Abstract
Skeletal health consequences associated with inflammatory diseases of the airways significantly contribute to morbidity. Sex differences have been described independently for lung and bone diseases. Repetitive inhalant exposure to lipopolysaccharide (LPS) induces bone loss and deterioration in male mice, but comparison effects in females are unknown. Using an intranasal inhalation exposure model, 8-week-old C57BL/6 male and female mice were treated daily with LPS (100 ng) or saline for 3 weeks. Bronchoalveolar lavage fluids, lung tissues, tibias, bone marrow cells, and blood were collected. LPS-induced airway neutrophil influx, interleukin (IL)-6 and neutrophil chemoattractant levels, and bronchiolar inflammation were exaggerated in male animals as compared to female mice. Trabecular bone micro-CT imaging and analysis of the proximal tibia were conducted. Inhalant LPS exposures lead to deterioration of bone quality only in male mice (not females) marked by decreased bone mineral density, bone volume/tissue volume ratio, trabecular thickness and number, and increased bone surface-to-bone volume ratio. Serum pentraxin-2 levels were modulated by sex differences and LPS exposure. In proof-of-concept studies, ovarectomized female mice demonstrated LPS-induced bone deterioration, and estradiol supplementation of ovarectomized female mice and control male mice protected against LPS-induced bone deterioration findings. Collectively, sex-specific differences exist in LPS-induced airway inflammatory consequences with significant differences found in bone quantity and quality parameters. Male mice demonstrated susceptibility to bone loss and female animals were protected, which was modulated by estrogen. Therefore, sex differences influence the biologic response in the lung-bone inflammatory axis in response to inhalant LPS exposures.
Introduction
The lung-bone inflammatory axis describes the relationship between lung inflammatory diseases and bone/articular diseases including osteopenia/osteoporosis and rheumatoid arthritis (RA). The lung has been suggested as the primary site for disease development of RA based upon the strong association of cigarette smoking with disease-specific autoimmunity and RA development (Perry et al. Citation2014). Moreover, male RA is emerging as a potential occupational disease because inhalation exposures such as silica, construction work, farming, pesticides, and dusts are associated with increased risk of RA (Murphy and Hutchinson Citation2017). Osteoporosis and fracture are also recognized as debilitating systemic features of several types of chronic inflammatory lung diseases including chronic obstructive pulmonary disease (COPD), asthma, cystic fibrosis, and post-lung transplant (Robertson and Macdonald Citation2010; Lehouck et al. Citation2011; Chauhan et al. Citation2012; Graat-Verboom et al. Citation2012; Jung et al. Citation2014). In COPD and asthma, low bone mineral density (BMD) and osteoporosis can occur independently of established osteoporosis risk factors, such as age, nutrition, medications, body mass index, cigarette smoking, and gender (Graat-Verboom et al. Citation2012; Jung et al. Citation2014). This suggests that lung inflammation is an independent risk factor for BMD loss. The potential for a lung-bone inflammatory axis is also suggested for agriculture workers because up to two-thirds of such workers develop respiratory diseases (e.g. rhinosinusitis, asthma, chronic bronchitis, COPD) (Dosman et al. Citation2004; Merchant et al. Citation2005; Eduard et al. Citation2009; May et al. Citation2012), and nearly 90% suffer from musculoskeletal disease, including increased rates of fracture and RA (Leon et al. Citation2011; Osborne et al. Citation2012; Parks et al. Citation2016).
Our laboratory has previously reported on an animal model wherein it was demonstrated that repetitive intranasal inhalation of agricultural dust extracts and its microbial components, including lipopolysaccharide (LPS)-induced systemic bone loss (Dusad et al. Citation2013, Citation2015; Staab et al. Citation2016). Whereas, those prior studies focused on male mice based on farming being a predominately male occupation, the National Institutes of Health has re-emphasized the importance of appropriate accounting for potential influences of sex on experimental outcomes in preclinical research (Collins and Tabak Citation2014). Indeed, sex differences have been independently described for lung inflammatory diseases and for bone quantity and quality parameters in several animal studies. For example, in an LPS-induced airway inflammatory and hyper-responsiveness study, male mice demonstrated an exaggerated response to LPS that was modulated, in part, by the effects of androgen (Card et al. Citation2006).
In comparison, in response to chronic smoke exposure, increased oxidative stress and small airway disease were more prominent in female mice as compared to in male mice; this effect was reduced in a setting of estrogen receptor antagonism (Tam et al. Citation2016). In regard to bone quality, female C57BL/6 mice have decreased BMD and volume compared to male counterparts, and female mice were more susceptible to age-related declines in bone volume and trabecular bone loss as compared to males (Glatt et al. Citation2007; Delahunty et al. Citation2009; Pashuck et al. Citation2009). Further, female cystic fibrosis mice had increased losses in trabecular bone volume and number as compared to male counterparts (Pashuck et al. Citation2009).
Until now, the influence of sex differences on lung inflammation and bone deterioration consequences have not been investigated. Accordingly, the aim of this study was to investigate the role of sex as a biological variable in mediating LPS-induced airway inflammation and subsequent bone loss and deterioration in mice. LPS was the focus of study here in that it is ubiquitous in the environment and a driver of inflammatory consequences in many occupations, such as agriculture and textile manufacturing/processing. Although we hypothesized that female mice would be more susceptible to repetitive inhalant LPS exposure-induced bone deterioration, the present results demonstrated male mice were in fact more susceptible while females appeared to be protected from this effect. This could be partly explained by female sex hormones, and more specifically, estrogen. Male mice also had exaggerated airway inflammatory responses to LPS exposures. The results of these studies might help inform the design of future preventative and/or therapeutic preclinical studies.
