Abstract
The overall objective of disease management in autoimmune diseases is to suppress chronic inflammation and prevent organ damage. Therapies often revolve around five drug classes: non-steroidal anti-inflammatory drugs (NSAIDS), anti-malarials, steroids, immunosuppressants, and bio-therapies. However, none of these is a ‘cure’ and each displays a potential for adverse events. In particular, while all of them suppress harmful autoimmune responses, they also impact on useful protective immune responses. T-Cell receptor (TCR) immunogenicity provides a rationale for T-cell vaccinations to induce anti-idiotypic immune responses with the purpose of down-regulating functionality of idiotype-bearing self-reactive T-cells. To explore this, in this study, 39 patients with progressive (chronic) multiple sclerosis (MS) were multiply immunized with autological polyclonal T-cell vaccines (TCVs). None of the TCV-treated patients experienced any significant side-effects during the entire follow-up period (2 years). T-Cell vaccination had no significant effects on T-cell sub-population contents in the blood of MS patients after 2 years of immunotherapy initiation. However, a substantial reduction in the frequency of CD4+ and CD8+ memory T-cells able to produce interferon (IFN)-γ following activation were noted in the blood of TCV-treated patients. Moreover, significant and sustained reduction in plasma IFNγ levels and concomitant increases in interleukin (IL)-4 levels were documented in these samples. The TCV-treated subjects, however, exhibited no significant changes in plasma IL-17 and IL-18. More importantly was a significant decline in proliferative T-cell responses to myelin antigens in the TCV-treated patients, indicating attenuation of myelin-specific T-cell activity. Collectively, the results suggest that polyclonal T-cell vaccination is safe to use, able to induce measurable, long-lasting, anti-inflammatory immune effects in patients with advanced MS.
Introduction
Multiple sclerosis (MS) is an inflammatory autoimmune disease that targets myelin-associated antigens (Goverman Citation2009). MS symptoms usually begin with relapses and remissions, and neurologic deficits accumulate over time with secondary progression, that is characterized by chronic progressive course (Compston & Coles Citation2002). It is believed that autoimmune T-helper (TH)-1 and TH17 cells specific for myelin-associated antigens play a major role in the MS pathogenesis, irrespective of the initial triggering event (Hellings et al. Citation2002; Mannie & Curtis Citation2013; Seledtsov et al., Citation2010). After being activated encephalitogenic T-cells invade the central nervous system (CNS), and, by producing pro-inflammatory cytokines such as interferon (IFN)-γ, tumor necrosis factor (TNF)-α, interleukin (IL)-2, IL-17, and granulocyte-macrophage colony-stimulating factor (GM-CSF), recruit macrophages, CD8+ T-cells, and NK cells in nervous tissue-destructive processes (Gutcher & Becher Citation2007; Mannie & Curtis Citation2013; Seledtsov et al. Citation2010). In addition, these T-cells help the development of B-cell responses resulting in the production of myelin-specific antibodies (Ab), that, in turn, increase the tissue destruction through both Fc receptor- and complement-mediated mechanisms (Okun et al. Citation2010).
The overall objective of disease management in autoimmune diseases is to suppress chronic inflammation and prevent organ damage. Therapies revolve around five drug classes: non-steroidal anti-inflammatory drugs, anti-malarials, steroids, immunosuppressive drugs, and biological therapies. However, none of these disease-modifying drugs is a “cure”, and each displays the potential for serious adverse events (Huang et al. Citation2014; Vandenbark & Abulafia-Lapid, Citation2008). In particular, all of them suppress not only harmful autoimmune, but also useful protective immune responses. Indeed, pathogenic T-cells differ essentially from nonpathogenic ones by only their antigenic receptors. Hence, solely modality-targeted T-cell receptors (TCR) could provide selective inactivation of pathogenic T-cells. In fact, idiotype determinants present in TCR variable regions finally form in the postnatal period, therefore, no inherent immune tolerance exist to them. TCR immunogenicity provides the rationale for using T-cell vaccination to induce anti-idiotypic immune responses with the purpose of down-regulating functionality of idiotype-bearing, self-reactive T-cells. The disappearance of T-cell clones used in the vaccination procedure has been one of the most consistent findings of the various studies (Vandenbark & Abulafia-Lapid Citation2008).
