ABSTRACT
Genetic diversity and relatedness among nine North American red raspberry (Rubus idaeus L.) cultivars and four Canadian breeding lines were studied using inter simple sequence repeat (ISSR) markers and pedigree analysis. Eighteen primers generated 306 polymorphic ISSR-PCR bands. Cluster analysis by the unweighted pair-group method with arithmetic averages (UPGMA) revealed a substantial degree of genetic diversity among the 13 genotypes, similarity values ranged from 24% to 49% that were in agreement with the principal coordinate (PCO) analysis. Geographical distribution for the place of breeding program explained only 1.0% of total variation as revealed by analysis of molecular variance (AMOVA). The UPGMA clustering for coancestry identified 3% to 25% similarity among the nine cultivars. The correlation coefficient between the genetic similarity values calculated from the Jaccard-based ISSR data and pairwise coefficients of coancestry of nine cultivars was positive but insignificant. The ISSR markers detected a sufficient degree of polymorphism to differentiate among raspberry genotypes, making this technology valuable for cultivar identification and for the more efficient choice of parents in the current raspberry breeding program.
INTRODUCTION
The genus Rubus contains over 500 highly variably and heterogeneous species within 12 subgenera, which occur in all parts of the world except the desert regions (CitationJennings et al., 1990). The most commercially important of the domesticated subgenera is Ideobatus (raspberries) that contains some 200 species showing considerable differentiation. Red raspberry (Rubus idaeus L.; 2n=2x=14) is an economically important berry crop with a high free radical scavenging capacity and it contains numerous bioactive compounds with potential health benefits (CitationDe Ancos et al., 2000). The most important red raspberries are the European red raspberry (R. idaeus ssp. idaeus L.) and the North American red raspberry (R. idaeus ssp. strigosus Michx).
There is a pressing need to develop reliable methods for identifying raspberry cultivars and for assessing genetic diversity/relatedness in raspberry genotypes for practical breeding purposes and proprietary-rights protection. Molecular markers are increasingly used in breeding programs of many horticultural crops. The introduction of molecular biology techniques, such as DNA-based markers, allows direct comparison of different genetic material independent of environmental influences (CitationWeising et al., 1995). The degree of similarity between the banding patterns can provide information about genetic similarity, and relationships between the samples studied.
Several polymerase chain reaction (PCR)-based marker systems are now available for use on plant species. Each marker system has its own strengths and limitations, making the choice of marker an important decision. Molecular markers used for detecting genetic diversity in Rubus species have been demonstrated for allozymes (CitationCousineau and Donnelly, 1992), restriction fragment length polymorphisms (RFLPs) (CitationWaugh et al., 1990), randomly amplified polymorphic DNAs (RAPDs) (CitationGraham and McNicol, 1995; CitationGraham et al., 1997; CitationWeber, 2003), amplified fragment-length polymorphisms (AFLPs) (CitationKollmann et al., 2000), minisatellites (CitationNybom, 1995), and inter simple sequence repeat markers (ISSRs) (CitationDebnath, 2007). ISSRs (CitationZietkiewicz et al., 1994) have also been used successfully in a number of horticultural crops including cashew (Anacardium occidentale L.; CitationArchak et al., 2003), chestnut (Castanea sative Mill.) (CitationCasasoli et al., 2001), Clematis species (CitationGardner and Hokanson, 2005), mulberry (Morus spp.) (CitationVijayan and Chatterjee, 2003), potato (Solanum tuberosum L.) (CitationPrevost and Wilkinson, 1999), and strawberry (CitationArnau et al., 2002). The ISSRs are the regions between two microsatellites (also called simple sequence repeats, SSRs). The ISSR primers target microsatellites that are abundant throughout the plant genome (CitationWang et al., 1994). These markers have proven to be more reproducible than RAPD markers and generally reveal higher levels of polymorphism because of the higher annealing temperature and longer primer sequences (CitationQian et al., 2001). They cost less and are easier to use than AFLPs and do not require prior knowledge of flanking sequences like SSRs (CitationReddy et al., 2002).
The purpose of this study was (1) to evaluate the use of ISSR markers for distinguishing among nine raspberry cultivars and four breeding lines developed in Canada and in the United States, (2) to estimate the genetic relatedness of the cultivars using these ISSR markers, and (3) to determine the correlations between the molecular-based similarity values and known pedigree information. ISSR-PCR fingerprints have not been documented in raspberries. Although pedigree studies have been conducted with red raspberries (CitationDale et al., 1989, Citation1993), these data have not been compared with ISSR-based similarity values.
MATERIALS AND METHODS
Plant Material and DNA Extraction
Thirteen raspberry genotypes (), maintained in the greenhouse under natural light conditions at a maximum photosynthetic photon flux of 90 μmol m−2 s−1 at 20 ± 2 (C, 85% RH, were evaluated in this study. Leaf tissues used for DNA extraction were collected from actively grown shoots, immediately placed on ice and then frozen at −80 °C until extraction. DNA was extracted by the GenElute Plant Genomic DNA Miniprep extraction kit (Sigma Chemical Co., Oakville, ON, Canada) following the manufacturer's instructions (CitationDebnath, 2007). The concentration of DNA was estimated spectrophotometrically (Ultrospec 2000, Pharmacia Biotech, Cambridge, UK) at 260 nm. DNA purity was measured by the ratio of the absorbance at 260 nm over 280 nm. Template DNA with an A260/A280 ratio of 1.8–2.1 in a dilution of 10 ng μL−1 was used for PCR.
TABLE 1. Raspberry cultivars and advanced selections sampled for ISSR analysis
ISSR Primers, PCR Amplification and Electrophoresis
A set of 100 primers representing di, tri, tetra, and pentamer repeats (UBC set 9) was procured from the Biotechnology Laboratory, University of British Columbia (UBC), Vancouver, BC, Canada. Out of these, 18 () that gave clear banding patterns were used in the final study. Different concentrations of template DNA and Taq polymerase were tested for optimal amplification. The optimized amplification reaction mixture (25 μL) contained 10 ng of DNA template, PCR buffer (50 mM KCl, 10 mM Tris-HCl pH 8.3, 1.5 mM MgCl2 and 0.001% [w/v] gelatin), 30 pmol primer, 200 μM of each dNTP, 1.25 U of Taq DNA polymerase (Sigma Chemical Co., Oakville, ON, Canada), and PCR grade dH2O (Sigma). DNA Reaction mixtures were amplified in a PTC-100 Programmable Thermal Controller (MJ Research Inc., Watertown, MA, USA) using an initial “hot start” of 94°C for 10 min, followed by 45 cycles of 1 min at 94°C, 1 min at annealing temperature () and 2 min at 72°C. The reaction was terminated with a final extension at 72°C for 10 min before holding the sample at 4°C for analysis. Optimal conditions were determined based on the resolvable PCR products generated by each primer. A negative control that contained all PCR components except DNA (replaced by water) was included in every experiment to test for DNA contamination of the reagents. Amplified fragments, along with a 1kb DNA ladder (Invitrogen, Burlington, ON, Canada), were resolved by 1.6% agarose gel electrophoresis in tris-borate-EDTA (TBE). After ethidium bromide staining for 35 min (0.5 μg L−1 of TBE) and a distilled water wash of 20 min, DNA was visualized using a trans-illuminating gel documentation system (GeneGenius, Syngene, Beacon House, Cambridge, UK).
TABLE 2. ISSR primers: their sequence, anchored end, repeat motif, and the data on DNA profile and polymorphism generated in nine raspberry cultivars and four advanced selections using 18 ISSR primersFootnote a
DNA amplification with each primer was repeated at least twice and congruence between replicates was verified. Gels were scored for polymorphic and monomorphic bands. Nonreplicated bands were eliminated from analyses. Bands of similar molecular weight and migration distance across individuals were assumed to be homologous (CitationAdams and Rieseberg, 1998). A 1 kb DNA ladder was used as a molecular weight standard.
Data Analysis
The ability of the most informative primers to differentiate between genotypes was assessed by calculating their Resolving power (Rp) (CitationPrevost and Wilkinson, 1999) that has been described to correlate strongly with the ability to distinguish between genotypes according to CitationGilbert et al. (1999):
where p is the proportion of the 13 genotypes containing the band. The Ib value was calculated for 18 informative ISSR primers.
The ISSR fragments were scored as present (1) or absent (0) for each of the markers. Since ISSR markers are typically dominant, it was assumed that each band represented the phenotype at a single bi-allelic locus. The presence of a band was interpreted as either a heterozygote or dominant homozygote and the absence of a band as a recessive homozygote. The basic data structure finally consisted of a binomial (0/1) matrix, representing the scored ISSR markers. Pairwise comparisons were calculated using the following coefficients, described by CitationSneath and Sokal (1973): Simple Matching coefficient: Sij = (a+d)/(a+b+c+d); Dice's coefficient: Sij = 2a/(2a + b + c), equivalent to the coefficient of CitationNei and Li (1979); and Jaccard's coefficient: Sij = a/(a+b+c), where Sij is the similarity between two individuals, i and j; a = bands shared by both individuals, b = bands presented by i but not by j; c = bands presented by individual j but not by i; and d = number of bands absent in both. Jaccard's and CitationNei and Li's (1979) coefficients calculate similarities based on shared presence but not the absence of DNA bands.
The similarity matrix was used as input data for cluster analysis by applying unweighted pair-group method with arithmetic averages (UPGMA) and to compute a principal coordinate (PCO) analysis (CitationGower, 1966) with the NTSYS-pc version 2.1 software program (CitationRohlf, 1998). The SAHN option was used to cluster the data according to the UPGMA method. Cophenetic matrices were created from the dendrogram and compared with the similarity matrix using the Mantel matrix comparison function in NTSYS to test whether clusters in the dendrogram agreed with information from the similarity matrix. The cophenetic correlation coefficient (CCC) value obtained gives a goodness of fit for the clusters (CitationRohlf, 1998). The similarity matrix was also used to perform a hierarchical analysis of molecular variance (AMOVA) (CitationExcoffier et al., 1992) by using Arlequin Software version 2.001 (CitationSchneider et al., 2000). This analysis enables partitioning of the total ISSR variation into within- and among-geographical region variation components and provides a measure of interregion genetic distances as the proportion of the total ISSR variation residing between raspberry genotypes of any two regions of breeding program (called Phi statistics; CitationExcoffier et al., 1992).
Pedigrees of nine cultivars were obtained from various sources including CitationJennings (1988), CitationAtila and Agaoglu (2006), and the Web sites of East Malling Kent, UK (http://www.emr.ac.uk/pdf/emr%20varieties.pdf), New York State Agricultural Experiment Station Bulletin (1885–1970) (http://ecommons.library.cornell.edu/handle/1813/4037), and Ribes-Rubus database (http://www.ribes-rubus.gf.vu.lt/RubusEDB.xls). The four unreleased breeding lines were not included. Coefficients of coancestry (= coefficient of parentage; CitationFalconer and Mackay, 1996) was computed for all pairwise combinations of nine cultivars with the Peditree software package (CitationVan Berloo and Hutten, 2005). UPGMA cluster analysis was performed as ISSR data analysis. To determine the level of correlation between the genetic similarity values calculated from the Jaccard-based ISSR data and pairwise coefficients of coancestry, Pearson product-moment correlation coefficients were calculated for nine cultivars.
RESULTS
ISSR Amplification
The selected primers and the maximum number of reproducible polymorphic bands produced by each primer are listed in . Of the 18 selected primers, 13 (seven 3′ mono-anchored, three 3′ di-anchored, and three 5′ tri-anchored) were designed to anneal to di-nucleotide repeats, three to tri-nucleotide, one to tetra-nucleotide, and one to penta-nucleotide repeats. The primers revealed the presence of polymorphisms in the amplified DNA fragments in a range from 300 to 3,300 bp. Representative banding pattern obtained with primer 866 are shown in . The number of polymorphic bands produced ranged from 11 for the primer 881 to 23 for the primers 801 and 835, with an average of 17 bands per primer. Under the optimized PCR conditions, the banding profiles were consistent among PCR experiments (data not shown).
FIGURE 1. ISSR banding pattern of 13 raspberry genotypes generated by primer 866. The genotype abbreviations are the same as in . 1KB: standard molecular size (1 kb ladder).
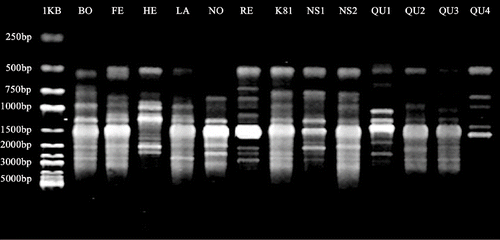
The ability of the 18 most-informative primers to differentiate raspberry genotypes was assessed on the basis of Rp. The Rp values varied between 4.0 for primer 881 and 11.2 for primer 801. Primers with higher Rp values were generally able to distinguish more genotypes and showed higher polymorphic bands ().
Genetic Relationships Among Raspberry Genotypes
The Simple Matching, Dice, and Jaccard coefficients were used to generate similarity matrices for ISSR cluster analysis. CCC measures the correspondence between the original genetic distances among samples and the distances defined along the phenogram (CitationSneath and Sokal, 1973). The CCC values for all three types of coefficients were very high, CCC for Jaccard was 0.88 and for Dice- and Simple-Matching-based cophenetic matrices was 0.87. The Jaccard-based matrix was used for UPGMA clustering ().
FIGURE 2. UPGMA (unweighted pair-group method with arithmetic averages) dendrogram estimating the genetic distance among 13 raspberry genotypes based on ISSR coefficient-derived Jaccard's similarity matrix.
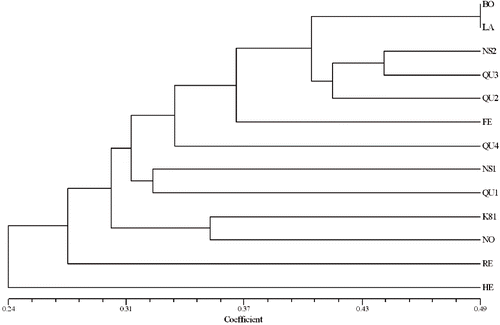
UPGMA clustering identified 11 genotypes into two clusters at a similarity index of approximately 0.30, leaving Heritage and Regency as outliers. Within the first main cluster, some subclusters were evident, which provided further information on the evaluated genotypes. Two subclusters were resolved in Cluster I: the first one with four cultivars and three breeding lines and the second subclusters with K93-9 and SJR941-1. In the first subcluster of Cluster I, Boyne and Latham were grouped together at 0.49 similarity value, and both were separated from K93-11. SJR941-18 and SJR942-7 at a similarity value of about 0.41. Within this subcluster, SJR944-2 was well separated from rest of the six genotypes at approximately 0.33 similarity value. K93-11 and SJR942-7 formed a group at about 0.44 similarity value and both of them were separated from SJR941-18 at a similarity value of approximately 0.42 in the first subcluster of Cluster I. K93-9 and SJR941-1 formed the second subcluster of Cluster I and were grouped together showing approximately 32% similarity. Two cultivars from Kentville, Nova Scotia, Canada: K81-6 and Nova formed Cluster II and were grouped together with each other at a similarity value of approximately 0.35 ().
AMOVA analysis indicated that geographical distribution for the place of cultivar development had little effect on the diversity of raspberry genotypes, and accounted for only 1.0% of the total variation. The major part of the variation was found among the genotypes (99.0% of the total variation).
PCO analysis on the frequencies of occurrence of the polymorphic ISSR markers among the genotypes confirmed the results of AMOVA: the plotting of the first three components, representing 11.6%, 10.3%, and 10.1% of the total variation for the first, second, and the third component, respectively, indicates that most of the variation was due to genotypes per se. No clear distinction between geographical localities of genotype origin could be drawn. Most of the genotypes are separated by the first or second or third PCO (), which demonstrates distinct groups of genotypes corresponding to cluster analysis (). The genotypes Boyne, Latham, SJR941-18, SJR942-7, and Festival were grouped together by the third axis () as was also observed by cluster analysis (). Similarly, Regency was well separated from rest of the genotypes corresponding to cluster analysis. However, Heritage, SJR944-2, K81-6, and K93-9 were clustered in PCO analysis although they were well separated in cluster analysis (). It is interesting to note that the third axis revealed another level of separation for Nova and this was not apparent from the dendrogram, thus showing the benefit of using more than one type of analysis ().
FIGURE 3. Three-dimensional plot of the principal coordinate (PCO) analysis of distance among 13 raspberry genotypes based on ISSR coefficient-derived Jaccard's similarity matrix.
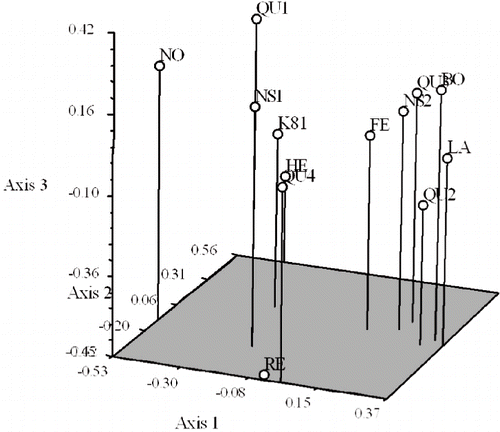
Coefficients of coancestry for nine released cultivars ranged from 0.005 between Latham and K93-9 to 0.250 between Boyne and Nova (). The coancestry coefficient-based UPGMA clustering for nine cultivars () identified two clusters at a similarity index of approximately 0.04 and leaved K93-9 as an outlier at 0.03 similarity index. In the first cluster, Nova and K93-11 were grouped together at a similarity index of 0.25 and both were separated from Boyne at approximately 0.19 similarity index. In the second cluster, Festival and Heritage were grouped together with approximately 0.13 similarity value and both of them formed a subcluster with Regency at a similarity value of 0.09. K81-6 was separated from Festival, Heritage, and Regency at approximately 0.06 similarity value in the second cluster (). The correlation coefficient between the genetic similarity values calculated from the Jaccard-based ISSR data and pairwise coefficients of coancestry of nine cultivars was positive but insignificant (r = 0.18).
TABLE 3. Coefficients of coancestry for all possible pairs of the nine raspberry cultivarsFootnote a
DISCUSSION
The ISSR markers were used in this study to evaluate the levels of genetic relatedness among nine North American raspberry cultivars and four Canadian advanced selections. The annealing temperature is a very important factor that affects the PCR amplification profile. Different primers require a specific annealing temperature. Primers with 3′-anchors were more successful in amplifying specific bands than primers with 5′-anchors. Although AT di-nucleotide SSRs are the most abundant and are highly polymorphic in plant genomes (CitationWang et al., 1994), the use of ISSR primers consisting of the bases A and T made it difficult to amplify specific bands that might be due to self-complementarity within the primer (CitationFang and Roose, 1997). Tri-, tetra-, and penta-nucleotide ISSR primers without an anchor were also successfully optimized in the present study. The optimization of these ISSRs with a long repeat motif suggests that ISSRs with a long repeat motif are abundant along the red raspberry genome.
Eighteen primers detected enough genetic variation and relatedness among the genotypes to allow for complete differentiation as was revealed from PCR-derived gel analysis. The ISSR primers varied in their ability to distinguish raspberry genotypes, and primers with high Rp values were generally more effective in distinguishing between genotypes (data not shown). This is in agreement with the findings of CitationDebnath (2007) in cloudberry, CitationPrevost and Wilkinson (1999) in potato, and of CitationGilbert et al. (1999) in lupin. The present data provide evidence of a genetic similarity and divergence among the tested genotypes. Previous studies reported molecular diversity analysis of raspberries using RAPD markers (CitationParent et al., 1993; CitationGraham et al., 1994, 1997).
This is the first known report on the use of ISSR markers for creating genetic fingerprints and determining genetic relationships among red raspberry genotypes. The results clearly demonstrate that ISSR markers can be used in a genetic diversity study as well as in genotypic identification of red raspberries, as noted for other plant species by CitationPrevost and Wilkinson (1999), CitationCasasoli et al. (2001), CitationArchak et al. (2003), CitationVijayan and Chatterjee (2003), CitationGardner and Hokanson (2005), and CitationDebnath (2007) or in helping breeders to select diverse parents with similar phenotypes for breeding.
Two different methods of multivariate molecular analysis, PCO and cluster analysis, were used to group the individuals in this study, as each method is designed to sort the data on a different basis and therefore can be used comparatively to elucidate relationships. The UPGMA clustering algorithm assumes a constant evolutionary rate among accessions or clongenotypes. The results of the present study showed 24% to 49% of genetic similarity among 13 raspberry cultivars and breeding lines. Based on 10 RAPD markers with 10 cultivars, CitationGraham et al. (1994) found more than 70% similarity among the modern raspberry cultivars grown in Scotland and reported that the cultivar Latham (derived from R. idaeus ssp. strigosus) displayed only 30% to 40% similarity to R. idaeus ssp. idaeus-derived raspberry cultivars. Another study by CitationTrople and Moore (1999) revealed through a similarity index from RAPD markers that the R. idaeus ssp. idaeus-derived cultivars Meeker and Tulameen were 77% similar. The comparison of measures of genetic similarity among different studies is not straightforward. Incongruity between various DNA marker systems is not uncommon (see CitationDegani et al., 2001). In the present study, out of 13 raspberry cultivars and lines, 9 were grouped in one cluster with about 31% similarity. K93-9 and K93-11, although derived from one common parent Glen Moy, were grouped into two clusters. Boyne, developed at Manitoba, has the maximum similarity with Latham that was developed in Minnesota. The Jaccard's similarity coefficient between K93-9 and the rest of the genotypes indicated that it is distantly related to the other genotypes.
Cluster analysis of the pedigree data yielded results that were more or less expected. The raspberry cultivar K93-11 was derived from Nova and both were grouped together at 25% similarity. Similarly, Boyne is one of the parents of Nova and grouped together with each other at a coancestry similarity value of approximately 0.19 ().
Coancestry and genetic similarity estimates based on DNA markers represent different approaches to determine genetic similarity among genotypes. Coancestry is an indirect measure that infers the degree of similarity between two individuals from their pedigree relationship, under several simplifying assumptions. In contrast, ISSR-based estimates evaluate the resemblance of two genotypes by direct sampling of the genome. Out of nine raspberry cultivars, eight were grouped into two clusters by coancestry UPGMA analyses, K93-9 did not group with any cluster (). However, clustering based on ISSR data was different from that based on the coefficient of coancestry, although both analyses showed considerable genetic diversity among the cultivars. Poor correlation was observed between the genetic similarity values calculated from the Jaccard-based ISSR data of nine cultivars and pairwise coefficients of coancestry. Similar results were also reported by CitationDegani et al. (2001) in strawberry who observed a poor correlation between the AFLP-derived genetic similarity values with those of the coefficients of coancestry but a better correlation between the RAPD-derived values and the coefficients of coancestry in 19 strawberry cultivars. In the present study, the degree of similarity as calculated from parentage was generally lower than those calculated from banding patterns. Similar results were also reported by CitationGraham et al. (1994). Poor correlations between genetic similarities values and coefficients of coancestry might be due to inaccuracy in estimates. The assumptions underlying the coefficient of coancestry calculations may introduce inaccuracies in these estimates (CitationMessmer et al., 1993). The pedigree data can be subjective and does not account for the effects of selection, mutation, and inadequate simplification in the underlying model that assumes equal parental contributions (CitationSouza and Sorells, 1989). These estimates may be biased due to selection pressure, unequal parental contribution, and the relatedness of ancestors without a known pedigree. DNA markers have the advantage of directly detecting sequence variation among genotypes and therefore the ability to bypass the assumptions that are inherent to pedigree analysis. DNA markers may be affected by the number of markers analyzed, their distribution over the genome, and the accuracy in scoring the marker (CitationSchut et al., 1997). Finally, incongruities can result from the clustering process whenever clusters are nonoverlapping due to which a genotype that is related to two other genotypes from separate clusters will only be grouped with the one to which it is most closely related. Therefore the pedigree and ISSR matrices should not be considered as relating to one another in a meaningful way. Each has it own merits, but they should be interpreted separately.
CONCLUSIONS
This study should facilitate the use of ISSR fingerprints making this technology valuable for cultivar identification and for the more efficient choice of parents in our current raspberry breeding program. The study identified primers that generated substantial polymorphism among raspberry cultivars and advanced selections developed in Canada and the United States. Similar genetic analysis might also be applicable to other raspberry cultivars.
Acknowledgments
The author thanks Dr. S. Khanizadeh of Horticultural Research and Development Centre, St-Jean-sur-Richelieu, QC, Canada and Dr. A.R. Jamieson of Atlantic Food and Horticulture Research Centre, Kentville, Canada for supplying raspberry cultivars and breeding lines. Thanks are also due to Darryl Martin, Sarah Devine, Glen Chubbs, and Shawn Foley for their excellent technical help. This work is the Atlantic Cool Climate Crop Research Centre contribution no. 199.
LITERATURE CITED
- