, et al. FOXM1 promotes hepatocellular carcinoma progression by regulating KIF4A expression. J Exp Clin Cancer Res. 2019;38(1):188. doi: 10.1186/s13046-019-1202-3
- Huang SC, Wei P-C, Hwang‐Verslues WW, et al. TGF-β1 secreted by Tregs in lymph nodes promotes breast cancer malignancy via up-regulation of IL-17RB. EMBO Mol Med. 2017;9(12):1660–1680. doi: 10.15252/emmm.201606914
- Liu Q, Hodge J, Wang J, et al. Emodin reduces breast cancer lung metastasis by suppressing macrophage-induced breast cancer cell epithelial-mesenchymal transition and cancer stem cell formation. Theranostics. 2020;10(18):8365–8381. doi: 10.7150/thno.45395
- Xu YM, Wang H-J, Chen F, et al. HRD1 suppresses the growth and metastasis of breast cancer cells by promoting IGF-1R degradation. Oncotarget. 2015;6(40):42854–42867. doi: 10.18632/oncotarget.5733
- Comprehensive molecular portraits of human breast tumours. Nature. 2012; 490: 61–70. 7418. doi: 10.1038/nature11412
- Bai F, Zhang L-H, Liu X, et al. GATA3 functions downstream of BRCA1 to suppress EMT in breast cancer. Theranostics. 2021;11(17):8218–8233. doi: 10.7150/thno.59280
- Xu JZ, Shao C-C, Wang X-J, et al. circTada2as suppress breast cancer progression and metastasis via targeting miR-203a-3p/SOCS3 axis. Cell Death Dis. 2019;10(3):175. doi: 10.1038/s41419-019-1382-y
- Kim C, Gao R, Sei E, et al. Chemoresistance evolution in triple-negative breast cancer delineated by single-cell sequencing. Cell. 2018;173(4):879–893 e813. doi: 10.1016/j.cell.2018.03.041
- Liu Y, Cheng G, Huang Z, et al. Long noncoding RNA SNHG12 promotes tumour progression and sunitinib resistance by upregulating CDCA3 in renal cell carcinoma. Cell Death Dis. 2020;11(7):515. doi: 10.1038/s41419-020-2713-8
- Kishikawa T, Higuchi H, Wang L, et al. WWP1 inactivation enhances efficacy of PI3K inhibitors while suppressing their toxicities in breast cancer models. J Clin Invest. 2021;131(24). doi: 10.1172/jci140436
- Lee YR, Yehia L, Kishikawa T, et al. WWP1 gain-of-function inactivation of PTEN in cancer predisposition. N Engl J Med. 2020;382(22):2103–2116. doi: 10.1056/NEJMoa1914919
- Yin WB, Yan M-G, Fang X, et al. Circulating circular RNA hsa_circ_0001785 acts as a diagnostic biomarker for breast cancer detection. Clin Chim Acta. 2018;487:363–368. doi: 10.1016/j.cca.2017.10.011
- Hu Y, Song Q, Zhao J, et al. Identification of plasma hsa_circ_0008673 expression as a potential biomarker and tumor regulator of breast cancer. J Clin Lab Anal. 2020;34(9):e23393. doi: 10.1002/jcla.23393
- Li X, Ma F, Wu L, et al. Identification of Hsa_circ_0104824 as a potential biomarkers for breast cancer. Technol Cancer Res Treat. 2020;19:1533033820960745. doi: 10.1177/1533033820960745
- Liu J, Peng X, Liu Y, et al. The diagnostic value of Serum exosomal Has_circ_0000615 for breast cancer patients. Int J Gen Med. 2021;14:4545–4554. doi: 10.2147/ijgm.S319801
- Wang YW, Xu Y, Wang Y-Y, et al. Elevated circRnas circ_0000745, circ_0001531 and circ_0001640 in human whole blood: potential novel diagnostic biomarkers for breast cancer. Exp Mol Pathol. 2021;121:104661. doi: 10.1016/j.yexmp.2021.104661
- Ameli-Mojarad M, Ameli-Mojarad M, Nourbakhsh M, et al. Circular RNA hsa_circ_0005046 and hsa_circ_0001791 May become diagnostic biomarkers for breast cancer early detection. J Oncol. 2021;2021:2303946. doi: 10.1155/2021/2303946
- Li Y, Li H. Circular RNA VRK1 correlates with favourable prognosis, inhibits cell proliferation but promotes apoptosis in breast cancer. J Clin Lab Analysis. 2020;34(1):e22980. doi: 10.1002/jcla.22980
- Liu C, Chen M, Shi Y. Downregulation of hsa_circ_0006220 and its correlation with clinicopathological factors in human breast cancer. Gland Surg. 2021;10(2):816–825. doi: 10.21037/gs-21-42
- Liu C, Han T, Shi Y. The decreased expression of hsa_circ_0043278 and its relationship with clinicopathological features of breast cancer. Gland Surg. 2020;9(6):2044–2053. doi: 10.21037/gs-20-825
- Lü LS, Sun J, Shi P, et al. Identification of circular RNAs as a promising new class of diagnostic biomarkers for human breast cancer. Oncotarget. 2017;8(27):44096–44107. doi: 10.18632/oncotarget.17307