We have developed a simple and relatively inexpensive method for preparing polyclonal T-cell vaccines without long-lasting cell cultivations. Such vaccines were found to be safe for clinical use and effective in inducing anti-idiotypic immune responses in MS patients. Considerable immune and clinical efficacy was noted in certain patients with relapsing MS (Ivanova et al. Citation2005, Citation2008). The aim of this study was to evaluate safety and immune efficacy of a polyclonal T-cell vaccination in patients with chronic MS in advanced stages of the disease when disease management is most problematic.
Materials and methods
Patients
Experimental and clinical studies were performed in compliance with the protocol approved by the Scientific Council and Ethical committee of the Institute for Clinical Immunology, Siberian Branch of the Russian Academy of Medical Sciences, Russia. An informed consent statement was obtained from each patient participating in this study. Clinical diagnosis was confirmed by magnetic resonance study. All patients had measurable progression in their functional status during the year prior to inclusion. They had no clinical evidence for any chronic gastrointestinal and renal diseases, as well as an additional autoimmune condition unrelated to MS or significant allergy. Hepatitis A, B, and C viruses, a cytomegalovirus, Type 1 and 2 herpes viruses were not detected in their bloods by a PCR analysis. The patients received no immunosuppressive therapy for at least 3 months before the onset of immunotherapy and in the course of its conducting.
T-Cell vaccine preparation
We have developed a two-step technology of T-cell vaccine preparation that is technically straightforward, and does not include long-lasting cell culturing procedures making it easily reproducible under the GMP conditions (Ivanova et al. Citation2005, Citation2008, Citation2014). Myelin has been isolated from porcine brain as described previously (Deibler et al. Citation1972). As Step 1 in preparing TCV, peripheral blood mononuclear cells (PBMC) were cultured at a concentration of 2 × 106 PBMC/ml in complete RPMI 1640 medium (i.e. RPMI supplemented with 10% inactivated autologous plasma, 5 mM HEPES, 2 mM l-glutamine, 50 μM β-mercaptoethanol) (all reagents purchased from Sigma, St. Louis, MO) in the presence of a myelin-associated antigens (50 μg/ml) for 7 days in a humidified incubator with 5% CO2. Optimal concentrations of all reagents have been determined in separate experiments. Step 2 consisted in the expansion of antigen-specific cells in the presence of 5 μg PHA/ml (phytohemagglutinin, Sigma) and recombinant IL-2 (100 U/ml, Biotech, St. Petersburg, Russia) for 5 d. T-Cells expanded in this manner have been previously shown to maintain increased reactivity to myelin antigens (Ivanova et al. Citation2008). The total cell number obtained from one patient ranged between 18 and 27 × 107. After being cultured, cells were cryopreserved routinely in plasma with 10% dimethylsulfoxide (DMSO; Sigma), and stored in liquid nitrogen until use. Vaccinal T-cells were validated for proliferative responses in the presence of myelin-associated antigens (1.8–2.0 fold increase vs. controls).
T-Cell vaccine administration and safety evaluation
The therapeutic regimen with the T-cell vaccine included four weekly subcutaneous injections (induction course) followed by subsequent monthly vaccinations. One vaccine dose was formulated in a volume of 2 ml of autological plasma to contain between 1.5 and 3.0 × 107 cells. Systemic toxicity was assessed according to the National Cancer Institute Common Toxicity scale. Safety evaluation also included hematological and biochemical tests (http://ctep.cancer.gov/protocolDevelopment/electronic_applications/docs/ctc manual_v4_10–4-99.pdf).
