Abstract
The interaction of poly-dispersed acid-functionalized single-walled carbon nanotubes (AF-SWCNT) with NK cells undergoing activation was examined. Exposure to AF-SWCNT during NK activation in vitro by interleukin (IL)-2, and in vivo by Poly(I:C) significantly lowered cytotoxic activity generated against YAC-1 tumor cells. Recoveries of spleen NK1.1+ cells as well as the activated subset of NK cells (NK1.1+CD69+ cells) were significantly reduced by the AF-SWCNT exposure. The proportion of apoptotic NK cells (NK1.1+ phosphatidylserine+) in the spleen cell preparations activated in vitro was also significantly elevated. Expression levels of CD107a [for assessing NK cell degranulation] as well as of FasL marker [mediating non-secretory pathway of NK cell killing] were significantly lower in cells exposed to AF-SWCNT during the activation phase. Intracellular levels of interferon (IFN)-γ and tumor necrosis factor (TNF)-α in the cells were also significantly reduced. Fluorescent AF-SWCNT (FAF-SWCNT) were internalized by the NK cells and uptake was significantly greater in activated cells. Confocal microscopy indicated the internalized FAF-SWCNT were localized to the cytoplasm of the NK cells. These results indicated that AF-SWCNT were internalized by NK cells and caused a general down-regulation of a variety of parameters associated with NK cell cytotoxicity and other cellular functions.
Introduction
Carbon nanotubes (CNT), by virtue of their high aspect ratio, unique mechanical, electrical, anisotropic and spectroscopic properties have tremendous potential in the field of nanomedicine (Valentini et al. Citation2013; Zhang et al. Citation2014). CNT, which resemble rolled-up tubes of graphite sheets of sp2-hybridized carbon atoms, exist in bundled forms and do not solubilize in aqueous solution. Acid-functionalized SWCNT (AF-SWCNT) are de-bundled, disperse easily in aqueous solution, and display an increased capacity to load ligands for therapeutic purposes (Kostarelos et al. Citation2007; Saxena et al. Citation2007). The CNT represent a new form of material that is finding a variety of commercial applications and is therefore being manufactured in huge quantities. In turn, the increased production of CNT, which can become airborne due to their low density and small size, has enhanced the risk for environmental/occupational exposure by humans (Cui et al. Citation2010).
Many studies in literature have focused on the effect of CNT on different components of the immune system (Andersen et al. Citation2012; Dumortier Citation2013; Orecchioni et al. Citation2014). CNTs were shown not to impair dendritic cell function (Wang et al. Citation2009) or ex vivo functionality of human T-cells (Delogu et al. Citation2012). In contrast, studies by Mitchell et al. (Citation2009), Laverny et al. (Citation2013) and Rizvi et al. (Citation2014) showed that CNT indirectly suppressed T- and B-cell responses in vivo and in vitro by inhibiting the process of antigen presentation. SWCNT induced an immunosuppressive effect leading to decreased bacterial clearance (Shvedova et al. Citation2008; Walling et al. Citation2013). Treatment with AF-SWCNT suppressed the capacity of primary lung epithelial cells to present BCG antigen to sensitized T-helper cells (Kumari et al. Citation2012) and induced anemia in mice (Bhardwaj & Saxena Citation2015). Intratracheal administration of AF-SWCNT induced cardiotoxic effects indicating that pulmonary exposure to AF-SWCNT may have systemic effects also (Tong et al. Citation2009). Our group previously studied the effect of CNT on generation of allo-immune cytotoxic T-lymphocytes (CTL) in mice and demonstrated that AF-SWCNT suppressed CTL responses via decreased T-cell activation and increased apoptosis (Alam et al. Citation2013).
NK cells constitute an important component of innate immune responses and play a vital role as a first line of defense against tumors and virus-infected cells (Caligiuri Citation2008). NK cells spontaneously kill a variety of tumor target cells through mechanisms that, unlike for cytotoxic T-cells, require neither a prior sensitization with target cells nor presentation of antigen in association with MHC-I complexes (Cantor et al. Citation1979; Saxena Citation1997). Cytotoxic activity of NK cells may be boosted in vitro and in vivo by cytokines like interleukin (IL)-2 and interferon (IFN)-γ (Saxena et al. Citation1981, Citation1983, Citation1984). Currently, little is known about the interaction of NK cells with CNT.
The present study undertook a detailed investigation into the effect of AF-SWCNT on NK cell activation in vitro and in vivo. The doses of carbon nanotubes used for in vitro as well as in vivo studies were well in the ranges of what other investigations have used to assess biological effects of carbon nanotubes (Oberdörster et al. Citation2015). In part, it was hoped these studies would ascertain whether AF-SWCNT induced apoptosis in NK cells, down-regulated NK activation processes, and/or imparted significant inhibitory effects on mechanisms utilized by NK cells to kill targets through secretory and non-secretory pathways. This study represented as first to detail interactions between NK cells and CNT.
Materials and methods
Animals
Inbred C57BL/6 mice (male, 8-12-wk-of-age, 20-25 g) were bred and maintained in the animal house facility at Jawaharlal Nehru University (JNU, New Delhi, India), or obtained from the National Institute of Nutrition (Hyderabad, India). All animals were housed in positive-pressure air conditioned units (25 °C, 50% relative humidity) and kept on a 12 h light/dark cycle. Filtered water and standard mouse chow were provided ab libitum. All experimental protocols were conducted strictly in compliance with the guidelines notified by the Committee for the Purpose of Control and Supervision on Experiments on Animals (CPCSEA), Government of India (CPCSEA guidelines www.envfor.nic.in/divisions/awd/cpcsea_laboratory.pdf). The study was duly approved by the JNU Institutional Animal Ethics Committee (IAEC Approved Project Code: 5/2010).
Cells and reagents
YAC-1, a NK sensitive murine lymphoma cell line, was maintained in complete RPMI 1640 medium containing 2 mM L-glutamine, 25 mM HEPES buffer (pH 7.2) and supplemented with 20 μM 2-mercaptaethanol, 120 μg gentamycin/ml (Sigma, New Delhi, India), and 10% fetal bovine serum (FBS; Hyclone, South Logan, UT). All monoclonal anitibodies (mAb) against mouse NK1.1 (FITC- or APC-conjugated), as well as anti-mouse CD107a (FITC-conjugated), CD16/32, CD69 (FITC-conjugated), Annexin V (FITC-conjugated), FasL (FITC-conjugated), IFNγ (FITC-conjugated) and TNFα (FITC-conjugated) were obtained from BD Pharmingen (San Diego, CA). Poly(I:C), bredfeldin, monensin and saponin were obtained from Sigma. Poly(I:C) solution was prepared in phosphate-buffered saline (PBS, pH 7.4). Recombinant mouse IL-2 was obtained from Peprotech (Rocky Hill, NJ). For the chromium release cytotoxicity assays (CRA), target cells were labeled with sodium chromate (51Cr, as Na2CrO4) obtained from the Board of Radiation and Isotope Technology (BARC, Mumbai, India).
