Abstract
Endosulfan (ENDO) is a widely used organochlorine (OC) pesticide and persistent organo-pollutant. Epidemiological studies have shown that high levels of OC exposure were related to colorectal cancer (CRC) incidence. The objectives of the present study were to evaluate histological changes in the colon, as well as in in situ expression of β-catenin and P-selectin, and serum levels of select pro-inflammatory cytokines in mice administered ENDO; there is a relationship between increased serum IL-6 and P-selectin levels in CRC patients and aberrant β-catenin signaling is important in initiation/maintenance of most CRCs. Mice were exposed to ENDO (at dose < LD50) orally once a week for up to 24 weeks, and monitored (inclusive) for a total of 42 weeks. The experiment was comprised of three groups, one that did not receive ENDO (olive oil vehicle), one administered 2 mg ENDO/kg/week and a positive control (for induction of CRC) given a weekly 20 mg 1,2-dimethylhydrazine (DMH)/kg injection. The results indicated that oral administration of ENDO provoked moderate inflammation starting at six weeks, and severe colonic inflammation with an appearance of dysplastic formations (aberrant crypts) in mice treated with ENDO (or DMH) for 12 weeks or longer. Serum IL-6 levels significantly increased starting at six weeks and rose to a peak of 15-fold higher than in controls at 42 weeks; TNFα levels likewise significantly increased, with a later peak (≈four-fold higher than controls) at 30–42 weeks. Immunohistochemical analysis of the colon also showed that expression of β-catenin and P-selectin increased with length of exposure to ENDO. Taken together, the results indicate that continued repeated oral exposure to ENDO induces increased expression of β-catenin and P-selectin, inflammation in the colon, and, ultimately, local tissue dysplasia.
Introduction
In recent years, there has been an increase in reports of diseases related to impaired immune responses, which may be largely due to environmental contamination by pesticides (Dar et al. Citation2012; Schaalan et al. Citation2012). In Mexico, there is a current problem with the deposition of endosulfan (ENDO), which is a pesticide still at present in use in Mexican agriculture (Wong et al. Citation2010). Endosulfan, an organochlorine insecticide (OC), was defined as a persistent organic pollutant (POP) by the Stockholm Convention on POP agents in 2011. Runoff from agricultural fields and/or discharge during the manufacture of the pesticide is responsible for the majority of ENDO contamination of air, water and soil environments (Guo et al. Citation2015). ENDO residues have also been detected in various fruits, vegetables, nuts, grains and fish (Canlet et al. Citation2013) with concentrations ranging from 0.0005 to 0.013 ppm, and in various sea foods from 0.2 ppt to 1.7 ppb.
It has been reported that over 90% of human exposure to ENDO is through food/liquid intake (Campoy et al. Citation2001; Silva & Carr Citation2010). Concordant with those studies, total ENDO isomers have been found in maternal milk (0.6–27.9 ng/ml) (Campoy et al. Citation2001), neonatal blood (9.2 ng/ml) (Schaalan et al. Citation2012) and adult serum (0.2–3 ng/ml) (Wade et al. Citation2002; Dar et al. Citation2012; Pi et al. Citation2016) in the general populations of countries where ENDO is still commonly used in agriculture. With respect to acute exposures, ENDO has been found up to 530 ng/ml in the blood of agricultural workers after application of ENDO in South Africa. In India, severe acute ENDO toxicity was observed when villagers ate foodstuffs contaminated with ENDO at a level of 676 ppm resulting in a serum levels of about 50–500 ng/ml, depending on exposure (Dewan et al. Citation2004).
Han et al. (Citation2007) showed, in an in vitro study with peritoneal macrophages, that ENDO stimulates the production of pro-inflammatory cytokines interleukin (IL)-1β, IL-6 and tumor necrosis factor (TNF)-α. The fundamental role of inflammation and inflammatory cytokines in the development of cancer is well known, and has recently been confirmed (Allavena et al. Citation2008). Dymicka-Piekarska et al. (Citation2007) demonstrated a relationship with increased serum IL-6 and P-selectin levels in colorectal cancer (CRC) patients. Endosulfan also directly up-regulated plasma levels of P-selectin, in rats treated with this pesticide (Zhang et al. Citation2015). Apart from P-selectin, other adhesion molecules (such as β-catenin) form adhesion complexes that play a major role in normal development and in the emergence of select diseases/tumors (Gottardi & Gumbiner Citation2004). Aberrant Wnt/β-catenin signaling is important in the initiation and maintenance of most CRCs. CRC has been reported as a result of uncontrolled proliferation of colon cells caused by hypertrophic Wnt/β-catenin signaling induced by OC (Song et al. Citation2014a).
ENDO has also been reported to have a possible carcinogenic potential based on a quantum chemical model analysis of its metabolites (Bedor et al. Citation2010). New research on links between OC exposure and cancer risk has recently been published (Song et al. Citation2014a,Citationb; Sharma et al. Citation2015; Pi et al. Citation2016). Epidemiological observations in humans have shown that high levels of OC exposure were related to CRC incidence; however, other reports found no significant correlation (Song et al. Citation2014a,Citationb). These contradictory epidemiological results make the contribution of OC exposures to CRC risk unclear. To address the possible correlation between OC exposure and cancer development, and the underlying mechanisms involved, it would be important to plan and conduct in vitro and in vivo studies.