Proliferative PBMC responses
Peripheral blood mononuclear cells (2 × 105/well) were cultured using complete RPMI 1640 medium in 96-well plates in the presence or absence of myelin-associated antigens (50 μg/ml) for 5 d at 37 °C in a humidified incubator with 5% CO2. Cell proliferation was measured using a standard [3H] thymidine incorporation method.
T-Cell analysis
Relative quantification of memory T-cells was performed by flow cytometry using CD4- and CD8-specific mouse anti-human antibody (Ab) conjugated with PE (phycoerythrin; Sorbent, Moscow, Russia) and mouse anti-human CD45RO-specific Ab conjugated with FITC (fluorescein isothiocyanate), as well as mouse anti-human CD62L-specific Ab conjugated with APC (allophycocyanin, eBioscience, San Diego, CA). The following cell populations were detected depending on the expression pattern of the corresponding molecular cell surface markers: naive T-cells (CD45RO-CD62L+), central memory T-cells (CD45RO + CD62L+), and effector memory T-cells (CD45RO + CD62L-). Relevant data were ultimately shown as percentage of each cell population from the total number of lymphocytes.
Quantification of IFNγ- and IL-4-producing lymphocytes was performed by an intra-cellular cytokine staining method in 4 h PBMC cultures in the presence of 30 phorbol ester ng/ml, 1 μg ionomycin/ml, and 10 μg brefeldin A/ml (all reagents from ICN, Irvine, CA). After cultivation, cells were incubated with mouse anti-human Ab against T-cell surface markers CD4 and CD8 labeled with PerCP (peridinin chlorophyl protein complex, BD Biosciences, San Jose, CA), fixed, permeabilized, and incubated with mouse anti-human Ab against IFNγ labeled with FITC and IL-4 labeled with PE (these reagents were from BD Biosciences).
In both series of studies, after labeling, cells were washed and then analyzed in a FACSCalibur™ system (BD Biosciences) using system associated software. A minimum of 50 000 events/sample was acquired in each analysis.
Cytokine measurements
Quantitative measurements of IFNγ, IL-4, IL-10, Il-17 and IL-18 were performed in plasma samples using commercial ELISA diagnostic kits (Vector-Best, Novosibirsk, Russia). All samples were analyzed in triplicate. The level of sensitivity of the kits was 2.0 pg IFNγ/ml, 0.4 pg IL-4/ml, 1.0 pg IL-10/ml, 2.0 pg IL-17/ml, and 2.0 pg IL-18/ml.
Neurological status evaluation
The neurological status of each patient was evaluated by the Kurtzke expanded disability status scale (EDSS, Expanded Disability Status Scale, range 0.0 = normal neurological status to 10.0 = death due to MS) following protocols outlined by the National MS Society (http://www.nationalmssociety.org/NationalMSSociety/media/MSNationalFiles/Brochures/10–2-3–29-EDSS_Form.pdf).
Statistical analysis
All data were reported as means ± SEM. All analyses were performed using a Wilcoxon–Mann–Whitney U-test and a Wilcoxon signed-rank test. Analyses were done using SPSS Software (IBM Corp., Armonk, NY).
Results
Safety of T-cell vaccination
Thirty-nine patients with definite MS and progressive course (9 with primary progressive and 30 with secondary progressive MS) were enrolled in this study; their clinical characteristics are presented in . None of the vaccine-treated patients experienced any significant side effect during the entire follow-up period (2 years). No fever, changes in respiratory rate, blood pressure or heart rate were noted. No abnormalities in the white blood counts or blood biochemistry were encountered. No unexpected pathology could be seen in any brain MRI.
Table 1. Clinical characteristics of MS patients (n = 39).
