Abstract
Toxicological testing of compounds often is conducted at the maximum tolerated dose to identify potential target organs. Toxicities observed at these high doses may result in decreased body weight gain, food consumption and activity. These clinical signs are often associated with a generalized stress response. It has been known that stress may cause increased levels of corticosterone, which causes changes in circulating leukocyte profiles, decreases in thymus and spleen weights and changes in the microscopic structure of lymphoid organs. This makes it difficult to differentiate between stress-related changes and direct toxicity to the immune system in standard non-clinical toxicity testing in rats. In mice, MHC Class II expression was found to be a very sensitive biomarker of stress and maybe useful for the rat. Therefore, the objective of studies presented was to further characterize the effects of corticosterone and stressors on the immune system and identify potential biomarkers of stress in rats. Rats were treated with exogenous corticosterone (20 or 30 mg/kg BID) or ethanol (5 g/kg) for either 1 or 4 days. Restraint stress was also evaluated for a 3-day period. Blood and urine samples were collected during the treatment period for corticosterone measurements. At necropsy, blood samples for leukocyte differentials were collected. Spleen and thymus weights, cellularity, lymphocyte subpopulations and histopathology were also evaluated. Urine corticosterone levels were also investigated as a surrogate to measuring serum corticosterone. The results demonstrate that the pattern of responses to corticosterone or the stressors is different in mice and rats. Although, decreases in MHC Class II were found to be a sensitive indicator of stress in mice, only slight decreases were observed in rats with similar serum corticosterone AUC levels. Decreases in thymus weight were greater than spleen weight with corticosterone or ethanol or restraint stressor. No other single parameter or combination of parameters tested were obvious candidates as sensitive biomarkers of stress in rats. However, the good correlation between urine and serum corticosterone levels suggest that urine corticosterone may be a potential biomarker of stress induced changes to the immune response.
INTRODUCTION
Nonclinical toxicity testing for compounds being developed as drugs requires the use of high doses to maximize drug exposure to identify potential target organs. The resulting toxicity observed may result in stress responses that involve the release of corticosterone. In addition, there is evidence that drugs that produce novel sensations or alterations in perception in rodents can cause a stress response (De Boer et al., Citation1991).
Stress responses have been shown to result in alteration in circulating leukocyte levels and profiles as well as decreased lymphoid organ weights and changes to the microscopic anatomy of lymphoid organs. Changes in these parameters have been shown to be correlated to blood corticosterone area under the curve (AUC) levels in mice (Pruett et al., Citation1999, Citation2000a and Citationb, Citation2003; Pruett and Fan, Citation2000; Schwab et al., Citation2005). Thus, the stress associated with test compound administration may result in changes to the immune system. In these situations it may be difficult to differentiate between stress-related changes and direct toxicity to the immune system. This issue is discussed in the immunotoxicity testing guidelines (S8) from the International Committee on Harmonization (ICH). The guidelines recommend that if stress is thought to be the cause for immune system changes in toxicity studies, evidence supporting this conclusion should be provided. Administration of exogenous corticosterone to produce blood levels comparable to those induced by chemicals in rats was used to quantitatively establish the amount of change in several immunological parameters induced by the corticosterone component of stress responses.
We previously used other methods (a corticosterone antagonist) to confirm that effects of drug and chemical stressors in mice were mediated mostly by corticosterone (Han et al., Citation1993; Han and Pruett, Citation1995; Weiss et al., Citation1996). All the parameters measured in this study were parameters that were mostly changed by corticosterone after treatment with the indicated chemicals. It remains possible that these chemicals may act directly (not through the corticosterone) in rats, but this can be easily recognized if the effect of exogenous corticosterone is less than the effect of the chemical at a dosage that produces comparable area under the corticosterone concentration vs. time curve.
Biomarkers of stress would be very helpful to resolve this issue. In mice, the stress response and resulting changes in the immune system have been well characterized. Major histocompatibility complex (MHC) II expression on B-cells in mice was found to be a sensitive marker of stress. However, since most toxicity studies are conducted in rats, it is not known what types of changes in the rat are caused by high levels of corticosterone. Thus, the objectives of these studies were to characterize the immune system changes with corticosterone treatment and identify potential biomarkers of stress using a chemical stressor (ethanol) and a physical stressor (restraint).
MATERIALS AND METHODS
Animals
Female specific pathogen-free Sprague–Dawley rats were obtained from Charles River (Kingston, NY). Female rats were used because previous studies with mice to which we wanted to compare the results in rats utilized female mice. The rats were allowed to acclimate to our facility in metabolic cages for at least 2 weeks after arrival and were maintained on a 12-hr/12-hr light/dark cycle (0700–1900 light) with food (Purina Lab Chow 5002) and water available ad libitum. Rats were 8- to 12 weeks-of-age during experimental procedures. Sentinel rats housed in the same room were negative for antibodies to a panel of adventitious agents common to rats. Animal care and use were in accordance with the guidelines of the Institutional Care and Use Committee of the National Institute on Drug Abuse, National Institutes of Health, and the Guide for the Care and Use of Laboratory Animals (Institute of Laboratory Animal Resources, Commission on Life Sciences, National Research Council, 1996), as well as the regulations of Louisiana State University Health Sciences Center. The facility is accredited by the Association for Assessment and Accreditation of Laboratory Animal Care. A group size of six was used in these studies, which is similar to the group size of five used in our previous, similar studies in mice (Pruett et al., Citation1999).
To decrease stress-induced background corticosterone levels, animals were acclimated to handling by removing them from their cage, weighing and simulating injections and bleeding procedures once each day for several days prior to the start of the experiment. Exogenous corticosterone (20 mg/kg) was administered subcutaneously at 9 AM and again at 1 PM for each of four days. This was prepared as a suspension in β -cyclodextrin (2%, Sigma, St. Louis, MO) and methylcellulose (1%, Sigma) in phosphate buffered saline (Sigma) to delay the release of the corticosterone. The suspension was prepared fresh daily and emulsified immediately prior to injection. This delayed release dosing regimen was selected to yield an area under the serum corticosterone vs. time curve comparable to that of an exogenous stressor.
