ABSTRACT
Background
Military special operators, elite athletes, and others requiring uninterrupted optimal performance currently lack options for sleep and mood support without performance-inhibiting effects. Kavalactones, derived from the root of the kava plant (Piper methysticum Forst), have been shown to elevate mood and wellbeing by producing a feeling of relaxation without addiction or cognitive impairment.
Methods
In this placebo-controlled, crossover study (NCT05381025), we investigated the effects of 2 weeks of kavalactones use on cortisol (diurnal salivary), sleep (RSQ-W; Restorative Sleep Questionnaire, Weekly), mood (DASS-21; Depression Anxiety Stress Scale-21), and motivation state to expend (Move) or conserve (Rest) energy (CRAVE; Cravings for Rest and Volitional Energy Expenditure, Right Now) in a cohort of 15 healthy, physically fit young males engaged in a rigorous, two-a-day preparation class for special operations forces qualification.
Results
Cortisol, sleep, and mood were within normal, healthy parameters in this cohort at baseline. This remained unchanged with kavalactones use with no significant findings of clinical interest. However, a statistically similar, positive slope for within-group Move scores was seen in both groups during kavalactones loading (first group Move slope 2.25, second group Move slope 3.29, p = 0.299). This trend was seen regardless of order and with no apparent effects on the Rest metric (all p ≥ 0.05). Moreover, a significant between-group difference appeared after 1 week of kavalactones use in the first phase (p = 0.044) and persisted through the end of the first loading period (p = 0.022). Following the 10-day washout, this between-groups divergence remained significant (p = 0.038) but was reversed by 1 week after the crossover (p = 0.072), with Move scores once again statistically similar between groups and compared to baseline at study end. Furthermore, the group taking kavalactones first never experienced a significant decrease in Move motivation state (lowest mean score 21.0, highest 28.6, all p ≥ 0.05), while the group receiving kavalactones in the last 2 weeks of the study had Move scores that were statistically lower than baseline (lowest mean score 8.6, highest 25.9, all p ≤ 0.05) at all time points but the last (p = 0.063) after 2 weeks of kavalactones exposure.
Conclusions
We report a novel finding that kavalactones may support performance by maintaining or rescuing the desire to expend energy in the context of significant physical and mental strain in well-conditioned individuals, even in a context of already normal cortisol, sleep, and mood.
1. Introduction
The elite modern athlete or soldier is under tremendous physical and mental stress, both while training and when called upon during the execution phase of competition or operations. Acute physical stress as well as behavioral stress can interfere with sleep and cause cortisol to increase [Citation1–3]. This can have significant effects on the ability to perform as well as on general quality of life and, if chronic, on long-term health [Citation4,Citation5]. Support for a healthy stress response and quality sleep among elite performers must be safe, non-habit forming, efficacious, and must not hinder performance. At present, there is a paucity of satisfactory options available to these populations.
Kava (Piper methysticum Forst, also known as yaqona, ‘awa, ‘ava, and others) has been utilized for centuries throughout Oceania in ceremonial applications as well as informally for its relaxing properties. The bioactive phytocompounds in kava responsible for these effects are known as kavalactones, of which six (desmethoxyyangonin, dihydrokavain, yangonin, kavain, dihydromethysticin, and methysticin) comprise over 96% of the total [Citation6]. Kavalactones are the only compounds from the Kava plant with documented psychoactivity [Citation7]. The kava experience, attributed to the kavalactones, is described as a relaxed state of mind without the marked euphoria, impaired decision-making, or disinhibition often seen with substances such as alcohol and benzodiazepines [Citation8]. Importantly, kava is not considered habit-forming [Citation9,Citation10]. Such a mild yet noticeable shift in excitation–inhibition balance without cognitive impairment, tolerance factors, or habituation is the key reason kava generates interest for use in elite performers under stress. On the other hand, short-term muscle relaxation and sleepiness have also been described as effects associated with traditional kava use, presenting potential incompatibility with peak performance when consumed immediately before rigorous physical activity [Citation11,Citation12]. While rare cases of kava-associated idiosyncratic liver injury have been reported when co-medicating organic kava extracts with pharmaceuticals, centuries of chronic, often heavy consumption in Oceania have not yielded indications of common, significant adverse effects [Citation13] other than gradual-onset, reversible dermopathy. Dermopathy has been reported in heavy users that routinely use hundreds of grams of kava per week, at least 10× the amount of kava used in preparation of the extract used in the present study [Citation14].
Historically and traditionally, kava dosage has been approached in a similar manner to alcohol, in that it is typically used to effect rather than in a specific dosage. Dose in traditional settings is typically not quantified nor known. In dietary supplement form, 75 mg to 225 mg per day is commonplace in the market.
A recent study showed evidence that 1 week of kava use (225 mg kavalactones/day) reduced cortisol levels in individuals with elevated cortisol at baseline but did not impact cortisol in individuals with normal levels at baseline [Citation15]. We sought to further investigate the hypothalamic–pituitary–adrenal (HPA)-kava relationship in the context of sustained and repetitive physical and mental stress in healthy elite athletes preparing for application to the US military special operations forces. Secondary endpoints targeting sleep and mood were monitored to inform interpretation of results, while motivation state was an exploratory endpoint related to performance. We hypothesized that kava would support healthy cortisol, stress levels, and sleep quality while not impairing motivation state in the context of physically fit males participating in a physically and mentally demanding fitness program.
2. Materials and methods
2.1. Study design
This double-blind, placebo-controlled, crossover study () was conducted concurrently with a 6-week intensive Special Operations Selection Prep Class (Performance First, Virginia Beach, Virginia, USA) designed to prepare students to get to and through military operation selection courses such as Basic Underwater Demolition/SEAL Training (BUD/S). The class had a minimum fitness requirement of 50 sit-ups in 2 min, 50 push-ups in 2 min, 10 pull-ups, and a 12:30 min 1.5 mile run. Students did not need to be, and in this study were not, in the military.
Figure 1. Study schema. This 6-week study consisted of two 14-day loading periods separated by a 10-day washout and crossover of interventions. Participants took either 225 mg kavalactones or matching placebo nightly during loading. Saliva was collected for cortisol assessment four times per day (diurnal pattern) at the start and end of each loading period. Questionnaires included the RSQ-W, DASS-21, and CRAVE instruments as well as a small number of additional questions about sleep and were completed weekly during loading. L1 = first loading period, L2 = second loading period, s = start of loading period, m = middle of loading period, e = end of loading period, KL = kavalactones, PL = placebo, KL-First (solid box) = the group of participants who began the study taking kavalactones and switched to placebo (L1 N = 8, L2 N = 6), PL-First (dashed box) = the group of participants who began the study taking placebo and switched to kavalactones (N = 7).
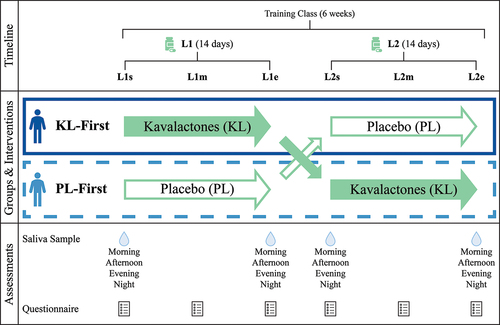
The class provided a semi-structured, physically and mentally demanding environment that was anticipated to acutely challenge participant stress responses and sleep quality during the study. The study began on the second day of the training class and consisted of two, 14-day loading phases separated by a 10-day washout and crossover period. The washout was dictated by the length of the training class, less the loading phases and some administrative/logistical time, and was anticipated to be sufficient to exhaust any effects of the kavalactones. Salivary cortisol was measured at the beginning and end of each 2-week loading phase. Weekly questionnaires during loading were utilized to assess quality of sleep and mood. To address the potential for carryover interference with the ability to perform optimally due to kava’s depressant properties, motivation to move (expend energy) and motivation to rest (conserve energy) were also monitored via the weekly questionnaire.
This study was approved by the Institutional Review Board WCG IRB (#20221311), registered at clinicaltrials.gov (NCT05381025), and conducted in accordance with the Declaration of Helsinki. It also complies with the Consolidated Standards of Reporting Trials (CONSORT) guidelines.
Informed consent was obtained from all participants involved in the study.
2.2. Supplementation
Participants took either 225 mg kavalactones (KL) (75 mg kavalactones capsules; Thorne Research, Inc., South Carolina, USA) or matched placebo (PL) (Thorne Research, Inc., South Carolina, USA) nightly during the two loading phases. Dosing at 225 mg was selected to align with recent clinical research [Citation16], and the typical dosing seen in the US dietary supplement marketplace. Capsules were taken 30 minutes to 1 hour before sleep in an effort to target kava’s relaxing effects toward sleep quality and reduce any potential interference with optimal performance during the day. The Tmax of each kavalactone has been measured at 1–2 hours when taken orally [Citation17], therefore dosing was optimally timed to support sleep at this interval. No capsules were taken during the washout.
Students in the class who opted into the trial were split into two groups. One group (KL-First) took KL during the first loading period (L1), then switched to PL in the second loading period (L2). The other group (PL-First) began the study with PL and switched to KL after the washout. Participants and study managers/course instructors were blinded to the identity of the capsules throughout the study. The rest of the study team had no direct contact with the participants during the study.
2.3. Participants
Healthy, physically fit (based on entrance requirements for the training class) males who enrolled in the training class were invited to participate in the study after class enrollment and prior to the start of the class. Individuals were eligible if they were generally healthy, 18 years old or older, male, free of known liver or kidney disease, had not used kava (Piper methysticum), over the counter, or prescription sleep aids within the prior 8 weeks, were not using acetaminophen or were willing to replace its use with an NSAID during the study, and had no known sensitivity, allergy, or prior adverse reaction to kava or related botanicals (peppers). During the study, participants were to refrain from the use of alcoholic beverages and prescription, over the counter, or other dietary supplement sleep aids.
Females were not included in this study because the target population (the training class) is typically only male (as was the case for this session). This was considered an opportunity for reduced variability, increasing the capacity to detect smaller changes with the understanding that the ability to extrapolate outcomes to other populations is reduced. Future studies will include a more varied population.
Of the 19 individuals enrolled in the class, 16 were eligible, provided consent, and were enrolled in the study. Prior to study start, one enrolled individual dropped out of the class, and therefore the study, due to an unrelated health issue. Fifteen individuals completed the first phase of the crossover study (KL-First = 8, PL-First = 7). Two individuals from KL-First dropped out of the study at the end of L1 citing morning grogginess. Thirteen individuals completed both phases of the study (KL-First = 6, PL-First = 7). Grogginess, or a carryover effect of the nervous system depressant action of kava into the next day, is a known possible effect of kava for some users and, as such, was an anticipated effect in this study and not considered an adverse effect [Citation18].
2.4. Participant training paradigm
Athletes were required to train twice daily, 6 days per week, over 6 continuous weeks as part of the training program design. The twice daily workouts consisted of two separate tracks, including 1) 2 hours of resistance/weight training at the training facility and 2) swimming or rucking (walking or running with a weighted backpack) on their own time, typically in groups. The rucking duration ranged between 20 min and 45 min, using weighted sandbags from 25 to 55 pounds. Weights were varied based on the distance, terrain, or desired speed. Swimming duration was roughly 60 minutes daily, which included a combination of skill work and time/distance-driven loading. The cohort was split in half with each group having alternate training schedules. Specifically, one cohort weight trained in the morning while the other group was rucking or swimming. In the afternoon, the groups switched activities. Although the study included a 10-day washout period during weeks three and four, at which time no test materials were ingested, training continued during this period.
2.5. Randomization
Participants were randomized into KL-First or PL-First groups upon enrollment using an alternating allocation scheme. Training program logistics did not allow for the randomization schedule to control for the distribution of KL-First and PL-First in the respective training groups, since the primary focus of the training program was not research but rather the successful entry of the participants into an active military SOF unit. There were no statistically significant differences in basic physical characteristics () or measured variables (see Results) between the two groups at baseline.
Table 1. Baseline participant characteristics.
2.6. Cortisol assessment
Cortisol was assessed using Ayumetrix Cortisol Diurnal Rhythm (Cx4) Test Kits (Ayumetrix LLC, Lake Oswego, Oregon, USA) according to kit instructions, which included avoiding lip products on collection day, or facial products for 3 days, food, drink (except water), or teeth brushing for at least 2 hours prior to collection. Participants were instructed to collect saliva at four time points on the first day of each loading phase (L1s, L2s) prior to taking the first study dose in the evening and on the day after the last dose of each loading phase (L1e, L2e). Collection times were aligned with a typical diurnal schedule: “Morning” to be within 30 minutes of waking, preferably before 8:00 am; “Afternoon” approximately 12:00 pm and before lunch; “Evening” approximately 4:00 pm and before the earlier of dinner or 6:00 pm; “Night” within 30 minutes to 1 hour of sleep and before taking the study dose at L1s and L2s.
Saliva samples were stored at room temperature until the next morning when they were collected and shipped overnight to Ayumetrix for analysis. Ayumetrix’s cutoffs for normal cortisol levels in males were utilized for interpretation purposes (Morning 1.5–9.6 ng/ml, Afternoon 0.6–4.1 ng/ml, Evening 0.2–2.3 ng/ml, Night 0.1–1.8 ng/ml).
2.7. Sleep assessment
Sleep was assessed weekly via an online questionnaire on the same days as cortisol was collected as well as at the midpoint of each loading phase (L1m, L2m). The Restorative Sleep Questionnaire, Weekly Version (RSQ-W) was used to assess sleep quality [Citation19]. This instrument yields a score ranging from 0 to 100. It does not have published cutoff values to indicate healthy vs unhealthy or restorative vs non-restorative sleep, however it does stipulate that higher scores indicate more restorative sleep.
Additional open or categorical questions about typical sleep and wake times, sleep regularity, latency, night waking, and self-reported quality and satisfaction were also asked on the questionnaires. These were non-validated questions used to provide context for data interpretation.
2.8. Mood assessment
Various aspects of mood were assessed weekly during loading periods on the same days as sleep via an online questionnaire containing the 21-item Depression Anxiety Stress Scale (DASS-21), a shorter, previously validated version of the DASS-42 [Citation20–22]. This instrument is non-diagnostic, yielding three sub-scores for each of depressed mood (Depression), anxious mood (Anxiety), and stressed mood (Stress). DASS-21 sub-scores range from 0 to 21 and, according to the instrument’s instructions, were doubled (range 0–42) for comparison to DASS-42 scores and reference ranges. Doubled scores are reported here. Lower scores reflect less disrupted moods. Non-diagnostic “normal” reference ranges on the 42-point scale are 0–9 for Depression, 0–7 for Anxiety, and 0–14 for Stress. The combined score (Composite) can be used to assess overall mood, but there is no reference range.
2.9. Motivation state assessment
Motivation state was also assessed weekly during loading via an online questionnaire containing the validated Cravings for Rest and Volitional Energy Expenditure (CRAVE) instrument, “Right Now” version [Citation23]. The CRAVE tool yields two sub-scores for opposing but decoupled motivation states, one for motivation to move or expend energy (Move) and one for motivation to rest or conserve energy (Rest). CRAVE scores range from 0 to 50. Higher scores reflect a stronger motivation state. Move and Rest scores were calculated separately per instrument instructions. The difference between Move and Rest was also calculated to evaluate net motivation state as influenced by both factors. Difference scores may range from −50 to +50. Positive scores are considered “Move-dominant” while negative scores are “Rest-dominant” and zero is neutral.
2.10. Statistical analysis
Baseline participant characteristics (age, height, weight, BMI) and study compliance were analyzed with the Student’s t-test. Cortisol data was analyzed using two-way repeated measures ANOVA within each group and three-way ANOVA for the total dataset. All other between-group comparisons (RSQ-W, DASS-21 (converted to −42), and CRAVE scores) were performed using the non-parametric Wilcoxon Rank Sum test (aka Mann-Whitney U test) for each of the six time points. All other within-group (repeated measures) data for each group were analyzed using the non-parametric paired samples Wilcoxon Signed Rank test for each time point vs L1s and for L1e vs L2s and L2s vs L2e. The Wilcoxon tests are the preferred tests for non-parametric data (interval or non-normal distribution), which applies to the qualitative/semi-quantitative questionnaire data collected in this study. Slopes and statistical comparison of slopes between groups were calculated by Linear Fit Comparison of Parameters. Figures depict mean values and error bars represent standard deviation (SD), an appropriate comparison to normal/healthy values for the various physiological/behavioral measures. To preserve data points and avoid skewing the small data set with imputed values, missing data from KL-First L2 dropouts were ignored such that data from L1 was calculated and reported here against N = 8 and data from L2 was calculated against N = 6 for KL-First. To assess the validity of this strategy, tests were conducted with and without the partial dataset from the two individuals who dropped from the study. Results were the same if all data points from the sets with missing values were omitted and analyses were made against N = 6 for both L1 and L2 instead. One missing cortisol point (sample error) was replaced by the same value as the two prior measurements. Calculations made with this value omitted did not yield different results. Statistical significance was considered p < 0.05 for all tests. Calculations were performed with R (4.2.1), Excel (Version 2211 Build 16.0.15831.20220 64-bit), OriginPro 2023 (Version 10.0.0.154), or OriginPro 2024 (Version 10.1.0.178).
3. Results
3.1. Cortisol
There were no clinically relevant statistical differences in mean cortisol levels between groups (p = 0.375) or between like time points within-groups (p = 0.145) at study start or throughout the study. Significant findings between different sample times, such as morning vs evening, are expected in the diurnal cortisol pattern and were not considered relevant to the hypothesis of this study.
Mean cortisol levels were within the normal physiological range, as defined by the test kit manufacturer (see Methods), at all time points throughout the study except at the L1s Evening time point for KL-First, which was higher by 0.05 ng/ml (2.35 ng/ml vs 2.30 ng/ml high-normal) (). Being collected at the first time point, this represented a baseline measurement, prior to any loading with KL or PL.
Figure 2. Diurnal salivary cortisol (ng/ml). Saliva was collected four times per day at the start (s) and end (e) of each loading period (L1, L2), and the means for each group were plotted as a typical diurnal cortisol curve. All means were within the normal range except L1s Evening, which was the result of a single data point that was unusually high. The mean for L1s Evening fell within the normal range when this data point was excluded from the calculation (triangle). No significant differences between or within groups were found. Filled circles = mean during kavalactones (KL) loading phase; hollow circles = mean during placebo (PL) loading phase; L1s Evening triangle = KL-First cortisol mean with the high data point excluded; solid line = KL-First group (L1 N = 8, L2 N = 6); dashed line = PL-First group (N = 7); error bars = SD; gray fill area = normal diurnal salivary cortisol range.
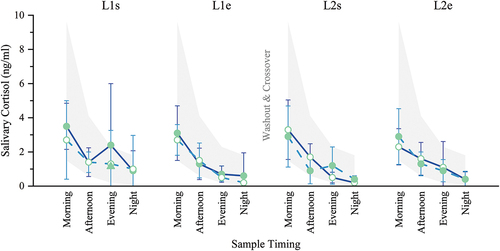
This higher-than-normal Evening result can be attributed to a single data point (10.8 ng/ml). The mean at this time point decreases from 2.35 ng/ml to 1.14 ng/ml, well within the normal range, with this data point omitted ( L1s Evening triangle). Despite this higher-than-normal value for the evening cortisol measurement at baseline, KL-First and PL-First were not statistically different at this time point (p = 0.510). This data point was included in all other calculations.
3.2. Sleep
Restorative sleep quality scores were not statistically different between groups at study start, and they did not change significantly within or between groups (all p ≥ 0.05) during the study (). Self-reported, subjective sleep satisfaction, quality, timing, and latency also did not show any significant changes during the study (data not shown).
Figure 3. Sleep quality. Restorative sleep questionnaire, weekly version (RSQ-W), scores range 0–100; higher is more restorative sleep. No significant differences between or within groups were found. Filled circles = mean during kavalactones (KL) loading phase; hollow circles = mean during placebo (PL) loading phase; solid line = KL-First group (L1 N = 8, L2 N = 6); dashed line = PL-First group (N = 7); error bars = SD.
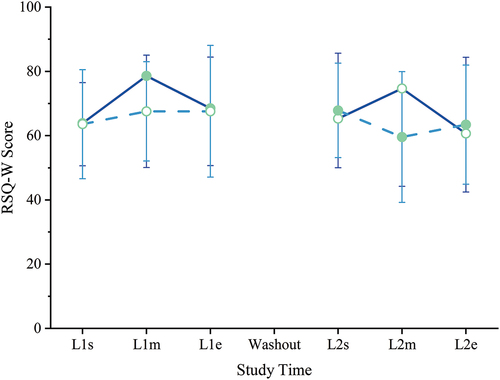
The highest individual score was 100 (occurring 3 times) and the lowest 33 (occurring once), with 36% of scores (30 of 84) at 75 or higher and 17% (14 of 84) below 50. The baseline (L1s) mean was 64 for all scores as well as for the KL-First and PL-First groups separately. Baseline median scores were 61, 64, and 58, respectively. Both mean and median for all RSQ-W scores was 67, and separately 69 and 72 for KL-First and 65 and 67 for PL-First, respectively.
The RSQ-W is a relative measure, with higher scores indicating more restorative sleep. Given the clustering of participant scores well above the midpoint of the 0–100 range for all groupings at baseline, we interpret that participants were getting relatively restorative sleep prior to study start. That scores remained high and statistically indistinct throughout the study could indicate that kavalactones are not able to improve the restorative quality of sleep above that level or that kavalactones do not influence the restorative quality of sleep. A separate study more deeply investigating various aspects of sleep in a cohort experiencing less restorative sleep at baseline would be needed to differentiate these possible scenarios.
3.3. Mood
Scores for all three aspects of mood (Depressed, Anxious, Stressed) and the Composite score for overall mood were not statistically different between groups at study start. There were no significant differences in depressed mood scores between groups or times throughout the study (all p ≥ 0.05) (). Overall, Depression scores were very low, with 71% (64 of 90) of scores being 0 and only one individual score (10) above the normal cutoff of 9, indicating that this cohort was not experiencing depressive mood at any point during this study.
Figure 4. Mood. Depression Anxiety Stress Scale (DASS), 21-question version scores are reported here after conversion to DASS-42 equivalent by multiplying outcome scores by two per instrument instructions. Scores range 0–42; higher is more disrupted mood. All means were within the normal range except Anxiety at baseline (L1s) for the KL-First group. (a) Depression scores, normal 0–9; (b) Anxiety scores, normal 0–7; (c) Stress scores, normal 0–14; (d) composite scores (sum of Depression, Anxiety, and Stress), no normal range. Filled circles = mean during kavalactones (KL) loading phase; hollow circles = mean during placebo (PL) loading phase; solid line = KL-First group (L1 N = 8, L2 N = 6); dashed line = PL-First group (N = 7); error bars = SD; gray fill area = normal range (non-diagnostic). † = P ≤ 0.05 KL-First, ‡ = P ≤ 0.05 PL-First; * = P ≤ 0.05 KL-First vs PL-First; see text for specific p-values.
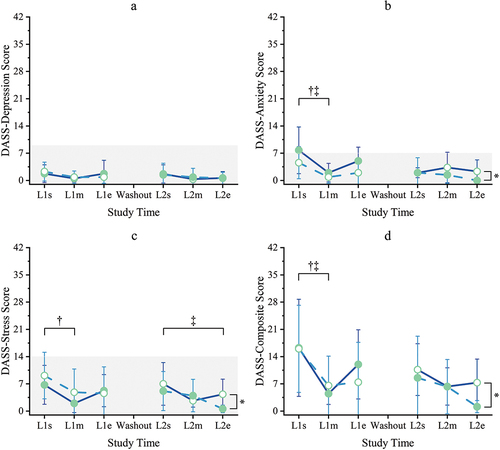
Anxious mood scores were not significantly different between groups until the end of the study (L2e p = 0.042), with PL-First significantly lower at L2e (). Within-group Anxiety was significantly lower than baseline for both groups at all time points (all p = 0.031) except L1e for KL-First (p = 0.141) and L2s for PL-First (p = 0.063). The mean Anxiety score of 7.8 for the KL-First group at baseline (L1s) was above the cutoff of 7 for normal anxiousness, although the median value (6) was within the normal range. The PL-First mean (4.6) and median (4) scores at baseline were both within the normal range. Despite this, the two groups were not statistically different at this time point (p = 0.263). All other Anxiety scores were within normal.
Stressed mood scores between groups were not significantly different until the end of the study (L2e p = 0.012), with PL-First significantly lower at L2e (). Within-group Stress decreased significantly (p = 0.031) for KL-First between L1s and L1m, with KL loading, but then rose to non-different from baseline for the remainder of the study (all p ≥ 0.05). PL-First Stress was not significantly lower than baseline during placebo loading (L1m p = 0.063, L1e p = 0.125), but fell to a statistically lower level during kavalactones loading (L2s p = 0.016, L2m p = 0.047, L2e p = 0.016). All Stress scores (mean and median) were within the normal range for this instrument (0–14) for all time periods.
