Abstract
Workers exposed to benzene frequently suffer from toxicities of the bone marrow as well as the central nervous, immune, and reproductive systems. This toxicity most likely is a result of the oxidative metabolism of benzene to reactive products. As green tea possesses antioxidant effects, the objective of this study was to examine any amelioration of benzene-induced oxidative stress in pump workers drinking 6 cups (150 ml/cup) of freshly prepared tea daily. Sixty male non-smoking subjects, divided into four groups: no benzene exposure/no green tea; no exposure/tea; exposure/no tea; and, exposure/tea, were monitored after a 6 mo period. On the final day of the study, urine samples were collected for analyses of benzene, trans-trans muconic acid, and phenol. Blood was also collected at this time; plasma was assayed for total antioxidant activity, malondialdehyde (MDA), and glutathione (GSH) while erythrocytes were analyzed for activity of antioxidant enzymes glutathione peroxidase (GSHPX), superoxide dismutase (SOD), and catalase. The results demonstrated that urinary levels of benzene, trans-trans muconic acid, and phenol were elevated in all pump workers, and that this elevation was mitigated by consumption of green tea. The benzene exposures also led to significant reductions in plasma GSH levels and erythrocyte antioxidant enzyme activities; these effects were abrogated (to near-control levels) by the tea. Interestingly, among control subjects, tea ingestion itself caused significant increases in both GSHPX and catalase activities. Unlike with the other plasma parameters, while the benzene exposures also significantly increased plasma MDA levels and decreased total antioxidant activity, tea ingestion did not cause a near-total reversion to control values; the effects on these two endpoints were more like those noted with the urine parameters (mitigation, not abrogation). These studies demonstrate that drinking green tea during benzene exposure can reduce several parameters indicative of oxidative stress. As such, as a dietary supplement, green tea could represent a potential therapeutic agent in reducing certain aspects of benzene-induced toxicity.
INTRODUCTION
Human exposure to benzene occurs both occupationally in the chemical and fuel industry and environmentally through automobile exhaust, gasoline, and cigarette smoke (Wallace, Citation1989). Benzene is oxidized in the liver by cytochrome P450 2E1 to form benzene oxide (Lovern et al., Citation1997). In turn, the oxide can be converted non-enzymatically to phenol, or enzymatically (via epoxide hydrolase and then dihydrodiol dehydrogenase to form catechol. Phenol itself undergoes further hydroxylation reactions to yield catechol or hydroquinone; each of these products, in turn, can be hydroxylated to yield 1,2,4-benzenetriol. Ring-opened metabolites may also form; the most common of these found in the urine are trans-trans-muconic acid (t,t-MA) and 6-hydroxy- t,t-2,4-hexadienoic acid (Seaton et al., Citation1994; Snyder and Hedli, Citation1996). While each of these metabolites can exert its own toxicity in situ, various combinations of the metabolites can exert a synergistic effect on multiple cellular targets, leading to increased toxicity (Guy et al., Citation1991).
Toxicity of benzene most likely results from oxidative metabolism of benzene to reactive products. Benzene can be enzymatically bioactivated to reactive intermediates that can lead to increased formation of reactive oxygen species (ROS). The peroxidatic metabolism of the benzene metabolites, phenol, and hydroquinone, results in arachidonic acid peroxidation and oxygen activation to superoxide radicals, respectively (Seaton et al., Citation1995). Silva et al. (Citation2003) found that hydroquinone can be metabolized to benzoquinones that are potent haematotoxins, genotoxins, and carcinogenins that can also induce the formation of radical species, i.e., they can also ultimately predispose cells to oxidative damage. Chronic benzene poisoning may result in DNA break in bone marrow cells and peripheral blood lymphocytes and decrease in the activity of antioxidative enzymes (Pan et al., Citation2003). Oxidative stress resulting from increased free-radical production and (or) defects in antioxidant defenses is implicated in the pathogenesis of several diseases (Southorn and Powis, 1988).
Tea is one of the most popular beverages consumed worldwide. Tea, from the plant Camellia sinensis, is consumed in different parts of the world as green, black, or oolong tea. Green tea is favored in Japan, China, and Libya, and most of the initial research on the benefits of green tea has been carried out in these countries. Green tea contains many compounds and numerous studies available in the literature have extensively characterized the composition of green tea (i.e., Xie et al., Citation1998; Astill et al., Citation2001; Ferrara et al., Citation2001; Zuo et al., Citation2002) and in some cases have focused on the content of antioxidants in the tea samples from around the world (Cabrera et al., Citation2003).
One of the more important constituents of the tea is the class of agents termed polyphenols; epidemiologic studies have shows that polyphenolic agents present in tea can reduce the risk of a variety of diseases (Crespy and Williamson, Citation2004; reviewed in Erba et al., Citation2005; Cabrera et al., Citation2006). The epicatechin derivatives (commonly called ‘polyphenols’) are the active ingredients in green tea. While these agents are known to possess antioxidant, anti-inflammatory, and anticarcinogenic properties (Katiyar et al., Citation2001; reviewed in Erba et al., Citation2005; Beltz et al., Citation2006; Shankar et al., Citation2007; Tipoe et al., Citation2007), to date there are no studies that have reported on potential protective effects of the tea against benzene-induced oxidative stress. The objective of the study here was to examine if there are any such effects; this was done by assessing if there was any amelioration of several indices of benzene-induced oxidative tress in non-smoking benzene pump workers that concurrently drank green tea over a 6 mo period.
MATERIALS AND METHODS
Subjects
This 6-mo study was carried out on sixty non-smoking (in past and/or currently) adult males (25-45 yr-of-age) from the cities of Sirte and Buni Ghazi, Libya. Subjects designated as controls were healthy persons with no occupational exposure to benzene frequency-matched to exposed benzene pump subjects by age and lifestyle. Occupational histories (working time, work shifts, work environ), with medical history, smoking status, and past consumption of green tea were prepared for all subjects. All participants in these studies were free of any medical disease, had similar dietary habits, and were not known to be potentially exposed to other environmental pollutants (or more precisely, sources of pollutants). The control and benzene-exposed workers were then divided into four equal groups (15 individuals/group) as follows:
Group I: Control group not drinking green tea. Drank 6 cups hot water/d instead.
Group II: Control group drinking green tea (900 ml tea/d in 150 ml [1cup] amounts).
Group III: Benzene-exposed workers not drinking tea. Drank 6 cups hot water/d instead.
Group IV: Benzene-exposed workers drinking green tea (see above).
All subjects drank either the green tea or water solutions at rates of 1 cup every 4 hr; this meant that they were drinking at least 2 cups of tea or water during each 8 hr workshift and the remaining 4 cups at regular intervals over the course of their time not on the jobsite.