It was administered subcutaneously either as one dose of 20 mg/kg (Experiment 1), one dose of 30 mg/kg (Experiment 3), or two daily doses of 20 mg/kg, given at 9 AM and 1 PM, for 4 days (Experiment 5). Ethanol was administered at 5 g/kg by oral gavage as a 32% solution in tissue culture-grade water, as single dose (Experiment 2) or with sub-chronic daily dosing for 4 days (Experiment 4). Ethanol was administered at the same time of day as the first dose of corticosterone (9 AM). Restraint stress was induced by holding the animals for 8 hr per day for 3 days in clear acrylic devices designed for this purpose (Experiment 6). In all experiments there was a naive (untreated) control group and/or a vehicle control group in which the vehicle for the compound administered in that experiments was given in the same amount and by the same route as the compounds were given. Both ethanol and restraint have been demonstrated to cause robust stress responses in rats (Jerrells et al., Citation1990; Dhabhar et al., Citation1997).
Sample Collection
For serum corticosterone determination, rats were bled within 2 minutes of retrieval from their cages, serially over a 12-hr or 24-hr period on the final day of dosing, beginning immediately or 1 hr after the last dose (as indicated in ). This was done by quickly wrapping the rat in a towel and making a nick with a razor blade in a lateral tail vein to collect ≈ 100 μ l blood. This method was adapted from a procedure by Dhabhar et al. (Citation2000). Urine samples were collected by housing the rats individually in metabolic cages. The urine collection tubes with mesh screen filters were kept in ice during the period of collection. A 6-hr urine sample was taken, followed by an 18-hr sample. A 0.5 ml sample of urine was analyzed for creatinine by the LSU Hospital Clinical Lab within 24 hr, and the remainder of each sample was then stored frozen until analyzed for corticosterone. All urine samples were centrifuged and the supernatant decanted to remove cells and debris before analysis or storage. When dosing, bleeding was immediately followed by injection. Soon after the last sample collection (9 am on the fifth day), rats were injected with a lethal overdose (100 mg/kg) of sodium pentobarbitol, intraperitoneally. After the animal was unconscious, exsanguination by cardiac puncture was performed and blood was collected into lithium heparinized tubes for white blood cell counts and flow cytometry analysis.
FIG. 1 Serum corticosterone concentrations over time in rats exposed to stressors. Rats were stressed beginning at time 0, and blood samples were collected from tail at the indicated times after initiation of stressor. For experiments 5 and 6, stress was administered daily for 3 or 4 days, and corticosterone values were determined on the last day. Values shown represent means ± SEM and n = 5–6 per group. The numbers shown below each legend are the area under the corticosterone concentration vs. time curve (AUC), and these values provide a quantitative measure of excessive corticosterone exposure. Of course, the values in Experiments 5 and 6 do not reflect corticosterone produced on previous days of treatment. The AUC value in Experiment 6 was slightly less than 0, indicating that the rats had habituated to restraint stress by day 3, and no longer responded to this stressor.
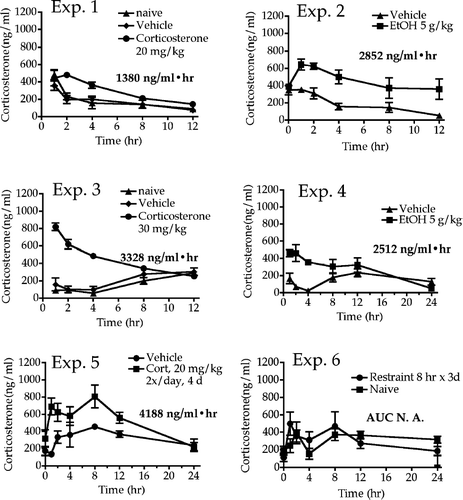
Blood smears were also prepared for manual differential counts. Following death, animals were weighed, and their spleen, thymus, and the ileocecal and inguinofemoral lymph nodes were removed. The spleen and thymus were weighed, then cut in half. The lymph nodes and half the spleen and thymus were immersion-fixed in buffered formalin and processed for histology within 3–4 days. The remaining portions of the spleen and thymus were processed to produce single cell suspensions for cell counts and flow cytometry, as described in a previous study (Pruett et al., Citation2000a). All cell counts were performed using a Coulter counter (Model Z1).
Corticosterone Analysis
Serum corticosterone was analyzed using a rat corticosterone Coat-a-Count Kit (DPC Products, Los Angeles, CA). Prism software (Graphpad, San Diego, CA) was used to calculate the area under the corticosterone vs. time curve. This method was validated against our previously published method using NIH Image (data not shown) (Pruett et al., Citation1999). Urine corticosterone concentrations were measured using a radioimmunoassay kit from ICN, and the corticosterone/creatinine ratio was calculated to normalize the results for the differences in urine volume collected.
Flow Cytometry Analysis and Cell Counts of Leukocyte Populations
Analysis of splenocytes and thymocytes by flow cytometry was performed as previously reported in our mouse studies with the exception of antibody selection (Hebert and Pruett, Citation2001). The subpopulation or surface marker and antibody reagents used are: B-cells, anti-CD45RA-phycoerythrin (PE, OX-33); MHC-II, anti-RT1B-flouroisothiocyanate (FITC, OX-6); T-cells, anti-CD4-FITC (OX-35) and anti-CD8a-PE (OX-8); and NK cells, anti-CD161a-PE (NKR-P1A). All antibodies were purchased from BD Pharmingen (San Diego, CA). Gating was based upon comparison with appropriate isotype control antibodies and typically contained fewer than 2% positive cells. Splenocytes, thymocytes (106 cells each in FACS buffer in V-bottom 96-well plates) and whole blood (150 μ l) were incubated 30 min in the dark at 4°C with various antibodies. Erythrocytes in blood were lysed by adding 4 ml RBC lysis buffer for 8 min at 37°C, gently vortexing at 4 min. Cells were washed and resuspended in FACS buffer and analyzed on a Becton-Dickinson Facscan flow cytometer using CellQuest software (Becton-Dickinson Immunocytometry Systems, Mansfield, MA).