Immune responses to T-cell vaccination
It was previously reported that the blood content of memory T-cells was significantly higher in patients with MS than in healthy control subjects (Okuda et al. Citation2005). Moreover, a higher percentage of CD3 + CD45RO+ cells was noted in MS patient blood at phases of exacerbation than during disease remission (Okuda et al. Citation2005). Here, the sub-population composition of blood T-lymphocytes in 10 healthy person (5 males, 5 females; average age 37 years) and in 11 evaluable chronic MS patients (3 males, 8 females; average age 40 years) was assessed (). The results showed there was an increase in central memory CD4 + CD45RO + CD62L+ and CD8 + CD45RO + CD62L+ T-cells in MS patients compared with healthy donors. The blood contents of effector CD8 + CD45RO + CD62L-, but not CD4 + CD45RO + CD62L-, cells were also much higher in the MS patients. TCV treatment carried out within 1 year had no significant effects upon T-cell subset contents in the blood of MS patients.
Table 2. Contents of memory T-cell subsets.
It is believed that IFNγ-producing T-cells promote MS development (Gutcher & Becher Citation2007; Mannie & Curtis Citation2013; Seledtsov et al. Citation2010) whereas T-cell formation of IL-4 could exert a neuroprotective effect (Seledtsov & Seledtsova Citation2012). The current study evaluated the frequencies of T-cells able to produce IFNγ/IL-4 in samples from 12 healthy subjects (5 males, 7 females; average age 33 years) and in 10 MS patients (3 males, 7 females; average age 39 years). The data in show that blood frequencies of naïve CD4 + CD45RO- and CD4 + CD45RO- but also memory CD4 + CD45RO+ and CD4 + CD45RO+ T-cells making either IFNγ or IFNγ + IL-4 in response to activation were initially significantly higher in the MS patients compared to healthy subjects. TCV-treated MS patients exhibited a noticeable decrease in blood frequencies of both CD4+ and CD8+ IFNγ-producing T-cells, suggesting a systemic T-cell functionality shift to an anti-inflammatory direction.
Table 3. Frequencies of T-cell subsets.
Consistent with this, significant and sustained reduction in plasma IFNγ levels and concomitant increases in IL-4 levels were documented in 19 MS patients (5 males, 14 females; average age 35 years) in the course of the TCV treatment. However, no significant changes in plasma IL-17 and IL-18 were noted in these same patients (). More importantly, there was a significant decline in proliferative PBMC responses to myelin-associated antigens among the TCV-treated MS patients, indicating attenuation of myelin-specific T-cell activity ().
Figure 1. Myelin-induced proliferative responses by PBMC from MS patients. PMBC were isolated from MS patients (n = 19; 5 male, 14 female) before and 1 year after onset of TCV-based treatment. In this assay, at each timepoint, PBMC were cultured without or with myelin-associated antigens for 5 days and then assessed for proliferative responses (in terms of cpm). Values shown are means (± SD) cpm. #Value significantly different between cultures with and without myelin-associated (p < .05). *Value significantly different between data before and after TCV-based treatment (p < .05).
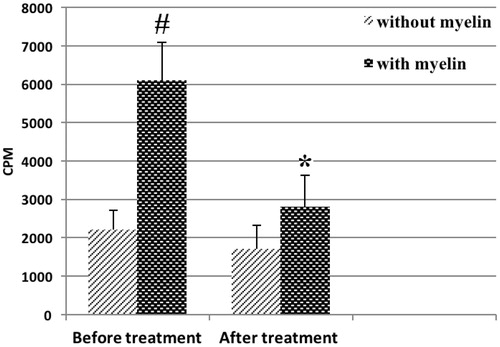
Table 4. Plasma cytokine levels.
Discussion
The limited clinical effectiveness of conventional treatments for autoimmune diseases, including MS, is mainly explained by a nonselective drug action at the cellular level. Pathogenic and nonpathogenic lymphocytes have no significant structural and biochemical differences and this very complicates the search for targeted medicines. In fact, autoantigen-recognizing TCR represents a sole specific marker of a self-reactive T-lymphocyte. Consequently, progress in developing modalities for autoimmune diseases should be primarily associated with technologies targeted at self-reactive TCR. Of great importance is that the variable TCR regions are finally formed in the post-natal period and, therefore, no innate immune tolerance to them exists in the adult body. Moreover, idiotypic TCR determinants are firmly established to be immunogenic and capable of eliciting an anti-idiotype immune responses, which are implicated in down-regulation of autoimmune processes (Seledtsov et al. Citation2010; Vandenbark & Abulafia-Lapid Citation2008).