Preparation of Green Tea
The green tea was obtained from Jafferjee Brothers Co. (Colombo, Sri Lanka) and consumed without any additive. Filtered cold water was used in preparation of the green tea (Imai and Nakachi, Citation1995). Each solution was prepared using 0.5 g tea/150 ml water. After bringing the water to a rolling boil, it was cooled to 70–80°C before being poured over the tea leaves. The tea sample was immediately consumed after preparation.
Measurement of Urinary Benzene and its Metabolites
Control subjects and benzene-exposed workers each provided a spot urine sample at the end of their workshift on the last day of the last week of the 6-mo study period. Samples were aliquoted immediately, transported on dry ice, and stored at −20°C until analysis of benzene and its metabolites. Based on the method of Waidyanatha et al. (Citation2001), urinary benzene was determined via headspace solid-phase microextraction using a Varian Model 8200 autosampler (Walnut Creek, CA) with an Autotherm Peltier controller and carousel (Strumenti Scientific, Padova, Italy). Briefly, 0.5 ml urine was transferred to a vial containing 0.5 g NaCl to which 1 μ l of a methanol solution of internal standards (containing 2.5 μ g/ml [2H6]-benzene) was added. The vials were capped and placed in a carousel at 45°C for 15 min to allow analytes to equilibrate. Analytes were concentrated from the vial headspace onto a Supelco (Bellefonte, PA) polydime-thylsiloxane fiber (10-mm × 100-μ m film thickness) for 15 min and then analyzed by GC-MS (gas chromatography-mass spectrometry) in a selected ion monitoring (SIM) mode using a Hewlett-Packard 5980 Series II gas chromatograph coupled to an HP 5971A mass selective detector (Hewlett Packard, Palo Alto, CA). A DB-5 fused silica capillary column was used (60 m, 0.25-mm inner diameter, 0.25-μ m film thickness). Quantitation was based on peak area ratios of the analytes to the internal standards.
Among the possible metabolites of benzene found in the urine, this study measures trans-trans muconic acid and phenol. The urine samples were purified and the trans-trans muconic acid was determined by the HPLC method, equipped with a Shimadzu/Lc-9a pump (1 ml/min) and an Aypersil ODS 250 × 4.6 mm column maintained at 40°C, with a UV (Gibson) spectrophotometer (at 259 nm) (Figa-talamanca and Salera, Citation2001). Phenol was determined spectrophotometrically in a PU 8630 UV-VIS spectrophotometer (Phillips, Sydney, Australia) using aminoantipyrine as reagent. Pure solutions of phenol (Sigma, St. Louis, MO) were used as standards (Verma et al., Citation1999). Urinary creatinine concentrations were analyzed using a standard method (after picric acid reaction) and monitoring absorbance at 520 nm (Baselt, Citation1980).
Collection of Blood Sample
On the last day of the last shift of the 6-mo period, a blood sample (10 ml) from a hand (antecubital) vein was taken between 8:00 and 9:00 AM after an overnight fasting. The blood was centrifuged at 1000 × g for 15 min and the plasma isolated. The separated erythrocytes were carefully sampled from the bottom of the tube to minimize leukocyte contamination. The cells were then washed three times with isotonic saline solution, lysed by addition (1:6, by volume) of double-distilled water containing 5 ml Triton X-100, vigorously vortexed, and then stored on ice for 10 min. A membrane-free hemolysate was then obtained by centrifugation of the solution at 10,000 × g for 5 min. Lysate hemoglobin (Hb) concentration was determined with a Coulter Counter T660 (Coulter Electronics, Margency, France). To assay superoxide dismutase (see below), Hb was first extracted from the hemolysate by addition of an equal volume of chloroform:ethanol (3:5, v/v), followed by centrifugation. In all cases, hemolytic, icteric, or turbid samples were discarded.
Assay of Plasma Total Antioxidant Status
Total antioxidant status of each plasma sample was measured in triplicate via a novel automated colorimetric measurement method (Erel, Citation2004). Briefly, hydroxyl radical is produced by a Fenton reaction and reacts with colorless o-dianisidine substrate to produce dianisyl radical (bright yellow-brown color). Upon addition of a 100 μ l aliquot of plasma sample, oxidative reactions initiated by hydroxyl radicals present in the mixture are suppressed by the antioxidant components in the plasma. By comparison against a reference blank lacking serum, the extent of prevention of the color change associated with dianisyl formation provides an effective measure of total antioxidant capacity. Precision of the assay is very high, with an in-run coefficient of variation of < 3.5% (Cao and Prior, Citation1998). All assay results are expressed as mmol Trolox equivalence/L.
Assay of Erythrocyte Glutathione Peroxidase (GSHPX) Activity
GSHPX activity in each hemolysate was determined in triplicate in a commercially-available kit (Ransel, Randox Labs, Crumlin, North Ireland). The assay is based upon the GSHPX-mediated oxidation of GSH during reduction of cumene hydroperoxide substrate (Paglia and Valentine, Citation1967). In the presence of glutathione reductase and NADPH, the oxidized glutathione is immediately converted to the reduced form with a concomitant oxidation of NADPH. Drabkin reagent (cyanide-ferricyanide solution [pH 7.0–7.4]) was prepared by dissolving potassium ferricyanide (200 mg), potassium cyanide (50 mg), and potassium dihydrogen phosphate (140 mg) in distilled water, making the volume up to 1 L, and mixing after the addition of 0.5 ml of a 250 g Brij 35/L solution. For the GSHPX assay, hemolysate (50 ml) was diluted with 1 ml Ransel diluting agent and incubated for 5 min, followed by addition of 1 ml Drabkin reagent and mixing (total dilution of hemolysate before assay, 1:82). The diluting agent reduces any GSSG present in the hemolysates to GSH because the cyanide in the Drabkin reagent rapidly inactivates GSSG. The cyanide also inhibits other peroxidases that may be present in human blood and prevents falsely high results. In the actual assay, 0.1 ml of the diluted hemolysate in combined with a solution containing 1 unit of the reductase and 0.1 ml of 0.2 mM NADPH. Upon addition of 40 μ l 1.5 mM cumene hydroperoxide solution, the absorbance was monitored at 340 nm over a 3 min period in a spectrophotometer to determine the rate of conversion of the NADPH to NADP+. A unit of activity in each sample is defined as 1 nmole NADPH oxidized/min.