Materials and methods
Animals
Male and female C57BL/6 mice were simultaneously purchased from Jackson Laboratory (Bar Harbor, ME) at 8-week-of-age. All mice were housed in specific pathogen-free facilities maintained at 20–24 °C with a 40% relative humidity and a 12-h light/dark cycle. All mice had ad libitum access to standard rodent chow and filtered tap water. After 1 week of acclimation, mice were utilized in the experimental procedures outlined below. All procedures were approved by the Institutional Animal Care and Use Committee at the University of Nebraska Medical Center and were in accordance with the NIH guidelines for the use of rodents.
Exposure model
An established intranasal (IN) inhalation exposure animal model (Poole et al. Citation2009) was used whereby mice were lightly sedated under isoflurane and then received daily treatment with 30 µl sterile saline (PBS; phosphate-buffered saline, pH 7.4) or LPS (100 ng) from Escherichia coli (Type O55:B5; Sigma, St. Louis, MO) for 3 weeks (weekends excluded). Commercially available LPS was used as the inflammatory agent to induce lung-bone consequences due to its availability to other researchers to enhance rigor, transparency, and reproducibility. Mice were euthanized (anesthetizing with isoflurane and then cervical dislocation) 5 h following their final treatment. No mice exhibited respiratory distress or weight loss (weight recorded daily) throughout the treatment period.
Serum
At euthanization, whole blood was collected from the axillary artery, placed in BD Microtainer Tubes (Becton-Dickinson, Franklin Lakes, NJ), centrifuged, and cell-free serum collected. To assess systemic responses, murine acute phase proteins that also serve as soluble pattern recognition receptors including serum pentraxin-1 (C-reactive protein), pentraxin-2 (serum amyloid P; recognizes LPS), and pentraxin-3 (TNF-inducible gene 14 protein) were quantified using Quantikine ELISA kits (R&D Systems, Minneapolis, MN) according to manufacturer instruction. The kits had sensitivities of 0.156, 1.56, and 0.23 ng/ml, respectively.
Bronchoalveolar lavage fluid neutrophil and cytokine/chemokine analysis
After the blood collection, the lungs of each mouse were lavaged using 3 × 1 ml PBS washes and bronchoalveolar lavage fluid collected (Poole et al. Citation2009). Each lavage was centrifuged; the supernatant of the first was isolated for use in analyses of tumor necrosis factor (TNF)-α, interleukin (IL)-6, and murine neutrophil chemoattractants CXCL1 and CXCL2 levels. These particular analyses were done using were ELISA kits (R&D Systems, Minneapolis, MN) with sensitivities of 7.2, 1.8, 2.0, and 1.5 pg/ml, respectively, as these cytokines/chemokines have been consistently associated with organic dust-induced airway disease (Poole et al. Citation2009) The remaining cell pellets from each lavage were resuspended and then pooled, and total cell numbers then enumerated: neutrophil and macrophage levels were determined from cytospin-prepared slides (cytocentrifuge; ELITechGroup, Logan, UT) stained with DiffQuick (Siemens, Newark, DE).
Histopathology
Following lavage, each whole lung was excised and slowly inflated (15 cm H2O pressure) with 10% of formalin (Sigma) for 24 h to preserve pulmonary architecture (Poole et al. Citation2009). The fixed lungs were then processed, embedded in paraffin, and entire sections cut (4–5 μm) and then stained with hematoxylin and eosin (H&E). Slides were reviewed at all scanning magnifications and semiquantitatively assessed for degree/distribution of lung inflammation by a pathologist (BJS) blinded to the treatment conditions, utilizing a previously published scoring system (Poole et al. Citation2009). This system evaluates spectra of inflammatory changes for both alveolar and bron-chiolar compartment inflammation. Each parameter was independently assigned a value from 0 to 3, with a higher score indicating greater inflammatory changes.
Micro-computed tomography (CT) analysis of tibias
Right hind limbs from each mouse were excised, processed, and prepared for micro-CT scanning and analysis (Dusad et al. Citation2013, Citation2015; Poole et al. Citation2015). In brief, the proximal tibia was scanned using high-resolution micro-CT (Skyscan 1172; Skyscan, Aartselaar, Belgium) with images acquired at a resolution of 6.07 µm, source set at 48 kV and 187 µ with 0.5-mm-thick aluminum filter with an exposure time of 620 ms. Scanning performed at 0.4° intervals, and six average frames were obtained for each rotation. NRecon (Skyscan) software was used to reconstruct scanned images with analysis of stacked reconstructed images using CTAn (Skyscan) software (Poole et al. Citation2015). To ensure proper orientation, growth plates were identified as the reference point and tibial position was corrected using Skyscan Dataviewer. Analysis started at 75 slices distal to the reference point, and mineralized cartilage was excluded from analysis. Final analysis was conducted on an interpolated region of interest (manually drawn to exclude the cortical shell) from a volume of interest of 1.82 mm distance (300 × 6.07 µm; 300 slides). CT-Vox and CT-Vol software (Skyscan) were used to construct 3D images.
Standard 3D parameters were measured for the trabecular bone in the proximal tibial metaphysis: BMD, BV/TV (bone volume to tissue volume ratio), BS/BV (bone surface to bone volume ratio), trabecular thickness, trabecular number, trabecular pattern factor (measure of bone structural connectivity), and polar moment of inertia (www.skyscan.be). BMD, BV/TV, trabecular thickness, and trabecular number decrease with quanti-tative bone loss, whereas trabecular separation, BS/BV, and trabecular pattern factor increase with bone deterioration. Polar moment of inertia represents the geometric index of bone strength to resist torsion, with lower values suggestive of lower bone quality (Bellido et al. Citation2010).
