Abstract
Sensitization to a contact allergen brings with it a lifelong risk to develop allergic contact dermatitis. Inflammation is an important part of the skin sensitizing mechanism, and understanding how different haptens stimulate the immune system, as well as the role played by different cell types present in skin, may be helpful for developing optimized in vitro models for risk assessment of new chemicals or mixtures. The aim of this study was to compare the cytokine profile following exposure of cells representing keratinocytes (HaCaT), monocytes (THP-1) and a co-culture of these cells to three clinically important skin sensitizers: cobalt (II) chloride (CoCl2), methylisothiazolinone (MI) and p-phenylenediamine (PPD). Secretion of ten pro-inflammatory cytokines was measured using multiplexing. The results showed that the cytokine response differed substantially between the three cell assays. CoCl2 caused an increase of IL-8 in HaCaT cells, while the induction of also IL-13 and IL-1β was observed in THP-1 cells and co-cultures. MI induced six cytokines in HaCaT cells but only IL-1β in the THP-1 cells and four cytokines in the co-culture. Interestingly, the IL-1β response was massive in the co-culture. PPD caused release of IL-1β in all three models as well as IL-8 in the co-culture. Control experiments with two non-sensitizers and irritants (lactic acid and sodium dodecyl sulfate) showed no effect on IL-8 or IL-1β in the co-culture. Taken together, results from this exploratory analysis show unique cytokine profiles dependent on the type of hapten and cell model. Importantly, all three haptens triggered secretion of IL-1β and IL-8 in a co-culture of HaCaT cells and THP-1 cells, representing the most robust test system.
Introduction
Induction of contact allergy (sensitization) results in lifelong susceptibility to developing allergic contact dermatitis (ACD) by subsequent skin exposure to the allergens. Epidemiological studies show that at least 20% of the general population in Europe, North America and Asia suffer from contact allergies (Alinaghi et al. Citation2019). ACD can have a serious impact on the quality of life and represents a common occupational health problem, owing particularly to hand eczema (Meding et al. Citation2005). ACD results from an overreaction of the adaptive immune system and involves two phases; the induction phase and a subsequent elicitation phase (Martin Citation2015). Patch testing with a baseline series, including metals, fragrances, preservatives, and additional substances, is used to diagnose contact allergy; but numerous other chemicals have also been shown to cause contact allergy (Johansen et al. Citation2015). Indeed, many metal ions are well known to cause ACD with cobalt (Co) being the most frequently sensitizing metal after nickel (Thyssen and Menné Citation2010; Alinaghi et al. Citation2019). Among preservatives causing ACD, isothiazolinones – including methylisothiazolinone (MI), are frequently and abundantly found in cosmetics and non-cosmetic products (Lidén et al. Citation2016; Schwensen et al. Citation2017). Several aromatic amine precursors found in hair dyes, such as p-phenylenediamine (PPD), are known to cause ACD (Lidén et al. Citation2016; Alinaghi et al. Citation2019; Ferguson et al. Citation2019).
Traditionally, animal models such as the mouse local lymph node assay (LLNA) have been used to identify skin sensitizers (Patlewicz et al. Citation2016). However, the European Union (EU) has since 2013 banned animal testing of cosmetic ingredients (EC Citation2013). There is, in general, a need to develop in vitro assays that can replace, reduce, and refine (3Rs) skin sensitization safety assessment (EURL-ECVAM Citation2015). The skin sensitization process can be described in the form of key events (KE) of an adverse outcome pathway (AOP) (OECD Citation2012). These KE are skin protein reactivity, activation of keratinocytes, activation of dendritic cells (DC) and, finally, activation and proliferation of T-cells (OECD Citation2014).
Several in chemico and in vitro tests have been developed based on the skin sensitization KE (Petry et al. Citation2017). The currently formally-validated and -adopted in vitro skin sensitization assays commonly use two cell types, keratinocytes (HaCaT) or the monocytic leukemia cell line (THP-1). These assays are based on activation of Keap1-Nrf2-ARE pathway in keratinocytes (KeratinoSens assay), analysis of surface markers (CD54 and CD86) on exposed THP-1 cells (human cell line activation test, h‐CLAT), and assessment of interleukin (IL)-8 induction using a reporter cell line based on THP-1 cells (Interleukin-8 receptor gene assay, IL-8 Luc) (OECD Citation2018a,b). Besides the already fully-validated tests, there are several other methods at different stages of development and acceptance (Bergal et al. Citation2020). Several of these are based on gene expression analysis either using a dendritic-like cell model (MUTZ-3) as in the GARD® (Genomic Allergen Rapid Detection) assay (Gradin et al. Citation2020) or using Reconstituted human Epidermis (RhE) models as in the SENS-IS assay (Cottrez et al. Citation2015), the EpiSensA (Saito et al. Citation2013), and RhE IL-18 potency test (Gibbs et al. Citation2013).
In ACD, the ability of a chemical to cause inflammation is an integral part of the mechanisms which lead to sensitization. These include the capacity to either directly or indirectly (via release of endogenous danger signals) stimulate innate pattern recognition receptors, followed by transcription of pro-inflammatory cytokines and inflammasome activation (Kaplan et al. Citation2012). Both keratinocytes (Roggen Citation2014) and immune cells have a capacity to produce various inflammatory cytokines related to ACD (Kim et al. Citation2019). Indeed, several studies have reported a release of pro-inflammatory cytokines by keratinocytes in response to different categories of skin sensitizers (Corsini et al. Citation2009; He et al. Citation2012; Galbiati et al. Citation2014, Citation2017; Chung et al. Citation2018; Jeon et al. Citation2019). However, none of the studies have investigated a larger repertoire of secreted cytokines. Further, the interplay between keratinocytes and immune cells is of interest and should be further elucidated. Recently, co-culture assays of HaCaT and THP-1 cells were developed (Hennen and Blomeke Citation2017; Eskes et al. Citation2019) and phenotypic biomarkers (e.g. CD86, CD54) on THP-1 were assessed similar to as in the h-CLAT assay.