SWCNT were purchased from Sigma (Cat. #636797, amorphous carbon <3%), though similar results were obtained from SWCNT procured from another source (purified HIPCO SWCNT, ash <15%, from Carbon Nanotubes Inc., Houston, TX). Acid-functionalized SWCNT (AF-SWCNT) were prepared by the method described by Saxena et al. (Citation2007). In brief, 20 mg SWCNT were suspended in 20 ml of 1:1 mixture of concentrated HNO3:H2SO4 at 20 [± 2] psi for 3 min resulting in an internal temperature of 138-150 °C. The suspensions were then cooled and dialyzed against water to remove acids. The resulting dilute suspension of AF-SWCNT was then lyophilized, weighed and known amounts re-suspended in water. Detailed characterization of the AF-SWCNT including elemental analysis, size/charge distribution, BET surface area and electron microscopic changes have been reported elsewhere (Tong et al. Citation2009; Sachar & Saxena Citation2011). In summary, particle size distributions of the AF-SWCNT aqueous suspensions obtained from a Zeta-sizer instrument (Malvern, PA) showed that 95% of the particles were in the range of 22-138 nm. The mean zeta potential of the AF-SWCNT was -57.2 mV and BET surface area was reduced one-third compared with that of the pristine SWCNT. The basic tubular structure of the AF-SWCNT was intact, though the sidewalls of nanotubes appeared roughened. In general, all changes were consistent with mild sulfonation/carboxylation of the nanotube sidewalls. Control and acid-functionalized CNT suspensions were sonicated for 2 min in ice using a probe sonicator (Branson sonifier, VWR Scientific, Bristol, CT) prior to use in the in vivo and in vitro studies.
Attachment of fluorescent probes to AF-SWCNT
Fluorescent probe (Alexafluor [AF]-633, Molecular Probes, Carlsbad, CA) was covalently attached to -COOH groups on AF-SWCNT as described in Sachar and Saxena (Citation2011). In brief, AF-SWCNT were suspended in water and treated with 1-ethyl 3-(3-dimethyl aminopropyl) carbodiimide (EDAC) and N-hydroxysuccinimide (NHS) to generate a succinimidyl-intermediate. The mixture was continually shaken for 2 h and then dialyzed extensively to remove excess NHS, EDAC and urea by-product. The AF-SWCNT thus activated were then incubated with AF633 hydrazide in the dark with continuous mixing followed by dialysis to remove free dye.
Generation of NK response in vitro and in vivo
For the in vitro assays, spleen cells from naive C57BL/6 mice were obtained using standard protocols (Saxena et al. Citation1984). Any contaminating erythrocytes in the suspensions were lysed using Tris-ammonium chloride buffer (ACK lysis buffer, pH 7.4). The final clean preparation of splenocytes, now devoid of erythrocytes, were then counted in a hemocytometer and then placed into 24-well plates (5 × 106 cell/well; 1 ml/well). The cells were then cultured (in quadruplicate) for 72 h in the presence of 500 U IL-2/ml in complete medium, with or without AF-SWCNT (10, 25 or 50 μg/ml).
For the in vivo assays, C57BL/6 mice (in groups of six) were given Poly(I:C) (200 μg in 0.1 ml PBS) by intraperitoneal injection. At the same time, AF-SWCNT (10, 50 or 100 μg/mouse in 0.1 ml PBS or 0.1 ml PBS alone for control mice) were administered intravenously and spleen cells then isolated 3 days later. This range of AF-SWCNT doses was selected based upon our previous studies (Sachar & Saxena Citation2011; Alam et al. Citation2013).
In the assay of NK function (cytotoxicity) itself, control and activated spleen cells from above were washed, counted and then used as effector cells (E). Specifically, cytotoxic activity was determined using YAC-1 target cells (T) in a 4-hour [51Cr] release assay as described in Saxena et al. (Citation1982). Control wells contained target cells alone to ascertain spontaneous release of Cr or with 0.2% Triton X-100 to determine maximum Cr release. Culture supernatants from all wells were collected to determine released [51Cr] using a gamma counter (PerkinElmer, Boston, MA). Lytic units (LU)/107 effector cells were calculated from the E:T ratio vs. percent lysis plots as described before (Cerottini et al. Citation1974, Bryant et al. Citation1992). In brief, E:T ratios corresponding to a 20% target lysis were determined from E:T ratio vs. percent lysis plots and the number of lymphocytes corresponding to this E:T ratio was considered as 1 LU.
Immunophenotyping and apoptosis measurement
In vitro cultured spleen cells or cells freshly isolated from spleens were stained with various mAb and analyzed by flow cytometry. Lymphocytes gated in a forward/side-scatter plots were further examined for each specific stain. Prior to staining, single cell suspensions were incubated with anti-CD16/32 antibody (Fc block, 1 μg/106 cells in 50 μl PBS/2% FBS solution) for 10 min followed by treatment with a manufacturer recommended level of a given mAb in the dark for 20 min at 4 °C. Parallel sets of cells were stained with isotype control antibodies to help in setting gates. To assess apoptosis, parallel sets of the cells were double stained with anti-mouse NK1.1-APC (20 μl/ml) and Annexin V-FITC (20 μl/ml) and then analyzed. In all cases, stained cells were washed with PBS containing 0.5% FBS and 0.02% NaN3, then fixed with 2% paraformaldehyde, and analyzed on a FACS-Calibur machine (BD Biosciences, San Jose, CA). A minimum of 10 000 events were analyzed using Cellquest software (BD Biosciences).
NK cell degranulation assay
Degranulation of NK cells in the presence of YAC-1 target cells was assessed by staining for CD107a as described previously (Alter et al. Citation2004; Zhao et al. Citation2011). In brief, spleen cells (2 × 106/ml) were cultured for 72 h in 24-well plates (1 ml/well) with IL-2 (500 U/ml) in the presence or absence of 50 μg AF-SWCNT/ml. Cells were then washed, re-suspended in complete media, and stimulated with YAC-1 cells (E:T ratio =5:1). CD107a mAb (20 μl/ml) was added to the cells and incubation continued for 1 h at 37 °C. The cells were further incubated with monensin (6 μg/ml) for an added 3 h at 37 °C and counter-stained with anti-mouse NK1.1 mAb (20 μl/ml) for 20 min at 4 °C. The proportion of NK1.1+ cells with CD107a expression was then assessed using the flow cytometer.