Values are reported as percentage of gated cells, and the gate was set as the minimal population of cells in forward scatter/side scatter plots that contained virtually all the CD4+ cells in the population. Absolute white blood cell (WBC) counts were determined using an electronic particle counter (Coulter Z1). The absolute counts of various subsets of cells were determined by multiplying the percentage of that particular cell type (expressed as a fraction) by the total number of nucleated cells. The percentage of neutrophils and lymphocytes in blood was determined using manual differential counts on blood smears. In the case of various lymphocyte types in the blood, absolute numbers were calculated by multiplying WBC/ml by the percentage of lymphocytes (determined by the differential count and expressed as a fraction) by the percentage of cells (expressed as a fraction) of the particular type of interest (e.g., B-cells) in the lymphocyte gate (as determined by flow cytometry).
Histology and Immunohistochemistry
Formalin-fixed, paraffin-embedded sections of thymus, spleen and lymph nodes were stained with hematoxylin and eosin and examined microscopically by the same veterinary pathologist. Generally all six animals per group were examined, except for Experiments 4 and 6 where only three per group were available for histology. Selected sections were also stained immunohistochemically using antibodies against CD3 for T-cells (rabbit polyclonal; Dako, Carpinteria, CA), CD79a for B-cells (mouse monoclonal Hm57; Dako), ED-1 for macrophages (mouse monoclonal RED-1; Serotec, Raleigh, NC), and cleaved caspase-3 for apoptotic cells (rabbit polyclonal; Cell Signaling Technology, Beverly, MA).
Statistical Analysis
Treatment groups included six rats each. Significant differences (p < 0.05) between treatments were determined by using Student's t-test (for experiments with two groups) or ANOVA followed by the SNK post-hoc test (for experiments with more than two groups). Data are reported as mean and standard error of the mean (SEM). Analyses were done using the Prism software package (GraphPad, San Diego, CA).
RESULTS
Corticosterone AUC Values in Rats Exposed to Stressors
Total corticosterone exposure, expressed as area under the serum corticosterone concentration vs. time curve was elevated by all the treatments except restraint in experiment 6 (). The AUC value expressed in each graph was calculated by subtracting the AUC for control rats from the AUC for the treated rats; as such, the value for the controls is reflected in each number shown. A single incident of restraint did produce a significant increase in the corticosterone AUC value (data not shown), but it seems likely that the two daily restraint sessions prior to the third day (on which blood samples were taken) caused the rats to become habituated to this stressor. In any case, some treatments yielded comparable AUC values as obtained with exogenous corticosterone. This permits inferences with regard to the portion of the effects induced by the stressor that are mediated by corticosterone.
Effects of Stressors on Blood Parameters
Changes in the absolute concentration () and the percentages () of several cell types were determined in most of the experiments in this study. There was a significant increase in the total concentration of white blood cells (WBC) in experiment 4 (ethanol at 5 g/kg, 24 hr after dosing), but no significant change in this parameter in other experiments. Corticosterone (Experiments 1 and 3) and ethanol (Experiment 4) significantly increased the percentage and/or number of circulating neutrophils ( and ). The percentage of neutrophils in most experiments tended to be increased by stressors, but variation within groups prevented some differences from being significant. This was the most consistent change noted in blood parameters in the present study. A similar trend was noted for absolute neutrophil concentrations, but the increase was significant only for Experiments 2 and 4. There was a corresponding tendency for the percentage and number of lymphocytes to decrease, but the changes were not as large and none were statistically significant. The subpopulations of lymphocytes were differentially affected only in Experiment 2, in which there was a decrease in the percentage but not the absolute concentration of CD8+ T-cells, and Experiment 4, in which the percentage of CD4+ T-cells decreased and the percentage and absolute concentration of CD8+ T-cells increased.
FIG. 2 Changes in the concentration of various blood cell types at 12 hr (for Experiments 1 and 2) or 24 hr (Experiments 3–6) after initiation of stressor. Values shown are means ± SEM for groups of 5–6 rats, and values in each experiment significantly different from the control value are indicated by * p < 0.05 or ** p < 0.01. In some experiments some parameters were not measured (indicated by N.D., not determined).
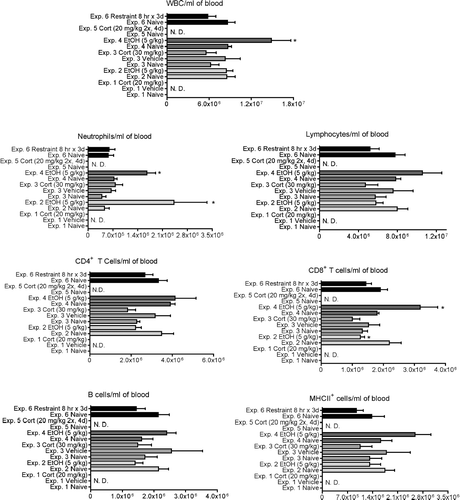
FIG. 3 Changes in the percentage of various blood cell types at 12 hr (for Experiments 1 and 2) or 24 hr (Experiments 3–6) after initiation of stressor. Values shown are means ± SEM for groups of 5-6 rats, and values in each experiment significantly different from the control value are indicated by * p < 0.05 or * p < 0.01.
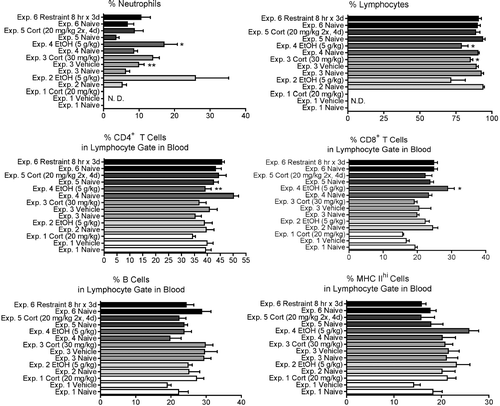
Effects of Stressors on Spleen Parameters
Exogenous corticosterone (Experiments 1, 3, and 5) significantly decreased the percentage of splenocytes expressing the highest concentration of MHC Class II protein. However, none of the other treatments affected this parameter. None of the treatments significantly affected the percentages of TH, TC, or B-cells in the spleen. Total cell numbers were not determined in these experiments, because part of the spleen was used for histological analysis. Only exogenous corticosterone at 20 mg/kg given twice a day for 4 days (Experiment 5) significantly decreased spleen weight as a percentage of body weight (80% of controls) (). A decrease in spleen weight as a percentage of body weight (86% of controls) approaching statistical significance was also noted in Experiment 2. In Experiment 2, the spleens of 3 out of 5 treated rats were histologically similar to controls, while the other two showed slight to mild changes, consisting of cellular depletion and decreased size of the B-cell areas (marginal zones and follicular areas of the periarteriolar lymphoid sheath), confirmed by immunohistochemical staining for CD79a (, , , and ). No difference in histology was noted between control and treated spleens in other experiments.
FIG. 4 Changes in the spleen 12 hr (for Experiments 1 and 2) or 24 hr (for Experiments 3–6) after initiation of stressor. Values shown are means ± SEM for groups of 5–6 rats, and values in each experiment significantly different from the control value are indicated by * p < 0.05, or ** p < 0.01.
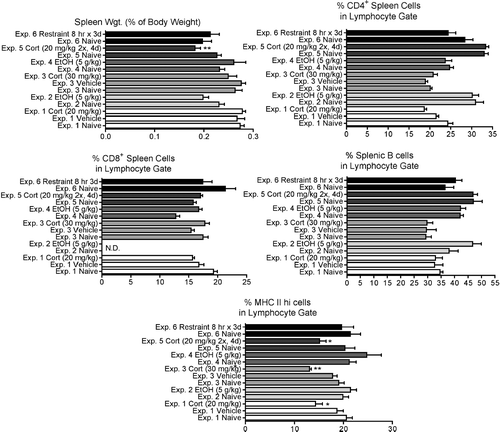
FIG. 5 Histological changes in the spleen and thymus in response to stressors. A, B: Spleen from a control rat from Experiment 2 (H&E and immunohistochemistry [IHC] for CD79a, 100X). C, D: Spleen from a treated Experiment 2 rat with decreased size and cellularity of the B-cell areas, including marginal zone (mz) (H&E and IHC for CD79a). E: Thymus from a treated Experiment 2 rat, showing increased numbers of macrophages containing apoptotic cell debris (H&E, 200X). F: Thymus from a control Experiment 2 rat, showing the normal density of apoptotic cells (IHC for cleaved caspase-3, 200X). G: Thymus from the same rat as in E, showing markedly increased positivity for cleaved caspase-3 (IHC for cleaved caspase-3, 200X). H: Thymus from a control Experiment 5 rat, showing the normal size and cellularity of cortex and medulla (H&E, 100X). I: Thymus from a treated Experiment 5 rat, showing marked thinning and depletion of lymphocytes in the cortical areas (H&E, 100X).
![FIG. 5 Histological changes in the spleen and thymus in response to stressors. A, B: Spleen from a control rat from Experiment 2 (H&E and immunohistochemistry [IHC] for CD79a, 100X). C, D: Spleen from a treated Experiment 2 rat with decreased size and cellularity of the B-cell areas, including marginal zone (mz) (H&E and IHC for CD79a). E: Thymus from a treated Experiment 2 rat, showing increased numbers of macrophages containing apoptotic cell debris (H&E, 200X). F: Thymus from a control Experiment 2 rat, showing the normal density of apoptotic cells (IHC for cleaved caspase-3, 200X). G: Thymus from the same rat as in E, showing markedly increased positivity for cleaved caspase-3 (IHC for cleaved caspase-3, 200X). H: Thymus from a control Experiment 5 rat, showing the normal size and cellularity of cortex and medulla (H&E, 100X). I: Thymus from a treated Experiment 5 rat, showing marked thinning and depletion of lymphocytes in the cortical areas (H&E, 100X).](/cms/asset/72a26ef5-2c78-4647-968e-0cc684903f69/iimt_a_211450_uf0005_b.gif)
Effects of Stressors on Thymus Parameters
In Experiment 2, three rats (including the two animals with splenic changes) had histologic changes in the thymus which consisted in mild to marked increases in the number of cortical apoptotic cells and of macrophages containing cell debris (, , and ). This was not associated with group differences in thymus weight or cellular subpopulations by flow cytometry. The only experiments in which significant changes in these parameters were noted were those involving daily administration of stressor for three or more days (Experiments 5 and 6, ). In these experiments, there was a significant decrease in thymic weights, the percentage of CD4+CD8+ thymocytes was significantly decreased and the percentage of all other subpopulations was increased. This is consistent with the known sensitivity of CD4+CD8+ thymocytes to stress-inducible corticosterone concentrations. In Experiment 5, this was associated histologically with loss of cellularity and thinning of the cortical areas in all animals, generally with a slight increase in the numbers of apoptotic cells and debris ( and ).
FIG. 6 Changes in the thymus 12 hr (for Experiments 1 and 2) or 24 hr (for Experiments 3–6) after initiation of stressor. Values shown are means ± SEM for groups of 5–6 rats, and values in each experiment significantly different from the control value are indicated by * p < 0.05, ** p < 0.01, or *** p < 0.001.
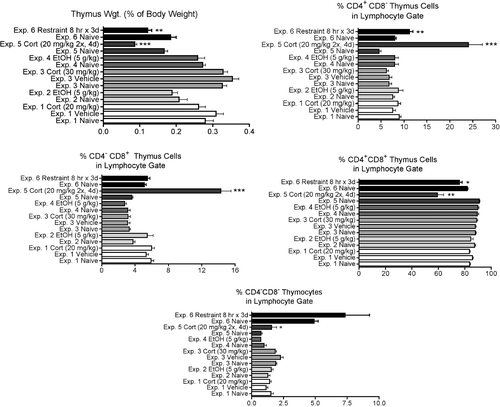
Quantitative Analysis of the Effects of Stressors on Selected Immune Parameters in Rats
In mice, changes in most immunological parameters could be accurately predicted on the basis of corticosterone AUC values, regardless of the stressor used to induce the corticosterone response. However, results from Experiments 3, 4, and 5 demonstrate that there was not a simple linear relationship between corticosterone AUC and immune parameters, except in the case of MHC Class II (). These experiments were selected for comparison because immune parameters were evaluated at the same time after initiation of stressor in all of them (24 hr). This was also true for Experiment 6. However, Experiment 6 was not suitable for inclusion because the corticosterone AUC value during the last stress session was not measurable, presumably due to habituation to the restraint procedure. Even though the effects of stressors on MHC Class II expression were roughly linear for Experiments 3, 4, and 5, only slight decreases in the percent positive MHC Class II positive splenocytes were observed at high corticosterone AUC.
FIG. 7 Relationships between area under the corticosterone concentration vs. time curve (AUC) and selected immunological endpoints. The results shown are means ± SEM for groups of 5–6 rats and represent results from Experiments 3, 4, and 5. The first point in each graph represents the normalized value of the control group (all control groups were arbitrarily defined as 100%). These are the experiments in which the endpoints were evaluated 24 hr after stressor initiation, but Experiment 6 was not included. In that experiment, the daily restrains sessions apparently produced habituation, leading to lack of a corticosterone response after the last restraint session. Also note that the results for Experiment 5 (AUC = 4188) may represent some degree of cumulative effects, because the rats in this experiment were treated daily for 4 days with exogenous corticosterone. The lines shown are the best fit to the data as indicated by analysis with Prism software.
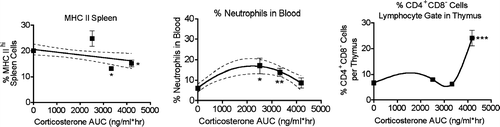
Thus, in comparison to the mouse, MHC Class II may not be an indicator of stress in rats. Experiment 2 (EtOH) and Experiment 3 yielded similar corticosterone AUC values, but MHC II was slightly increased in Experiment 2 and significantly decreased in Experiment 3. This may suggest that MHC Class II is affected by exogenous corticosterone alone but not when it is present with other stress mediators induced by EtOH (and presumably by other drugs and chemicals). The relationship between AUC and neutrophil percentage or % CD4+ cells may be inferred by comparing experiments in which a single administration of stressor occurred to experiments in which stressor was administered daily for 3 or 4 days (Experiments 5 and 6). However, it would be expected that this would yield greater than expected effects due to cumulative changes in cells over time. This was observed in Experiment 5 (corticosterone 20 mg/kg, 2 doses per day for 4 days) with regard to the changes in CD4+ T-cell percentage in the thymus. In contrast, the percentage of neutrophils in the blood was not significantly affected in Experiment 5, even though it was affected in experiments in which the corticosterone AUC value was lower. This suggests the possibility that multiple daily exposures to stressors induce tolerance with regard effects on neutrophils.
Direct Comparison of the Effects of Ethanol at 5 g/kg in Mice and Rats
Rats treated with EtOH at 5 g/kg and mice treated with EtOH at 6 g/kg exhibit similar corticosterone AUC responses (Pruett et al., Citation2003). Nevertheless, two parameters (most notably MHC Class II expression) were affected in mice but not in rats by these treatments. The results shown in illustrate this comparison. There was a significant increase in the percentage of neutrophils in the blood at 12 hr in both species. There was also a significant increase in neutrophils in the blood in two of four experiments in which parameters were evaluated 24 hr after initiation of the stressor. Also shown in is a comparison of the effects of corticosterone and ethanol on the number of CD4+CD8+ cells in the thymus 24 hr after treatment. These results are of interest because this population of cells is more sensitive than other thymic lymphocyte populations to stress and corticosterone (Pruett and Padgett, Citation2004), and thymic lymphocyte depletion is often regarded as a hallmark of stress-induced immunosuppression and as one of the most sensitive indicators of this condition. However, the results shown here indicate that significant elevation of corticosterone for as long as 6 hr (see , for example) is not sufficient to cause the selective loss of the most sensitive cell type (CD4+CD8+ cells) that is generally associated with exposure to stressors (Fuchs and Pruett, Citation1993). Repeated administration of corticosterone (Experiment 4) produced the greatest effects, probably because depletion was cumulative to some extent. The results do not suggest substantial differences between rats and mice with regard to sensitivity of thymocytes to corticosterone.
FIG. 8 Comparison of stress effects in mice and rats on the basis of corticosterone AUC values. Results for rats are from the present study, and results for mice are from previous studies. Some of these results have been published previously (Schwab et al., Citation2005), but the values are not the same as shown here. For the present study, the raw flow cytometry data from mice were reanalyzed using a gating scheme as similar as possible to the one used here for rats. In the published study (Schwab et al., Citation2005), significantly different gating was used, and this produced different values. The times indicated are the times after initiation of stressor at which the parameter was analyzed. Results shown are means ± SEM and are normalized by defining the control value of each experiment as 100%. Values that differed significantly from the control within each experiment are indicated by * p < 0.05, ** p < 0.01, or *** p < 0.001.
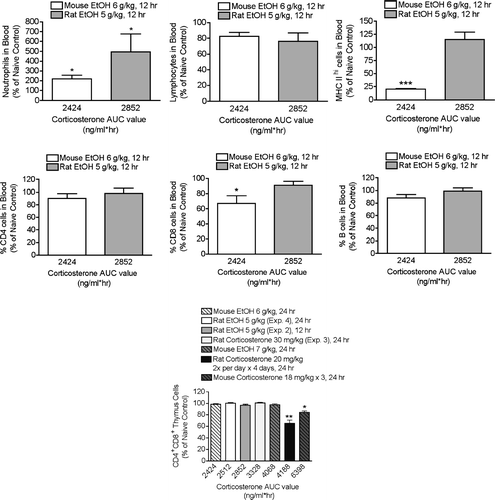
Correlation between Serum Corticosterone AUC Values and Urine Corticosterone Concentration
To determine if urinary corticosterone values correspond reasonably well to serum corticosterone AUC values, urinary corticosterone values were compared to corticosterone AUC values in the serum. The results shown in demonstrate good correlation between the serum corticosterone AUC results over the first 8 hr and urine corticosterone over the first 24 hr after exogenous corticosterone administration (R2 = 0.74). A less robust, but still highly significant association was also noted when corticosterone in a 6 hr urine sample was compared to the corticosterone AUC value in serum (8 hr).
FIG. 9 Relationships between area under the corticosterone concentration vs. time curve (AUC) for serum and urine corticosterone concentration. The results shown are means for groups of 5–6 rats treated with exogenous corticosterone or untreated. The linear relationship, 95% confidence intervals, and R2 values are shown.
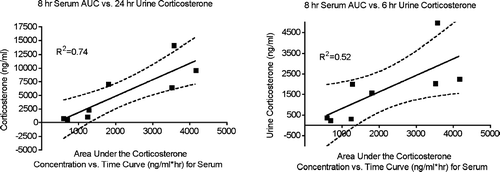
DISCUSSION
Determining the role of chemical-induced stress in immunotoxicity is relevant with regard to mechanisms of immunotoxicity and with regard to mechanisms by which other stressors (particularly psychological stress) adversely affect the immune system. In addition, ICH S8 Immunotoxicology Testing Guidelines indicate that signs of immunotoxicity in initial non-clinical trials (e.g., changes in weight or histology of lymphoid organs) may trigger the need for additional immunotoxicology studies. Since a variety of drugs and chemicals induce neuroendocrine stress responses (especially drugs that affect the CNS) and these responses can cause changes to the immune system (Pruett et al., Citation1999, Citation2000b, Citation2003; Pruett and Fan Citation2000; Pruett et al., Citation2003; Myers et al., Citation2005), it may be difficult to differentiate between stress-induced changes to the immune system from direct immuntoxicity. However, when significant levels of stress are produced and several endpoints are affected, the changes to the immune system may be more readily attributed to stress rather than a direct effect.
For example, if decreases in thymus and spleen weight are only observed at doses only associated with decreased body weight gain, clinical signs of decreased or increased activity and unkempt appearance, the effects on the thymus and spleen are likely attributed to stress. In other situations with more subtle changes, it may be difficult to conclude that changes to the immune system were caused by stress. In the mouse, MHC Class II expression on B-cells was found to be a sensitive biomarker of stress. Moreover, quantitative analyses of serum corticosterone AUC levels and changes to various parameters demonstrated linear relationships (Pruett et al., Citation1999, Citation2000b, Citation2003; Pruett and Fan, Citation2000; Myers et al., Citation2005).
Therefore, studies were carried out to determine if biomarkers of stress could be identified in rats, the species primarily used for toxicity studies. This goal was first addressed by evaluating the effects of corticosterone on immune parameters in the rat to identify the most sensitive parameter and evaluate stress produced by acute ethanol exposure and restraint stress. Additionally, serum corticosterone AUC was determined in rats treated with corticosterone or other stressors to determine if there is a quantitative relationship between these parameters as observed with the mouse. Urine corticosterone levels were also evaluated as a potential, non-stressful method of measuring corticosteroid levels.
The results observed in these studies demonstrated that there are important differences between the effect of corticosterone and stressors on various immune parameters in rats and mice. This is not primarily because rats respond less vigorously to stressors. The corticosterone AUC values obtained in these experiments in rats can be compared to those obtained in previous studies with mice, using similar treatments. For example, a single dose of ethanol at 5 g/kg (as in Experiment 2, ), produced an AUC value of 1271 ng/ml*hr in mice (Pruett et al., Citation2003). This is considerably less than the value obtained in rats, but it should be noted lower dosages of ethanol are generally required in rats than in mice to produce similar biological effects (Hillbom et al., Citation1983; Finn et al., Citation1989). The AUC value for mice given ethanol at 6 g/kg (2424 ng/ml*hr) (Schwab et al., Citation2005) is similar to the value obtained in rats at 5 g/kg (2512–2852 ng/ml*hr). Similarly, a higher dosage of exogenous corticosterone (30 mg/kg, Experiment 3, ) is required in rats to produce a similar AUC value as reported previously in mice treated with exogenous corticosterone at 18 mg/kg (3010 ng/ml*hr) (Pruett et al., Citation1999). Thus, immune parameters in rats seem less sensitive to the effects of corticosterone than in mice. Although this would suggest that rats are not the ideal species to discern stress-mediated effects, the expense associated with changing the species used pre-clinical testing would likely be prohibitive. Therefore, even though it will be more challenging than for mice, it remains important to continue seeking methods to distinguish stress-mediated changes in the rat immune system.
Our previous studies in mice indicated a tendency for WBC counts to be decreased at 12 hr after initiation of stressor (Schwab et al., Citation2005). Thus, the differences between those results and the lack of a decrease reported here at 24 hr may reflect differences in the dynamics of changes in WBC trafficking after stress rather than species differences. However, comparable increases in the percentage of neutrophils and decreases in the percentages of lymphocytes were observed in mice as in the present study with rats, and these changes seemed to be the most sensitive and consistent immunological parameter affected by stress or corticosterone in the present study.
Most spleen and thymus parameters were not affected by the treatments in this study, unless they were administered more than once (as in Experiments 5 and 6). Interestingly, histological analysis revealed changes in the thymus and spleen in Experiment 2 that were not indicated by changes in weight or percentages of cell types, while Experiments 1, 3 and 4 in which corticosterone levels were also increased did not show visible histologic changes. This may have been due to individual variations in the histologic expression of stress changes; indeed the thymus and spleen changes in Experiment 2 were only seen in a subset of treated animals. This suggests that the sensitivity of histologic changes to stress or corticosterone between animals may be quite significant, especially in acute stress.
Changes in Experiment 5 were detected both by histology and by other methods. The apparently greater sensitivity of detection in experiment 5 is probably related to the greater corticosterone exposure than in Experiment 2, but the time at which the thymus was evaluated (12 hr for Experiment 2 and 24 hr for Experiment 5) may also have been a factor. The thymus was significantly affected when either exogenous corticosterone or restraint were administered daily for 3 or 4 days (Experiments 5 and 6). The spleen to body weight ratio was significantly decreased in one experiment (Experiment 5) involving administration of corticosterone for 4 days, and a small but significant decrease in cells expressing the high levels of MHC Class II protein was noted in each experiment in which exogenous corticosterone was given. Interestingly, ethanol (5 g/kg) was given daily for 2 days in two separate experiments, and the effects were not meaningfully different from those observed after a single dose (data not shown). As in the case of restraint, this may indicate rapid induction of tolerance or habituation to the stress-inducing properties of some chemicals, which we have also observed in mice (manuscript in preparation). Although MHC Class II was affected by exogenous corticosterone, it was not affected by ethanol or restraint, in contrast to the situation in mice, in which all stressors decreased its expression, even at corticosterone AUC values similar to or lower than noted here in rats () (Pruett et al., Citation1999, Citation2003; Schwab et al., Citation2005). In contrast, rats and mice seemed similarly sensitive to corticosterone and ethanol-induced thymic atrophy (). Thus, MHC Class II expression is not the sensitive, reliable indicator of stress in rats that it seems to be in mice. However, the AUC values for ethanol in rats in this study were less than the lowest value for exogenous corticosterone that caused suppression of MHC Class II expression in mice. Thus, it remains possible that chemical or drug-induced stressors that produce slightly higher corticosterone AUC values will suppress MHC Class II. However, until such evidence is available, MHC Class II cannot be considered an ideal biomarker for stress in rats.
The effects on the thymus in rats were similar to those reported previously in mice, in which a single dose of ethanol or corticosterone in the dosage range used in the present study caused minimal loss of cells or changes in subpopulation percentages in the thymus 24 hr after dosing (Pruett et al., Citation2000a, Citation2003). Clearly, there is evidence that more profound stress (including higher dosages of ethanol) (Han and Pruett, Citation1995) can cause thymic atrophy, but such effects required repeated exposure to the milder stressors used in the present study.
Among the blood parameters evaluated here, the percentage and absolute concentration of neutrophils in the blood seem to be the most sensitive and consistent indicators of stress in rats. An increased percentage of neutrophils in the blood is a characteristic response to many stressors in mammals (Engler et al., Citation2004; Reid et al., Citation2004). However, it is usually reported to be a relatively transient phenomenon. The observation of changes that persist in some cases for 24 hr was somewhat unexpected. It may be that neutrophil percentages or numbers will be a useful biomarker for stress. However, the results shown in raise the possibility that administration of stressors daily for several days may lead to tolerance or habituation with regard to this parameter. Most repeat dose toxicity studies are usually 2 or 4 weeks in length and stress often is often observed only during the first week (tolerance to the stress may occur post Drug Week 1). Thus, if the first set of samples for hematology is collected during the second week (a common study design), the utility of neutrophils as a biomarker for stress would be limited. Twenty-eight-day exposure of mice to ethanol and propanil did lead to tolerance or habituation with regard to increases in neutrophils (manuscript in preparation). Therefore, more studies are needed to determine if this is a common occurrence in rats that would preclude the routine use of neutrophil number or percentage as a biomarker for stress.
The results shown in suggest that urinary corticosterone can be used to indicate the degree of corticosterone exposure experienced by rats in pre-clinical studies. Determining the correlation between urinary corticosterone concentrations and standard immunological parameters that are already measured in pre-clinical testing should provide a method for estimating whether the magnitude of the stress response is sufficient to explain the immunological changes. In previous studies in mice, it has been noted that the quantity of change in several immunological parameters can be predicted by determining the corticosterone AUC value associated with administration of chemical stressors (Pruett et al., Citation1999, Citation2003; Schwab et al. Citation2005). It is expected that this will also be the case for rats and that urine corticosterone concentrations can be used as a surrogate for serum corticosterone AUC values, because 6- or 24-hr urine samples reflect the amount of excess corticosterone present during the period of the stress response. However, it would be useful if additional biomarkers could be identified that are consistently associated with exposure to stressors.
Unfortunately, the most sensitive parameter in mice, MHC Class II expression, was much less sensitive to stressors in rats than in mice. Future studies will utilize agents or dosages that induce a larger stress response, and additional parameters will be measured. Additionally, it would be interesting to focus on dosing daily for an extended period of time, as multiple doses of corticosterone were more effective than a single dose in the present study. In these screening studies, high throughput methods such as microarry analysis may be particularly useful.
Although the results presented here are not yet sufficient to provide biomarkers that can establish that a particular immunological effect is mediated by a stress response, they could provide reasonably definitive evidence for specific (non-stress mediated) immunotoxicity. If a drug affected any of the immunological parameters measured here to a much greater extent than observed in this study, even though it induced a similar or lower corticosterone value in the urine, this would strongly indicate that the drug was an immunotoxicant.