Accordingly, the present study assessed the possible impacts of ENDO on inflammation and the development of CRC using an in vivo 1,2-dimethyl hydrazine (DMH) mouse model. The study also elucidated the mechanism of ENDO action in the distal colon on the expression of inflammation-induced molecules such as β-catenin and P-selectin in situ and upon serum levels of IL-6 and TNFα.
Materials and methods
Animals
Female BALB/c mice (Universidad de Guadalajara, Centro Universitario de Ciencias de la Salud, breeding colony) were used. Mice were 6–8 weeks of age at initiation of the study and were housed 10 mice/large cage according to institutional guidelines in facilities maintained at 22 ± 2 °C with a 60 ± 10% relative humidity and a 12-h light:dark cycle. All mice had ad libitum access to standard rodent chow and filtered water throughout the study. All mice were maintained at the same estrous cycle.
All procedures in this study were conducted according to the National Regulations on Health Research Matters (Mexico) and directive 86/609/EEC for animal experiments. All efforts were made to minimize animal suffering and the number of animals used. Weight and general health of the animals were tracked for the duration of the study.
Reagents/chemicals
Commercially-sourced technical grade ENDO (Thiodan, 35% EC) was procured from local agricultural suppliers (Bayer, Mexico City, Mexico). Positive control 1,2-dimethyl hydrazine (DMH) was purchased from Sigma (St. Louis, MO). Polyclonal antibodies against P-selectin (sc-6943) and β-catenin (sc-1496), rabbit anti- goat biotinylated secondary (sc-2774) antibody, avidin and biotinylated horseradish peroxidase (AB reagent) and diaminobenzidine (DAB) chromogen were bought from Santa Cruz Biotechnology (Santa Cruz, CA).
Experimental design
In this study, three groups were generated by random allocation of the mice (N = 10 for control and N = 30/each experimental group). In the studies, control mice were treated with olive oil only. Endosulfan was diluted in olive oil and administered (2 mg/kg) to groups of mice via oral gavage once a week for 24 weeks. A third group of animals was treated with a 20 mg/kg subcutaneous injection (200 μl) of 1,2-dimethylhydrazine (DMH, dissolved in sterile 1 mM EDTA [pH 6.5] solution) once a week as a positive control. The study was designed to continue until the appearance of cancer in the DMH group, which occurred at Week 42. Starting at Week 2 – every two weeks, three mice/experimental (treated) group were euthanized via intraperitoneal injection of 0.1 ml Penta-Hypnol (pentobarbital, 6.5 g/100 ml solution). Blood was extracted from the mice via cardiac puncture and the samples were processed to yield serum that was then stored at −20 °C until analyzed. Controls (aged-matched mice given weekly gavage of olive oil only, no ENDO) were euthanized at each timepoint (N = 1/timepoint) and the serum from those mice was used as the control values in subsequent ELISA assays. Use of a lower number of control mice/timepoint was based on preliminary analyses of the various endpoints herein that showed no significant variations as a function of host age among control mice (data not shown).
The dose of ENDO used in this study was based on previous reports with mouse models. A three-month study showed that 7.5 mg ENDO/kg led to ≈50% mortality; lower doses, from 2.13 to 0.24 mg/kg, did not induce mortality or toxic effects (Barnard et al. Citation1985; WHO/FAO Citation1990). A six-week study showed that a dietary dose of 18 ppm (≈2.13 mg/kg) ENDO led to 20% mortality (Donaubauer et al. Citation1985; WHO/FAO Citation1990). An LD50 for ENDO in BALBC/c mice was defined in an earlier study in our laboratory as 11.5 mg ENDO/kg for male and 8 mg ENDO/kg for female mice. The dose of 20 mg DMH/kg is in common use (Rosenberg et al. Citation2009) and was validated for this model in a pilot study here.
Histochemistry/immunohistochemistry
At necropsy, complete gross autopsies were performed on all mice. Tissues were removed and fixed in 10% formaldehyde, embedded in paraffin and processed for histology. Resulting 5-μm sections were then de-pariffinized, re-hydrated and stained using conventional hematoxylin–eosin (H&E) protocols. For immunohistochemical analysis, sections were treated with 3% H2O2 in methanol for 30 min to quench endogenous peroxidase activity. The sections were then washed three times in PBS, blocked with 10% normal rabbit serum (30 min, 37 °C) and, finally, incubated with a given primary antibody for 4 h in a humidified chamber held at 37 °C. The sections were then washed three times with PBS and incubated (protected from light) with the biotinylated-rabbit secondary antibody for 1 h. Thereafter, the sections were washed three times with PBS and then incubated for 30 min (protected from light) in a freshly-prepared AB (HRP-Biotin-Avidin) solution. After the sections were gently washed with PBS, the slides were coated with DAB reagent for 20 min, then rinsed in deionized water before being coverslipped. The slides were then evaluated in a blinded manner by a certified pathologist using an Olympus CH30 light microscope (Olympus, Tokyo, Japan) to ascertain the types/extent of any induced histopathologic changes in the organ. Histological changes were characterized according to a 12-point matrix (). Bright-field photos were also taken using an affixed camera.