Using both mono-cultures and co-cultures of HaCaT and THP-1 cells, the study reported here explored the immune response (at the level of cytokines) to well-known skin sensitizers representing different types of haptens. The aim was to compare cytokine profiles following exposure of cells representing keratinocytes (HaCaT), monocytes (THP-1) and a co-culture of these cells to three clinically important skin sensitizers: CoCl2, MI, and PPD. For this, a quantitative and relatively high-throughput technique for cytokine profiling was employed to ensure that the resulting data could be validated under standardized testing conditions.
Materials and methods
Chemicals and reagents
Cobalt chloride hexahydrate (CoCl2*6H2O; purity 99%, CAS# 7646-79-9), methylisothiazolinone (MI, purity 95%, CAS# 2682-20-4), and p-phenylenediamine (PPD, purity ≥ 99%, CAS# 106-50-3) were selected since they are strong or extreme sensitizers (according to LLNA) and are also clinically important. In addition, two controls were tested: lactic acid (LA, purity ≥ 85%, CAS #50-21-5, non-sensitizing and skin irritant) and sodium dodecyl sulfate (SDS, purity ≥ 99%, CAS #151-21-3, non-sensitizing in humans, but false-positive in the LLNA, and skin irritant). Chemicals at the highest purity available were purchased from Sigma Aldrich Chemie Gmbh (Munich, Germany). The maximum solubility of each chemical was first identified by dissolving in phosphate-buffered saline (PBS, pH 7.4).
Cell culture medium (RPMI-1640, Lot# 2085125), DMEM [1X] Lot# 2062193), PBS, fetal bovine serum (FBS, Lot# 42Q9079K), penicillin/streptomycin mixture, L-glutamine, and TrypLETM express were all purchased from Gibco Life Technologies (Stockholm, Sweden). Alamar blue (Lot #2119424) was obtained from Thermo Scientific (Invitrogen, Carlsbad, CA) and the pro-inflammatory panel 1 (human) (Multi-spot® 96-well plates, Lot #Z0047146) was from MSD (Solna, Sweden). The HaCaT human keratinocyte cell line was purchased from the German Cancer Research Center (DKFZ, CLS services, Heidelberg, Germany) and THP-1 cells (human monocyte leukemia cell line) from the Sigma (Stockholm).
Culturing and exposure of mono- and co-cultures
In human keratinocyte mono-culture assays, the HaCaT cells were cultured in DMEM (supplemented with 10% FBS and 1% penicillin-streptomycin) at 37 °C in a humidified incubator containing 5% CO2. As previously described (Chung et al. Citation2018), the HaCaT cells were seeded in 96-well plates at 1.2 × 104 cells/well (100 μl/well) and incubated for 24 hr. Thereafter, the medium was replaced with fresh medium containing specific concentrations of exposure chemical or vehicle control. The human monocytic THP-1 line was grown in RPMI-1640 supple-mented 10% FBS, 1% penicillin/streptomycin, and 1% L-glutamine. For the THP-1 mono-culture, cells were plated as described in Cappellini et al. (Citation2020) at 4 × 104 cells/well (80 µl) in 96-well plates and treated with 80 µl of medium containing the test chemical or vehicle control (160 µl total volume/well).
The co-culture experiments were performed according to Eskes et al. (Citation2019). HaCaT cells were first (Day 1) harvested, counted, and seeded into 96-well plates as 1.2 × 104 cells/well (100 µl). Cells were then cultivated at 37 °C for 24 h at which time they were confluent. The next (Day 2), THP-1 cells were harvested, counted, and 4 × 104 cells (in 80 µl) was added to each well on top of the HaCaT cells. After 1–2 h incubation, 80 µl of the exposure chemical (in THP-1 medium) was added to each well. The final volume/well including test chemicals was 160 µl.
The exposure protocol was identical for the mono- and co-cultures. Cells were exposed to the test chemicals along with increasing concentrations of CoCl2 (0, 10, 100, 250, 500, 750, 1000 µg/ml) and of PPD and MI (0, 10, 25, 50, 100, 250, 500 µg/ml), LA (0, 10, 100, 250, 500, 750, 1000 µg/ml), and SDS (0, 5, 10, 20, 30, 50, 60 µg/ml). Test chemicals stock solutions were freshly-prepared in PBS and diluted with culture medium (HaCaT, THP-1) prior to use.
Cell viability measurement by Alamar blue assay
An Alamar blue assay was used to determine cytotoxicity of test chemicals in the mono- and co-cultures. In brief, after 24 h of exposure, the wells of the plates each received 100 µl of 10% Alamar blue solution (Invitrogen, Carlsbad, CA USA) and then were incubated for 2 h at 37 °C. The plates were then evaluated in an Infinite F 200 microplate reader (with associated Magellan 7.2 Software; Tecan, Grodig, Austria) that read sample fluorescence at a 560 nm excitation and a 590 nm emission. Background optical density (OD) values (i.e. 10% Alamar blue in culture medium) were subtracted from each well value; no chemical background interference was observed. OD values for each concentration tested were converted to percent cell viability (%) relative to control using % = 100 × [Absorbance of treated cells/Absorbance of control]). Four independent experiments (n = 4) were performed. The concentration at which 80% viability was observed (IC20) was calculated for all three chemicals in the mono- and co-culture cell models.
Multiplex cytokine assay/cytokine measurements
To measure the secretion of inflammatory mediators, the cultures (mono- and co-culture) were exposed to the GraphPad-estimated IC20 concentration of the three chemicals (CoCl2, MI, PPD) for 24 h at 37 °C. Thereafter, cell-free culture supernatants (n = 4) were collected by centrifuging the plates at 1200 rpm for 4 min. All supernatants were stored at −80 °C until used for cytokine measurements. A MSD® multi-spot assay system pro-inflammatory panel 1 was used to analyze the following cytokines: interferon (IFN)-γ, interleukin (IL)-10, -12p70, -13, -1β, -2, -4, -6, -8, and tumor necrosis factor (TNF)-α. The MSD assay is designed for the quantitative measurement of multiple cytokines in a single well using as little as 50 μl sample. All assays were performed according to manufacturer instructions (www.mesoscale.com). All assay plates were analyzed on a MESO QuickPlex SQ 120 imager (MSD). All calibrators were measured in duplicate. The raw data was measured as electro-chemiluminescence signal (light) detected by photodetectors and analyzed using MSD Discovery Workbench® (MSD). A 4-parameter logistic fit curve was generated for each analyte using the standards and the concentration of each sample calculated. As a positive control for the MSD assay, lipopolysaccharide (LPS, from Escherichia coli Type O111:B4) was provided at 100 ng/ml for 24 h to stimulate the THP-1 cells was used.