The results presented here were unexpected to some degree in that some parameters (e.g., neutrophil percentage in blood and thymus cell number and weight) were similar in sensitivity to corticosterone exposure in rats as compared to mice. Comparison of sensitivity to corticosterone among different rat strains has revealed remarkable differences (Dhabhar et al., Citation1997). In any case, the similarity between Sprague–Dawley rats and B6C3F1 mice (with the exception of MHC Class II expression) is of interest and has not been previously reported.
ACKNOWLEDGMENTS
This work was supported by a grant from Pfizer, Inc.
REFERENCES
- De Boer S. F., Van Der Gugten J., Slangen J. L. Effects of chlordiazepoxide, flumazenil, and DMCM on plasma catecholamine and corticosterone concentrations in rats. Pharmacol. Biochem. Behav. 1991; 38: 13–19
- Dhabhar F. S., McEwen B. S., Spencer R. L. Adaptation to prolonged or repeated stress—comparison between rat strains showing intrinsic differences in reactivity to acute stress. Neuroendocrinology 1997; 65: 360–368
- Dhabhar F. S., Satoskar A. R., Bluethmann H., David J. R., McEwen B. S. Stress-induced enhancement of skin immune function: A role for gamma interferon. Proc. Natl. Acad. Sci. USA 2000; 97: 2846–2851
- Engler H., Dawils L., Hoves S., Kurth S., Stevenson J. R., Schauenstein K., Stefanski V. Effects of social stress on blood leukocyte distribution: The role of alpha- and beta-adrenergic mechanisms. J. Neuroimmunol. 2004; 156: 153–162
- Finn D. A., Bejanian M., Jones B. L., Syapin P. J., Alkana R. L. Temperature affects ethanol lethality in C57BL/6, 129, LS, and SS mice. Pharmacol. Biochem. Behav. 1989; 34: 375–380
- Fuchs B. A., Pruett S. B. Morphine induces apoptosis in murine thymocytes in vivo but not in vitro: Involvement of both opiate and glucocorticoid receptors. J. Pharmacol. Exp. Ther. 1993; 266: 417–423
- Han Y. C., Pruett S. B. Mechanisms of ethanol-induced suppression of a primary antibody response in a mouse model for binge drinking. J. Pharmacol. Exp. Ther. 1995; 275: 950–957
- Han Y. C., Lin T. L., Pruett S. B. Thymic atrophy caused by ethanol in a mouse model for binge drinking: Involvement of endogenous glucocorticoids. Toxicol. Appl. Pharmacol. 1993; 123: 16–25
- Hebert P., Pruett S. B. Selective loss of viability of mouse NK cells in culture is associated with decreased NK cell lytic function. In Vitro Molec. Toxicol. 2001; 14: 71–82
- Hillbom M. E., Sarviharju M. S., Lindros K. O. Potentiation of ethanol toxicity by cyanamide in relation to acetaldehyde accumulation. Toxicol. Appl. Pharmacol. 1983; 70: 133–139
- Jerrells T. R., Marietta C. A., Weight F. F., Eckardt M. J. Effect of adrenalectomy on ethanol-associated immunosuppression. Int. J. Immunopharmacol. 1990; 12: 435–442
- Myers L. P., Fan R., Zhen Q., Pruett S. B. Sodium methyldithiocarbamate causes thymic atrophy by an indirect mechanism of corticosterone upregulation. J. Immunotoxicol. 2005; 2: 97–106
- Pruett S. B., Collier S., Wu W. J., Fan R. Quantitative relationships between the supression of selected immunological parameters and the area under the corticosterone concentration vs. time curve in B6C3F1 mice subjected to exogenous corticosterone or to restraint stress. Toxicol. Sci. 1999; 49: 272–280
- Pruett S. B., Fan R. Quantitative modeling of suppression of IgG1, IgG2a, IL-2, and IL-4 responses to antigen in mice treated with exogenous corticosterone or restraint stress. J. Toxicol. Environ. Health 2000; 62: 175–189
- Pruett S. B., Fan R., Myers L. P., Wu W. J., Collier S. D. Quantitative analysis of the neuroendocrine-immune axis: Linear modeling of the effects of exogenous corticosterone and restraint stress on lymphocyte subpopulations in the spleen and thymus in female B6C3F1 mice. Brain Behav. Immun. 2000a; 14: 270–287
- Pruett S. B., Fan R., Zheng Q., Myers L. P., Hebert P. Modeling and predicting immunological effects of chemical stressors: Characterization of a quantitative biomarker for immunological changes caused by atrazine and ethanol. Toxicol. Sci. 2003; 75: 343–354
- Pruett S. B., Fan R., Zheng Q., Myers L. P., Hébert P. Modeling and predicting selected immunological effects of a chemical stressor (3,4 dichloropropionanalide) using the area under the corticosterone concentration vs. time curve. Toxicol. Sci. 2000b; 58: 77–87
- Pruett S. B., Padgett E. L. Thymus-derived glucocorticoids are insufficient for normal thymus homeostasis in the adult mouse. BMC Immunol. 2004; 5: 24
- Reid S. A., Speedy D. B., Thompson J. M., Noakes T. D., Mulligan G., Page T., Campbell R. G., Milne C. Study of hematological and biochemical parameters in runners completing a standard marathon. Clin. J. Sport Med. 2004; 14: 344–353
- Schwab C. L., Fan R., Zheng Q., Myers L. P., Hebert P., Pruett S. B. Modeling and predicting stress-induced immunosuppression in mice using blood parameters. Toxicol. Sci. 2005; 83: 101–113
- Weiss P. A., Collier S. D., Pruett S. B. Role of glucocorticoids in ethanol-induced decreases in expression of MHC Class II molecules on B-cells and selective decreases in spleen cell number. Toxicol. Appl. Pharmacol. 1996; 139: 153–162