Intracellular cytokine and FasL staining of NK cells by flow cytometry
Intracellular levels of FasL, IFNγ and TNFα were assessed by flow cytometry as in Saxena et al. (Citation2002) and Reefman et al. (Citation2010). Here, cells (2 × 106) were incubated for 5 h at 37 °C in 24-well plates in the presence of 2 × 106 YAC-1 target cells. Brefeldin A (1 μg/ml) was added to assay wells 1 h after the start of the incubation. At the end of the incubation period, all cells were stained with NK1.1 mAb (20 μl/ml), washed, fixed in 2% paraformaldehyde, and permeabilized with 0.2% saponin in PBS for the intracellular staining for cytokines using fluorescence-labeled antibodies to IFNγ, TNFα or FasL (1.5 μg in 50 μl; manufacturer recommended levels) for 30 min at 4°C. All samples were then analyzed by flow cytometry as outlined above.
Interaction of AF-SWCNT with NK cell and confocal microscopy
To study the interactions of AF-SWCNT with the cells, splenocytes were incubated for 72 h with fluorescent AF-SWCNT (FAF-SWCNT; 2 μg/ml). Unbound/loosely bound particles were removed by washing the cells twice with PBS after the incubation period. The cells were then counter-stained with anti-mouse NK1.1 mAb and analyzed on the flow cytometer (see above).
Confocal microscopy was also used in these analyses as described earlier (Alam et al. Citation2013). In brief, IL-2 activated spleen cells were incubated for 24 h in complete medium with FAF-SWCNT, then counter stained with NK1.1 mAb, and NK1.1+ cells were isolated using a fluorescence activated cell sorter (Aria, BD Biosciences). The purified NK cells were then cultured on poly-L-lysine-coated coverslips for 3 h at 37 °C. Thereafter, the cells were fixed with paraformaldehyde, incubated with DAPI (0.2 mg/ml) for 20 min on ice, and then examined in a blinded manner using a Nikon A1R fluorescence confocal microscope (Nikon, Tokyo, Japan). A total of 10 cells/4 slides/sample were examined in five non-adjacent microscopic fields - each along the horizontal and vertical axis of the slide; results were viewed at different depths (Z: from -3.24 to 4.26 μm).
Statistical analysis
Statistical analysis using a two-way analysis of variance (ANOVA) and a Student’s t-test were done using Sigmaplot and Sigmastat software (Systat Software Inc, San Jose, CA). A p value <0.05 was considered significant.
Results
Effects of AF-SWCNT on IL-2 activated NK cell activity in vitro and in vivo
Effects of AF-SWCNT on basal as well as IL-2-induced NK responses were examined. For this, spleen cells were cultured for 72 h with or without IL-2 in the presence or absence of three different concentrations of AF-SWCNT. The cytotoxic activity of the cultured cells was then assessed against YAC-1 cells in a 4-hour chromium release assay (CRA). Results in show AF-SWCNT caused significant inhibition of IL-2-activated NK cell cytotoxicity at 25 and 50 μg/ml (p < 0.05). Inhibition at the lower concentration (10 μg AF-SWCNT/ml) was not significant. Lytic units (LU)/107 cells, a quantitative measure of total cytotoxicity activity generated in IL-2 activated spleen cell cultures in the presence or absence of AF-SWCNT, were calculated from individual cytotoxicity curves in . A maximum suppression of 44% was observed with 50 μg AF-SWCNT/ml (, inset). Addition of pristine SWCNT without acid functionalization did induce significant inhibition of IL-2 activated NK cytotoxicity (results not shown). Addition of AF-SWCNT during the CRA also had no significant effect on target cell killing by IL-2 activated NK cells, indicating the AF-SWCNT did not interfere directly in effector-target interactions (Supplementary data; Figure S1).
Figure 1. Effect of AF-SWCNT on generation of NK cell responses in vitro and in vivo. NK cells were activated in vitro by culturing spleen cells with IL-2/ml in absence or presence of AF-SWCNT/ml as described in the “Materials and methods” section. Cells were washed and their cytotoxic activity against YAC target cells were determined at E:T ratios of 100, 50, 25 and 12.5 in a 4-h chromium release assay. Values shown from a representative experiment are means [±SEM] of percent target lysis at different E:T ratios for NK cells activated with IL-2 in the absence (O = 0 μg/ml) or presence (▾ =10 μg, Δ = 25 μg, ▪ = 50 μg/ml) of AF-SWCNT [main Panel A]. Lytic units of cytotoxic activity were calculated for NK cells activated with IL-2 in presence of various concentrations of AF-SWCNT. Inset figure in Panel A shows dose-related decline in lytic units of NK cell activity generated in presence of different concentrations of AF-SWCNT. *p < 0.05 vs. AF-SWCNT untreated (ANOVA). Similar results for in vivo activation of NK cells by Poly(I:C) are shown in Panel B. Different groups of C57BL/6 mice were administered Poly(I:C) along with AF-SWCNT as described in “Materials and methods” section. In main Panel B, % target lysis at different E:T ratios of cells from Poly(I:C)-treated mice injected without (O = 0 μg/mouse) or with (▾ =10 μg, Δ = 50 μg, ▪ = 100 μg/mouse) AF-SWCNT are shown. Inset figure in Panel B reflects a dose-related inhibition of NK activation by AF-SWCNT. *p < 0.05, **p < 0.01 vs. AF-SWCNT untreated (ANOVA).