Phenotyping bone marrow for osteoclast precursor (OCP) populations
Bone marrow cells from the long bone of each hind limb (i.e. femur, tibia) were flushed with 10 ml sterile PBS and passed through a 70-µM pore nylon mesh (Thermo Fisher Scientific Waltham, MA) to remove any large fragments. Red blood cells in the resulting suspension were lysed by briefly suspending the cells in cold sterile water and subsequent re-suspension in PBS. Following centrifugation, remaining cells were re-suspended in 0.1% BSA/PBS solution for staining with a LIVE/DEAD Fixable Violet Dead Cell Stain kit (Life Technologies, Carlsbad, CA) to assess viability. The data indicated no differences in cell viability between groups (data not shown). After washing, bone marrow cells from each mouse were stained with monoclonal antibodies (mAb) directed against T-cell lineage (CD3), B-cell lineage (B220/CD45R), monocyte/macrophage lineage (Mac-1/CD11b), and against markers of progenitor cells: (c-fms/CD115, c-kit/CD117, and CD27) (BD Biosciences, San Jose, CA). Parallel cell preparations were treated with appropriate isotype control mAb. Compensation was performed with antibody capture beads (eBiosciences, San Diego, CA) stained separately with each individual mAb used in test samples.
The gating strategy for OCP populations utilized several published, stepwise approaches to define bone marrow OCPs (Jacquin et al. Citation2006; Jacome-Galarza et al. Citation2011, Citation2014; Xiao et al. Citation2013). After exclusion of debris and dead cells, initial gating was on triple negative (TN: CD45R-, CD3-, CD11blo) cell population because most of the osteoclastogenic activity of total bone marrow resides in the TN fraction. Next, CD115 (receptor for M-CSF) and CD117 (c-kit; receptor for stem cell factor) were utilized to further dissect the TN population. Both are markers of early hematopoietic lineage as well as OCPs. Jacquin et al. (Citation2006) showed that most early osteoclastogenic activity of the TN bone marrow fraction was contained in the TN CD115+CD117+ population. Xiao et al. (Citation2013) subsequently showed CD27 expression on TN CD115+CD117+ populations further discriminated cells that were highly enriched for osteo-clastogenic potential.
Ovariectomized (Ovx) and estradiol supplementation studies
In separate proof-of-concept studies, female mice were randomly assigned to sham surgery, ovariectomy (Ovx), or Ovx + estradiol (E2) supplementation to test the hypothesis that estrogen was bone protective against inhalant LPS-induced bone loss. A small skin and muscle incision on the dorsomedial/lateral side of the rib cage was made in the sham and Ovx groups, and extrusion of the ovary and oviduct, ligation of the ovarian artery, and section of the ovary was conducted only in the Ovx animals, which was followed by closure of muscle and skin layers in all female groups. Animals were allowed a 10-d recovery, and then an estradiol (E2) 60-d slow release pellet (0.72 mg/pellet at 12 μg/d; Innovative Research of America, Sarasota, FL) was implanted in the lateral side of the neck between the ear and shoulder by trocar of a subgroup of Ovx female animals and a subgroup of male mice. Uterine water retention following estrogen replacement was used to validate estrogen effect. After a 7-d recovery, the three groups of female animals plus the two separate groups of equivalent-aged male mice (with and without E2 implantation) were treated with intranasal inhalation of LPS (100 ng) daily for 3 weeks as above.
Statistical methods
Data are presented as the mean ± SEM, unless otherwise indicated. To detect significant changes between three or more groups, a one-way analysis of variance (ANOVA) was utilized and a Mann–Whitney nonparametric test was applied if significant differences existed between two groups. Statistical analyses were performed using SPSS software (SPSS, Chicago, IL). Statistical significance was accepted at p < 0.05.
Results
Weights differed between male and female mice
All mice gained weight during the 3 weeks of LPS inhalation. Male mice started off weighing more than female mice. Mean weights (N = 8 mice/group from two independent studies) at initiation and completion of studies were: (1) saline-treated male mice: 23.07 [± 1.70] and 24.81 [± 1.70] g (4.5% gain); (2) LPS-treated male mice: 22.59 [± 1.14] and 24.13 [±1.35] g (6.4% gain); (3) saline-treated female mice: 18.50 [± 0.88] and 18.91 [± 0.85] g (2.2% gain); and (4) LPS-treated female mice: 17.88 [± 0.62] and 18.85 [± 0.49] g (5.4% gain).
Airway inflammatory cell influx and cytokine/chemokine response following repetitive LPS exposure was reduced in female mice
LPS inhalation induced neutrophil influx and release of pro-inflammatory mediators in both male and female mice intranasally treated daily for 3 weeks as compared to in saline controls (). There were no differences in total cell or macrophage influx between LPS-treated male and female mice, but there was a significant (p = 0.03) reduction in LPS-induced neutrophil influx in female mice as compared to in males (). There were also signifi-cant reductions in LPS-induced IL-6 (p < 0.05) and neutrophil chemoattractants CXCL1 and CXCL2 (p < 0.01) in female mice as compared to in males.
Figure 1. Airway inflammatory cell influx and cytokine/chemokine response following repetitive LPS exposure is reduced in female mice. Male and female mice received intranasal inhalation of saline or LPS (100 ng) daily for 3 weeks and bronchoalveolar lavage fluid was collected 5 h following final exposure. Bar graphs depict mean (±SE) of (A) total cells, neutrophils, and macrophages, and (B) cytokine/chemokine levels. N = 8 mice/treatment group from two independent experiments. Significant difference (*p < 0.05, **p < 0.01, ***p < 0.001) vs. matched saline; significant differences between male and female denoted by line (#p < 0.05, ##p < 0.01).
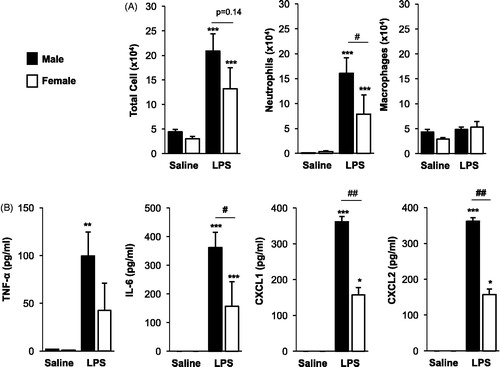
Repetitive LPS-induced lung pathology was mildly reduced in female mice
Consistent with previous studies in male mice, lung histology was notable for increased (vs. saline) inflammatory cells in the alveolar and bronchiolar compartments following intranasal LPS inhalation in both male and female mice (). Microscopy revealed the degree and distribution of inflammation was reduced in LPS-treated female mice as compared to in LPS-treated males. Semi-quantitative assessments showed LPS-induced parenchymal inflammation was reduced in both the alveolar and bronchiolar compartments in female mice as compared to males, although this sex-difference achieved statistical significance (p < 0.05) only with respect to bronchiolar inflammation ().
Figure 2. Repetitive LPS exposure-induced lung parenchymal cellular influx is mildly increased in male mice. Male and female mice received intranasal inhalation of saline or LPS (100 ng) daily for 3 weeks and whole lung sections (4–5 μm) were stained with hematoxylin and eosin. (A) Representative lung section from each treatment group is shown at 10× magnification. Arrows indicate inflammatory cell infiltrates. (B) Bar graph depicts means (±SE) of semi-quantitative degree and distribution of alveolar and bronchiolar compartment inflammation. Line scale is 100 μm. N = 8 mice/treatment group from two independent experiments. Significant difference (*p < 0.05, **p < 0.01, ***p < 0.001) vs. matched saline; significant differences between male and female denoted by line (#p < 0.05).
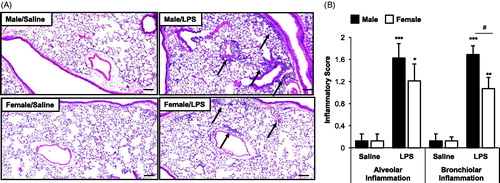
Inhalant LPS-induced trabecular bone loss demonstrated in male but not female mice
Repetitive LPS inhalation induced trabecular bone loss in male mice as shown in 3D-reconstructed CT images (). As compared to saline control, parameters describing both bone quantity and quality demonstrated that there was loss of BMD, BV/TV, trabecular thickness, trabecular number, and mean polar moment of inertia in LPS-treated male mice (). Correspondingly, bone deterioration measurements of increased levels of trabecular pattern factor and BS/BV in LPS-treated male mice as compared to saline control were observed. There were significant differences in the bone quantity and quality between male and female saline control animals (). Namely, female saline control mice had significant reduced BMD, BV/TV, trabecular thickness, trabecular numbers, and mean polar moment of inertia with increased BS/BV and trabecular pattern factor parameters as compared to their saline control male counterparts (). However, in contrast to the impact of LPS-induced bone deterioration findings in the male mice, there was no difference between saline and LPS-treated female mice ().
Figure 3. Male, but not female, mice were susceptible to the systemic bone deterioration response following repetitive LPS inhalation exposure by micro-CT imaging and analysis. (A) A representative three-dimensional (3D) reconstructed image from region of interest of proximal tibia from one mouse per treatment group (eight mice/group for two independent studies). (B) Trabecular bone of proximal tibia was analyzed for bone parameters by micro-CT analysis. Bar graphs depict means (± SE) of these bone parameters from eight mice/treatment group from two independent studies. Note that saline-treated (control) female animals have significant differences (##p < 0.01, ###p < 0.001) compared to male control mice. Statistical difference in LPS-induced changes (*p < 0.05, **p < 0.01, ***p < 0.001) only demonstrated in male mice as compared to saline.
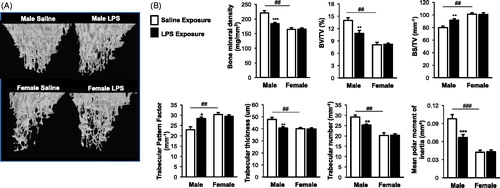
No sex differences demonstrated with inhalant LPS exposure-induced bone marrow OCP populations
To determine if repetitive inhalant LPS exposure and sex differences impacted OCP populations as an explanation for bone loss differences, bone marrow OCPs were identified by flow cytometry (). The data indicated there were no differences in frequency of OCP populations between saline-treated male and female mice (). There was a significant increase in the frequency of triple negative (TN) CD115+CD117+ (p < 0.001) and TN CD115+CD117+CD27+ (p < 0.01) bone marrow OCP populations in both male and female LPS-treated mice as compared to in saline control counterparts (). However, there was no difference in OCP populations between male and female LPS-treated animals.
Figure 4. Repetitive inhalant LPS exposures increase osteoclast precursor populations with no sex differences demonstrated. Female and male mice were in treated with saline or LPS (100 ng) daily for 3 weeks, then euthanized and bone marrow cells were collected and analyzed by flow cytometry. After exclusion of debris and dead cells, triple negative (TN) cells were gated based upon CD45R-CD3-CD11blo phenotype. TN cells were gated based on CD115, CD117, and CD27 expression. Osteoclast precursor (OCP) populations defined as frequency of TN cells expressing CD115 and CD117 and TN cells expression CD115, CD117, and CD27. (A) A representative contour plot of OCPs from saline and LPS-treated animal are shown. (B) Bar graphs depict the mean percentage of OCP populations as normalized to live bone marrow cells with standard error bars. N = 6 mice/group from two independent studies. ANOVA with Mann–Whitney nonparametric test. Significant difference vs. respective saline is denoted (**p < 0.01, **p < 0.001).