The concept of T-cell vaccination is based on multiple immunizations with self-reactive T-cells to delete or inactivate targeted T-cells. TCV-induced anti-idiotypic T-cells have been shown to consist of both CD4+ and CD8+ T-cells, with fine specificity for hyper-variable TCR regions, including both CDR2 and CDR3 determinants (Zang et al. Citation2000). The CD8+ T-cells may be cytotoxic and/or inhibitory for CD4+ target T-cells (Buenafe et al. Citation2004). By secreting a variety of cytokines, including IL-4 and IL-10, the CD4+ T-cells may down-regulate the function of pathogenic immune cells. Moreover, the TCV-stimulated, CD4+ FoxP3+ T-regulatory (Treg) cells can inhibit the activation of target T-cells through cognate T-T interactions (Seledtsov et al. Citation2010; Vandenbark & Abulafia-Lapid Citation2008).
The current study reflects on our recent development of a relatively cheap simple technology to produce individual polyclonal T-cell vaccines, i.e. one that does not require long-lasting cell culturing and other labor-consuming procedures. It has been previously shown that the number of idiotypic determinants such vaccine is quite sufficient for inducing measurable anti-idiotypic immune responses in MS patients. PBMC isolated from vaccinated MS patients demonstrated an increase in proliferative activity in the presence of vaccinal myelin-reactive, but not collagen-reactive T-cells. Anti-idiotypic T-cell lines raised from TCV-treated MS patients produced IL-4 and IL-10 in response to specific activation (Ivanova et al. Citation2008).
Polyclonal T-cell vaccines prepared with a whole complex of myelin antigens have apparent advantages over the monoclonal or oligoclonal T-cell vaccines. From available data (Fox et al. Citation2012; Huang et al. Citation2014), it appears that myelin basic protein (MBP), proteolipid protein (PLP), myelin oligodendrocyte glycoprotein (MOG) and possibly other yet-unidentified molecules can be implicated in MS development. Hence, antigenic specificity of monoclonal or oligoclonal vaccinal T-cells may be distinguished from self-reactive Т-cells dominating in autoimmune process by antigenic specificity of their TCR. It is also conceivable that more than one neural antigen may be simultaneously targets for self-reactive cells. In this case, TCV-induced inactivation of only small portion of autoimmune T-cells with the limited antigenic specificity could be ineffective in inhibiting overall MS development.
It is important to note that the cell compositions in the TCV prepared in our laboratory were determined by individual autoimmune T-cell reactivities, In fact, more autoaggressive Т-cells received correspondingly more growth preferences in the presence of a variety of myelin-associated antigens in the culture. Consequently, their idiotypic determinant present in TCV more likely reached threshold quantitative levels to induce idiotype-specific immune responses. It is also important to note that a polyclonal TCV can be readily obtained from any patient more than one time. Such TCV could be effective against new autoaggressive T-cell clones that appear over time.
A whole ТCV has also significant advantages over the TCR peptide-based vaccines, as in addition to the anti-idiotypic immune regulation, vaccinal T-cells trigger anti-ergotypic immune suppression, which is based on the immune recognition of molecules associated with T-cell activation, such as CD25, HSP60, and the TNFα receptor. This bystander suppression may be important in modulating disease particularly during the vaccination period and shortly thereafter (Seledtsov et al. Citation2010; Vandenbark & Abulafia-Lapid Citation2008).
No serious side-effects of TCV administration were documented in our study, but also in all other clinical investigations performed so far (Huang et al. Citation2014; Seledtsov et al. Citation2010). An exclusive safe T-cell vaccination is extremely important for a chronically ill patient whose health is compromised by not only the disease but also its treatments.