![Figure 1. Effect of AF-SWCNT on generation of NK cell responses in vitro and in vivo. NK cells were activated in vitro by culturing spleen cells with IL-2/ml in absence or presence of AF-SWCNT/ml as described in the “Materials and methods” section. Cells were washed and their cytotoxic activity against YAC target cells were determined at E:T ratios of 100, 50, 25 and 12.5 in a 4-h chromium release assay. Values shown from a representative experiment are means [±SEM] of percent target lysis at different E:T ratios for NK cells activated with IL-2 in the absence (O = 0 μg/ml) or presence (▾ =10 μg, Δ = 25 μg, ▪ = 50 μg/ml) of AF-SWCNT [main Panel A]. Lytic units of cytotoxic activity were calculated for NK cells activated with IL-2 in presence of various concentrations of AF-SWCNT. Inset figure in Panel A shows dose-related decline in lytic units of NK cell activity generated in presence of different concentrations of AF-SWCNT. *p < 0.05 vs. AF-SWCNT untreated (ANOVA). Similar results for in vivo activation of NK cells by Poly(I:C) are shown in Panel B. Different groups of C57BL/6 mice were administered Poly(I:C) along with AF-SWCNT as described in “Materials and methods” section. In main Panel B, % target lysis at different E:T ratios of cells from Poly(I:C)-treated mice injected without (O = 0 μg/mouse) or with (▾ =10 μg, Δ = 50 μg, ▪ = 100 μg/mouse) AF-SWCNT are shown. Inset figure in Panel B reflects a dose-related inhibition of NK activation by AF-SWCNT. *p < 0.05, **p < 0.01 vs. AF-SWCNT untreated (ANOVA).](/cms/asset/9fc436db-9e78-4adb-bd48-f18cdd59a15c/iimt_a_1191562_f0001_b.jpg)
Effects of AF-SWCNT were also examined on NK cell activation ex vivo. Mice were administered poly I:C intraperitoneally (IP) with simultaneous intravenous (IV) administration of different doses of AF-SWCNT (25, 50 or 100 μg/mouse). After 72 h, their spleen cells were harvested and anti-YAC-1 cytotoxic activity assessed in a 4 h CRA. Results in show that treatment with 50 or 100 μg AF-SWCNT resulted in significant (p < 0.01) decreases in ex vivo spleen cell NK activity. Cytotoxic activity of spleen cell preparations expressed in terms of LU/107 cells indicated suppressions of 36% (p < 0.05) and 47% (p < 0.01) in NK cell cytotoxicity with the 50 and 100 μg doses, respectively. Inhibition from the lowest dose of AF-SWCNT (10 μg) was not significant (). Effects of pristine SWCNT without acid functionalization were also examined, but found not to be significant (data not shown). These results indicated a single IV dose of AF-SWCNT at ≥50 μg/mouse could suppress Poly(I:C)-induced NK cell activation in situ. This study found no significant effect of administration of AF-SWCNT on total cells recovered from the spleen, indicating that even a single dose of 100 μg AF-SWCNT did not have a generalized toxic effect on the spleen.
Effect of AF-SWCNT on NK cell activation and apoptosis
Suppression of IL-2 induced NK cell cytotoxicity by AF-SWCNT could be due to a possible interference with the NK cell proliferation/activation process, or a loss of NK cells due to AF-SWCNT toxicity, or both. Actual enumeration of NK1.1+ cells in the cultures here indicated that AF-SWCNT reduced the proportion of NK cells from a mean of 30.6% in control IL-2 activated spleen cell cultures to 25.3% when AF-SWCNT was present (17.3% decline, p < 0.05, ). Similarly, NK cells among spleen cells activated in vivo with Poly(I:C) indicated a comparable decline in the proportion of NK1.1+ cells induced by AF-SWCNT.
Table 1. Suppression of NK cell generation and activation in response to IL-2 (in vitro) and Poly(I:C) (in vivo) by AF-SWCNT.
NK cell activation is preceded by CD69 up-regulation (Craston et al. Citation1997). The proportion of CD69+ cells within the NK1.1+ cell population was enumerated in spleen cells activated both in vitro and in vivo in the absence/presence of AF-SWCNT. Data in show that 53% of NK1.1+ cells in IL-2-activated spleen cell cultures expressed CD69 marker. Addition of AF-SWCNT along with IL-2 resulted in a significant decline in CD69-expressing NK cells (a 22% decline; p < 0.05). A significant decline in the proportion of CD69+ cells among NK cells was also seen when NK activation was carried out in vivo with Poly(I:C).
Apoptotic responses among NK cells was examined by staining isolated cells with Annexin V. Results in show that while only 11% of the NK1.1+ cells in IL-2-activated splenocyte cultures stained for Annexin V, co-treatment with AF-SWCNT resulted in 22% of the cells expressing the marker, indicating a significant increase in apoptosis among these NK cells. Similar experiments with in vivo-activated NK cells did not show a difference in apoptotic NK cell levels among splenocytes obtained from mice treated with Poly(I:C), with or without AF-SWCNT. This could be due to the fact that internal homeostatic mechanisms ensure that dead/dying cells do not accumulate in a host. Recognition of externalized phosphatidylserine (PS) on apoptotic cells by receptors on phagocytes results in rapid removal of the cells (Hochreiter-Hufford & Ravichandran Citation2013).
Effect of AF-SWCNT on NK1.1+ cell effector functions in vitro
NK cell lytic mechanisms include release of perforin and granzyme-containing granules (Mace et al. Citation2014). Degranulation of NK cells is associated with a significant up-regulation of expression of Lysosomal Associated Membrane Protein-I (LAMP-I or CD107a) on the surface of NK cells, an event that correlates with both secretion of cytokines and lytic granules (Alter et al. Citation2004; Aktas et al. Citation2009). To test the possible effect of AF-SWCNT on NK cell degranulation, spleen cells activated with IL-2- with or without AF-SWCNT were further co-cultured with YAC-1 targets and NK degranulation was then assessed by flow cytometry (i.e. by evaluating CD107a expression on NK1.1+ cells). Among IL-2-activated splenocytes co-cultured with YAC-1 cells, 57% of the NK1.1+ cells expressed CD107a (, left histograms). Addition of AF-SWCNT to the IL-2-activated culture resulted in down-regulation of CD107a expression (to 44%) on NK1.1+ cells (a 22% decline; p < 0.01). These results suggested to us that AF-SWCNT induced inhibition of degranulation of activated NK cells and this may be a contributing factor in the observed reduced cytotoxic activity of AF-SWCNT-treated NK cells.
Figure 2. Effect of AF-SWCNT on IL-2-activated NK cell sub-populations in vitro. Mouse spleen cells (2 × 106/ml) were cultured with 500 U IL-2/ml in absence (□) or presence (▪) of 50 μg AF-SWCNT/ml. After 72 h, cells were washed and double-stained with a combination of antibodies against NK1.1 and CD107a markers or NK1.1 and FasL markers. Values shown are means ± SEM from four independent experiments. **p < 0.01 vs. AF-SWCNT untreated (Student’s t-test).