- Lü L, Sun J, Shi P, et al. Identification of circular RNAs as a promising new class of diagnostic biomarkers for human breast cancer. Oncotarget. 2017;8(27):44096–44107. doi: 10.18632/oncotarget.17307
- cancer.org|1.800.227.2345
- Wang S, Xue X, Wang R, et al. CircZNF609 promotes breast cancer cell growth, migration, and invasion by elevating p70S6K1 via sponging miR-145-5p. Cancer Manage Res. 2018;10:3881–3890. doi: 10.2147/cmar.S174778
- Xu JH, Wang Y, Xu D. Hsa_circ_001569 is an unfavorable prognostic factor and promotes cell proliferation and metastasis by modulating PI3K-AKT pathway in breast cancer. Cancer Biomark. 2019;25(2):193–201. doi: 10.3233/cbm-182293
- Zou Y, Zheng S, Xiao W, et al. circRAD18 sponges miR-208a/3164 to promote triple-negative breast cancer progression through regulating IGF1 and FGF2 expression. Carcinogenesis. 2019;40:1469–1479. doi: 10.1093/carcin/bgz071
- Zhang X, Su X, Guo Z, et al. Circular RNA La-related RNA-binding protein 4 correlates with reduced tumor stage, as well as better prognosis, and promotes chemosensitivity to doxorubicin in breast cancer. J Clin Lab Analysis. 2020;34(7):e23272. doi: 10.1002/jcla.23272
- Cai F, Fu W, Tang L, et al. Hsa_circ_0000515 is a novel circular RNA implicated in the development of breast cancer through its regulation of the microRNA-296-5p/CXCL10 axis. FEBS J. 2021;288(3):861–883. doi: 10.1111/febs.15373
- Zhou B, Mo Z, Lai G, et al. Targeting tumor exosomal circular RNA cSERPINE2 suppresses breast cancer progression by modulating MALT1-NF-?B-IL-6 axis of tumor-associated macrophages. J Exp Clin Cancer Res. 2023;42(1):48. doi: 10.1186/s13046-023-02620-5
- Rivera E, Gomez H. Chemotherapy resistance in metastatic breast cancer: the evolving role of ixabepilone. Breast Cancer Res. 2010;12(S2):S2. doi: 10.1186/bcr2573
- Liu G, Zhang Z, Song Q, et al. Circ_0006528 contributes to paclitaxel resistance of breast cancer cells by regulating miR-1299/CDK8 axis. Onco Targets Ther. 2020;13:9497–9511. doi: 10.2147/ott.S252886
- Yang W, Gong P, Yang Y, et al. Circ-ABCB10 contributes to paclitaxel resistance in breast cancer through let-7a-5p/DUSP7 axis. Cancer Manage Res. 2020;12:2327–2337. doi:10.2147/cmar.S238513
- Zang H, Li Y, Zhang X, et al. Circ-RNF111 contributes to paclitaxel resistance in breast cancer by elevating E2F3 expression via miR-140-5p. Thoracic Cancer. 2020;11(7):1891–1903. doi: 10.1111/1759-7714.13475
- Hao J, Du X, Lv F, et al. Knockdown of circ_0006528 suppresses cell proliferation, migration, invasion, and Adriamycin chemoresistance via regulating the miR-1236-3p/CHD4 axis in breast cancer. J Surg Res. 2021;260:104–115. doi: 10.1016/j.jss.2020.10.031
- Huang L, Ma J, Cui M. Circular RNA hsa_circ_0001598 promotes programmed death-ligand-1-mediated immune escape and trastuzumab resistance via sponging miR-1184 in breast cancer cells. Immunol Res. 2021;69(6):558–567. doi: 10.1007/s12026-021-09237-w
- Wang S, Wang Y, Li Q, et al. A novel circular RNA confers trastuzumab resistance in human epidermal growth factor receptor 2-positive breast cancer through regulating ferroptosis. Environ Toxicol. 2022;37(7):1597–1607. doi: 10.1002/tox.23509
- Vernieri C, Milano M, Brambilla M, et al. Resistance mechanisms to anti-HER2 therapies in HER2-positive breast cancer: Current knowledge, new research directions and therapeutic perspectives. Crit Rev Oncol Hematol. 2019;139:53–66. doi: 10.1016/j.critrevonc.2019.05.001
- Zhang H, Yan C, Wang Y. Exosome-mediated transfer of circHIPK3 promotes trastuzumab chemoresistance in breast cancer. J Drug Target. 2021;29(9):1004–1015. doi: 10.1080/1061186x.2021.1906882
- Li S, Teng S, Xu J, et al. Microarray is an efficient tool for circRNA profiling. Brief Bioinform. 2019;20(4):1420–1433. doi: 10.1093/bib/bby006
- Zhang W, Liu Y, Min Z, et al. circMine: a comprehensive database to integrate, analyze and visualize human disease–related circRNA transcriptome. Nucleic Acids Res. 2022;50(D1):D83–d92. doi: 10.1093/nar/gkab809
- Hussen BM, Mohamadtahr S, Abdullah SR, et al. Exosomal circular RNAs: New player in breast cancer progression and therapeutic targets. Front Genet. 2023;14:1126944. doi: 10.3389/fgene.2023.1126944