Statistical analysis and stimulation index (SI) calculation
All Alamar blue experiments were performed at least four times (n = 4) and each concentration was in each experiment tested in three wells. Alamar blue assay results were analyzed by a one-way analysis of variance (ANOVA); p-values ≤ 0.001 were considered as statistically significant (with respect to control). For the MSD assay, samples (cell supernatants) collected from four individual 96-well plates (n = 4) were measured using one MSD plate (Pro-inflammatory panel 1). Results of that assay were analyzed by one-way ANOVA; p-values ≤ 0.05 or ≤ 0.01 were considered significantly different with respect to the control. All analyses were performed using Prism software (v.8.2.0, GraphPad, San Diego, CA).
The SI value represents the “fold-increase of a cytokine” as compared to control cells. SI values were calculated as [Inflammatory mediator (pg/ml) with chemical-treated cells]/[inflammatory mediator (pg/ml) with control cells]. The SI value indicates the quantitative numerical value of fold-change in cytokine for each chemical (CoCl2, MI, PPD) in the various cell models.
Results
Cytotoxicity of CoCl2, MI, and PPD in the different cell models (Mono- and co-cultures)
The cytotoxicity of CoCl2, MI, and PPD in the three different cell models was first assessed in an Alamar blue assay. All chemicals were initially tested at doses up to 1000 µg/ml in a screening test, after which concentrations were adjusted based on maximum cytotoxicity. The results of the Alamar blue assays were normalized to cell viability (). The decreased cell viability compared with controls was significant for all three chemicals in the concentration range tested. The toxicity in the mono-cultures was generally higher than in the co-cultures in the same exposure range; this can possibly be explained by the fact a total higher number of cells were present. The cytotoxicity for MI and PPD was higher compared against CoCl2 (). Further, particularly regarding PPD, HaCaT cells in the mono-cultures were more sensitive than THP-1 cells (or vs. co-cultures outcomes). The GraphPad dose-response curve-fitted calculated IC20 concentration (80% cell viability) was subsequently used for MSD cytokine profiling.
Figure 1. Cell viability. Viability was determined by an Alamar blue assay after 24-h exposure of three cell culture systems (HaCaT or THP-1 mono-cultures and HaCaT/THP-1 co-culture) with different concentraions (µg/ml) of CoCl2, MI, and PPD. Each bar represents mean ± SE of four experiments. Statistical analysis: one-way ANOVA or Bonferroni’s multiple comparison. Value significantly different from unexposed/control cells, ***p ≤ 0.001.
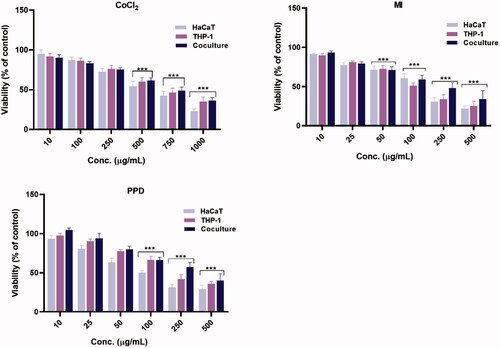
Cytokine profiles in response to CoCl2, MI and PPD in the three different cell models
Following the cytotoxicity testing, cytokine profiles in the test systems were evaluated following exposure of the three different cell models to CoCl2, MI, and PPD. The cell models were exposed to the estimated IC20 concentration (80% viability) of the three chemicals for 24 h at 37 °C and then culture supernatants were collected for cytokine measurement. The data from the cytokine expression analyses from four independent experiments (n = 4; ) revealed that concentrations of cytokines already differed between the models at a baseline level. Most strikingly, levels of IL-8 were significantly higher in supernatants of HaCaT control cells compared to in the THP-1 and co-culture controls.
Figure 2. Effect of CoCl2 on secretion of pro-inflammatory cytokines in the three culture systems. IC20 concentrations used; HaCaT= 117.5, THP-1 = 128.7 and co-culture= 101.5 µg/ml. Assessment of cytokines was performed after 24 h of exposure. Each bar (n = 4, mean ± SE) represents the concentration of the mediator (pg/ml). Statistical analysis: one-way ANOVA or Bonferroni’s multiple comparison. Value significantly different from corresponding control: *p ≤ 0.05, **p ≤ 0.01.
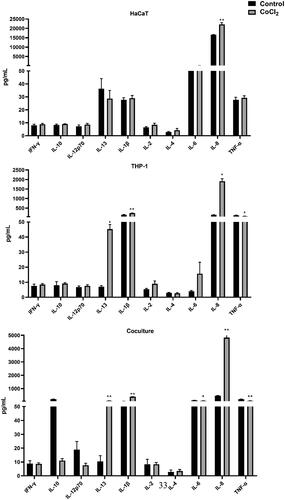
Figure 3. Effect of MI on secretion of pro-inflammatory cytokines in the three culture systems. IC20 concentrations used; HaCaT = 25.1, THP-1 = 16.5 and co-culture = 17 µg/ml. Assessment of cytokines was performed after 24 h of exposure. Each bar (n = 4, mean ± SE) represents the concentration of the mediator (pg/ml). Statistical analysis: one-way ANOVA or Bonferroni’s multiple comparison. Value significantly different from corresponding control: *p ≤ 0.05, **p ≤ 0.01.
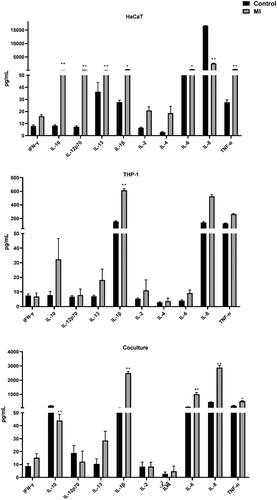
Figure 4. Effect of PPD on secretion of pro-inflammatory cytokines in the three culture systems. IC20 concentrations used; HaCaT = 15.0, THP-1 = 38.0 and co-culture= 77.7 µg/ml. Assessment of cytokines was performed after 24 h of exposure. Each bar (n = 4, mean ± SE) represents the concentration of the mediator (pg/ml). Statistical analysis: one-way ANOVA or Bonferroni’s multiple comparison. Value significantly different from corresponding control: *p ≤ 0.05, **p ≤ 0.01.
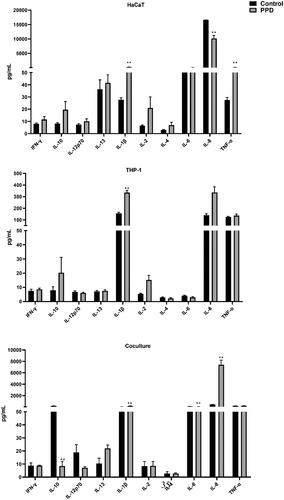
shows the effect of CoCl2 on cytokine release in the three cell models. In HaCaT cells, IL-8 was the only cytokine released at a significantly higher level compared to by controls. With the THP-1 cells there was a higher release of three mediators (IL-13, IL-8, IL-1β). Levels of these same cytokines were also greater than control values in the co-cultures. Only IL-6 and TNFα levels were slightly lower in CoCl2 exposed co-cultures when compared with controls.
Similarly, the response by the cultures to MI was also modified, depending on types of cells in the assay. indicates there was a significant effect of MI on cytokine levels in the three models. HaCaT cells were generally more responsive than THP-1 cells, releasing significantly higher levels (vs. control) of six cytokines (IL-10, -12p70, −13, −1β, −6, and TNFα); THP-1 cells released a significantly higher level of only IL-1β. Increases in IL-1β, −6, −8, and TNFα were all induced in the co-cultures. The most striking result of the MI exposure was a clear IL-1β response in all three cell models. The concentrations were particularly high with the co-cultures (average 2488 pg/ml compared with only 51 pg/ml in controls).
shows the significant effects of PPD on cytokine levels in the three models. Compared to CoCl2 and MI, the PPD response was less pronounced. As with MI, PPD induced IL-1β in all three models, though the average levels were not as high as in the MI exposures (334 vs 615 pg/ml in THP1 and 192 vs 2488 pg/ml in co-cultures). Except for IL-1β, TNFα was the only other significantly higher cytokine measured in the medium of exposed HaCaT cells, and IL-8 the lone one in the co-culture.
Comparison of stimulation index (SI) values for each cytokine in the three models
Stimulation index (SI) values, i.e. fold-increase in cytokine concentration compared to value with corresponding control, were caluculated for each chemical in the test systems. To get a good overview of the different cytokine profiles, all SI values are shown in ; a shade gradient indicates the extent of SI increase according to: SI =2.0–3.0, 3.0–10.0, and ≥ 10. Changes that reached the threshold (SI > 2) but were not statistically significant are shown in parentheses.
Table 1. Summary of cytokine responses.
First, IFNγ was not significantly induced in any cell model by any of the sensitizers tested. Changes observed for IL-2 and IL-4 were rather small with high variation and were non-significant. IL-10 and IL-12p70 were induced only by MI exposure and HaCaT cells were the most responsive cell model regarding these cytokines (SI of 9.9 and 23.0, respectively). In contrast, a clear induction of IL-13 was mainly observed after CoCl2 exposure, although a slight increase was also observed for MI and PPD. TNFα and IL-6 showed diverging results depending on the cell model and the response was most clear for MI. A universal cytokine response that could be observed after all three exposures was secretion of IL-8 and IL-1β in the co-culture. IL-8 levels were increased 10.5-, 6.3-, and 16.2-times with CoCl2, MI, and PPD, respectively. The corresponding values of IL-1β release were 7.6-, 48.7-, and 3.8-times.
Interestingly, levels of released cytokines were generally higher in the co-cultures, except for in the case of PPD-triggered IL-1β, which was most pronounced in HaCaT cells. The IL-1β response to CoCl2 was only significant in the co-culture, and the response to MI was extreme (SI = 49) in the co-culture. Similarly, only the co-culture responded clearly to PPD regarding IL-8 secretion. Taken together, this data suggests that assessment of IL-1β and IL-8 in a co-culture model could be a useful approach for detecting skin sensitizers.
Control experiments with LA and SDS
To confirm that the cytokine response observed for the strong sensitizers would not be present for typical non-sensitizers or skin irritants, additional experiments with LA (non-sensitizer and irritant) and SDS (non-sensitizer in humans but false-positive in the LLNA, and irritant) were performed using the co-culture. The data on cytotoxicity showed that LA was non-cytotoxic with an IC20 of >1000 µg/ml whereas SDS was cytotoxic with an IC20 value of 14.6 µg/ml, which is similar as the cytotoxic potency as MI. The SI values for the different cytokines, compared to non-exposed cells, are shown in . The results show no response in terms of IL-1β and IL-8 release, as observed for the sensitizers tested.
Table 2. Summary of cytokine responses to control agents.
Discussion
The study reported here explored the release of cytokines as a response to well-known skin sensitizers representing different types of haptens, with a focus on investigating how the design of the cell model may influence the outcome. The MSD® multi-spot assay was employed and the results indicated that assessment of cytokines IL-1β and IL-8 in a co-culture model gave the most robust result, in terms of a clear increase for all three haptens. However, the tested non-sensitizers and irritants did not cause such a response.
The results also illustrated the generation of unique cytokine profiles, which depended on the type of hapten, as well as on the design of the test system. Further, these studies demonstrated that all three test haptens triggered the secretion of IL-1β and IL-8 in a highly-reproducible manner in the co-cultures of HaCaT cells and THP-1 cells, and that the co-culture represented the most robust test system. Interestingly, the cytokines were more reliably induced, and released at much higher levels, in the co-culture compared to the mono-cultures. This type of outcome indicated a synergy between the two cell types. For example, release of IL-1β in response to CoCl2 was significant only in the co-culture, and the response to MI was extremely high in the co-culture compared to in either mono-culture. Moreover, only in the co-culture was IL-8 clearly released in response to PPD. It seems reasonable that a co-culture could provide advantages over a mono-culture as the former allows for inter-cellular crosstalk, including amplified immune signaling, provision of danger signals, and other support between cell types (Koppes et al. Citation2017). Moreover, inclusion of both keratinocytes and immune cells in the same assay provides an opportunity to combine assessment of multiple key events in a single assay. An assay based on co-culture may therefore offer an increased applicability domain (Thelu et al. Citation2020), in addition to potentially improving the ability to predict sensitizing potency.
Other studies have reported that co-cultures can improve the inflammation process prediction. For instance, Cao et al. (Citation2012) found – in line with the current study – that the surface expression of CD86 and CD54 on THP-1 cells after exposure to skin sensitizers was higher when the cells were cultured together with keratinocytes compared to when the THP-1 cells were alone. Alfaro-Moreno et al. (Citation2008) noted a higher release of several cytokines upon exposure to particles in co-cultures including THP-1 cells (as well as lung cells and mast cells) compared to the sum of the single cultures. Another example was that when THP-1 cells were cultured together with HaCaT cells, this strongly increased their expression of markers related to necrosis, apoptosis, and inflammation due to chemical exposure (Balszuweit et al. Citation2014). Interestingly, Galbiati et al. (Citation2020) showed recently that keratinocytes facilitated the full maturation of dendritic cells (DC) in the presence of moderate and weak allergens. For extreme allergens, complete maturation of DC was observed also in the absence of keratinocytes. One may indeed also consider a model with more than two cell types. A tri-culture model with MUTZ-3-derived Langerhans cells, HaCaT keratinocytes, and primary dermal fibroblasts has been proposed to improve the detection of pre- and pro-haptens when some cytokines (IL-8, MIP-1β, GM-CSF) were assessed as potential biomarkers for skin sensitization (Lee et al. Citation2018). Together, the data here and from the cited studies indicate that co-culture models seem promising for predicting toxicity. However, further mechanistic understanding is needed to properly forecast the complex process of skin sensitization and to standardize the readout of the test system (Thelu et al. Citation2020).
The data here regarding IL‐1β support the previous notion that inflammasome activation appears to be a feature common to all sensitizing haptens (Kaplan et al. Citation2012). IL‐1β has also been shown to be up‐regulated in the epidermis after challenge with a sensitizer in a mouse model (Shornick et al. Citation2001). Traditionally, inflammasome activation has mainly been studied in immune cells, but it is now known to be activated also in airway epithelia (Zheng et al. Citation2017; Brostrom et al. Citation2018; Ghalali et al. Citation2020) and keratinocytes (Galbiati et al. Citation2019). Inflammasome activation (Koppes et al. Citation2017) and IL‐1β secretion (Kaplan et al. Citation2012) can be initiated by toxicological stress in epithelial cells which release danger-associated molecular patterns (DAMP), such as ATP or/and autotaxin (ATX), and affect neighboring cells (Zheng et al. Citation2017; Brostrom et al. Citation2018; Ghalali et al. Citation2020).
A similar mechanism seems possible for IL-8 as well as pro- and active forms of IL-18 (Liu et al. Citation2016; Miyabe et al. Citation2019). In line with this, IL-18 is a basis for another assay proposed to assess skin sensitization potency, i.e. the in vitro reconstructed human epidermis (Rhe) 1 L-18 (Gibbs et al. Citation2013). The current study data are also in line with a recent study on THP-1 cells, suggesting that IL-1β could be a useful marker for assessing skin sensitization potency (Kim et al. Citation2019). In that study, IL-1β was assessed by Western blot, thus limiting efficient screening; further, the fold-increase in IL-1β was in general low (e.g. only ≈ 1.5 for MI) when compared to in the current study.
The chemicals in the present study were chosen to represent different types of haptens, e.g. a metal salt (CoCl2), a hydrophilic organic hapten (MI), and a pro-/pre-hapten (PPD). Metals and pro-haptens have been shown to be difficult to properly assess with in vitro assays (Gibbs et al. Citation2018); accordingly, it is of great interest to include such chemicals in the development and testing of new assays. For example, there are some difficulties in assessing PPD with the h-CLAT assay (Sakaguchi et al. Citation2006) and no increased CD86 expression was observed with peripheral blood monocyte-derived DC (de Avila et al. Citation2019).
Following exposure to CoCl2, a clear activation of IL-8 formation was found in all three cell models here. Cobalt, similarly to nickel, can interact directly with TLR4 (Toll-like receptor for LPS), leading to activation and pro-inflammatory signaling (Raghavan et al. Citation2012; Schmidt and Goebeler Citation2015). In addition, CoCl2 caused substantially increased IL-13 secretion, a T-helper cell Type 2 (TH2) cytokine that polarizes macrophages to an M2 state and plays a role in allergic lung disease in part by mediating excess mucus production and fibrosis (Gour and Wills-Karp Citation2015). IL-13 may also exacerbate contact dermatitis (Nakagomi et al. Citation2015). MI was the most potent chemical in the current study with regard to the extent of induced cytokine secretion. In particular, formation of IL-1β in the co-cultures was extremely high in response to MI. In contrast, PPD acts as pro-/pre-hapten and thus, the activation processes may influence responses to PPD in cell models (Aeby et al. Citation2009). Apart from auto-oxidation, PPD is enzymatically-acetylated to monoacetyl-PPD (MAPPD) and N,N'-diacetyl-PPD (DAPPD) in skin and keratino-cytes (Blömeke et al. Citation2008; Rustemeyer et al. Citation2020). Thus, the extent of activation may indeed impact on the ability of PPD to induce pro‐inflammatory responses. In the present study, a clear induction of IL‐1β, TNFα, and IL‐6 was observed with the PPD, which was in concordance with results reported for PPD-exposed mouse skin (Bonefeld et al. Citation2010). Here too, the most robust increase in formation of IL-8 (SI = 16.2) was observed in the co-cultures, again emphasizing the role of interaction between the keratinocytes and immune cells for a robust response.