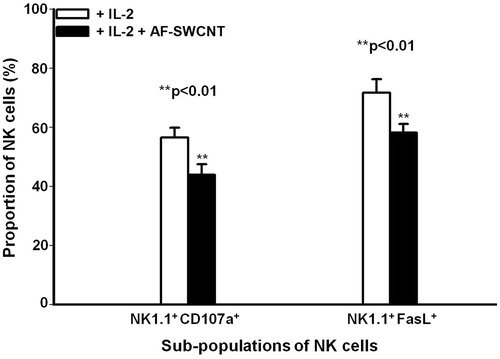
An alternative mechanism of NK cell-mediated killing involves FasL expression on NK cells; this ligand engages with Fas expressed on target cells and induces a lytic response (Bossi & Griffiths Citation1999). Effect of AF-SWCNT on expression of FasL in IL-2-activated NK1.1+ cells was examined. Results in (right histograms) show that 72% of the activated NK1.1+ cells expressed FasL that was significantly inhibited (20%; p < 0.01) by AF-SWCNT. These results showed that AF-SWCNT could significantly down-regulate both secretory as well as non-secretory mechanisms of NK cell killing.
Effect of AF-SWCNT on cytokine responses of activated NK cells
Activated NK cells secrete IFNγ and TNFα that each has been shown to have a role in cytotoxicity and other effector functions of NK cells (Reefman et al. Citation2010). The effect of AF-SWCNT on intracellular levels of IFNγ and TNFα in NK cells obtained from mice treated with Poly(I:C) with or without AF-SWCNT was examined here. Spleen cells were co-cultured in the presence/absence of YAC-1 targets and intracellular levels of IFNγ and TNFα on NK1.1+ cells were then assessed by flow cytometry. The results in illustrate that spleen cells obtained from control mice expressed low levels of IFNγ and TNFα in the absence or presence of the YAC-1 challenge in vitro. In mice administered Poly(I:C), nearly 21.3% of the splenic cells expressed NK1.1+; of these, nearly 14% and 11% cells expressed IFNγ and TNFα, respectively. Upon in vitro YAC-1 challenge, spleen cells obtained from Poly(I:C)-treated mice showed an increased level of IFNγ and TNFα, up to 41 and 20%, respectively, in their NK1.1+ cells. Poly(I:C)-treated mice co-treated with AF-SWCNT had cells that displayed a significant decline in levels of IFNγ and TNFα, by 32% (p < 0.01) and 44% (p < 0.01), respectively ().
Table 2. Intracellular expression of IFNγ and TNFα by NK cells derived from mice treated with poly I:C with or without AF-SWCNT.
Interaction of AF-SWCNT with NK cells in vitro and intracellular distribution of FAF-SWCNT internalized by IL-2 activated NK cells
Uptake of AF-SWCNT by NK1.1+ cells was examined using FAF-SWCNT. Results in show that in control spleen cells cultured with FAF-SWCNT for 24 h, nearly 53% of the NK1.1+ cells stained with FAF-SWCNT; this proportion went up to 72% in IL-2-activated NK1.1+ cells (). Results in depict the kinetics of binding/uptake of FAF-SWCNT by the control and IL-2-activated NK cells. These results clearly indicate that AF-SWCNT binding/uptake was substantially greater in activated as compared to control NK cells. Internalization of AF-SWCNT by NK cells was also examined by confocal microscopy. Results in show that the fluorescent nanotubes were present in cytoplasm but not in the nuclei of NK1.1+ cells. These results indicated AF-SWCNT were internalized by NK cells and localized essentially within the cytoplasmic spaces.
Figure 3. Uptake of FAF-SWCNT by control and IL-2-induced NK1.1 cells in culture. Mouse spleen cells (2 × 106/ml) were cultured in the absence (control) or presence of 500 U IL-2/ml along with 2 μg FAF-SWCNT/ml. After 12, 24, 48 or 72 h, cells were washed, stained with NK1.1 mAb and analyzed on a flow cytometer using NK 1.1 and FAF-SWCNTs [colors on X and Y axis, respectively]. Representative histograms for control and IL-2-activated cells at 24 h time-point are shown in Panels A and B, respectively. Values shown in each quadrangle indicate are the means [±SEM] fractions of cells in that quadrangle, from three replicate experiments. At all four timepoints, the percentages of NK 1.1+FAF-SWCNT+ cells as a percentage of all NK1.1+ cells were computed from flow histograms and plotted vs. time (Panel C). Values shown are mean [± SEM] percentages of NK cells positive for FAF-SWCNT at all timepoints, from three replicate experiments (Panel C). *p < 0.05, **p < 0.01 vs. control (Student’s t-test).
![Figure 3. Uptake of FAF-SWCNT by control and IL-2-induced NK1.1 cells in culture. Mouse spleen cells (2 × 106/ml) were cultured in the absence (control) or presence of 500 U IL-2/ml along with 2 μg FAF-SWCNT/ml. After 12, 24, 48 or 72 h, cells were washed, stained with NK1.1 mAb and analyzed on a flow cytometer using NK 1.1 and FAF-SWCNTs [colors on X and Y axis, respectively]. Representative histograms for control and IL-2-activated cells at 24 h time-point are shown in Panels A and B, respectively. Values shown in each quadrangle indicate are the means [±SEM] fractions of cells in that quadrangle, from three replicate experiments. At all four timepoints, the percentages of NK 1.1+FAF-SWCNT+ cells as a percentage of all NK1.1+ cells were computed from flow histograms and plotted vs. time (Panel C). Values shown are mean [± SEM] percentages of NK cells positive for FAF-SWCNT at all timepoints, from three replicate experiments (Panel C). *p < 0.05, **p < 0.01 vs. control (Student’s t-test).](/cms/asset/00363dbc-2aae-4599-986d-24d9cfefc3d5/iimt_a_1191562_f0003_b.jpg)
Figure 4. Intracellular distribution of FAF-SWCNT internalized by IL-2-activated NK1.1+ cells. Mouse spleen cells (2 × 106/ml) were cultured in presence of 500 U IL-2/ml and 2μg FAF-SWCNT/ml. After 24 h, cells were washed, stained with anti-mouse NK1.1 mAb, and NK1.1+ cells were sorted on a fluorescence activated cell sorter. Purified activated NK cells were then stained with DAPI and examined under a confocal microscope. Representative confocal images show combined DAPI/FAF-SWCNT fluorescent image at different depths (Z: -3.24 to 4.26 μm) (Magnification =60×).