Assay of Erythrocyte Superoxide Dismutase (SOD) Activity
SOD activity in each hemolysate sample was measured in triplicate in a commercially-available kit (RANSOD, Randox Labs). The assay employs xanthine oxidase to generate superoxide radicals that, in turn, react with INT (2-(4-iodophenyl)-3-(4-nitrophenol)-5-phenyltetrazolium chloride) to form red formazan dye. The SOD activity in a sample is then estimated from the degree of inhibition of this reaction (i.e., based upon the competition between the SOD and INT for the oxidase-generated radicals). For the assay, each lysate sample (20 μ l) was first diluted 25-fold in 0.01 mM phosphate buffer (pH 7.0) before addition to the reaction mixture. The sample absorbance was then monitored at 505 nm for 3 min at 37°C in a spectrophotometer. Actual activity in each sample was then calculated by extrapolation from a calibration curve generated with purified SOD. Phosphate buffer or various amounts of standard SOD in place of sample were used as blanks or for standard curve determinations, respectively. The unit of enzyme activity was defined as the amount of enzyme that inhibited rate of formazan dye formation by 50% (i.e., relative to activity in buffer blank).
Assay of Erythrocyte Catalase (CAT) Activity
CAT activity in hemolysate was assayed in triplicate by the method of Aebi (Citation1984) and is based on the determination of the rate constant (sec− 1; k) for the decomposition of H2O2. Briefly, a 10 μ l aliquot of hemolysate was diluted with phosphate buffer (50 mM, pH 7.0) to a final volume of 5 ml. CAT activity was then measured by adding 1 ml H2O2 to 2 ml diluted hemolysate and monitoring decreases in absorbance at 240 nm for 3 min (at 37°C) in a spectrophotometer; blanks consisted of 1 ml deionized water in place of the H2O2. One unit of CAT activity was defined as the amount of enzyme that degraded one mmol H2O2/min at 37°C and pH 7.4, using an initial concentration of 10 mM H2O2. Activity was ultimately expressed as K/g hemoglobin (K: rate constant of first order reaction, as defined by Aebi [1974]).
Assay of Plasma Malondialdehyde
Concentration of MDA in plasma was assayed using a chromogenic assay (Bioxytech LPO-586, Oxis International Inc., Portland, OR) that measures free and protein-bound MDA without undue interference from other lipid peroxidation products. All samples (each time, 100 μ l aliquots of serum) were analyzed in triplicate. Standard curves (range 0-20 μ M) were prepared for each assay using the chromogen supplied in the kit. The assay has a detection limit of 0.1 μ M and an interassay variation of < 5%.
Assay of Erythrocyte Reduced Glutathione (GSH)
The level of erythrocyte reduced glutathione was assayed in triplicate by the method of Beutler et al. (Citation1963). A 0.2 ml aliquot of whole blood and 1.8 ml distilled water were mixed in a test tube to prepare a hemolysate. Precipitating solution (1.67 g glacial meta-phosphoric acid, 0.2 g Na2EDTA, and 30 g NaCl dissolved in 100 ml distilled water) was added (3 ml) and the entire solution filtered through coarse grade filter paper after 5 min. An “Assay” cuvette received 2.0 ml filtrate; a “Blank” cuvette received 1.2 ml precipitating solution and 0.8 ml distilled water. Reagent buffer (0.8 ml 0.3 M Na2HPO4 solution) and DTNB (1.0 ml 0.75 mM 5,5′-dithiobis-2-nitrobenzoic acid) solution were then added to each cuvette. Both cuvettes were capped, inverted three times, and their absorbance at 412 nm measured after 3 min. Concentrations of GSH in each sample were extrapolated from a standard calibration curve prepared using different GSH calibrators (50, 10, 5, 4, and 2 mg/dl); ultimately, true GSH content in the samples was adjusted to accommodate the presence of hemoglobin, viz. GSH (mg/dl of RBC) = GSH concentration (based on calibration curve)/Hb content present (gm/dl) (i.e., end-value in terms of μmole/g Hb).
Statistical Analysis
Data are expressed as (mean ± SD). Statistical comparison between different groups was performed using one way analysis of variance (ANOVA), followed by Tukey-Kramer multiple comparison test to compare the difference between various groups. Significance was accepted at p < 0.05.
RESULTS
The evaluation of risk posed by exposure to benzene from occupational and environmental sources is an active area of research. This study focused on benzene-induced oxidative stress and the potential role that green tea might have in attenuating the oxidative stress induced by benzene in occupationally-exposed populations, here, benzene pump workers.
The average level of benzene in air was found to be ≤ 25 ppm for all workers from the first day of their starting on the job. The average period of exposure (hr/8 hr workday) to the benzene-bearing atmosphere was 4.18 (± 0.75 SD) and 4.17 (± 0.72 SD) for workers in Group III and Group IV, respectively. All workers exposed to benzene showed a significant elevation in urinary benzene and its metabolites (trans-trans muconic acid and phenol) levels as compared with levels of each product in subjects in both control groups (). While daily consumption of the green tea among the subjects in Group IV appeared to decrease the degree of excretion of benzene and its metabolites relative to that in non-tea drinking counterparts (i.e., levels signifi-cantly [i.e., 29.8, 29.7, and 39.6% respectively] less than that of non-tea-drinking colleagues in Group III), levels of urinary benzene, trans-trans muconic acid, and phenol in this single sample at the end of the last day of the study were still higher than in any of the non-benzene-exposed counterparts (tea drinking or not).
TABLE 1 Urinary benzene and its metabolites (trans, trans muconic acid and phenol) in benzene pump workers and controls
The present work showed no significant difference in total antioxidant activity in plasma in the control subjects due to drinking of the green tea. While there was significant reduction in total antioxidant activity in the plasma of all benzene pump workers compared to their respective control counterparts (; Group III vs. Group I = 30.6% reduction, Group IV vs. Group II = 15.6% reduction), the degree of reversal of the benzene-induced effect on this parameter – although trending positively – was not yet statistically significant.
FIG. 1 Total antioxidant activity in benzene-exposed pump workers and controls. Values shown represent the mean ± SD levels associated with venous blood samples collected from each of 15 subjects/group at the end of the 6-mo study period. Treatments were: Group I: Control group not drinking green tea; Group II: Control group drinking green tea daily; Group III: Benzene-exposed workers not drinking green tea; Group IV: Benzene-exposed workers drinking green tea daily. *Value significantly different from Group I at p < 0.05. #Value significantly different from Group II at p < 0.05. “Value significantly different from Group III at p < 0.05.