Taken together, the results of these studies indicate the importance of a co-culture system for analyzing the ability of different types of haptens, including metals and pro/prehaptens, to activate inflammatory mediators in in vitro test models. Importantly, no response was observed to the irritant SDS suggesting that the effects are not general to cytotoxic substances. Likely, keratinocytes provide support and release a set of alarmins important for the immune cells and the cytokine signaling (Koppes et al. Citation2017). As observed for cobalt, the immune cells seemed critical for this detection since no increased cytokine secretion was observed in the HaCaT monoculture.
It should be emphasized that this was an exploratory study and the approach used was based on analysis of effects in response to an IC20 concentration at only one timepoint (24 h). This is similar as with other in vitro assays for skin sensitization (Johansson et al. Citation2013). Indeed, different responses may be observed at different timepoints and test agent concentrations. Prior to selecting the 24 h timepoint, a shorter exposure time (6 h) was also tested for the PPD and MI in the co-culture. The results suggested that at 6 h, the agents induced lower levels of IL-8 and IL-1β but higher levels of TNFα (data not shown). Thus, the time-dependency of responses by the various cytokines could be interesting to explore further. It should also be noted that the total number of cells able to respond were greater in the co-cultures; further, the IC20 concentrations used were slightly higher for the co-cultures as compared to in the mono-cultures (based on cytotoxicity). It appears, however, unlikely that these circumstances explain the much higher responses observed in the co-cultures, particularly for IL‐1β in response to MI. Possibly, the co-culture model can be combined/integrated with other validated assays to predict the sensitizing potential and potency of new substances.
Conclusions
To our knowledge, this is the first comparative in vitro study evaluating multiple cytokine profiles in mono- and co-cultures of HaCaT and THP-1 cells with well-known and clinically important strong sensitizers. The results showed there were unique cytokine profiles dependent on the type of hapten and cell model. Of note, all three haptens triggered formation of IL-1β and IL-8 in the co-cultures. This response was not observed for the non-sensitizers and irritants tested. Overall, these findings provide insight into the release of cytokines by skin sensitizers and suggest that using two cell lines as a model for testing can give more robust result.
Acknowledgments
The authors would like to thank colleagues that contributed at an early stage of the project, including Dr. Aram Ghalali, Dr. Huiyuan Zheng, and Master of Science students Maria Toma and Jie-Hyun Choi.
Disclosure statement
The authors declare no conflicts of interest. The authors alone are responsible for the content of this manuscript.
Additional information
Funding
References
- Aeby P, Sieber T, Beck H, Gerberick G, Goebel C. 2009. Skin sensitization to p-phenylenediamine: the diverging roles of oxidation and N-acetylation for dendritic cell activation and the immune response. J Invest Dermatol. 129(1):99–109.
- Alfaro-Moreno E, Nawrot T, Vanaudenaerde B, Hoylaerts M, Vanoirbeek J, Nemery B, Hoet P. 2008. Co-cultures of multiple cell types mimic pulmonary cell communication in response to urban PM10. Eur Respir J. 32(5):1184–1194.
- Alinaghi F, Bennike N, Egeberg A, Thyssen J, Johansen J. 2019. Prevalence of contact allergy in the general population: A systematic review and meta-analysis. Contact Dermatitis. 80(2):77–85.
- Balszuweit F, Menacher G, Bloemeke B, Schmidt A, Worek F, Thiermann H, Steinritz D. 2014. Development of a co-culture of keratinocytes and immune cells for in vitro investigation of cutaneous sulfur mustard toxicity. Chem Biol Interact. 223:117–124.
- Bergal M, Puginier M, Gerbeix C, Groux H, Roso A, Cottrez F, Milius A. 2020. In vitro testing strategy for assessing the skin sensitizing potential of “difficult to test” cosmetic ingredients. Toxicol in Vitro. 65:104781.
- Blömeke B, Pietzsch T, Merk H. 2008. Elicitation response characteristics to mono- and to N,N'-diacetyl-para-phenylenediamine. Contact Dermatitis. 58(6):355–358.
- Bonefeld C, Larsen J, Dabelsteen S, Geisler C, White I, Menne T, Johansen J. 2010. Consumer available permanent hair dye products cause major allergic immune activation in an animal model. Br J Dermatol. 162(1):102–107.
- Brostrom J, Ghalali A, Zheng H, Högberg J, Stenius U, Littorin M, Tinnerberg H, Broberg K. 2018. Toluene diisocyanate exposure and autotaxin-lysophosphatidic acid signalling. Toxicol Appl Pharmacol. 355:43–51.
- Cao Y, Ma P, Liu W, Zhou W, Tao Y, Zhang M, Li L, Chen Z. 2012. Evaluation of the skin sensitization potential of chemicals in THP-1/keratinocyte co-cultures. Immunopharmacol Immunotoxicol. 34(2):196–204.
- Cappellini F, Di Bucchianico S, Karri V, Latvala S, Malmlof M, Kippler M, Elihn K, Hedberg J, Odnevall Wallinder I, Gerde P, et al. 2020. Dry generation of CeO2 nanoparticles and deposition onto a co-culture of A549 and THP-1 cells in air-liquid interface – Dosimetry considerations and comparison to submerged exposure. Nanomaterials. 10(4):618.
- Chung H, Quan H, Jung D, Ravi G, Cho A, Kang M, Kim E, Che J, Lee E, Jeong T, et al. 2018. Intra- and inter-laboratory reproducibility and predictivity of the HaCaSens assay: A skin sensitization test using human keratinocytes, HaCaT. Toxicol in Vitro. 46:304–312.
- Corsini E, Mitjans M, Galbiati V, Lucchi L, Galli C, Marinovich M. 2009. Use of IL-18 production in a human keratinocyte cell line to discriminate contact sensitizers from irritants and low molecular weight respiratory allergens . Toxicol in Vitro. 23(5):789–796.
- Cottrez F, Boitel E, Auriault C, Aeby P, Groux H. 2015. Genes specifically modulated in sensitized skins allow the detection of sensitizers in a reconstructed human skin model. Development of the SENS-IS Assay. Toxicol in Vitro. 29(4):787–802.