The Composite mood scores reflected the patterns found in the Anxiety and Stress categories, showing no significant differences between groups until study end when the PL-First group reported significantly less overall mood disruption (mean 1.1, median 0) than KL-First (mean 7.3, median 4) following 2 weeks of KL use in the last 2 weeks of the training course (p = 0.015) (). A significant decrease in mood disruption was seen for both groups following acclimation to the class (L1s vs L1m p = 0.016 KL-First, p = 0.031 PL-First). Both KL-First and PL-First scores were lower than at study start throughout the rest of the study, but KL-First levels were only statistically lower at L1m (p = 0.016), L2m, and L2e (both p = 0.031), while PL-First scores were statistically lower than baseline at all subsequent time points (all p = 0.016). Since there are no interpretive cutoff values available for the Composite score, these levels only reflect overall mood disruption relative to no disruption. These results suggest that the groups experienced a similar overall mood state throughout the study, and that mood was relatively undisrupted.
3.4. Motivation state
There was no statistical difference in mean motivation to move the body/expend energy (Move) scores between groups at study start (p = 0.757) (). After 1 week with placebo, the PL-First group’s Move score was significantly lower than KL-First (p = 0.044), which had not dropped with kavalactones loading. A lower Move score indicates less desire to move the body or expend energy. The PL-First Move score continued to be significantly lower than KL-First through the end of the first loading period (L1e p = 0.022) and after the washout (L2s p = 0.038). Following crossover to kavalactones, the PL-First group’s Move score increased such that it was no longer statistically lower than KL-First after 1 week (L2m p = 0.072) and remained so through the end of the study (L2e p = 0.508).
Figure 5. Motivation state – move. Cravings for Rest and Volitional Energy Expenditure (CRAVE), Right Now version scores range 0–50; higher is more motivated to move/expend energy. Filled circles = mean during kavalactones (KL) loading phase; hollow circles = mean during placebo (PL) loading phase; solid line = KL-First group (L1 N = 8, L2 N = 6); dashed line = PL-First group (N = 7); error bars = SD. *p ≤ 0.05 PL-First vs baseline, †P ≤ 0.05 between groups; see text for specific p-values.
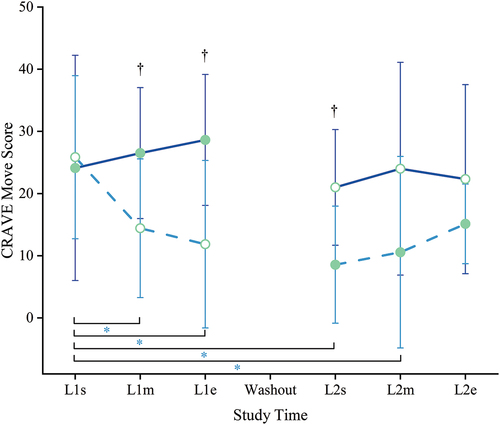
During the initial loading period (L1), while KL-First was receiving kavalactones, the KL-First group’s Move score did not change significantly from baseline (L1m p = 0.711, L1e p = 0.438). Additionally, their score remained stable throughout the study, never statistically deviating from baseline (all p ≥ 0.05) even with placebo. In contrast, PL-First experienced a significant decrease in Move in the first loading period (L1) versus baseline (L1m p = 0.047, L1e p = 0.031).
All participants continued the rigorous two-a-day training schedule through the 10-day washout period. No study materials were ingested during that time. Both groups experienced a non-significant decrease in Move across the washout (L1e vs L2s p = 0.438, slope −7.5 KL-First, p = 0.203, slope −3.3 PL-First).
Following the washout and crossover to kavalactones, PL-First Move remained significantly lower than baseline at L2s (p = 0.016) and L2m (p = 0.016) but rebounded by the end of the study back to statistically undifferentiated from study start (L2e p = 0.063).
Although not a statistically significant change, the slopes of the Move scores for both groups while taking kavalactones were positive (2.25 KL-First, 3.29 PL-First) and statistically similar (p = 0.299). The same was not seen for placebo, with KL-First slope 0.67 and PL-First slope −7.0 not statistically different (p = 0.118). A comparison of all four slopes indicated that there was a statistical difference (p = 0.026).
Unlike Move, we found no significant changes in mean motivation to rest the body/conserve energy (Rest) scores in either group or between groups throughout the study (all p ≥ 0.05) (). We noted a non-significant upward trajectory in Rest scores for both groups as the study progressed (slope 1.53 KL-First, 0.64 PL-First, slope p = 0.500, intercept p = 0.696), as may be expected given the persistent rigor of the training class.
Figure 6. Motivation state – Rest. Cravings for Rest and Volitional Energy Expenditure (CRAVE), Right Now version scores range 0–50; higher is more motivated to rest/conserve energy. No significant differences between or within groups were found. Filled circles = mean during kavalactones (KL) loading phase; hollow circles = mean during placebo (PL) loading phase; solid line = KL-First group (L1 N = 8, L2 N = 6); dashed line = PL-First group (N = 7); error bars = SD.
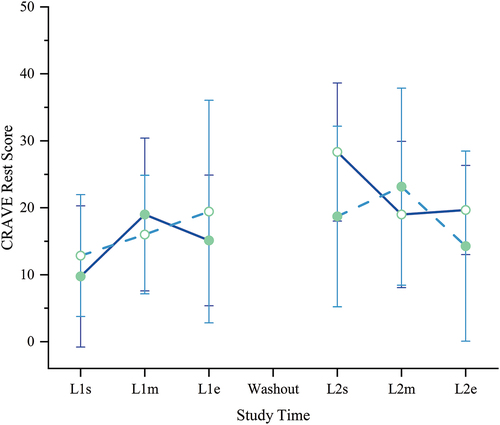
Since Move and Rest are decoupled states, we calculated the difference between Move scores and Rest scores at each time point to assess their net influence on overall motivation state for each group (). Both groups began the study in the Move-dominant zone (Move – Rest > 0) at a statistically similar level (p = 0.955). The two groups were never statistically different during the study (all p ≥ 0.05). However, the KL-First group remained Move-dominant throughout the study, except at L2s when they briefly dropped into the Rest-dominant domain (Move – Rest < 0) following the washout. In contrast, the PL-First group became Rest-dominant within 1 week, during placebo loading in L1, and remained so until eventually rebounding into the Move-dominant zone following 2 weeks of kavalactones use in the second loading phase. This shift was statistically significant compared to baseline at L1e, L2s, and L2m (each p = 0.016), aligning well with the Move findings for this group. Additionally, the net shift from Rest- to Move-dominant over the 2 weeks with kavalactones was significant (L2s vs L2e p = 0.031).