Table 1. Histological scoring system for effects of ENDO in the colon.
A quantitative colorimetric assay was also utilized for the immunohistochemical analyses. In brief, using the Fiji distribution of ImageJ software (http://www.fiji.sc; Schneider et al. Citation2012), images were de-convoluted and the DAB color signal separated (Brey et al. Citation2003). Ten fields/slide were chosen, focusing on the glandular epithelial cells of the mucosa, and avoiding tissue folds, voids, and staining artifacts. The mean optical densities of the fields analyzed from the respective experimental conditions were then compared across the experimental groups.
ELISA
Serum levels of IL-6 and TNFα were determined using commercial ELISA kits from Abcam (Cambridge, United Kingdom) according to the manufacturer’s instructions. Kit sensitivity was <2 pg IL-6/ml and <60 pg TNFα/ml.
Statistical analysis
All data are reported as means ± SD. All data were evaluated for significant differences using a one-way analysis of variance (ANOVA) and Tukey’s test for all pair-wise comparisons of mean responses of the different experimental groups. Data analyses were performed using Centurion software (Statgraphics, StatPoint, Inc., Warrenton, VA). Significance was accepted at p < 0.05.
Results
General histology
Based on histological analyses performed at six-weeks intervals over the entire course of the 42-week study (), mice treated with 2 mg ENDO/kg began to show morphological changes in the colon starting at Week 6, but had significant levels of histological changes from Week 12 onwards. Even at Week 24 control mice had normal structures in the gut tissues (). In comparison, in ENDO mice – by Week 6 – discreet depletion of mucosal secretion in some crypts, weak inflammation and edema was seen in the tissues (). At a gross analytical level, the total diameter of the colon was notably thicker than in the controls. Stool from these mice was loose and contained slight levels of mucus. Progressing from Weeks 8 to 10 to 12, mice in the ENDO group exhibited signs of discomfort and increased diarrhea with feces containing higher levels of mucus. At a tissue level, an increased number of crypts in the colon of these mice exhibited modified architecture, with nuclear changes and notable mucin depletion, and mononuclear infiltration and edema as signs of moderate inflammation (). The dissected colon was grossly larger than healthy controls at this timepoint. Mice began to die at Week 12; due to this, the experiment was modified and surviving mice were euthanized for sample collection every subsequent six, not two, weeks. At Week 18, continued inflammation in the colon was observed (data not shown). At Week 24, the loss of colon architecture, presence of numerous aberrant crypts, vascular constriction and numerous erythrocytes were noted (). Mice at this timepoint presented with diarrhea, bloody stool and occult blood in their feces.
Figure 1. Histological changes in intestinal tissues of mice exposed to ENDO. (A) Histological scores of ENDO-mediated changes in sections with respect to scoring matrix in . HC = healthy control. Among ENDO hosts, *p < 0.05 for Week 6 samples vs. those at Weeks 12, 24, 30 and 42. (B–G) Representative H&E-stained sections demonstrating varying degrees of inflammation in the intestinal tissues. (B) Week 24: healthy control with normal epithelial architecture. Inset: normal crypt. (C) Week 6: ENDO mouse with some crypts with thickened epithelia and altered luminal openings, signs of weak inflammation: edema, and lymphocyte infiltration. Inset: crypt with mucosal depletion. (D) Week 12: ENDO mouse, the majority of crypts show changed structure with diminished mucus secretion, and moderate inflammation with lymphocyte infiltration. Inset: crypt with nuclear irregularities, loss of cell polarity. (E) Week 24: ENDO mouse with increase in aberrant crypt formation, strong vascular congestion. Inset: aberrant crypt with nuclear proliferation, prominent nucleoli. (F) Week 30 (six week post-final ENDO dose): treated mouse with continuous vascular congestion and lymphocyte infiltration. Inset: aberrant crypt with nuclear irregularity. (G) Week 42 (18 weeks post-final ENDO dose): treated mouse with severe colonic inflammation. Inset: example of aberrant crypt surrounded by infiltrating cells. Magnification =10×, inset =40×.
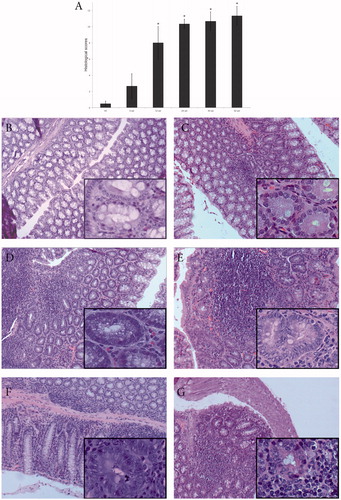
Administration of ENDO was stopped at Week 24 and the remaining weeks of the experiments involved monitoring cumulative effects of the already-administered ENDO. At Weeks 30 and 36 (6 and 12 weeks post-end of ENDO administration), samples were observed to have the same or higher levels of inflammation and disturbed colon architecture (). Mice at this stage exhibited difficulty with movement, feeding and passing stool. Both the spleen and liver in these hosts were larger than normal and one of the three mice at Week 30 showed evidence of lymphoma/leukemia in the liver. At Week 42 (18 weeks post-end of ENDO dosing), samples were observed to have the same/higher levels of inflammation and evident dysplastic colon architecture (). After performing necropsies on animals that died in the course of the study or sacrificed for timepoint experiments, we did not find any macroscopic evidence of colon cancer. Based on the histological scores, significant differences were found between control mice and mice exposed to ENDO at all timepoints, and significant differences were also found between mice exposed to ENDO for six weeks versus 24, 30 and 42 weeks ().