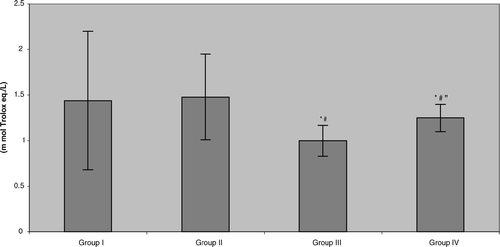
In contrast, it seemed that drinking the tea caused a significant increase in erythrocyte glutathione peroxidase (GSHPX) activity in the control populations (). Once again, the data indicated that all of the benzene-exposed pump workers displayed small but significant reductions in this activity relative to their respective control counterparts (Group III vs. Group I = 8.4%, Group IV vs. Group II = 4.0%). With this parameter, consumption of the tea by the benzene-exposed workers did return activity to levels seen in non-tea drinking controls, but surprisingly, not all the way up to the levels in the tea-drinking controls (i.e., Group II). Accordingly, this indicates that green tea consumption could help to normalize erythrocyte glutathione peroxidase levels in benzene pump workers, i.e., activity level is statistically greater than in non-tea drinking benzene-exposed counterparts.
FIG. 2 Erythrocyte glutathione peroxidase (GSHPX) activity in benzene-exposed pump workers and controls. Values shown represent the mean ± SD levels associated with venous blood samples collected from each of 15 subjects/group at the end of the 6-mo study period. Treatments are as outlined in legend to . *Value significantly different from Group I at p < 0.05. #Value significantly different from Group II at p < 0.05. “Value significantly different from Group III at p < 0.05.
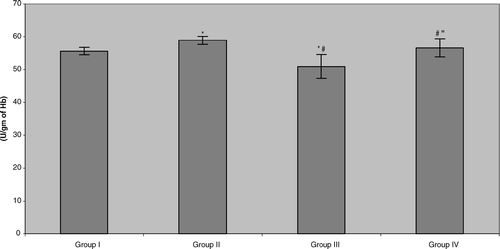
The present study also showed that erythrocyte superoxide dismutase (SOD) activity in the plasma of benzene pump workers was significantly reduced (≈ 28.9%) compared with both control groups (). Unlike with the other two parameters reported above, in this case, tea consumption did cause significant, and near-total, reversal of the benzene-induced effect. Similar results were noted in the assessments of catalase and glutathione ( and ). The data indicated that all benzene-exposed workers displayed small but significant reductions in catalase activity relative to their respective control counterparts (Group III vs. Group I = 7.8%, Group IV vs. Group II = 7.9%). As with the GSHPX, consumption of the tea by these workers did return activity to levels seen in non-tea drinking controls, but not to the levels in Group II tea-drinking controls. Erythrocyte reduced glutathione levels in the benzene-exposed workers were significantly depleted (≈ 40.4%) compared with both control groups. More akin to the results for the SOD, the workers that were drinking green tea during the 6-mo period reattained/maintained, GSH levels that reflected near complete abrogation/mitigation of the benzene-induced toxicity.
FIG. 3 Erythrocyte superoxide dismutase (SOD) activity in benzene-exposed pump workers and controls. Values shown represent the mean ± SD levels associated with venous blood samples collected from each of 15 subjects/group at the end of the 6-mo study period. Treatments are as outlined in legend to . *Value significantly different from Group I at p < 0.05. #Value significantly different from Group II at p < 0.05. “Value significantly different from Group III at p < 0.05.
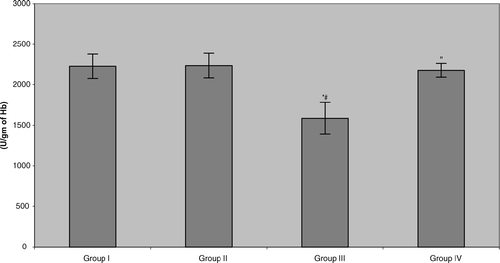
FIG. 4 Erythrocyte catalase (CAT) activity in benzene-exposed pump workers and controls. Values shown represent the mean ± SD levels associated with venous blood samples collected from each of 15 subjects/group at the end of the 6-mo study period. Treatments are as outlined in legend to . *Value significantly different from Group I at p < 0.05. #Value significantly different from Group II at p < 0.05. “Value significantly different from Group III at p < 0.05.
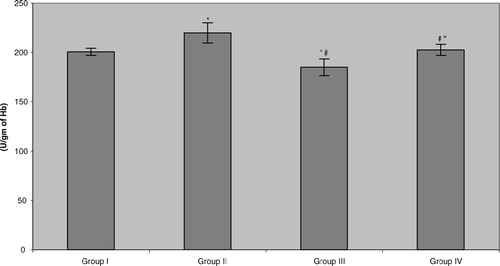
FIG. 5 Erythrocyte reduced glutathione (GSH) levels in benzene-exposed pump workers and controls. Values shown represent the mean ± SD levels associated with venous blood samples collected from each of 15 subjects/group at the end of the 6-mo study period. Treatments are as outlined in legend to . *Value significantly different from Group I at p < 0.05. #Value significantly different from Group II at p < 0.05. “Value significantly different from Group III at p < 0.05.
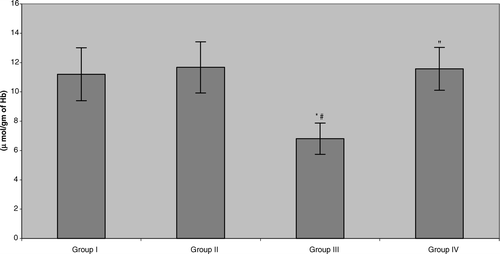
Unlike all of the other aforementioned parameters analyzed, plasma malondialdehyde (MDA) levels in all benzene-exposed workers were significantly elevated (> 3-fold) compared with the levels seen in subjects in both control groups (). Nevertheless, consumption of the green tea led to significant reductions – albeit not to actual levels of those in the controls (i.e., now only ≈ 70% above control) – in measured MDA.
FIG. 6 Plasma malondialdehyde (MDA) levels in benzene-exposed pump workers and controls. Values shown represent the mean ± SD levels associated with venous blood samples collected from each of 15 subjects/group at the end of the 6-mo study period. Treatments are as outlined in legend to . *Value significantly different from Group I at p < 0.05. #Value significantly different from Group II at p < 0.05. “Value significantly different from Group III at p < 0.05.
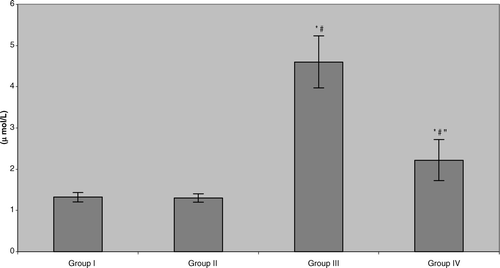
DISCUSSION
Most assessments of the potential adverse health effects of benzene have focused on occupationally-exposed workers, in particular, men. We conducted this study to evaluate oxidative stress associated with benzene exposure and the potential role for green tea to attenuate this toxicity. To perform this evaluation, 30 pump workers currently exposed to benzene and 30 non-benzene-exposed control workers, were recruited for this study. Within each population, half then consumed 900 ml (6 cups) of freshly-prepared green tea daily over a 6-mo period. Biomarkers of benzene exposure and several biochemical parameters associated with antioxidative protection in the body were then analyzed at the study's end.