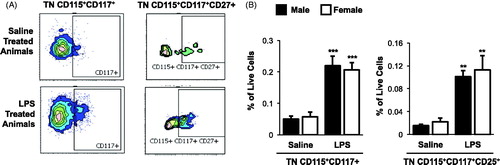
Serum pentraxins were impacted by sex differences and LPS inhalation treatments
Serum pentraxins (acute phase proteins) were measured to assess systemic inflammatory responses (). The results showed there was no difference in serum pentraxin-1 (C-reactive protein) concentration between male and female mice (within treatment) or between saline and LPS treatment groups (within gender). In contrast, there was a striking sex difference in pentraxin-2 (serum amyloid P) levels of saline control animals marked by significant (p < 0.001) increased pentraxin-2 levels in female mice (mean ± SEM: 3.05 [± 0.39] μg/ml) as compared to in males (0.56 [± 0.09] μg/ml). Pentraxin-2 levels were significantly increased in male mice who had undergone repetitive inhalation of LPS as compared to saline (p < 0.01) and there was a nonsignificant (p = 0.09) increase in female mice treated with LPS as compared to saline (). There was also a slight but significant increase in serum pentraxin-3 levels in control female mice (40.95 [± 1.72] ng/ml) as compared to in control male mice (35.00 [± 1.11] ng/ml). There was no increase in pentraxin-3 levels in male or female mice treated with LPS ().
Figure 5. Systemic response of serum pentraxins 1, 2, 3 (acute phase proteins) in male and female mice treated with inhalant LPS. Bar graphs depict mean with standard error bars of serum pentraxin levels in treatment groups. Significant difference denoted by line as *p < 0.05, **p < 0.01, and ***p < 0.001. Nonsignificant p values shown when p > 0.05 between groups. N = 8 mice/group from two independent studies.
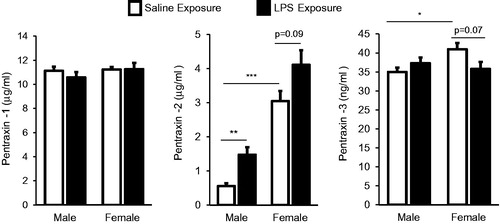
Potential role of female sex hormones in mediating protection against inhalant LPS-induced bone loss
In these proof-of-concept studies, female Ovx mice were found to be susceptible to LPS-induced bone deterioration as compared to sham (non-Ovx) female mice (). Specifically, there was significant loss of BMD, BV/TV, trabecular thickness, trabecular number and mean moment of inertia with increased BS/TV in LPS-treated Ovx female mice as compared to LPS-treated sham females. However, there were no statistical differences in weight, airway neutrophil influx, cytokine/chemokine levels, lung bronchiolar and alveolar inflammatory score, or serum pentraxin-2 levels between LPS-treated Ovx and non-Ovx (sham) female mice (). These findings suggest that female sex hormones protect bone quantity and quality following inhalant LPS-induced airway inflammation. Because estrogen is well recognized to play important roles in bone physiology with it major effect to prevent bone resorption, a subgroup of female Ovx mice were treated with supplemental estradiol (E2) in side-by-side studies. There were trends toward increased airway neutrophil influx (p = 0.18) and bronchiolar inflammation (p = 0.07) in LPS-treated Ovx + E2 female mice as compared to LPS-treated sham female mice. There was an increase in serum pentraxin-2 in the female LPS + Ovx + E2 (4.53 ± 0.68 × 103 ng/ml) as compared to LPS-treated sham females (2.09 ± 0.18 × 103 ng/ml; p < 0.05). The impact of the implanted E2 on the trabecular bones (tibia) of female Ovx + LPS mice was robust with marked increases in BMD, BV/TV, trabecular number and mean moment inertia in LPS-treated Ovx + E2 as compared to LPS-treated sham and Ovx female mice. Correspondingly, BS/TV was reduced, and there were no differences in trabecular thickness.
Table 1. A role for female sex hormone in modulating inhalant LPS-induced bone deterioration.
In side-by-side studies with the aforementioned studies using female animals, male mice were treated with LPS or E2 + LPS (). There was no difference in airway neutrophil, cytokine/chemokine levels or bronchiolar and alveolar inflammation between treatment groups. However, serum pentraxin-2 levels were significantly increased in LPS + E2 treated male mice (7.11 ± 0.9 × 103 ng/ml) as compared to LPS-treated male mice without E2 (0.98 ± 0.16 × 103 ng/ml). The impact of the implanted E2 on the LPS-treated male bones was consistent with the findings demonstrated in the female mice in that there was a strong and overcompensated protective response marked by an increase in BMD, BV/TV, trabecular number, and mean moment and correspondingly reduction in BS/TV as compared to LPS-treated male mice without implanted E2 ().
Discussion
In this study, inhalant LPS challenges given daily for 3 weeks resulted in airway neutrophil influx, inflammatory mediator release, and lung inflammatory infiltrates that were exaggerated in male mice as compared to female mice. In contrast, saline control female mice had significantly reduced BMD, bone volume, trabecular thickness, trabecular number, and bone strength to resist torsion with increased specific bone surface and trabecular pattern factor as compared to male mice. Interestingly, female animals were completely protected against inhalant LPS-induced bone deterioration. In contrast, male mice demonstrated susceptibility to LPS-induced adverse changes in bone quantity and quality parameters. Importantly, these sex-based differences did not appear to be mediated by bone marrow OCP cells nor did it appear to be driven by LPS-induced differences in acute phase responses as the serum markers of inflammation did not correspond to bone deterioration parameters. Removal of ovaries resulted in susceptibility of female mice to inhalant LPS-induced bone deterioration while supplemental estrogen reversed this responsiveness. These data provide important insight regarding the influence of sex as a biological variable to help guide future preclinical studies examining the airway inflammatory-bone deterioration disease axis.
In the first animal model description of a lung inflammatory-bone loss paradigm, the inhalant environmental inflammatory agents were LPS, Gram-positive peptidoglycan, and complex organic dust extract as collected and prepared from local swine confinement facilities (Dusad et al. Citation2013). This initial work demonstrated that all three agents induced neutrophilic airway disease with resultant loss of bone quantity and quality, and were conducted solely in male mice. In general, agriculture work has been predominately a male occupation in the USA. A recent 2017 study reported that only 11% of dairy workers residing in Colorado were women with the majority in this occupation composed of nonsmoking, Hispanic men aged 25–45 years (Davidson et al. Citation2018). In general, osteoporosis in men continues to be underdiagnosed and untreated (Mazokopakis and Starakis Citation2011), and it is estimated that one in eight men older than 50 years will experience an osteoporotic fracture (Ringe et al. Citation2006). Osteoporotic fractures are also associated with greater morbidity and mortality in men compared with women (Ringe et al. Citation2006; Mazokopakis and Starakis Citation2011). Based on our current studies that confirm earlier work (Dusad et al. Citation2013) that male mice are susceptible to inhalant LPS-induced bone deterioration as well as work by others that male mice have an increased airway inflammatory response to LPS (Card et al. Citation2006), increased attention is needed to protect at-risk workers from both the airway and skeletal health consequences in high LPS exposure occupational settings.
Bone homeostasis is regulated through activities of osteoblasts (bone forming cells) and osteoclasts (bone-resorbing cells), and moreover, osteoclasts are myeloid-derived cells that can be activated by inflammatory mediators. Previously, we demonstrated that inhalant swine confinement organic dust extract exposure induced bone marrow OCP populations that was dependent upon systemic IL-6 (Wells et al. Citation2017), and in this study, osteoclast progenitor cells were also increased following repetitive inhalant LPS exposures. However, there was no difference between male and female mice in these precursor cell populations. There was also no detection of serum inflammatory mediators including IL-6, TNF-α, and IL-17 following repetitive LPS inhalation (data not shown). Therefore, pentraxins were analyzed as markers of an activated systemic inflammatory response.
Pentraxins are a family of evolutionarily conserved proteins, also referred to as acute phase proteins, which respond to and regulate systemic inflammatory responses (Cox et al. Citation2014). Inhalant LPS exposures and sex differences did not impact serum pentraxin-1 (C-reactive protein) and only a subtle effect was found for pentraxin-3 levels. However, there were striking differences demonstrated for pentraxin-2, which is also referred to as serum amyloid P. Namely, saline control female animals demonstrated an approximate six-fold increase in pentraxin-2 levels as compared to male mice, and inhalant LPS exposures increased pentraxin-2 levels in male and female mice, but only reached statistical significance in males (). This finding may be important because pentraxin-2 binds to LPS and is a regulator of neutrophil accumulation in tissues, cytokine and complement activation, and changes in adhesion receptors (Cox et al. Citation2014). Recombinant pentraxin-2 is also under investigation for its potential to reduce disease in idiopathic pulmonary fibrosis (van den Blink et al. Citation2016). Interestingly, similar sex differences in baseline serum pentraxin-2 levels have been previously demonstrated for Syrian hamsters (Rudnick and Dowton Citation1993) and rats (Hashimoto et al. Citation1995), which contradicts observations in humans. In humans, pentraxin-2/serum amyloid P levels are lower in females compared to males, and estrogen supplementation in post-menopausal females led to reduction in these levels (Hashimoto et al. Citation1997). Collectively, these observations support a sex-specific role for pentraxin-2 in potentially regulating the systemic inflammatory response to LPS exposures, but underscores limitations in the interpretation and application of rodent sex difference investigations.
Age has already been established as an important factor in regulating the lung-bone inflammatory axis in male mice. Older C57BL/6 male mice (age 12–14 months) had dramatically reduced BMD (31% reduction), bone volume (59% reduction), trabecular number (67% reduction), and bone strength to resist torsion (72% reduction) as compared to younger counterparts (age 7–9 weeks) in the absence of airway inflammatory challenges (Poole et al. Citation2015), which is consistent with the known influence of age and bone health in mice (Ferguson et al. Citation2003). In the setting of repetitive airway inflammatory challenges with swine confinement facility organic dust extract, there was no further impact on bone deterioration parameters (with the exception of trabecular thickness) in the older mice (Poole et al. Citation2015). Based upon these age-driven differences in bone quantity and quality and that reproductive senescence is usually complete by 12 months of age in mice (Diaz Brinton Citation2012), the widely used ovariectomy (Ovx) approach to model human menopause was utilized to broadly gain insight into the role of female sex hormones/menopause in the lung-bone inflammatory axis. Indeed, the absence of female sex hormones (Ovx treatment group) increased the susceptibility of female mice to the enhanced bone deterioration consequences following repetitive inhalant LPS exposures. This suggests that an ovariectomy approach could be utilized in predictive translational models of human meno-pause to address bone consequences following inhalation exposures, and that there could be increased risk of airway inflammatory disease-associated bone loss in post-menopausal women.
It is well-established that estrogen is bone protective through its role in estrogen receptor (ER)-α and ER-β in osteoclast apoptosis, leading to attenuation in endocortical and trabecular bone resorption (Manolagas et al. Citation2013; Pavone et al. Citation2017). In those proof-of-concept studies, estrogen supplementation reversed the bone deterioration in both LPS-exposed Ovx female mice and in male mice. Ligand-activation of ER-α can lead to binding to p50 and p65 subunits of NF-κΒ, which in turn prevents them from binding to their response elements and so impact immune responses. Moreover, ligand-activated ER-α dimers can also attach to estrogen response elements on DNA and activate or repress gene transcription (Manolagas et al. Citation2013). Future studies could exploit the ER signaling pathways to impact immune responses. The over-compensated response likely reflects the supra-physiologic dosing used in these experiments. However, future “dose-finding” studies are likely to be of limited clinical value as estrogen replacement is not recommended for osteoporosis treatment in post-menopausal women because of its potential increased risk for venous thromboembolic disorders, cardiac events, stroke, and breast and endometrial cancer (Qaseem et al. Citation2017). Furthermore, estrogen has been implicated in mediating adverse respiratory health conditions in asthma and COPD (Tam et al. Citation2011; Kokturk et al. Citation2016; Warren et al. Citation2017). In this study, estrogen supplementation was also associated with nonsignificant trends toward worsening LPS-induced airway inflammatory consequences.
In conclusion, sex exerts a significant biological influence in regulating the lung inflam-matory-bone deterioration relationship following repetitive inhalant LPS exposures in a preclinical rodent model.