Positive control mice administered DMH developed aberrant crypt foci earlier and more intensely than their ENDO treated littermates. By Week 24, the presence of dysplasia was evident and increasingly mice began to die in this group. At Week 30 since initiation (six-week post-end of DMH administration), metaplastic structures and formation of polyps were seen in the colon. At Week 42 (18-week post-DMH administration), well-differentiated adenocarcinoma of the colon mucosa that corresponds to a typical colon cancer lesion was seen ().
Figure 2. DMH and histological changes in the colon. Representative sections are shown from each group. Representative normal tissues are shown in Figure 1(B). (A) Week 6: weak inflammation, discreet depletion of mucus secretion, cryptic abscesses. (B) Week 12: chronic inflammation, notable edema and depletion of mucosal secretion. (C) Week 24: appearance of dysplastic aberrant crypt foci (center of field), strong depletion of mucosal secretion. (D) Week 30 (six week post-final dose): metaplastic structures and numerous infiltrating lymphoid cells. (E) Week 42 (18 week post-final dose): well differentiated adenocarcinoma of the colon mucosa that corresponds to a typical colon cancer lesion. Magnification =10×; H&E staining.
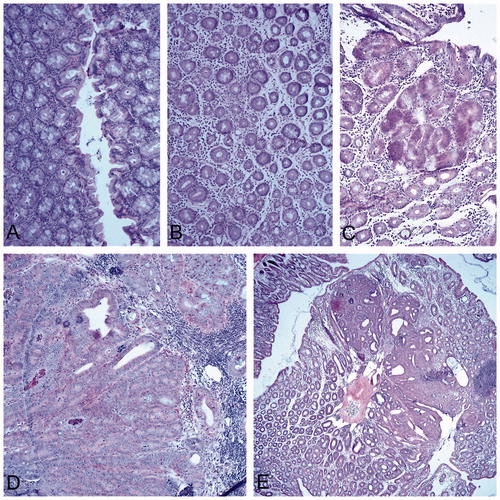
β-Catenin and P-selectin expression increases with ENDO exposure
The immunohistochemical changes in the gut tissues were quantified and graphed (). By Week 6 of the experiment, an increase in β-catenin-accumulating crypts was evident. Among control hosts, even at Week 24, there was weak staining of regenerating epithelial cells (). In comparison, as noted above, by Week 12 of ENDO administration, crypts had begun to change structure and β-catenin staining was stronger in dysplastic crypts closer to the intestinal lumen. By Week 24, aberrant crypt formation was increased and β-catenin staining was stronger in these colonic regions. Administration of ENDO was stopped at Week 24 and the subsequent observations report on the cumulative effects of the ENDO that had been given. In the Week 30, 36 and 42 samples, staining was also stronger in parts of the colon with radically changed architecture, indicating pre-neoplastic formations (with aberrant crypt foci at Week 42) (). Mice treated with DMH displayed stronger β-catenin staining, evident at 30 weeks (). Optical density analysis of the staining showed significant differences between control mice and mice exposed to ENDO at all timepoints, and significant differences were found between mice exposed to ENDO for six weeks versus 24, 30 and 42 weeks.
Figure 3. Immunohistochemical analyses of β-catenin expression in ENDO exposed mice. (A) Level of β-catenin expression in microscope fields, assessed via optical density. HC = healthy control. Among ENDO hosts, *p < 0.05 for Week 6 samples vs. those at Weeks 24, 30 and 42. (B–H) Representative DAB-stained sections from each group. (B) Week 24, healthy control: weak staining of regenerating epithelial cells. (C) Week 6, ENDO mouse: increased staining of β-catenin-accumulating crypts. (D) Week 12, ENDO mouse: crypts beginning to change structure, staining is stronger in crypts with changed structure closer to intestinal lumen. (E) Week 24, ENDO mouse: aberrant crypt formation with increase in number of β-catenin-accumulating cells and staining intensity in aberrant crypts. (F) Week 30 (six week post-final dose), ENDO mouse: β-catenin-accumulating cell staining with levels stronger in cells closer to intestinal lumen. (G) Week 42 (18 weeks post-final dose, ENDO mouse: strong staining in dysplastic aberrant crypt foci. (H) Week 30 (six week post-final dose), DMH mouse (positive control for strong inflammation): polyp formation, staining is more notable in crypts associated with polyps. Magnification =10×.