Under the conditions that prevailed at the work place, benzene was delivered into the body primarily by inhalation; however, skin absorption of benzene in the pumps as well as in droplets in the workshop atmosphere was also considered to be potentially significant (Verma and Rana, Citation2001). Under normal exposure scenarios, in an adult male ≈ 17% of the benzene entrained in each breath is exhaled. The remaining 83% (i.e., the absorbed dose) is metabolized and eventually excreted as a variety of urinary products, including phenolic compounds (e.g., phenol, hydroquinone, catechol, trihydroxybenzene), t,t-muconic acid (MA), and S-phenylmercapturic acid (SPMA), as well as a small amount of unmetabolized benzene. In fact, all metabolites, except 1,2,4-trihydroxybenzene (benzene triol, BT), are good markers for benzene exposure (Waidyanatha et al., Citation2001). Urinary t,t-MA is considered a specific indicator of benzene exposure; the concentration of urinary t,t-MA among non-exposed individuals in the general population is at-or-below detection levels in most assays (Ghittori et al., Citation1995).
In the present study, urinary levels of benzene and at least two of its metabolites (phenol and t,t−MA) were significantly elevated among all benzene pump workers as compared to levels in the control subjects, but trended back toward the control levels among workers that had consumed the green tea over the 6-mo period. Mulder et al. (Citation2005) found that short-term (i.e., over a 2 d period) green tea consumption by healthy adults resulted in a general increase in the excretion of several phenolic compounds (primarily derived from tea constituents). However, those Authors did not provide any information as to whether the tea caused any increases in excretion of non-tea-derived phenolic agents. Nevertheless, based on those findings, it was expected here that levels of benzene metabolite excretion would be greater in Group IV subjects. Oddly, consumption of tea by those pump workers seemed to lead to decreased excretion of benzene/metabolites as compared to that by non-tea-drinking benzene-exposed counterparts.
There are three reasonable explanations for these unexpected findings. (1) Some factors in the tea caused decreases in overall metabolism/excretion of benzene; the findings of increases (after tea consumption) in antioxidant levels and activities of enzymes critical to maintenance of antioxidant levels seem to preclude this. (2) The tea did cause increases in excretion of benzene and its metabolites, but the workday's exposure itself masked the effect (i.e., urinary levels prior to workshift were probably closer to that of controls [and much lower than baseline levels of Group III subjects] and, as expected, rose in response to the day's exposure [albeit not to levels of Group III subjects]). Future investigations will need to verify whether pre-workshift urine samples in tea-drinking workers significantly differ from controls. Third, due to the presence in the tea of significant amounts of caffeine (Mizukami et al., Citation2007) which is known to increase both the number of urinations/day and volume/voiding (Bird et al., Citation2005), it is likely there was both increased metabolism of benzene and greater total excretion of it and each metabolite over the course of the entire workday (i.e., and so the appearance of a lower value in the single sampling [of a larger void volume] that took place at end of the workday). Whether there are differences in total amounts of benzene/each metabolite excreted over the course of the entire workday will need to be examined during a repeat of this study. It would be of great interest in future studies to determine if treatment with green tea after cessation of all exposure to benzene causes more rapid “clearance” of any remaining benzene as compared to rates in counterparts not provided tea (i.e., an inexpensive potential treatment for any accidental benzene exposure).
Oxidative stress is an imbalance between oxidant production and antioxidant defenses (Kunsch and Medford, Citation1999). Reactive oxygen species (ROS), which include hydrogen peroxide and the superoxide, hydroperoxyl, and hydroxyl radicals, are generated in many physiological processes. While the cell has developed an array of non-enzymatic and enzymatic anti-oxidative mechanisms to detoxify ROS, oxidative stress can occur when xenobiotic bioactivation leads to an imbalance between ROS formation and detoxification (i.e., a situation evolves favoring a net increase in formation/persistence of ROS; Gutteridge and Halliwell, 2000). This increased presence of ROS can lead to several types of damage in cells; molecular targets of ROS-initiated damage in cells include proteins, lipids, and DNA. With respect to benzene, specific metabolites have been shown to initiate extensive oxidative damage in HL60 cells (Kolachana et al., Citation1993; Shen et al., Citation1996; Ruiz-Ramos et al., Citation2005) and to cause lipid oxidation in an animal model (Gaido and Wierda, Citation1987). These findings provide support for asserting that there might be a potential role for ROS in benzene-initiated toxicity. Georgieva et al. (Citation2002) also found that benzene induced misbalance in the antioxidant status. Ahmed et al. (1994) reported that an observed decrease in antioxidant potentials in treated rats may have been due to oxidation reactions initiated by benzene metabolites, particularly hydroquinone and 1,2,4-benzenetriol. Because Erba et al. (Citation2005) found that green tea consumption (twice daily for 42 d) by adult female volunteers resulted in elevated plasma total antioxidant activity levels, the present study sought to determine if consumption of the tea during the course of a chronic benzene exposure could also mitigate/abrogate these expected induced imbalances. Results here clearly showed that while benzene exposure induced significant reduction in total antioxidant activity, concurrent drinking of green tea increased the levels of total antioxidant activity significantly back in the direction of the levels found in non-benzene-exposed subjects.
For each of the enzymes critical to preventing oxidative stress that were studied here (i.e., GSHPX, SOD, CAT), there is evidence that benzene exposure(s) can significantly reduce each of their activities. What is not clear is if in all cases the observed reductions in activity were based upon effects on the enzymes per se (i.e., [redox-based] modifications of the protein structures), normal inhibition (competitive, non-, or uncompetitive) mechanisms, or simple over-utilization. For example, Georgieva et al. (Citation2002) and Chen (Citation1992) each demonstrated that GSHPX activity levels in the plasma of benzene-exposed workers were significantly decreased. Fabiani et al. (Citation2005) found that SOD was critical to mitigating potential DNA-damaging effects that could evolve from intra/extracellular reactions of serum components with benzoquinone metabolite; as such, levels of SOD might be expected to become “depleted” due any near-constant presence of benzene/its metabolites. Unfortunately, neither Ugurnal et al. (Citation1995) nor Georgieva et al. (Citation2002) found significant decreases in the activities of SOD in their respective benzene-exposed animal or human hosts. Lastly, as an H2O2 scavenger, CAT has been shown to play an important role in the protection of cells against toxicity from the hydroquinone metabolite (Horita et al., Citation1997; Ahmed et al., 2000). Furthermore, Czerski and Szymanska (Citation2005) suggested that the decreases in catalase activity levels they noted in livers of rats fed brominated benzene compounds could be a likely result of enzyme inactivation by certain metabolites of benzene. Based on these earlier findings, except in contrast to the surprising “lack of effect” on SOD noted above, it was expected that benzene exposures of the workers here should have led to significant decrements in the activities of all three enzymes in their blood. In the absence of any tea consumption, the results here confirmed these expectations.