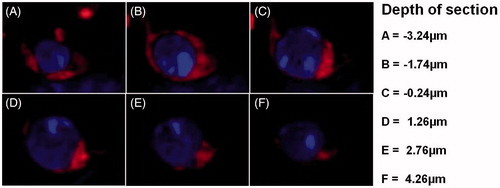
Discussion
NK cells were initially discovered as a population of large granular lymphocytes with the ability to kill tumor cells without requiring a prior sensitization (Caligiuri Citation2008). Unlike cytotoxic T-cells, NK cells are not MHC-restricted in their interaction with the target cells, and their ability to kill target cells is inversely correlated with the levels of MHC-I expression on target cells (Saxena Citation1996). The mechanism of cytotoxicity, however, appears to be common for CTL and NK cells since both may kill targets through secretion of granules containing perforin and granzymes (secretory pathway) as well as through FasL-Fas interactions (non-secretory pathway) (Kagi et al. Citation1996). We recently reported a significant down-regulation of the CTL response due to AF-SWCNT exposure (Alam et al. Citation2013). The current study constitutes the first report of a detailed investigation of NK cell interaction with such carbon nanotubes.
Initial experiments to determine the effects of SWCNT and AF-SWCNT on control and activated NK cells indicated that SWCNT did not have a significant effect on cytotoxicity (data not shown). SWCNT - being hydrophobic - exist in highly agglomerated bundled insoluble forms in aqueous solution and so interact poorly with biological systems. In contrast, AF-SWCNT are negatively charged by virtue of a presence of -COOH and -SO3H groups and remain stable in aqueous suspensions. Functionalized nanotubes are therefore amenable to attachment of drugs and proteins and are increasingly being assessed as drug delivery agents (Zhang et al. Citation2014). It is pertinent to ask the question whether functionalized carbon nanotubes when administered in vivo would adversely affect various physiological functions. Our studies have previously described significant inflammatory effects of AF-SWCNT in mouse lungs (Saxena et al. Citation2007), induction of anemia in mice (Sachar & Saxena Citation2011; Bhardwaj & Saxena Citation2015), and suppression of allo-immune cytotoxic T-cell responses in mice (Alam et al. Citation2013). Building on these earlier findings of toxicity, the current study focused on evaluating effects of AF-SWCNT on NK cell activation.
In normal mice, NK cells with NK1.1+ phenotype constitute ≈5% of all spleen cells (Salem et al. Citation2012). Another category of NKT cells express both NK1.1 as well as CD3 markers and comprises <1% of spleen cells (Becker et al. Citation2010). In the current study, NK1.1 marker was used to define NK cells and, as such, this population would include minor NKT cell populations as well. Here, IL-2 was used as a potent NK activating agent in vitro as described before (Saxena et al. Citation1984). While IL-2 also activates NK cells in vivo (Saxena et al. Citation1983), this requires constant infusion of IL-2. A relatively easier way to activate NK cells in vivo is by administering Poly(I:C) that exerts its effect by inducing secretion of interferon (Saxena et el. 1981; Miyake et al. Citation2009). For enumerating NK cells, NK1.1 mAb were used and the proportion of NK1.1+ cells was derived as a percentage of all gated lymphocytes (and not of all spleen leukocytes). Using this protocol, it was noted that NK1.1+ cells comprised ≈10% of the gated lymphocytes. In IL-2-activated cultures, the proportion of NK cells rose to ≈30%, whereas in spleen cells from Poly(I:C)-activated mice, the NK cell proportion rose to ≈20%. It should be noted that mature T- and B-cells present in spleen do not proliferate in response to IL-2. Basal T-cells acquire IL-2 receptors only upon activation with antigens; in spleens of control mice, such antigen-activated cells are not present in significant numbers. The increased NK cell proportion in the IL-2-activated spleen cell systems here thus reflected proliferation as well as activation of NK cells, as previously reported (Saxena et al. Citation1984).
While the current study demonstrated the inhibitory effects of AF-SWCNT on activated NK cells, it also used control non-activated NK cells for this purpose. However, the basal levels of NK cytotoxic activity were low and so the study only found marginal further decreases in activity caused by the AF-SWCNT. As a result, the remainder of the current study basically focused on studying effects of AF-SWCNT on NK cells undergoing activation.
AF-SWCNT induced inhibition of NK activation demonstrated in this study could theoretically result from a variety of factors including inhibition of NK cell proliferation, down-regulation of NK cell activation processes, and/or suppression of mechanisms in NK cells responsible for target lysis. Proportion of NK cells in IL-2-activated splenocyte cultures, as well in spleen cells derived from Poly(I:C)-treated mice, was significantly lower if AF-SWCNT was added to the cultures or administered to the Poly(I:C)-treated mice. Further, staining with Annexin V indicated that AF-SWCNT treatment induced apoptotic response in in vitro IL-2 activated NK cells. Unlike in the in vitro activation system, accumulation of PS+ NK cells was not seen in spleen cells derived from AF-SWCNT treated Poly(I:C)-treated mice. Lack of accumulation of PS+ NK cells in this case could be due to rapid clearance of such cells in vivo by spleen macrophages. Taken together, these observations point to a possibility of decreased NK cell proliferation as well induction of apoptotic cell death due to AF-SWCNT exposure. In addition, expression of CD69 (an early cell activation marker [Marzio et al. Citation1999]) was significantly lower in NK cells treated with AF-SWCNT, indicating the nanotubes also interfered with NK cell activation processes.
NK cells kill target cells through release of target directed granules that contain cytotoxic mediators perforin and granzymes (Kagi et al. Citation1996). Degranulation of NK cells was quantified by staining for membrane expression of Lysosomal Associated Membrane Protein (LAMP1 or CD107a). It was seen here that CD107a expression on IL-2 activated NK1.1+ cells was lowered among cells treated with AF-SWCNT, indicating that the degranulation process was impeded. Levels of FasL expression were also significantly lower in AF-SWCNT treated cells, suggesting non-secretory cytolytic mechanisms of NK cells may also be inhibited by the AF-SWCNT. In addition to target killing, NK cells release cytokines like IFNγ and TNFα that not only facilitate the cytotoxic reaction but also mediate a variety of other immunomodulatory functions (Guidotti & Chisari Citation2001). Intracellular expressions of IFNγ and TNFα were also significantly lower in IL-2 activated NK cells exposed to the AF-SWCNT.
Taken together, the results here indicated there was an overall down-regulation of NK cell system by AF-SWCNT. Confocal microscopy showed that IL-2 activated NK cells internalized fluorescent AF-SWCNT (FAF-SWCNT), but that the internalized particles were essentially localized in the cytoplasm and no significant uptake was noted in the nucleus. For now, the precise mechanism of uptake of FAF-SWCNT by NK cells is unclear. Cellular uptake of diesel exhaust particles (DEP, another category of nanoparticles that essentially are comprised of elemental carbon), by macrophages and lung epithelial cells was blocked by cytochalasin D (Saxena et al. Citation2008), an agent that reduces active transport by inhibiting the membrane actin-myosin system. Since internalization of AF-SWCNT has been demonstrated in erythrocytes that lack membrane phagocytic functions (Sachar & Saxena Citation2011), it is possible that an active internalization processes may not be the only mechanism for the internalization of AF-SWCNT.
It remains to be seen if AF-SWCNT are actively internalized by NK cells or if by virtue of their long needle-like structure, AF-SWCNT may pierce cell membranes and enter into the cyto-plasm. A general down-regulation of NK cytotoxic activity and other cellular functions may result from the presence of AF-SWCNT in the cytoplasm that may interfere with cellular machinery responsible for NK cell function. It is interesting to note that uptake of AF-SWCNT was significantly higher in IL-2-activated NK cells as compared to control NK cells. A likely implication of this observation is that NK cells in activated states may be more susceptible to toxicities inducible by AF-SWCNT. Further investigations are clearly warranted.
Funding information
This work was supported by a grant by the Department of Science and Technology, Government of India (Grants SR/S0/HS/0261/2012) to RKS. AA received Senior Research Fellowship from Indian Council of Medical Research, New Delhi.
Supplementary Figure 1
Download TIFF Image (256.2 KB)Disclosure statement
The authors declare no conflicts of interest. The authors alone are responsible for the content of the paper.
References
- Aktas E, Kucuksezer UC, Bilgic S, Erten G, Deniz G. 2009. Relationship between CD107a expression and cytotoxic activity. Cell Immunol. 254:149–154.
- Alam A, Sachar S, Puri N, Saxena RK. 2013. Interactions of poly-dispersed single-walled carbon nanotubes with T-cells resulting in downregulation of allogeneic CTL responses in vitro and in vivo. Nanotoxicology. 7:1351–1360.
- Alter G, Malenfant JM, Altfeld M. 2004. CD107a as a functional marker for the identification of natural killer cell activity. J Immunol Meth. 294:15–22.
- Andersen AJ, Wibroe PP, Moghimi SM. 2012. Perspectives on carbon nanotube-mediated adverse immune effects. Adv Drug Deliv Rev. 64:1700–1705.
- Becker AM, Blevins JS, Tomson FL, Eitson JL, Medeiros JJ, Yarovinsky F, Norgard MV, van Oers NS. 2010. Invariant NKT cell development requires a full complement of functional CD3 zeta immunoreceptor tyrosine-based activation motifs. J Immunol. 184:6822–6832.
- Bhardwaj N, Saxena RK. 2015. Selective loss of younger erythrocytes from blood circulation and changes in erythropoietic patterns in bone marrow and spleen in mouse anemia induced by poly-dispersed single wall carbon nanotubes. Nanotoxicology. 9:1032–1040.
- Bossi G, Griffiths GM. 1999. Degranulation plays an essential part in regulating cell surface expression of Fas ligand in T-cells and natural killer cells. Nat Med. 5:90–96.
- Bryant J, Day R, Whiteside TL, Herberman RB. 1992. Calculation of lytic units for the expression of cell-mediated cytotoxicity. J Immunol Meth. 146:91–103.
- Caligiuri MA. 2008. Human natural killer cells. Blood. 112:461–469.
- Cantor H, Kasai M, Shen FW, Leclerc JC, Glimcher L. 1979. Immunogenetic analysis of “natural killer” activity in the mouse. Immunol Rev. 44:3–12.
- Cerottini JC, Engers HD, Macdonald HR, Brunner KT. 1974. Generation of cytotoxic T-lymphocytes in vitro. I. Response of normal and immune mouse spleen cells in mixed Leukocyte cultures. J Expert Med. 140:703–717.
- Craston R, Koh M, Mc Dermott A, Ray N, Prentice HG, Lowdell MW. 1997. Temporal dynamics of CD69 expression on lymphoid cells. J Immunol Meth. 209:37–45.
- Cui HF, Vashist SK, Al-Rubeaan K, Luong JH, Sheu FS. 2010. Interfacing carbon nanotubes with living mammalian cells and cytotoxicity issues. Chem Res Toxicol. 23:1131–1147.
- Delogu LG, Venturelli E, Manetti R, Pinna GA, Carru C, Madeddu R, Murgia L, Sgarrella F, Dumortier H, Bianco A. 2012. Ex vivo impact of functionalized carbon nanotubes on human immune cells. Nanomedicine (London). 7:231–243.
- Dumortier H. 2013. When carbon nanotubes encounter the immune system: desirable and undesirable effects. Adv Drug Deliv Rev. 65:2120–2126.
- Guidotti LG, Chisari FV. 2001. Noncytolytic control of viral infections by the innate and adaptive immune response. Annu Rev Immunol. 19:65–91.
- Hochreiter-Hufford A, Ravichandran KS. 2013. Clearing the dead: apoptotic cell sensing, recognition, engulfment, and digestion. Cold Spring Harbor Perspect Biol. 5:a008748.
- Kagi D, Ledermann B, Bürki K, Zinkernagel RM, Hengartner H. 1996. Molecular mechanisms of lymphocyte-mediated cytotoxicity and their role in immunological protection and pathogenesis in vivo. Annu Rev Immunol. 14:207–232.
- Kostarelos K, Lacerda L, Pastorin G, Wu W, Wieckowski S, Luangsivilay J, Godefroy S, Pantarotto D, Briand J, Muller S, et al. 2007. Cellular uptake of functionalized carbon nanotubes is independent of functional group and cell type. Nat Nanotechnol. 2:108–113.
- Kumari M, Sachar S, Saxena RK. 2012. Loss of proliferation and antigen presentation activity following internalization of poly-dispersed carbon nanotubes by primary lung epithelial cells. PLoS One. 7:e31890.
- Laverny G, Casset A, Purohit A, Schaeffer E, Spiegelhalter C, de Blay F, Pons F. 2013. Immuno-modulatory properties of multi-walled carbon nanotubes in peripheral blood mononuclear cells from healthy subjects and allergic patients. Toxicol Lett. 217:91–101.