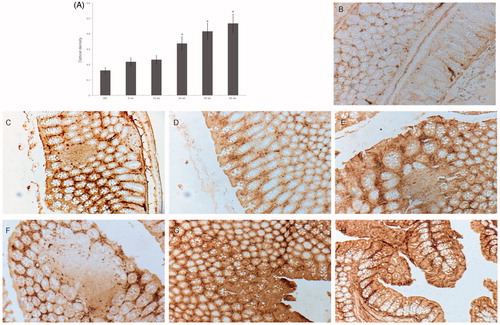
Changes in P-selectin staining were not as evident as the β-catenin staining, yet there were clear increases in P-selectin staining at the end of ENDO treatment (Week 24) and continuing through the remaining weeks of the study (). Based on density analysis of the P-selectin staining, increased exposure significantly increased staining vs. controls, however significant differences between timepoints were not found (data not shown). Mice treated with DMH displayed stronger P-selectin staining in concert with higher levels of tissue dysplasia, with the staining markedly evident in aberrant crypt foci ().
Figure 4. P-selectin expression in later-stage of ENDO and DMH exposure regimens. (A) Week 30, colon of representative section from ENDO mouse: strong staining in epithelia of individual aberrant crypts (circled). (B) Week 30, representative section from colon of DMH mouse: strong staining of pre-neoplastic formations (circled). Magnification =10×; DAB staining.
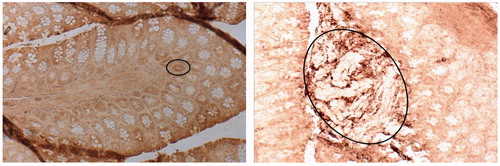
Serum IL-6 and TNFα
Among ENDO mice, a significant increase in IL-6 was observed starting at Week 6, and levels continued to be significantly higher than controls for all timepoints, with the highest values recorded at Week 42 (≈15-fold increase vs. control, p < 0.05) (). Values for the four healthy controls (one euthanized at Weeks 6, 24, 30, and 42) averaged 7.01 (±0.94) pg IL-6/ml; in mice exposed to ENDO for Weeks 6, 24, 30 and 42, these values were, respectively, 84.12 (±0.84), 85.68 (±2.93), 58.66 (±1.08), and 104.98 (±0.82) pg IL-6/ml.
Figure 5. Serum levels of IL-6 and TNFα. White bars = HC, Black bars = ENDO groups. (A) IL-6. (B) TNFα. HC = healthy control. *p < 0.05 vs. HC at given timepoint.
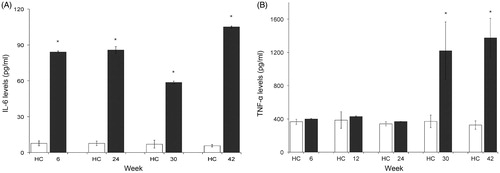
With TNFα, there was a small non-significant increase in serum levels in the ENDO mice starting at Week 6. Mice at Weeks 12 and 24 continued to show small non-significant increases when compared to counterpart control levels. At Weeks 30 and 42, however, ENDO mice displayed a strong significant increase (≈four-fold vs. control values, p = 0.002) (). Values for the five healthy controls (one euthanized at Weeks 6, 12, 24, 30 and 42) averaged 352.40 (±17.67) pg TNFα/ml; in mice exposed to ENDO for 6, 12, 24, 30 and 42 weeks, values were, respectively, 399.90 (±7.07), 428.65 (±3.83), 367.40 (±3.53), 1218.65 (±348.25) and 1373.65 (±235.11) pg TNFα/ml.
Discussion
The major findings in this study were that (1) the OC pesticide ENDO (ENDO) induced inflammation in the murine colon related to changes in β-catenin expression and IL-6 production and (2) ENDO continued to influence/exacerbate alterations in the intestinal mucosa up to 18 weeks after exposure had stopped. To date, we have not found equivalent studies in mice investigating the serum half-life of similar doses of ENDO. In rabbits, a 2 mg/kg dose was found to have a maximum plasma half-life of 400 h (Gupta & Ehrnebo Citation1979). In rats dosed with 5 mg ENDO/kg, serum half-life was found to be ≈193 h (Chan et al. Citation2005). The time required to reach 95% steady-state [drug] concentration after initiation or change in dose has been estimated to be ≈5× an agent’s serum half-life (Shargel et al. Citation2012). Since total body clearance is a pharmacokinetic measurement describing how quickly drugs are eliminated, metabolized or distributed throughout a body, the volume of distribution of a given substance represents the extent to which it is distributed throughout tissues, rather than in the plasma. Organochlorine pesticides have been reported to have a large volume of distribution, indicating a slower clearance (Sundlof et al. Citation1995). With respect to the above mentioned experimentally determined ENDO serum half lives, this would then imply that clearance in the host would take about 40–80 days. We surmise that here, ENDO “exposures” were continuous even after the end of application period. For this reason, the mice were monitored beyond the final 24 weeks ENDO application. As liposoluble ENDO (and its metabolites) are deposited in fatty tissues and the liver (Sutherland et al. Citation2004), we expected there was likely accumulation of the pesticide, and that effects would be present up to the final timepoints (i.e. Week 42) of the study.