Effects from consumption of green tea upon each of these enzymes has similarly been documented. For example, Hamdaoui et al. (Citation2005) found that consumption of green tea in drinking water by rats for a period of 6 wk resulted in significant elevations in blood levels of GSHPX. Sabu et al. (Citation2002) noted that providing green tea for 15 d to alloxan-induced diabetic rats led to significant dose-related increases in the activities of hepatic SOD. Lastly, Negishi et al. (Citation2004) observed that there were significantly increased levels of catalase expression in aortic tissues recovered from rats provided green tea daily for a period of 3 wk. In each of these studies, the significant increases were against normal/diabetic counterparts that had not received the tea in their water during the respective studies. It is interesting to note that in this current study, the activities of GSHPX and CAT were both significantly increased by tea consumption among the control non-benzene-exposed workers, but that the SOD activity levels appeared to remain unaffected. Whether the measurably increased SOD activity noted by the Sahu group is limited to the liver and not a systemic effect remains to be determined in future investigations. Nevertheless, as a result of these stimulatory effects, the simple consumption of green tea by the pump workers throughout the exposure period was enough to completely abrogate the inhibitory effects from the hydrocarbon on each of these critical antioxidant enzymes.
A widely used index to estimate oxidative stress in vivo is to measure lipid peroxidation end products, i.e., plasma malondialdehyde (MDA). The common approach to assay MDA levels is indirect and based on quantifying levels of thiobarbituric acid–reacting substances (TBARS) in a sample (Belch et al., Citation1991). Benzene exposure has been associated with increased lipid peroxidation in plasma, as shown by an increase in the overall formation of TBARS, including MDA (Chen, Citation1992; Ahmed et al., 1994). The present study showed that plasma MDA levels were significantly elevated (i.e., more than 3-fold) in benzene pump workers compared with controls. Catechins that are major components (constitute 30% of the dry weight) of green tea can prevent oxidative damage either through free radical-quenching, metal-chelation, or recyc-ling other antioxidants such as Vitamin E (Arts et al., Citation2001). Both Coimbra et al. (Citation2006) and Hirano-Ohmori et al. (Citation2005) in healthy adult humans, and Crespy and Williamson (Citation2004) in normal animal models, reported that serum MDA levels were significantly decreased after daily green tea intake over anywhere from 2–4 wk. In line with these findings, the results of the present study clearly showed that the plasma level of MDA in benzene pump workers that were drinking green tea daily was significantly lower than that in counterparts not drinking green tea. As with the total antioxidant activity results, but unlike the results with the three enzymes studies here, the reversing effect was not total, i.e., the levels seen in the Group IV workers did not reach those of either control group subjects. As noted earlier, we believe that this might be related to the fact that these workers are being near-continuously exposed to benzene. Again, it would be of interest in future studies to verify if treatment with green tea after cessation of exposure to benzene could cause more rapid “clearance” of any remaining benzene as compared to rates in counterparts not provided the tea.
The final biochemical parameter analyzed in each of the study subjects was levels of reduced glutathione (GSH). While GSH is a small intracellular thiol (-SH) molecule, its strong electron donating capacity (i.e., high negative redox potential) combined with high intracellular concentration underlies its potent role as an antioxidant, enzyme co-factor, and critical compo-nent of a complex thiol exchange system that hierarchically regulates cell activity (Kosower and Kosower, Citation1978). Among the other major functions of GSH in cells is its role in the reductive elimination of H2O2 and other organoperoxide reactive oxygen species/metabolites that can irreversibly damage hemoglobin and cleave C-C bonds in phospholipid tails present in the cell membrane (Voet and Voet, Citation1999). As a result of the redox-active nature of many of the metabolites of benzene, it is then not unexpected for GSH levels in exposed cells/hosts to be modulated. Recalling that the first step in benzene metabolism is the formation of the benzene oxide epoxide, GSH in cells/biological mileus can be consumed during the complexation reaction that yields phenylmercapturic acid (Smith et al., Citation1989). Depletion of GSH can also occur in indirect manners. For example, 2,3,5-tris-(glutathion-S-yl)-hydroquinone (TGHQ), a putative metabolite of benzene, induces depletion of glutathione (GSH) in an ROS-independent manner (Bratton et al., Citation2000). The benzene metabolite p-benzoquinone is also highly reactive and can readily deplete cells of their GSH content (Brunmark and Cadenas, Citation1988).
A major flavonoid component of green tea is (-)-epigallocatechin gallate (EGCG). It has been shown that EGCG is itself a potent antioxidant and that treatment with this compound can result in increased levels of hepatic glutathione (Fiorini et al., Citation2005). Almurshed (2005) showed that green tea consumption itself could cause elevations in systemic GSH in rats and provide protection against subsequent toxicities from exposure to carbon tetrachloride. As in these cited and many other studies of the effects of green tea or its individual constituents upon glutathione (reduced, oxidized, or total forms), it remains unclear whether the effects observed are due to (A) modifications in synthesis of the thiol, (B) shifts in its status (i.e., oxidized vs. reduced) at the time of measurements due to modulation of the activity of GSH-utilizing enzyme(s), or (C) reductions in the level of GSH consumption (i.e., modified formation of Phase II-type products). Regardless of the precise mechanism of effect, the results of the study here showed that drinking green tea daily completely prevented/reversed the GSH-depleting effects from benzene exposure among the pump workers.
The results of these studies clearly demonstrate that drinking of green tea during benzene exposure can reduce (or completely reverse/prevent) several toxicities that are indicative of benzene-induced oxidative stress in pump workers. As such, green tea, as a dietary supplement, could represent a potential therapeutic agent for reducing certain aspects of benzene-induced toxicity.