Acknowledgements
The authors wish to thank Lisa Chudomelka for assistance with manuscript submission, Jacqueline Pavlik and Jane DeVasure for technical assistance, and the Tissue Sciences Facility at the Department of Pathology and Microbiology (University of Nebraska Medical Center, Omaha, NE) for assistance with lung images.
Disclosure statement
The authors declare no conflicts of interest to disclose. All authors have read the journal authorship agreement and policy on disclosure of potential conflicts of interest.
Data availability statement
Data that support the findings of this study are available from the corresponding author upon reasonable request.
Additional information
Funding
References
- Bellido M, Lugo L, Roman-Blas J, Castaneda S, Caeiro J, Dapia S, Calvo E, Largo R, Herrero-Beaumont G. 2010. Subchondral bone microstructural damage by increased remodelling aggravates experimental osteoarthritis preceded by osteoporosis. Arthritis Res Ther. 12:R152.
- Card J, Carey M, Bradbury J, DeGraff L, Morgan D, Moorman M, Flake G, Zeldin D. 2006. Gender differences in murine airway responsiveness and LPS-induced inflammation. J Immunol. 177:621–630.
- Chauhan V, Ranganna K, Chauhan N, Vaid M, Kelepouris E. 2012. Bone disease in organ transplant patients: Pathogenesis and management. Postgrad Med. 124:80–90.
- Collins F, Tabak L. 2014. Policy: NIH plans to enhance reproducibility. Nature. 505:612–613.
- Cox N, Pilling D, Gomer R. 2014. Serum amyloid P: A systemic regulator of the innate immune response. J Leukoc Biol. 96:739–743.
- Davidson M, Schaeffer J, Clark M, Magzamen S, Brooks E, Keefe T, Bradford M, Roman-Muniz N, Mehaffy J, Dooley G, et al. 2018. Personal exposure of dairy workers to dust, endotoxin, muramic acid, ergosterol and ammonia on large-scale dairies in the high plains Western United States. J Occup Environ Hyg. 15:182–193.
- Delahunty K, Horton L, Coombs H, Shultz K, Svenson K, Marion M, Holick M, Beamer W, Rosen C. 2009. Gender- and compartment-specific bone loss in C57BL/6J mice: Correlation to season? J Clin Densitometry. 12:89–94.
- Diaz Brinton R. 2012. Minireview: Translational animal models of human menopause: Challenges and emerging opportunities. Endocrinology. 153:3571–3578.
- Dosman J, Lawson J, Kirychuk S, Cormier Y, Biem J, Koehncke N. 2004. Occupational asthma in newly employed workers in intensive swine confinement facilities. Eur Resp J. 24:698–702.
- Dusad A, Thiele G, Klassen L, Gleason A, Bauer C, Mikuls T, Duryee M, West W, Romberger D, Poole J. 2013. Organic dust, lipopolysaccharide, and peptidoglycan inhalant exposures result in bone loss/disease. Am J Resp Cell Mol Biol. 49:829–836.
- Dusad A, Thiele G, Klassen L, Wang D, Duryee M, Mikuls T, Staab E, Wyatt T, West W, Reynolds S, et al. 2015. Vitamin D supplementation protects against bone loss following inhalant organic dust and lipopolysaccharide exposures in mice. Immunol Res. 62:46–59.
- Eduard W, Pearce N, Douwes J. 2009. Chronic bronchitis, COPD, and lung function in farmers: The role of biological agents. Chest. 136:716–725.
- Ferguson V, Ayers R, Bateman T, Simske S. 2003. Bone development and age-related bone loss in male C57BL/6J mice. Bone. 33:387–398.
- Glatt V, Canalis E, Stadmeyer L, Bouxsein M. 2007. Age-related changes in trabecular architecture differ in female and male C57BL/6J mice. J Bone Mineral Res. 22:1197–1207.
- Graat-Verboom L, Smeenk F, van den Borne B, Spruit M, Donkers-van Rossum A, Aarts R, Wouters E. 2012. Risk factors for osteoporosis in caucasian patients with moderate chronic obstructive pulmonary disease: A case control study. Bone. 50:1234–1239.
- Hashimoto S, Kato M, Dong Y, Terada S, Inoue M. 1995. Effect of sex steroids on serum amyloid P-component (female protein) in rats. Nihon Sanka Fujinka Gakkai Zasshi. 47:1041–1047.
- Hashimoto S, Katou M, Dong Y, Murakami K, Terada S, Inoue M. 1997. Effects of hormone replacement therapy on serum amyloid P component in postmenopausal women. Maturitas. 26:113–119.
- Jacome-Galarza C, Lee S, Lorenzo J, Aguila H. 2011. Parathyroid hormone regulates the distribution and osteoclastogenic potential of hematopoietic progenitors in the bone marrow. J Bone Mineral Res. 26:1207–1216.
- Jacome-Galarza C, Soung D, Adapala N, Pickarski M, Sanjay A, Duong L, Lorenzo J, Drissi H. 2014. Altered hematopoietic stem cell and osteoclast precursor frequency in cathepsin K null mice. J Cell Biochem. 115:1449–1457.
- Jacquin C, Gran D, Lee S, Lorenzo J, Aguila H. 2006. Identification of multiple osteoclast precursor populations in murine bone marrow. J Bone Miner Res. 21:67–77.
- Jung J, Kang H, Kim J, Lee S, Kim S, Cho S. 2014. Are asthmatic patients prone to bone loss? Annals Allergy Asthma Immunol. 112:426–431.
- Kokturk N, Kilic H, Baha A, Lee S, Jones P. 2016. Sex difference in chronic obstructive lung disease. Does it matter? A concise review. COPD. 13:799–806.
- Lehouck A, Boonen S, Decramer M, Janssens W. 2011. COPD, bone metabolism, and osteoporosis. Chest. 139:648–657.
- Leon M, Beane Freeman L, Douwes J, Hoppin J, Kromhout H, Lebailly P, Nordby K. 2011. AGRICOH: A consortium of agricultural cohorts. Int J Environ Res Public Health. 8:1341–1357.
- Manolagas S, O’Brien CA, Almeida M. 2013. The role of estrogen and androgen receptors in bone health and disease. Nat Rev Endocrinol. 9:699–712.
- May S, Romberger D, Poole J. 2012. Respiratory health effects of large animal farming environments. J Toxicol Environ Health. 15:524–541.
- Mazokopakis E, Starakis I. 2011. Recommendations for diagnosis and management of osteoporosis in COPD men. ISRN Rheumatol. 2011:901416
- Merchant J, Naleway A, Svendsen R, Kelly K, Burmeister L, Stromquist A, Taylor C, Thorne P, Reynolds S, Sanderson W, et al. 2005. Asthma and farm exposures in a cohort of rural Iowa children. Environ Health Perspect. 113:350–356.
- Murphy D, Hutchinson D. 2017. Is male rheumatoid arthritis an occupational disease? A review. Open Rheumatol J. 11:88–105.
- Osborne A, Blake C, Fullen B, Meredith D, Phelan J, McNamara J, Cunningham C. 2012. Prevalence of musculoskeletal disorders among farmers: A systematic review. Am J Indust Med. 55:143–158.
- Parks C, Hoppin J, de Roos A, Costenbader K, Alavanja M, Sandler D. 2016. Rheumatoid arthritis in agricultural health study spouses: Associations with pesticides and other farm exposures. Environ Health Perspect. 124:1728–1734.
- Pashuck T, Franz S, Altman M, Wasserfall C, Atkinson M, Wronski T, Flotte T, Stalvey M. 2009. Murine model for cystic fibrosis bone disease demonstrates osteopenia and sex-related differences in bone formation. Pediatric Res. 65:311–316.
- Pavone V, Testa G, Giardina S, Vescio A, Restivo D, Sessa G. 2017. Pharmacological therapy of osteoporosis: A systematic current review of literature. Front Pharmacol. 8:803.
- Perry E, Kelly C, Eggleton P, de Soyza A, Hutchinson D. 2014. The lung in ACPA-positive rheumatoid arthritis: An initiating site of injury? Rheumatology (Oxford). 53:1940–1950.
- Poole J, Romberger D, Wyatt T, Staab E, Van De Graaff J, Thiele G, Dusad A, Klassen L, Duryee M, Mikuls T, et al. 2015. Age impacts pulmonary inflammation and systemic bone response to inhaled organic dust exposure. J Toxicol Environ Health. 78:1201–1216.
- Poole J, Thiele G, Alexis N, Burrell A, Parks C, Romberger D. 2009. Organic dust exposure alters monocyte-derived dendritic cell differentiation and maturation. Am J Physiol. 297:L767–L776.
- Poole J, Wyatt T, Oldenburg P, Elliott M, West W, Sisson J, Von Essen S, Romberger D. 2009. Intranasal organic dust exposure-induced airway adaptation response marked by persistent lung inflammation and pathology in mice. Am J Physiol. 296:L1085–L1095.
- Qaseem A, Forciea M, McLean R, Denberg T, Clinical Guideline Committee [American College of Physicians]. 2017. Treatment of low bone density or osteoporosis to prevent fractures in men and women: A clinical practice guideline update from American College of Physicians. Annals Int Med. 166:818–839.
- Ringe J, Faber H, Farahmand P, Dorst A. 2006. Efficacy of risedronate in men with primary and secondary osteoporosis: Results of a 1-year study. Rheumatology Intl. 26:427–431.
- Robertson J, Macdonald K. 2010. Prevalence of bone loss in a population with cystic fibrosis. Br J Nurs. 19:636–639.
- Rudnick C, Dowton S. 1993. Serum amyloid P (female protein) of the Syrian hamster: Gene structure and expression. J Biol Chem. 268:21760–21769.
- Staab E, Thiele G, Clarey D, Wyatt T, Romberger D, Wells A, Dusad A, Wang D, Klassen L, Mikuls T, et al. 2016. Toll-like receptor 4 signaling pathway mediates inhalant organic dust-induced bone loss. PLoS One. 11:e0158735
- Tam A, Churg A, Wright J, Zhou S, Kirby M, Coxson H, Lam S, Man S, Sin D. 2016. Sex differences in airway remodeling in a mouse model of chronic obstructive pulmonary disease. Am J Resp Crit Care Med. 193:825–834.
- Tam A, Morrish D, Wadsworth S, Dorscheid D, Man S, Sin D. 2011. The role of female hormones on lung function in chronic lung diseases. BMC Women’s Health. 11:24.
- van den Blink B, Dillingh M, Ginns L, Morrison L, Moerland M, Wijsenbeek M, Trehu E, Bartholmai B, Burggraaf J. 2016. Recombinant human pentraxin-2 therapy in patients with idiopathic pulmonary fibrosis: Safety, pharmacokinetics and exploratory efficacy. Eur Resp J. 47:889–897.
- Warren K, Sweeter J, Pavlik J, Nelson A, Devasure J, Dickinson J, Sisson J, Wyatt T, Poole J. 2017. Sex differences in activation of lung-related type 2 innate lymphoid cells in experimental asthma. Annals Allergy Asthma Immunol. 118:233–234.
- Wells A, Romberger D, Thiele G, Wyatt T, Staab E, Heires A, Klassen L, Duryee M, Mikuls T, Dusad A, et al. 2017. Systemic IL-6 effector response in mediating systemic bone loss following inhalation of organic dust. J Interferon Cytokine Res. 37:9–19.
- Xiao Y, Song J, de Vries T, Fatmawati C, Parreira D, Langenbach G, Babala N, Nolte M, Everts V, Borst J. 2013. Osteoclast precursors in murine bone marrow express CD27 and are impeded in osteoclast development by CD70 on activated immune cells. Proc Natl Acad Sci USA. 110:12385–12390.