Figure 7. Motivation state – Move vs Rest. The difference between Move and Rest mean scores from the Cravings for Rest and Volitional Energy Expenditure (CRAVE), Right Now instrument were calculated for each time point and each group. Maximum range + 50 to − 50; positive is Move-dominant (more motivated to move/expend energy than rest/conserve energy), negative is Rest-dominant (more motivated to rest/conserve energy than move/expend energy), zero is balanced motivation state (Move=rest). Solid outline = KL-First group (L1 N = 8, L2 N = 6); dashed outline = PL-First group (N = 7); filled bars = Move-Rest difference during kavalactones (KL) loading phase; hollow bars = Move-Rest difference during placebo (PL) loading phase. *p ≤ 0.05 PL-First; see text for specific p-values.
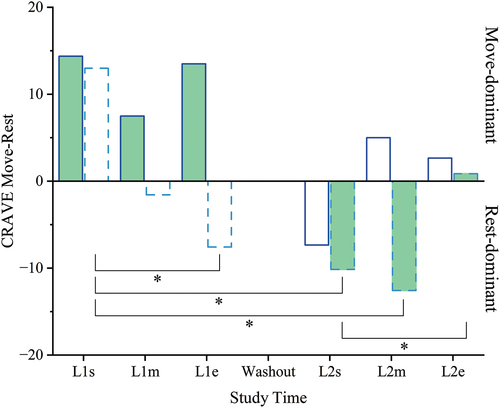
3.5. Study compliance
Study compliance was high, with 100% of the questionnaires returned and 100% of the required questions answered, 99.6% of the saliva samples submitted, and 97% dosing compliance (drops not counted as missed for compliance purposes). Only two individuals (one from each group) reported missing any doses. There were no significant differences in compliance between groups (p = 0.269).
3.6. Adverse events
No adverse events were reported.
4. Discussion
We report here the novel finding that 225 mg nightly of oral kavalactones provided to healthy young adult males undertaking a rigorous, 6-week SOF preparation training course, favorably impacted motivation to move or exert physical energy as assessed by the desire for activity component of the CRAVE score (Move). Regardless of order, whether taking kavalactones for the first 2 weeks or starting 4 weeks into the rigorous training regimen, neither group experienced a significant decrease in motivation to move while taking kavalactones. Furthermore, whereas the group beginning the study with placebo experienced a significant drop in motivation state (p = 0.022 vs KL-First, L1s vs L1e p = 0.031), falling to approximately one-third of their starting score by the beginning of the second loading period, the group beginning the study with kavalactones never experienced a significant decrease in motivation to move. Although the increase in motivation state during kavalactones loading was not statistically significant in this study (L1s vs L1e p = 0.438 KL-First, L2s vs L2e p = 0.063 PL-First), motivation to move during kavalactones loading in both groups demonstrated a positive and similar slope for each group (2.25 KL-First, 3.29 PL-First, p = 0.299) and the group receiving kavalactones second recovered their depleted Move score to starting level (p = 0.063L1s vs L2e) with kavalactones. Thus, rather than exerting a negative influence on motivation state, as may have been speculated due to known properties of kavalactones, this suggests the possibility that kavalactones may exert positive influence on motivation state for movement or energy expenditure when ingested at night and in the context of physical demands.
It is not known whether the Move score of the KL-First group remained stable rather than decreasing with placebo as a result of some indirect, long-term effect of their early kavalactones use, of circumstances related to the training such as acclimation, or of some other random or unmeasured factor. We are unable to assert that the kavalactones were having a direct, persistent effect, since serum levels were not measured. Given the half-life of kavalactones (<10 hours), the direct effect is not expected. However, we cannot rule out the possibility that the biological effect of having ingested the kava for 2 weeks may have persisted in some manner, though this was not directly examined in this study.
Of particular note was that these effects occurred without meaningful changes in sleep or mood, and salivary cortisol was not different between groups. We suspect that the transient elevation in Anxiety score seen at baseline was likely due to acute anxiety associated with the start of the class, which normalized after a few days of acclimation in both groups. The significant differences in Anxiety and Stress scores between groups at the end of the study are unlikely to be practically meaningful given that all values remained low and well within the normal ranges.
Perhaps not surprisingly given the demands of the training course and the relationship between fatigue and the desire to rest [Citation23], mean Rest scores sloped non-significantly upward in both groups as the course progressed (slope 1.53 KL-First, 0.64 PL-First, p = 0.500), suggesting that both groups became more desirous of rest as the demands of the training course accumulated. Despite the increasing desire for rest, however, participants ingesting kavalactones also remained willing to expend energy.
This raises a question as to whether this apparent protective or rescuing effect simply masked distress, which could potentially lead to a more dramatic decline following cessation of dosing, or whether the kavalactones supported healthy resistance to or recovery from physical or mental duress. Our finding that the group receiving kavalactones first experienced only a mild, non-significant decrease in motivation for the remainder of the study following withdrawal of kavalactones while continuing for an additional 4 weeks in the rigorous training regimen would suggest the likelihood that kavalactones are not simply concealing or delaying the deleterious effects of stress but rather supporting a healthier response to or recovery from stress or fatigue.
Furthermore, using a Move-Rest difference calculation to evaluate net motivation state, we found that, while both groups began in the Move-dominant domain, the group beginning the training program with kavalactones existed almost exclusively in the Move-dominant state throughout the study, while the group receiving kavalactones only after 4 weeks in the training program fell into the Rest-dominant state rapidly and remained Rest-dominant until after 2 weeks of kavalactones. We suggest that this observation further supports the notion that kavalactones taken at night have a positive influence on the desire to move or expend energy, even in the context of high allostatic load and an increasing desire to rest or conserve energy.