To our knowledge, the present study is the first to investigate effects of chronic oral exposure to ENDO on histological changes in the colon and the in situ expression of β-catenin and P-selectin, and serum IL-6 and TNFα, in mice. Here, an oral dose of 2 mg ENDO/kg/week (equivalent to 12 ppm; Hack et al. Citation1995) provoked moderate inflammation starting at Week 6, and severe colonic inflammation [with appearance of pre-neoplastic elements (aberrant crypts)] in mice treated with ENDO (or DMH) for 12 weeks or longer. It was also noted that with increased exposure time, levels of β-catenin and P-selectin increased in concert with those of pro-inflammatory IL-6 and TNFα in the blood.
Atalay et al. (Citation2015) showed the relationship between PSGL-1 expression in the bone marrow and the development of multiple myeloma disease. Several lines of evidence indicate that even in tumors not directly linked to pathogens, the microenvironment is characterized by the presence of smoldering inflammation, fueled primarily by stromal leukocytes (Allavena et al. Citation2008). In the absence of Wnt signaling, β-catenin is targeted via coordinated phosphorylation for ubiquitination and proteasomal degradation. In the presence of Wnt signaling, β-catenin becomes stable and accumulates in the nucleus where it then binds T-cell factor/lymphoid enhancer factor to trigger transcription of target genes such as c-Myc and cyclin D1, both of which are involved in CRC progression (Song et al. Citation2014a). Colorectal cancer has been reported as a result of uncontrolled proliferation of colon cells caused by hypertrophic Wnt/β-catenin signaling induced by OC pesticides mediated by oxidative stress (Song et al. Citation2014b). Endosulfan has been found to up-regulate oxidative stress in immune cells from Nile tilapia (Tellez-Bañuelos et al. Citation2009).
In the current study, experiments to quantify local (tissue) levels of cytokines were not undertaken due to the fact that serum IL-6 and TNFα levels and intestinal tissue levels are very similar in inflammatory bowel disease (Hosokawa et al. Citation2001; Mudter & Neurath Citation2007). The tandem increase in both seric pro-inflammatory cytokines IL-6 and TNFα and increase in tissue levels of P-selectin and β-catenin is consistent with the recent report of Zhang et al. (Citation2015) noting increased P-selectin in the plasma and IL-1β in the sub-endothelial layer of the abdominal aorta in rats treated with 5 or 10 mg ENDO/kg/day. Although Howsam et al. (Citation2004) correlated exposure to OC pesticides, such as ENDO, with a risk of colon cancer. Bedor et al. (Citation2010) also reported on the carcinogenic potential of these compounds. In the current experiments, we did not find colon cancer in ENDO-treated mice observed up to 42 weeks; however, dysplasia of the colon mucosa – which may well be pre-cancerous – was observed.
The current study also found that beginning at Week 6 and again at Week 24, ENDO-treated mice had increased serum levels of IL-6 and at Weeks 30–42, increased levels of TNFα. The increases in these pro-inflammatory cytokines could be correlated with the onset of inflammation and significant lymphocytic infiltration in the colon at Weeks 12–30. This is consistent with the in vitro studies of Han et al. (Citation2007) that found that pro-inflammatory effects of ENDO – mediated through NF-κB – increased host levels of TNFα, IL-6, IL-1β and iNOS, and another study that found that activation of the GABA receptor (a major target of OC pesticides; Rand et al. Citation2010) enhanced synthesis of IL-6 and TNFα (Reyes-García et al. Citation2007). Mendu et al. (Citation2012) confirmed the presence of the GABAergic system in mammalian immune cells. It is known that patients with CRC have elevated levels of IL-6 in the serum and that these concentrations correlate with an increase of this cytokine in tumor tissues. Should ENDO be confirmed a carcinogen for the colon or other tissues, it is notable that IL-6 would play a role in the survival of transformed cells as it can promote angiogenesis by inducing expression of VEGF (Balkwill & Mantovani Citation2001). In light of this, the finding here of a probable case of lymphoma/leukemia in one Week 30 mouse is significant and should be investigated further.
In this vein, inflammation damages colonic tissue repair and induces increased epithelial replication. These, of course, can increase the rate of mutations that in turn may potentiate the development of cancer. An example of this is the predisposition that patients with chronic inflammatory bowel disease and Crohn's disease have to develop CRC (Mellemkjær et al. Citation2000).
Acknowledgements
The authors thank MSc. Miguel Enrique Magaña Virgen for his invaluable professional assistance and Biol. Lidia Adriadna Chavez Rubio for her technical assistance.
Disclosure statement
The authors declare no conflicts of interest. The authors alone are responsible for the content and writing of the paper.
Funding
This work was supported by the Universidad de Guadalajara, P3E [grant number 108126].
References
- Allavena P, Garlanda C, Borrello M, Sica A, Mantovani A. 2008. Pathways connecting inflammation and cancer. Curr Opin Genet Dev. 18:3–10.