- de Ávila RI, Veloso DF, Teixeira GC, Rodrigues T, Lindberg T, Lindstedt M, Fonseca SG, Lima EM, Valadares MC. 2019. Evaluation of in vitro testing strategies for hazard assessment of the skin sensitization potential of “real-life” mixtures: The case of henna-based hair-colouring products containing p-phenylenediamine. Contact Dermatitis. 81(3):194–209. DOI:https://doi.org/10.1111/cod.13294.
- EC 2008. Regulation (EC) No. 1272/2008 of the European Parliament and of the Council of 16 December 2008 on classification, labelling and packaging of substances and mixtures, amending and repealing directives 67/548/EEC and 1999/45/EC, and Amending Regulation (EC) No. 1907/2006. http://data.europa.eu/eli/reg/2008/1272/2020-05-01.
- EC. 2013. Commission Regulation (EU) No. 344/2013 of 4 April 2013 amending Annexes II, III, V and VI to Regulation (EC) No. 1223/2009 of the European Parliament and of the Council on Cosmetic Products Text with EEA Relevance. Chapter 13. 63:253–311. Online: http://data.europa.eu/eli/reg/2013/344/oj.
- Eskes C, Hennen J, Hoffmann S, Frey S, Goldinger Oggier D, Peter N, Schellenberger M, van Vliet E, Blomeke B. 2019. The HaCaT/THP-1 Cocultured Activation Test (COCAT) for skin sensitization: A study of intra-lab reproducibility and predictivity. Altex. 36(4):613–622.
- EURL-ECVAM 2015. EURL-ECVAM status report on the development, validation, and regulatory acceptance of alternative methods and approaches (2015). European Commission, Joint Research Centre, Institute for Health and Consumer Protection. http://publications.jrc.ec.europa.eu/repository/handle/JRC97811
- Ferguson F, Pongpairoj K, Basketter D, White I, McFadden J. 2019. Addressing the conundrums of p‐phenylenediamine hair dye allergy by applying Friedmann’s principles of contact sensitization. Contact Dermatitis. 80(4):234–237.
- Galbiati V, Cornaghi L, Papale A, Donetti E, Marinovich M, Corsini E. 2019. Study on the inflammasome Nlrp3 and Blimp-1/Nlrp12 after keratinocyte exposure to contact allergens. Toxicol Lett. 313:130–136.
- Galbiati V, Maddalon A, Iulini M, Marinovich M, Corsini E. 2020. Human keratinocytes and monocytes co-culture cell system: An important contribution for the study of moderate and weak sensitizers. Toxicol in Vitro. 68:104929.
- Galbiati V, Papale A, Galli C, Marinovich M, Corsini E. 2014. Role of ROS and HMGB1 in contact allergen-induced IL-18 production in human keratinocytes. J Invest Dermatol. 134(11):2719–2727.
- Galbiati V, Papale A, Marinovich M, Gibbs S, Roggen E, Corsini E. 2017. Development of an in vitro method to estimate the sensitization induction level of contact allergens. Toxicol Lett. 271:1–11.
- Ghalali A, Ye Z, Hogberg J, Stenius U. 2020. PTEN and PHLPP crosstalk in cancer cells and in TGFβ-activated stem cells. Biomed Pharmacother. 127:110112.
- Gibbs S, Corsini E, Spiekstra S, Galbiati V, Fuchs H, DeGeorge G, Troese M, Hayden P, Den W, Roggen E. 2013. An epidermal equivalent assay for identification and ranking potency of contact sensitizers. Toxicol Appl Pharmacol. 272(2):529–541.
- Gibbs S, Kosten I, Veldhuizen R, Spiekstra S, Corsini E, Roggen E, Rustemeyer T, Feilzer A, Kleverlaan C. 2018. Assessment of metal sensitizer potency with the reconstructed human epidermis IL-18 assay. Toxicology. 393:62–72.
- Gour N, Wills-Karp M. 2015. IL-4 and IL-13 signaling in allergic airway disease. Cytokine. 75(1):68–78.
- Gradin R, Johansson A, Forreryd A, Aaltonen E, Jerre A, Larne O, Mattson U, Johansson H. 2020. The GARD potency assay for potency-associated subclassification of chemical skin sensitizers-rationale, method development, and ring trial results of predictive performance and reproducibility. Toxicol Sci. 176(2):423–432.
- He Q, You H, Li X, Liu T, Wang P, Wang B. 2012. HMGB1 promotes the synthesis of pro-IL-1β and pro-IL-18 by activation of p38 MAPK and NF-κB through receptors for advanced glycation end-products in macrophages. Asian Pac J Cancer Prev. 13(4):1365–1370.
- Hennen J, Blomeke B. 2017. Keratinocytes improve prediction of sensitization potential and potency of chemicals with THP-1 cells. Altex. 34(2):279–288.
- Jeon B, Kim M, Kim Y, Han H, Yun J, Kim J, Huang Y, Choi Y, Cho C, Kang B, et al. 2019. Optimization and validation of a method to identify skin sensitization hazards using IL-1α and IL-6 secretion from HaCaT. Toxicol in Vitro. 61:104589
- Johansen J, Aalto-Korte K, Agner T, Andersen K, Bircher A, Bruze M, Cannavo A, Giménez-Arnau A, Gonçalo M, Goossens A, et al. 2015. European Society of Contact Dermatitis Guideline for diagnostic patch testing – recommendations on best practice. Contact Dermatitis. 73(4):195–221.
- Johansson H, Albrekt A, Borrebaeck C, Lindstedt M. 2013. The GARD assay for assessment of chemical skin sensitizers. Toxicol in Vitro. 27(3):1163–1169.
- Kaplan D, Igyarto B, Gaspari A. 2012. Early immune events in the induction of allergic contact dermatitis. Nat Rev Immunol. 12(2):114–124.
- Kim M, Kim K, Kim H, Lee B. 2019. Alternative skin sensitization prediction and risk assessment using pro-inflammatory biomarkers, interleukin-1 beta (IL-1β) and inducible nitric oxide synthase (iNOS). J Toxicol Environ Health A. 82(5):361–378.