The mechanism of the kavalactone effect is important to examine, as long-term use is considered for high performing individuals. The primary inhibitory synaptic inputs generated by kavalactones are hypothesized to be related to allosteric upregulation of signal at GABA-A receptors [Citation24] and direct interaction with calcium and sodium voltage-gated ion channels [Citation25,Citation26]. However, a host of other central nervous system targets including acetylcholinesterase [Citation27], glycine receptors [Citation28], monoamine oxidases [Citation29,Citation30], TNF-α [Citation31], and NRF2 [Citation32] also interact with kavalactones. The pharmacodynamic target promiscuity of kavalactones, the distinct nature of those dynamics with respect to one another, and the disparate pharmacodynamic profiles of individual kavalactones [Citation17] each contribute formidable complexity in the efforts to unravel the connection between kava and motivation state.
Since kavalactones shift the central nervous system’s excitation–inhibition balance toward inhibition [Citation11,Citation12] in a manner that could interfere with performance, this study examined whether the use of 225 mg kavalactones in the evening had a potential for carryover effects that could adversely impact performance the following day. Such impediments to performance would be wholly unacceptable in such applications as the military or elite athletics where optimum performance is critical to success and even survival. We cite these findings as a preliminary indicator that pre-sleep use of kavalactones is likely to be compatible with optimal waking performance. These novel findings further suggest that kavalactones may actually facilitate rather than interfere with optimal performance through support of the motivation state during sustained periods of heavy physical loading, encouraging rather than discouraging a desire for physical activity.
This study had several limitations. First, the sample size was small, with no formal power calculation. Second, only males were included in the study due to restriction to training class enrollment, which limits generalizability to females. Third, the study was conducted with fit, healthy, young individuals, so the findings cannot easily be extrapolated to older, deconditioned individuals, or those with comorbidities. Fourth, due to limitations on study activity intrusion into the course schedule, there was variability in salivary cortisol collection times making it difficult to draw definitive conclusions about the lack of an effect on salivary cortisol observed in this study. Likewise, we were unable to control the exact timing of the CRAVE instrument assessments between individuals. Given the natural volatility of the motivation states, often fluctuating within minutes, many factors including time of day or immediately preceding or following activities could have influenced these results [Citation33]. Furthermore, with cortisol, sleep, and mood values all falling within normal ranges, it was not possible to assess any normalizing effect of kavalactones on any of these features. Finally, other than cortisol, study circumstances did not permit assessment of molecular markers, which may have allowed for the elucidation of a potential mechanism for the observed actions of the kavalactones on motivation to move.
In conclusion, nighttime ingestion of kavalactones may improve the day-time motivation state for movement or energy expenditure in physically fit males engaged in rigorous physical activities over a period of at least 2 weeks. The extent to which this effect is repeatable or sustainable over prolonged periods and generalizable to other populations is unknown. Kava has a long history of safe use when consumed within the therapeutic range [Citation13,Citation34], which makes it a promising candidate for use in high-performance environments in such groups as military, athletes, astronauts, expeditioners, and others engaged in extreme operations. Further research is underway in larger cohorts to better understand the mechanism of action, the durability of this effect, the impact on states such as burnout, and the translation of this effect to females, deconditioned individuals, and those with comorbidities.
Geolocation information
The study took place in Virginia Beach, Virginia, USA.
Acknowledgments
We extend a special thanks to Jeff Nichols and Katherine Grimm of Performance First for providing the research site, participant cohort, and on-site study management. Thanks also to Cem Meydan of Weill Cornell Medicine for his statistical insights and Jacqueline Jacques for her contribution to early study concepts. A special thanks goes out to Rick Kingston of the University of Minnesota and Chengguo (Chris) Xing of the University of Florida for their insights into the chemistry, clinical dynamics, and novel composition of the special kavalactone extract utilized in this study.
Disclosure statement
S.S., C.A., T.D., J.G., L.K., L.L., and S.P. are employees of Thorne HealthTech, Inc., the manufacturer of the study supplement and sponsor of the study. M.S. is the founder of Sovaris Aerospace and owns shares in Sovaris Holdings. C.S. and J.S. own shares in Sovaris Holdings. Sovaris Aerospace is a scientific advisor to Thorne HealthTech, Inc. No authors have received or will receive any special compensation in association with this work.
Additional information
Funding
References
- Bani-Issa W, Radwan H, Marzooq FA, et al. Salivary cortisol, subjective stress and quality of sleep among female healthcare professionals. JMDH. 2020;13:125–19. doi: 10.2147/JMDH.S229396
- Obmiński Z, Supiński J, Rydzik Ł, et al. Stress responses to one-day athletic tournament in sport coaches: a pilot study. Biology (Basel). 2022;11(6):828. doi: 10.3390/biology11060828
- Wright KP, Drake AL, Frey DJ, et al. Influence of sleep deprivation and circadian misalignment on cortisol, inflammatory markers, and cytokine balance. Brain Behav Immun. 2015;47:24–34. doi: 10.1016/j.bbi.2015.01.004
- Echouffo-Tcheugui JB, Conner SC, Himali JJ, et al. Circulating cortisol and cognitive and structural brain measures: the Framingham heart study. Neurology. 2018;91(21):e1961–e1970. doi: 10.1212/WNL.0000000000006549
- Halson SL. Sleep in elite athletes and nutritional interventions to enhance sleep. Sports Med. 2014;44(S1):13–23. doi: 10.1007/s40279-014-0147-0