Memory T-cells able to differentiate in effector T-cells producing IFNγ and other pro-inflammatory cytokines play key roles in developing MS (Bhargava and Calabresi Citation2015). Memory T-cells are identified by the expression of the marker CD45RO. Two major subtypes of memory T-cells are circulating in the blood: central and effector. CD62L+ central memory T-cells retain their ability to migrate into secondary lymphoid tissues. These cells have a greater proliferative capacity and are longer-lived compared to CD62L- effector memory T-cells circulating into peripheral non-lymphoid tissues (Tanel et al. Citation2009). The percentage of memory T-cells in the blood has been shown to be significantly higher in MS patients than in healthy control subjects. MS patients also showed a higher blood content of CD4+ memory cells at the phase of exacerbation than during remission (Okuda et al. Citation2005). The available data suggest that the activation of memory CD4+ T-cells is associated with the MS exacerbation, whereas activation of memory CD8+ T-cells reflects systemic immunological dysregulation in MS patients (Bhargava and Calabresi Citation2015; Okuda et al. Citation2005). Consistent with the above-mentioned data, the current study found significantly increased blood contents of both CD4+ and CD8+ central memory cells in MS patients. The frequency of CD8+, but not CD4+, effector memory cells was also increased in those patients. The TCV-based treatment did not appear to change blood content of memory T-cells. However, more importantly, vaccination led to a noticeable decrease in blood frequencies of both СD4 and CD8 memory T-cells capable of producing IFNγ when in activated states. A significant reduction in plasma IFNγ levels and a concomitant increase in IL-4 and IL-10 levels could also indicate attenuation of pro-inflammatory overall T-cell activities in TCV-treated MS patients. These effects might be due, at least in part, to TCV-induced anti-ergotypic immune down-regulation.
The present study was unable to detect any measurable effects of T-cell vaccination on plasma levels of IL-17 and IL-18 which, as previously reported (Gutcher & Becher Citation2007), may be implicated in MS development.
We propose that attenuation of overall systemic inflammation could be a necessary condition for the functioning highly specific immune mechanisms responsible for the selective inactivation of idiotype-bearing, autoimmune T-cells. Indeed, in our study polyclonal T-cell vaccination led to a considerable reduction of proliferative responses of T-cells to myelin-associated antigens, suggesting a significant decrease in activity of autoimmune T-cells in TCV-treated MS patients. Since TCV stimulates anti-idiotypic immune memory, long-lasting immune and clinical effects could be expected.
It has been previously shown that T-cell vaccination can be effectively used in the treatment of relapsing forms of MS (Fox et al. Citation2012; Huang et al. Citation2014). A progressive MS is usually less sensitive to therapeutic manipulations compared to relapsing MS. The present study demonstrated the feasibility/reasonability of T-cell vaccination at relatively late advanced stages of the disease when immunological mechanisms controlling autoimmune pro-inflammatory responses become unable to interrupt [even temporarily] pathological processes leading to tissue destruction. Interestingly, some TCV-treated patients experienced considerable neurological improvement, indicating a possibility of retaining certain regenerative potential by nerve tissues even at advanced stages of MS. Inhibition of autoimmune inflammation could create the necessary conditions for realizing this potential.
Collectively, the current findings provide the basis for an assumption that immune system down-regulation may maintain a sensitivity to T-cell vaccinations in patients with advanced autoimmune diseases. Undoubtedly, further clinical studies with a larger group of patients and longer follow-up periods are needed in this direction.
Acknowledgments
The authors are grateful to physicians of the regional center of multiple sclerosis (Novosibirsk, Russia) for recruitment and management of patients. Also, the authors thank researchers Pronkina and Savkina for help in quantifying IFNγ- and IL-4-producing cells.
Disclosure statement
The authors declare no conflict of interests. The authors alone are responsible for the content of this manuscript.
References
- Bhargava P, Calabresi P. 2015. Novel therapies for memory cells in autoimmune diseases. Clin Exp Immunol. 180:353–360.