REFERENCES
- Aebi H. Catalase. Methods of Enzymatic Analysis, Vol. 2, H. U. Bergmeyer. Verlag. Chemie, Weinheim 1974; 673–678
- Aebi H. Catalase in vitro. Meth. Enzymol. 1984; 105: 121–126
- Ahmad S., Agrawal R., Agrawal D. K., Rao G. S. Bioreactivity of glutathionyl hydroquinone with implications to benzene toxicity. Toxicology 2000; 150: 31–39
- Ahmad S., Singh V., Rao G. S. Antioxidant potential in serum and liver of albino rats exposed to benzene. Indian J. Exp. Biol. 1994; 32: 203–206
- Almurshed K. S. Protective effect of black and green tea against carbon tetrachloride-induced oxidative stress in rats. Saudi Med. J. 2006; 27: 1804–1809
- Arts I. C. W., Hollman P., Feskens E., Mesquital B., Kromhout D. Catechin intake might explain the inverse relation between tea consumption and ischemic heart disease: The Zutphen Elderly Study. Am. J. Clin. Nutr. 2001; 74: 227–232
- Astill C., Birch M. R., Dacombe C., Humphrey P. G., Martin P. T. Factors affecting the caffeine and polyphenol contents of black and green tea infusions. J. Agr. Food Chem. 2001; 49: 5340–5347
- Baselt R. C. Biological Monitoring Methods for Industrial Chemicals. Biomedical Publications, Davis, CA 1980; 207–211
- Belch J. J., Bridges A. B., Scott N., Chopra M. Oxygen free radicals and congestive heart failure. Br. Heart J. 1991; 65: 245–248
- Beltz L. A., Bayer D. K., Moss A. L., Simet I. M. Mechanisms of cancer prevention by green and black tea polyphenols. Curr. Med. Chem. - Anti-Cancer Agents 2006; 6: 389–406
- Beutler E., Duron O., Kelly B. M. Improved method for the determination of blood glutathione. J. Lab. Clin. Med. 1963; 61: 882–888
- Bird E. T., Parker B. D., Kim H. S., Coffield K. S. Caffeine ingestion and lower urinary tract symptoms in healthy volunteers. Neurourol. Urodyn. 2005; 24: 611–615
- Bratton S. B., Lau S. S., Monks T. J. The putative benzene metabolite 2,3,5-tris(glutathion-S-yl)hydroquinone depletes glutathione, stimulates sphingomyelin turnover, and induces apoptosis in HL-60 cells. Chem. Res. Toxicol. 2000; 13: 550–556
- Brunmark A., Cadenas E. Reductive addition of glutathione to p-benzoquinone, 2-hydroxy-p-benzoquinone, and p-benzoquinone epoxides. Effect of hydroxy- and glutathionyl substituents on p-benzoquinone autooxidation. Chem. Biol. Interact. 1988; 86: 273–298
- Cabrera C., Artacho R., Gimenez R. Beneficial effects of green tea - a review. J. Am. Coll. Nutr. 2006; 25: 79–99
- Cabrera C., Gimenez R., Lopez M. C. Determination of tea components with antioxidant activity. J. Agr. Food Chem. 2003; 51: 4427–4435
- Cao G., Prior R. L. Comparison of different analytical methods for assessing total antioxidant capacity of human serum. Clin. Chem. 1998; 44: 1309–1315
- Chen Y. Y. Effects of benzene on lipid peroxidation and the activity of relevant enzymes in humans. Chinese J. Prevent. Med. 1992; 26: 336–338
- Coimbra S., Castro E., Rocha-Pereira P., Rebelo I., Rocha S., Santos-Silva A. The effect of green tea in oxidative stress. Clin. Nutr. 2006; 25: 790–796
- Crespy V., Williamson G. A. Review of the health effects of green tea catechins in animal models. J. Nutr. 2004; 134: 3431S–3440S
- Czerski J., Szymanska J. A. The effect of repeated administration of selected benzene bromoderivatives on the liver catalase activity in rats. Int. J. Occup. Med. Environ. Health 2005; 18: 275–279
- Erba D., Riso P., Bordoni A., Foti P., Biagi P. L., Testolin G. Effectiveness of moderate green tea consumption on antioxidative status and plasma lipid profile in humans. J. Nutr. Biochem. 2005; 16: 144–149
- Erel O. A novel automated method to measure total antioxidant response against potent free radical reactions. Clin. Biochem. 2004; 37: 112–119
- Fabiani R., De Bartolomeo A., Morozzi G. Involvement of oxygen free radicals in the serum-mediated increase of benzoquinone genotoxicity. Environ. Mol. Mutagen. 2005; 46: 156–163
- Ferrara L., Montesano D., Senatore A. The distribution of minerals and flavonoids in the tea plant (Camellia sinensis). Farmaco 2001; 56: 397–401
- Figa-talamanca I., Salera E. A. Exposure to benzene among workers in a petroleum transport company. J. Occup. Health 2001; 43: 53–55
- Fiorini R. N., Donovan J. L., Rodwell D., Evans Z., Cheng G., May H. D., Milliken C. E., Markowitz J. S., Campbell C., Haines J. K., Schmidt M. G., Chavin K. D. Short-term administration of (-)-epigallocatechin gallate reduces hepatic steatosis and protects against warm hepatic ischemia/reperfusion injury in steatotic mice. Liver Transpl. 2005; 11: 298–308
- Gaido K. W., Wierda D. Suppression of bone marrow stromal cell function by benzene and hydroquinone is ameliorated by indomethacin. Toxicol. Appl. Pharmacol. 1987; 89: 378–390
- Georgieva T., Michailova A., Panev T., Popov T. Possibilities to control the health risk of petrochemical workers. Int. Arch. Occup. Environ. Health 2002; 75: 21–26
- Ghittori S., Maestri L., Fiorentino M. L., Imbriani M. Evaluation of occupational exposure to benzene by urineanalysis. Int. Arch. Occup. Environ. Health 1995; 67: 195–200
- Gutteridge J. M., Halliwell B. Free radicals and antioxidants in the year. Ann. N.Y. Acad. Sci. 2002; 899: 136–147
- Guy R. L., Hu P., Witz G., Goldstein B. D. Depression of iron uptake into erythrocytes in mice by treatment with the combined benzene metabolites p-benzoquinone, muconaldehyde and hydroquinone. J. Appl. Toxicol. 1991; 11: 443–446
- Hamdaoui M. H., Chahed A., Ellouze-Chabchoub S., Marouani N., Ben Abid Z., Hedhili A. Effect of green tea decoction on long-term iron, zinc, and selenium status of rats. Ann. Nutr. Metab. 2005; 49: 118–124
- Hirano-Ohmori R., Takahashi R., Momiyama Y., Taniguchi H., Yonemura A., Tamai S., Umegaki K., Nakamura H., Kondo K., Ohsuzu F. Green tea consumption and serum malondialdehyde-modified LDL concentrations in healthy subjects. J. Am. Coll. Nutr. 2005; 24: 342–346
- Horita M., Wang D. H., Tsutsui K., Sano K., Masuoka N., Kira S. Involvement of oxidative stress in hydroquinone-induced cytotoxicity in catalase-deficient Escherichia coli mutants. J. Cell Biochem. 1997; 27: 59–67
- Imai K., Nakachi K. Cross sectional study of effects of drinking green tea on cardiovascular and liver diseases. Br. Med. J. 1995; 310: 693–696
- Katiyar S. K., Bergamo B. M., Vyalil P. K., Elmets C. A. J. Green tea polyphenols: DNA photodamage and photoimmunology. Photochem. Photobiol. B 2001; 65: 109–114
- Kolachana P., Subrahmanyam V. V., Meyer K. B., Zhang L., Smith M. T. Benzene and its phenolic metabolites produce oxidative DNA damage in HL60 cells in vitro and in the bone marrow in vivo. Cancer Res. 1993; 53: 1023–1026
- Kosower N. S., Kosower E. M. The glutathione status of cells. Intl. Rev. Cytol. 1978; 54: 109–157
- Kunsch C., Medford R. M. Oxidative stress as a regulator of gene expression in the vasculature. Circ. Res. 1999; 85: 753–766
- Lovern M. R., Turner M. J., Meyer M., Kedderis G. L., Bechtold W. E., Schlosser P. M. Identification of benzene oxide as a product of benzene metabolism by mouse, rat, and human liver microsomes. Carcinogenesis 1997; 18: 1695–1700
- Mizukami Y., Sawai Y., Yamaguchi Y. Simultaneous analysis of catechins, gallic acid, strictinin, and purine alkaloids in green tea by using catechol as an internal standard. J. Agric. Food Chem. 2007; 55: 4957–4964
- Mulder T. P., Rietveld A. G., van Amelsvoort J. M. Consumption of both black tea and green tea results in an increase in the excretion of hippuric acid into urine. Am. J. Clin. Nutr. 2005; 81: 256S–260S
- Negishi H., Xu J. W., Ikeda K., Njelekela M., Nara Y., Yamori Y. Black and green tea polyphenols attenuate blood pressure increases in stroke-prone spontaneously hyper-tensive rats. J. Nutr. 2004; 134: 38–42
- Paglia D. E., Valentine W. N. Studies on the quantitative and qualitative characterization of erythrocyte glutathione peroxidase. J. Lab. Clin. Med. 1967; 70: 158–169
- Pan H. Z., Na L. X., Tao L. DNA damage and changes of antioxidative enzymes in chronic benzene poisoning mice. Chinese J. Ind. Hyg. Occup. Dis. 2003; 21: 423–425
- Ruiz-Ramos R, Cebrian M. E., Garrido E. Benzoquinone activates ERK/MAPK signaling pathway via ROS production in HL-60 cells. Toxicology 2005; 209: 279–287
- Sabu M. C., Smitha K., Kuttan R. Anti-diabetic activity of green tea polyphenols and their role in reducing oxidative stress in experimental diabetes. J. Ethnopharmacol. 2002; 83: 109–116
- Seaton M. J., Schlosser P. M., Bond J. A., Medinsky M. A. Benzene metabolism by human liver microsomes in relation to cytochrome P450 2E1 activity. Carcinogenesis 1994; 15: 1799–1806
- Seaton M. J., Schlosser P., Medinsky M. A. In vitro conjugation of benzene metabolites by human liver: Potential influence of inter-individual variability on benzene toxicity. Carcinogenesis 1995; 16: 1519–1527
- Shankar S., Ganapathy S., Srivastava R. K. Green tea polyphenols: Biology and therapeutic implications in cancer. Front. Biosci. 2007; 12: 4881–4899
- Shen Y., Shen H. M., Shi C. Y., Ong C. N. Benzene metabolites enhance reactive oxygen species generation in HL60 human leukemia cells. Human Exp. Toxicol. 1996; 15: 422–42
- Shvedova A. A., Kommineni C., Jeffries B. A., Vincent Castranova V., Tyurina Y. Y., Tyurin V. A., Elena A., Serbinova E. A., Fabisiak J. P., Kagan V. E. Redox cycling of phenol induces oxidative stress in human epidermal keratinocytes. J. Invest. Dermatol. 2000; 114: 354–364
- Sliva M. D., Gaspar J., Silva I., Leao D., Rueff J. Mechanisms of induction of chromosomal aberrations by hydroquinone in V79 cells. Mutagenesis 2003; 18: 491–496
- Smith M. T., Yager J. W., Steinmetz K. Peroxidase-dependent metabolism of benzene's phenolic metabolites and its potential role in benzene toxicity and carcinogenicity. Environ. Health Perspect. 1989; 82: 23–29
- Snyder R., Hedli C. C. An overview of benzene metabolism. Environ. Health Perspect. 1996; 104(S6)1165–1171
- SPSS/PC-V11.0. Base manual. SPSS, Chicago, IL 2001
- Tipoe G. L., Leung T. M., Hung M. W., Fung M. L. Green tea polyphenols as an anti-oxidant and anti-inflammatory agent for cardiovascular protection. Cardiovasc. Hematol. Disorders Drug Targets 2007; 7: 135–144
- Ugurnal B., Uysal M., Toker G. Effect of benzene treatment on hepatic lipid peroxidation and antioxidant enzyme activities in mice. Biochem. Arch. 1995; 11: 155–160
- Verma A. K., Saxena R. K., Mahapatra J. K., Prashant S., Swain S. C. Biological monitoring of benzol workers by urine phenol estimation. Indian J. Occup. Environ. Med. 1999; 3: 119–121
- Verma Y., Rana S. V. S. Biological monitoring of exposure to benzene in petrol pump workers and dry cleaners. Ind. Health 2001; 39: 330–333
- Fundamental Biochemistry. 1st Edition, D. Voet, J. G. Voet. John Wiley & Sons, New York 1999; 422–423
- Waidyanatha S., Rothman N., Fustinoni S., Smith M. T., Hayes R. B., Bechtold W., Dosemeci M., Guilan L., Yin S., Rappaport S. M. Urinary benzene as a biomarker of exposure among occupationally exposed and unexposed subjects. Carcinogenesis 2001; 22: 279–286
- Wallace L. A. The exposure of the general population to benzene. Environ. Health Perspect. 1989; 82: 165–169
- Xie M., Von Bohlen A., Klockenkamper R., Jian X., Gunther K. Multielement analysis of Chinese tea (Camellia sinensis sinensis) by total-reflection X-ray fluorescence. Z. Lebens. Unters. Forsch 1998; 207: 31–38
- Zuo Y., Chen H., Deng Y. Simultaneous determination of catechins, caffeine and gallic acids in green, Oolong, black, and pu-erh teas using HPLC with a photodiode array detector. Talanta 2002; 57: 307–316