- Côté CS, Kor C, Cohen J, et al. Composition and biological activity of traditional and commercial kava extracts. Biochem Biophys Res Commun. 2004;322(1):147–152. doi: 10.1016/j.bbrc.2004.07.093
- Volgin A, Yang L, Amstislavskaya T, et al. DARK classics in chemical neuroscience: kava. ACS Chem Neurosci. 2020 [cited 2022 Mar 20];11(23):3893–3904. Available from: https://pubs.acs.org/doi/pdf/10.1021/acschemneuro.9b00587
- Aporosa SA. De-mythologizing and re-branding of kava as the new ‘world drug’ of choice. Drug Sci, Policy Law. 2019;5:2050324519876131. doi: 10.1177/2050324519876131
- Wang D, Yang L, Wang J, et al. Behavioral and physiological effects of acute and chronic kava exposure in adult zebrafish. Neurotoxicol Teratol. 2020;79:106881. doi: 10.1016/j.ntt.2020.106881
- Sarris J, Stough C, Teschke R, et al. Kava for the treatment of generalized anxiety disorder RCT: analysis of adverse reactions, liver function, addiction, and sexual effects. Phytother Res. 2013;27(11):1723–1728. doi: 10.1002/ptr.4916
- LaPorte E, Sarris J, Stough C, et al. Neurocognitive effects of kava (Piper methysticum): a systematic review. Hum Psychopharmacol. 2011;26(2):102–111. doi: 10.1002/hup.1180
- Apo’Aporosa S, Ballard H, Pandey R, et al. The impact of traditional kava (Piper methysticum) use on cognition: implications for driver fitness. J Ethnopharmacol. 2022;291:115080. doi: 10.1016/j.jep.2022.115080
- Abbott P. Kava: a review of the safety of traditional and recreational beverage consumption [Internet]. Rome: Food and Agriculture Organization of the United Nations World Health Organization (FAO/WHO); 2016 [cited 2019 Jun 25]. Available from: http://www.fao.org/3/a-i5770e.pdf
- Mathews JD, Riley MD, Fejo L, et al. Effects of the heavy usage of kava on physical health: summary of a pilot survey in an aboriginal community. Med J Aust. 1988;148(11):548–555. doi: 10.5694/j.1326-5377.1988.tb93809.x
- Wang Y, Narayanapillai SC, Tessier KM, et al. The impact of one-week dietary supplementation with Kava on biomarkers of tobacco use and nitrosamine-based carcinogenesis risk among active smokers. Cancer Prev Res (Phila). 2020;13(5):483–492. doi: 10.1158/1940-6207.CAPR-19-0501
- Sarris J, Byrne GJ, Bousman CA, et al. Kava for generalised anxiety disorder: a 16-week double-blind, randomised, placebo-controlled study. Aust N Z J Psychiatry. 2020;54(3):288–297. doi: 10.1177/0004867419891246
- Kanumuri SRR, Mamallapalli J, Nelson R, et al. Clinical pharmacokinetics of kavalactones after oral dosing of standardized kava extract in healthy volunteers. J Ethnopharmacol. 2022;297:115514. doi: 10.1016/j.jep.2022.115514
- Apo’Aporosa S, Tomlinson M. Kava Hangover and gold-standard science. Anthropologica. 2014;56:163–175.
- Drake CL, Hays RD, Morlock R, et al. Development and evaluation of a measure to assess restorative sleep. J Clin Sleep Med. 2014;10(7):733–741. doi: 10.5664/jcsm.3860
- Lovibond PF, Lovibond SH. The structure of negative emotional states: comparison of the depression anxiety stress scales (DASS) with the beck depression and anxiety inventories. Behav Res Ther. 1995;33(3):335–343. doi: 10.1016/0005-7967(94)00075-U
- Henry JD, Crawford JR. The short-form version of the depression anxiety stress scales (DASS-21): construct validity and normative data in a large non-clinical sample. Br J Clin Psychol. 2005;44(2):227–239. doi: 10.1348/014466505X29657
- Ng F, Trauer T, Dodd S, et al. The validity of the 21-item version of the depression anxiety stress scales as a routine clinical outcome measure. Acta Neuropsychiatr. 2007;19(5):304–310. doi: 10.1111/j.1601-5215.2007.00217.x
- Stults-Kolehmainen MA, Blacutt M, Fogelman N, et al. Measurement of motivation states for physical activity and sedentary behavior: development and validation of the CRAVE scale. Front Psychol [Internet]. 2021 [cited 2022 Feb 23];12. doi: 10.3389/fpsyg.2021.568286
- Chua HC, Christensen ETH, Hoestgaard-Jensen K, et al. Kavain, the major constituent of the anxiolytic Kava extract, Potentiates GABAA receptors: functional characteristics and molecular mechanism. Barnes S, editor. PLOS One. 2016;11(6):e0157700. doi: 10.1371/journal.pone.0157700
- Walden J, von Wegerer J, Winter U, et al. Effects of kawain and dihydromethysticin on field potential changes in the hippocampus. Prog Neuropsychopharmacol Biol Psychiatry. 1997;21(4):697–706. doi: 10.1016/S0278-5846(97)00042-0
- Friese J, Gleitz J. Kavain, dihydrokavain and dihydromethysticin non-competitively inhibit the specific binding of [3 H]-batrachotoxinin-A 20-α-benzoate to receptor site 2 of voltage-gated Na+ channels. Planta Med. 1998;64(5):458–459. doi: 10.1055/s-2006-957482
- Noor NA. Anxiolytic action and safety of Kava: effect on rat brain acetylcholinesterase activity and some serum biochemical parameters. AJPP. 2010;4:823–828.
- Hegazy NH, Breitinger H-G, Breitinger U. Kavalactones from Kava (Piper methysticum) root extract as modulators of recombinant human glycine receptors. Biol Chem. 2019;400(9):1205–1215. doi: 10.1515/hsz-2019-0112
- Uebelhack R, Franke L, Schewe H-J. Inhibition of platelet MAO-B by Kava pyrone-enriched extract from Piper methysticum Forster (Kava-Kava). Pharmacopsychiatry. 1998;31(5):187–192. doi: 10.1055/s-2007-979325
- Li X, Song L, Xu S, et al. Kava root extracts hinder prostate cancer development and tumorigenesis by involvement of dual inhibition of MAO-A and LSD1. J Transl Genet Genom. 2021;5:163–172. doi: 10.20517/jtgg.2021.22
- Huck O, Han X, Mulhall H, et al. Identification of a kavain analog with efficient anti-inflammatory effects. Sci Rep. 2019;9(1):12940. doi: 10.1038/s41598-019-49383-8
- Fragoulis A, Siegl S, Fendt M, et al. Oral administration of methysticin improves cognitive deficits in a mouse model of Alzheimer’s disease. Redox Biol. 2017;12:843–853. doi: 10.1016/j.redox.2017.04.024
- Budnick C, Stults-Kolehmainen M, Dadina C, et al. Motivation states to move, be physically active and sedentary vary like circadian rhythms and are associated with affect and arousal. Front Sports Act Living. 2023;5. doi: 10.3389/fspor.2023.1094288
- Smith K, Leiras C. The effectiveness and safety of Kava Kava for treating anxiety symptoms: a systematic review and analysis of randomized clinical trials. Complement Ther Clin Pract. 2018;33:107–117. doi: 10.1016/j.ctcp.2018.09.003