- Buenafe A, Tsaknaridis L, Spencer L, Hicks K, McMahan R, Watson L, Culbertson N, Latocha D, Wegmann K, Finn T, et al. 2004. Specificity of regulatory CD4+CD25+ T-cells for self-T-cell receptor determinants. J Neurosci Res. 76:129–140.
- Compston A, Coles A. 2002. Multiple sclerosis. Lancet. 359:1221–1231.
- Deibler GE, Martenson RE, Kies MW. 1972. Large scale preparation of myelin basic protein from central nervous tissue of several mammalian species. Prep Biochem. 2:139–165.
- Fox E, Wynn D, Cohan S, Rill D, McGuire D, Markowitz C. 2012. A randomized clinical trial of autologous T-cell therapy in multiple sclerosis: Subset analysis and implications for trial design. Mult Scler.18:843–852.
- Goverman J. 2009. Autoimmune T-cell responses in the central nervous system. Nat Rev Immunol. 9:393–407.
- Gutcher I, Becher B. 2007. APC-derived cytokines and T-cell polarization in autoimmune inflammation. J Clin Invest. 117:1119–1127.
- Hellings N, Raus J, Stinissen P. 2002. Insights into the immunopathogenesis of multiple sclerosis. Immunol Res. 25:27–51.
- Huang X, Wu H, Lu Q. 2014. The mechanisms and applications of T cell vaccination for autoimmune diseases: A comprehensive review. Clin Rev Allergy Immunol. 47:219–233.
- Ivanova I, Seledtsova G, Mamaev S, Shishkov A, Seledtsov V. 2014. Immune responses induced by T-cell vaccination in patients with rheumatoid arthritis. Hum Vaccin Immunother. 10:1221–1227.
- Ivanova I, Seledtsov V, Banul N, Samarin D, Seledtsova G, Savkin I, Shirinsky V, Pronkina N, Kozlov V. 2005. Preparation of T-cell vaccine and application in treating multiple sclerosis. Med Immunol (Russia). 7:27–32.
- Ivanova I, Seledtsov V, Seledtsova G, Mamaev S, Potyemkin A, Seledtsov D, Kozlov V. 2008. Induction of anti-idiotypic immune response with autologous T-cell vaccine in patients with multiple sclerosis. Bull Exp Biol Med. 146:133–138.
- Mannie M, Curtis A. 2013. Tolerogenic vaccines for multiple sclerosis. Hum Vaccin Immunother. 9:1032–1038.
- Okuda Y, Okuda M, Apatoff B, Posnett D. 2005. The activation of memory CD4+ T-cells and CD8+ T-cells in patients with multiple sclerosis. J Neurol Sci. 235:11–17.
- Okun E, Mattson M, Arumugam T. 2010. Involvement of Fc receptors in disorders of the central nervous system. Neuromolecular Med. 12:164–178.
- Seledtsov D, Seledtsov V, Ivanova I, Litvinova L. 2010. Antigen-specific immunotherapy of multiple sclerosis. Cytokines Inflamm (Russia). 1:3–12.
- Seledtsov VI, Seledtsova GV. 2012. A balance between tissue-destructive and tissue-protective immunities: A role of toll-like receptors in regulation of adaptive immunity. Immunobiology. 217:430–435.
- Tanel A, Fonseca S, Yassine-Diab B, Bordi R, Zeidan J, Shi Y, Benne C, Sékaly R. 2009. Cellular and molecular mechanisms of memory T-cell survival. Expert Rev Vaccines. 8:299–312.
- Vandenbark A, Abulafia-Lapid R. 2008. Autologous T-cell vaccination for multiple sclerosis: A perspective on progress. BioDrugs. 22: 265–273.
- Zang Y, Hong J, Rivera V, Killian J, Zhang J. 2000. Preferential recognition of TCR hypervariable regions by human anti-idiotypic T-cell induced by T cell vaccination. J Immunol. 164:4011–4017.