- Mace EM, Dongre P, Hsu HT, Sinha P, James AM, Mann SS, Forbes LR, Watkin LB, Orange JS. 2014. Cell biological steps and checkpoints in accessing NK cell cytotoxicity. Immunol Cell Biol. 92:245–255.
- Marzio R, Mauël J, Betz-Corradin S. 1999. CD69 and regulation of the immune function. Immunopharmacol Immunotoxicol. 21:565–582.
- Mitchell LA, Lauer FT, Burchiel SW, McDonald JD. 2009. Mechanisms for how inhaled multi-walled carbon nanotubes suppress systemic immune function in mice. Nat Nanotechnol. 4:451–456.
- Miyake T, Kumagai Y, Kato H, Guo Z, Matsushita K, Satoh T, Kawagoe T, Kumar H, Jang MH, Kawai T, et al. 2009. Poly(I:C)-induced activation of NK cells by CD8α+ dendritic cells via the IPS-1 and TRIF-dependent pathways. J Immunol. 183:2522–2528.
- Oberdörster T, Castranova V, Asgharian B, Sayre P. 2015 Inhalation exposure to carbon nanotubes (CNT) and carbon nanofibers (CNF): Methodology and dosimetry. J Toxicol Environ Health 18:121–212.
- Orecchioni M, Bedognetti D, Sgarrella F, Marincola FM, Bianco A, Delogu LG. 2014. Impact of carbon nanotubes and graphene on immune cells. J Transl Med. 12:138.
- Reefman E, Kay JG, Wood SM, Offenhäuser C, Brown DL, Roy S, Stanley AC, Low PC, Manderson AP, Stow JL. 2010. Cytokine secretion is distinct from secretion of cytotoxic granules in NK cells. J Immunol. 184:4852–4862.
- Rizvi ZA, Puri N, Saxena RK. 2014. Lipid antigen presentation through CD1d pathway in mouse epithelial cells, macrophages and dendritic cells and its suppression by poly-dispersed single walled carbon nanotubes. Toxicol In Vitro. pii:S0887-2333(14)00213–6.
- Sachar S, Saxena RK. 2011. Cytotoxic effect of poly-dispersed single walled carbon nanotubes on erythrocytes in vitro and in vivo. PLoS One. 6:e22032.
- Salem ML, Al-Khami AA, El-Nagaar SA, Zidan AA, Al-Sharkawi IM, Marcela Díaz-Montero C, Cole DJ. 2012. Kinetics of rebounding of lymphoid and myeloid cells in mouse peripheral blood, spleen and bone marrow after treatment with cyclophosphamide. Cell Immunol. 276:67–74.
- Saxena RK. 1996. Understanding inverse correlation between the levels of Class I Major Histocompatibility Complex antigens on tumor cells and their susceptibility to natural killer cells: Evidence of competition experiments. Curr Sci. 70:143–146.
- Saxena RK. 1997. Ontogeny of inhibitory receptors for MHC molecules on NK cells. Immunol Today. 18:146–147.
- Saxena RK, Alder WH, Nordin AA. 1981. Modulation of natural cytotoxicity by alloantibodies. IV. A comparative study of the activation of mouse spleen cell cytotoxicity by anti H-2 antisera, interferon and mitogens. Cell Immunol. 63:28–41.
- Saxena RK, Saxena QB, Alder WH. 1982. Defective T-cell response in beige mutant mice. Nature. 295:240–241.
- Saxena RK, Saxena QB, Alder WH. 1984. Interleukin-2 activation of natural killer activity in spleen cells from old and young mice. Immunology. 51:719–726.
- Saxena RK, Saxena QB, Collins GD, Adler WH. 1983. Augmentation of spleen natural killer activity in mice treated with IL-2 preparation. Indian J Exp Biol. 21:54–58.
- Saxena RK, Gilmour MI, Hays MD. 2008. Isolation and quantitative estimation of diesel exhaust and carbon black particles ingested by lung epithelial cells and alveolar macrophages in vitro. BioTechniques. 44:799–805.
- Saxena RK, Wiessman D, Saxena QB, Simpson J, Lewis DM. 2002. Kinetics of changes in lymphocyte sub-populations in mouse lungs after intrapulmonary infection with M. bovis (Bacillus Calmette-Guerin) and identity of cells responsible for IFNγ responses. Clin Exp Immunol. 128:405–410.
- Saxena RK, Williams W, McGee JK, Daniels MJ, Boykin E, Gilmour MI. 2007. Enhanced in vitro and in vivo toxicity of poly-dispersed acid-functionalized single walled carbon nanotubes. Nanotoxicology. 1:291–300.
- Shvedova A, Fabisiak J, Kisin E, Murray A, Roberts J, Tyurina Y, Antonini J, Feng W, Kommineni C, Reynolds J, et al. 2008. Sequential exposure to carbon nanotubes and bacteria enhances pulmonary inflammation and infectivity. Am J Respir Cell Mol Biol. 38:579–590.
- Tong H, McGee JK, Saxena RK, Kodavanti UP, Devlin RB, Gilmour MI. 2009. Influence of acid functionalization on the cardiopulmonary toxicity of carbon nanotubes and carbon black particles in mice. Toxicol Appl Pharmacol. 239:224–232.
- Valentini F, Carbone M, Palleschi G. 2013. Carbon nanostructured materials for applications in nano-medicine, cultural heritage, and electrochemical biosensors. Anal Bioanal Chem. 405:451–465.
- Walling BE, Kuang Z, Hao Y, Estrada D, Wood JD, Lian F, Miller LA, Shah AB, Jeffries JL, Haasch RT, et al. 2013. Helical carbon nanotubes enhance the early immune response and inhibit macrophage-mediated phagocytosis of Pseudomonas aeruginosa. PLoS One. 8:e80283.
- Wang J, Sun RH, Zhang N, Nie H, Liu JH, Wang JN, Wang H, Liu Y. 2009. Multi-walled carbon nanotubes do not impair immune functions of dendritic cells. Carbon. 47:1752–1760.
- Zhang Y, Petibone D, Xu Y, Mahmood M, Karmakar A, Casciano D, Ali S, Biris AS. 2014. Toxicity and efficacy of carbon nanotubes and graphene: the utility of carbon-based nano-particles in nanomedicine. Drug Metab Rev. 46:232–246.
- Zhao L, Gao X, Peng Y, Joyee AG, Bai H, Wang S, Yang J, Zhao W, Yang X. 2011. Differential modulating effect of natural killer (NK) T-cells on IFNγ production and cytotoxic function of NK cells and its relationship with NK subsets in Chlamydia muridarum infection. Immunology. 134:172–184.