- Koppes SA, Engebretsen KA, Agner T, Angelova-Fischer I, Berents T, Brandner J, Brans R, Clausen M-L, Hummler E, Jakasa I, et al. 2017. Current knowledge on biomarkers for contact sensitization and allergic contact dermatitis. Contact Dermatitis. 77(1):1–16. DOI:https://doi.org/10.1111/cod.12789.
- Lee H, Greenstein T, Shi L, Maguire T, Schloss R, Yarmush M. 2018. Tri-culture system for pro-hapten sensitizer identification and potency classification. Technology (Singap World Sci). 6(2):67–74.
- Lidén C, Yazar K, Johansen J, Karlberg A, Uter W, White I. 2016. Comparative sensitizing potencies of fragrances, preservatives, and hair dyes. Contact Dermatitis. 75(5):265–275.
- Liu Q, Li A, Tian Y, Wu J, Liu Y, Li T, Chen Y, Han X, Wu K. 2016. The CXCL8-CXCR1/2 pathways in cancer. Cytokine Growth Factor Rev. 31:61–71.
- Martin S. 2015. New concepts in cutaneous allergy. Contact Dermatitis. 72(1):2–10.
- Meding B, Wrangsjo K, Järvholm B. 2005. Fifteen-year follow-up of hand eczema: Predictive factors. J Invest Dermatol. 124(5):893–897.
- Miyabe C, Miyabe Y, Nagai J, Miura NN, Ohno N, Chun J, Tsuboi R, Ueda H, Miyasaka M, Miyasaka N, et al. 2019. Abrogation of lysophosphatidic acid receptor 1 ameliorates murine vasculitis. Arthritis Res Ther. 21(1):191.
- Nakagomi D, Suzuki K, Meguro K, Hosokawa J, Tamachi T, Takatori H, Suto A, Matsue H, Ohara O, Nakayama T, et al. 2015. Matrix metalloproteinase 12 is produced by M2 macrophages and plays important roles in the development of contact hypersensitivity. J Allergy Clin Immunol. 135(5):1397–1400.
- OECD. 2012. The adverse outcome pathway for skin sensitization initiated by covalent binding to proteins. Part 2: Use of the AOP to develop chemical categories and integrated assessment and testing approaches. Series on Testing and Assessment No. 168. ENV/JM/MONO (2012)/PART2. Paris: OECD Publishing.
- OECD. 2014. The adverse outcome pathway for skin sensitization initiated by covalent binding to proteins, OECD series on testing and assessment, no. 168. Paris: OECD Publishing.
- OECD. 2018a. Test no. 442D: In vitro skin sensitisation: ARE-Nrf2 luciferase test method, OECD guidelines for the testing of chemicals, Section 4. Paris: OECD Publishing.
- OECD. 2018b. Test No. 442E: In vitro skin sensitisation: In vitro skin sensitisation assays addressing the key event on activation of dendritic cells on the adverse outcome pathway for skin sensitization, OECD guidelines for the testing of chemicals, section 4. Paris: OECD Publishing.
- Patlewicz G, Casati S, Basketter D, Asturiol D, Roberts D, Lepoittevin J, Worth A, Aschberger K. 2016. Can currently available non-animal methods detect pre and pro-haptens relevant for skin sensitization? Regul Toxicol Pharmacol. 82:147–155.
- Petry T, Bosch A, Coste X, Eigler D, Germain P, Seidel S, Jean P. 2017. Evaluation of in vitro assays for the assessment of the skin sensitization hazard of functional polysiloxanes and silanes. Regul Toxicol Pharmacol. 84:64–76.
- Raghavan B, Martin S, Esser P, Goebeler M, Schmidt M. 2012. Metal allergens nickel and cobalt facilitate TLR4 homodimerization independently of MD2. EMBO Rep. 13(12):1109–1115.
- Roggen E. 2014. In vitro approaches for detection of chemical sensitization. Basic Clin Pharmacol Toxicol. 115(1):32–40.
- Rustemeyer T, van Hoogstraten IMW, von Blomberg BME, Scheper RJ. 2020. Mechanisms of allergic contact dermatitis, kanerva's occupational dermatology. Cham: Springer International Publishing; p. 151–190. DOI:https://doi.org/10.1007/978-3-319-68617-2_14.
- Saito K, Nukada Y, Takenouchi O, Miyazawa M, Sakaguchi H, Nishiyama N. 2013. Development of a new in vitro skin sensitization assay (Epidermal Sensitization Assay; EpiSensA) using reconstructed human epidermis. Toxicol in Vitro. 27(8):2213–2224.
- Sakaguchi H, Ashikaga T, Miyazawa M, Yoshida Y, Ito Y, Yoneyama K, Hirota M, Itagaki H, Toyoda H, Suzuki H. 2006. Development of an in vitro skin sensitization test using human cell lines; Human Cell Line Activation Test (h-CLAT) II. An inter-laboratory study of the h-CLAT. Toxicol in Vitro. 20(5):774–784.
- Schmidt M, Goebeler M. 2015. Immunology of metal allergies. J Dtsch Dermatol Ges. 13(7):653–659.
- Schwensen J, Uter W, Bruze M, Svedman C, Goossens A, Wilkinson M, Giménez Arnau A, Gonçalo M, Andersen K, Paulsen E, et al. 2017. The epidemic of methylisothiazolinone: A European prospective study. Contact Dermatitis. 76(5):272–279.
- Shornick L, Bisarya A, Chaplin D. 2001. IL-1β is essential for langerhans cell activation and antigen delivery to the lymph nodes during contact sensitization: Evidence for a dermal source of IL-1β. Cell Immunol. 211(2):105–112.
- Thelu A, Catoire S, Kerdine-Römer S. 2020. Immune-competent in vitro co-culture models as an approach for skin sensitisation assessment. Toxicol in Vitro. 62:104691
- Thyssen JP, Menné T. 2010. Metal allergy-a review on exposures, penetration, genetics, prevalence, and clinical implications. Chem Res Toxicol. 23(2):309–318.
- Zheng H, Hogberg J, Stenius U. 2017. ATM-activated autotaxin (ATX) propagates inflammation and DNA damage in lung epithelial cells: A new mode of action for silica-induced DNA damage? Carcinogenesis. 38(12):1196–1206.