- Atalay F, Atesoglu E, Yıldız S, Firatli-Tuglular T, Karakus S, Bayık M. 2015. Relationship of P-selectin glycoprotein ligand-1 to prognosis in patients with multiple myeloma. Clin Lymphoma Myeloma Leuk. 15:164–170.
- Balkwill F, Mantovani A. 2001. Inflammation and cancer: Back to Virchow? Lancet. 357:539–545.
- Barnard A, Jones D, Powell L, Heywood R, Street A, Gibson W, Gopinath G, Majeed S, Almond R. 1985. Endosulfan-active ingredient technical: 13-week toxicity study in rats followed by 4-week withdrawal period. HST 230/84176. Huntingdon, UK; Huntingdon Research Centre.
- Bedor C, Morais R, Cavalcanti L, Ferreira J, Pavão A. 2010. Carcinogenic potential of endosulfan and its metabolites based on a quantum chemical model. Sci Total Environ. 408:6281–6284.
- Brey E, Lalani Z, Johnston C, Wong M, McIntire L, Duke P, Patrick C. 2003. Automated selection of DAB-labeled tissue for immunohistochemical quantification. J Histochem Cytochem. 51:575–584.
- Campoy C, Jiménez M, Olea-Serrano M, Moreno Frias M, Cañabate F, Olea N, Bayés R, Molina-Font J. 2001. Analysis of organochlorine pesticides in human milk: Preliminary results. Early Human Dev. 65:S183–S190.
- Canlet C, Tremblay-Franco M, Gautier R, Jérôme M, Métais B, Estrada F, Payrastre G. 2013. Specific metabolic fingerprint of a dietary exposure to a very low dose of endosulfan. J Toxicol. 2013. doi:/10.1155/2013/545802.
- Chan M, Morisawa S, Nakayama A, Kawamoto Y, Sugimoto M, Yoneda M. 2005. Toxicokinetics of 14C-endosulfan in male Sprague-Dawley rats following oral administration of single or repeated doses. Environ Toxicol. 20:533–541.
- Dar S, Das S, Ramachandran V, Bhattacharya S, Mustafa M, Banerjee B, Verma P. 2012. Alterations in T-lymphocyte subset profiles and cytokine secretion by PBMC of systemic lupus erythematosus patients upon in vitro exposure to organochlorine pesticides. J Immunotoxicol. 9:85–95.
- Dewan A, Bhatnagar V, Mathur M, Chakma T, Kashyap R, Sadhu H, Sinha S, Saiyed H. 2004. Repeated episodes of endosulfan poisoning. J Toxicol Clin Toxicol. 42:363–369.
- Donaubauer H, Leist K, Kramer M. 1985. Endosulfan, substance technical: 42-day feeding study in mice. Report No. 88.0574. Frankfurt, Germany: Hoechst Aktiengesellschaft, Pharma Development Corporate Toxicology.
- Dymicka-Piekarska V, Matowicka-Karna J, Gryko M, Kemona-Chętnik I, Kemona H. 2007. Relationship between soluble P-selectin and inflammatory factors (interleukin-6 and C-reactive protein) in colorectal cancer. Thromb Res. 120:585–590.
- Gottardi C, Gumbiner B. 2004. Distinct molecular forms of beta-catenin are targeted to adhesive or transcriptional complexes . J Cell Biol. 167:339–349.
- Guo F, Zhang L, Wei J, Li Y, Shi Z, Yang Y, Zhou X, Sun Z. 2015. Endosulfan induced arrest of the cell cycle through inhibiting the signal pathway mediated by PKC-α and damaging the cytoskeleton in spermatogonial cells of mice in vitro. Toxicol Res. 4:508–518.
- Gupta P, Ehrnebo M. 1979. Pharmacokinetics of alpha- and beta-isomers of racemic endosulfan following intravenous administration in rabbits. Drug Metab Dispos. 7:7–10.
- Hack R, Ebert E, Leist KH. 1995. Chronic toxicity and carcinogenicity studies with the insecticide endosulfan in rats and mice. Food Chem Toxicol. 33:941–950.
- Han E, Hwang Y, Kim H, Jeong G. 2007. Inflammatory effect of endosulfan via NF-kappaB activation in macrophages. Biochem Biophys Res Commun. 355:860–865.
- Hosokawa T, Kusugami K, Ina K, Ando T, Shinoda M, Imada A, Ohsuga M, Sakai T, Matsuura T, Ito K, et al. 2001. Interleukin-6 and soluble interleukin-6 receptor in the colonic mucosa of inflammatory bowel disease. J Gastroenterol Hepatol. 14:987–996.
- Howsam M, Grimalt J, Guinó E, Navarro M, Martí-Ragué J, Peinado M, Capellá G, Moreno V. 2004. Organochlorine exposure and colorectal cancer risk. Environ Health Perspect. 112:1460–1466.
- Maranghi F, Mantovani A. 2012. Targeted toxicological testing to investigate the role of endocrine disrupters in puberty disorders. Reprod Toxicol. 33:290–296.
- Mellemkjær L, Johansen C, Gridley G, Linet M, Kjær S, Olsen J. 2000. Crohn's disease and cancer risk (Denmark). Cancer Causes Control. 11:145–150.
- Mendu S, Bhandage A, Jin Z, Birnir B. 2012. Different subtypes of GABA-A receptors are expressed in human, mouse and rat T-lymphocytes. PLoS One. 7:e42959.
- Mudter J, Neurath M. 2007. IL-6 signaling in inflammatory bowel disease: Pathophysiological role and clinical relevance. Inflamm Bowel Dis. 13:1016–1020.
- Pi N, Chia S, Ong C, Kelly B. 2016. Associations of serum organohalogen levels and prostate cancer risk: Results from a case-control study in Singapore. Chemosphere. 144:1505–1512.
- Rand G, Carriger J, Gardinali P, Castro J. 2010. Endosulfan and its metabolite, endosulfan sulfate, in freshwater ecosystems of South Florida: A probabilistic aquatic ecological risk assessment. Ecotoxicology. 19:879–900.
- Reyes-García M, Hernández-Hernández F, Hernández-Téllez B, García-Tamayo F. 2007. GABA(A) receptor subunits RNA expression in mice peritoneal macrophages modulate their IL-6/IL-12 production. J Neuroimmunol. 188:64–68.
- Rosenberg D, Giardina C, Tanaka T. 2009. Mouse models for the study of colon carcinogenesis. Carcinogenesis. 30:183–196.
- Schaalan M, Abdelraouf S, Mohamed W, Hassanein F. 2012. Correlation between maternal milk and infant serum levels of chlorinated pesticides (CP) and the impact of elevated CP on bleeding tendency and immune status in some infants in Egypt. J Immunotoxicol. 9:15–24.
- Schneider C, Rasband W, Eliceiri K. 2012. NIH Image to ImageJ: 25 years of image analysis. Nat Methods. 9:671–675.
- Shargel L, Wu-Pong S, Yu A. 2012. Applied biopharmaceutics and pharmacokinetics. 6th ed. New York: McGraw Hill (on-line); [cited 2016 Jun 16]. Available from: http://202.74.245.22:8080/xmlui/bitstream/handle/123456789/338/Chapter%205.%20Intravenous%20Infusion?sequence =6.
- Sharma T, Banerjee BD, Mazumdar D, Tyagi V, Thakur G, Guleria K, Ahmed RS, Tripathi AK. 2015. Association of organochlorine pesticides and risk of epithelial ovarian cancer: A case control study. J Reprod Health Med. 1:76–82.
- Silva M, Carr W. 2010. Human health risk assessment of endosulfan: II. Dietary exposure assessment. Regul Toxicol Pharmacol. 56:18–27.
- Song L, Liu J, Jin X, Li Z, Zhao M, Liu W. 2014b. p, p′-Dichlorodiphenyldichloroethylene induces colorectal adenocarcinoma cell proliferation through oxidative stress. PLoS One. 9:e112700.
- Song L, Zhao J, Jin X, Li Z, Newton IP, Liu W, Xiao H, Zhao M. 2014a. Organochlorine p,p′-dichlorodiphenyltrichloroethane induces colorectal cancer growth through Wnt/β-catenin signaling. Toxicol Lett. 229:284–291.
- Sundlof S, Riviere J, Arthur L, Craigmill A. 1995. Chapter 3: Chlorinated hydrocarbon insecticides. In: Sundlof S, Riviere J, Craigmill A, editors. Handbook of comparative veterinary pharmacokinetics and residues of pesticides and environmental contaminants. Boca Raton (FL): CRC Press. p. 37–51.
- Sutherland T, Horne I, Weir K, Russell R, Oakeshott J. 2004. Toxicity and residues of endosulfan isomers. Rev Environ Contam Toxicol. 183:99–113.
- Tellez-Bañuelos M, Santerre A, Casas-Solis J, Bravo-Cuellar A, Zaitseva G. 2009. Oxidative stress in macrophages from spleen of Nile tilapia (Oreochromis niloticus) exposed to sublethal concentration of endosulfan. Fish Shellfish Immunol. 27:105–111.
- Wade M, Foster W, Younglai E, McMahon A, Leingartner K, Yagminas A, Blakey D, Fournier M, Desaulniers D, Hughes C. 2002. Effects of subchronic exposure to a complex mixture of persistent contaminants in male rats: Systemic, immune, and reproductive effects. Toxicol Sci. 67:131–143.
- WHO/FAO (World Health Organization/Food and Agricultural Organization). 1990. Pesticide residues in food – 1989. FAO Plan Production and Protection Paper 100/2. Rome: Food and Agriculture Organization of the United Nations. p. 95–115.
- Wong F, Alegria H, Bidleman T. 2010. Organochlorine pesticides in soils of Mexico and the potential for soil-air exchange. Environ Pollut. 158:749–755.
- Zhang L, Wei J, Guo F, Duan J, Li Y, Shi Z, Yang Y, Zhou X, Sun Z. 2015. Endosulfan activates the extrinsic coagulation pathway by inducing endothelial cell injury in rats. Environ Sci Pollut Res Int. 22:15722–15730.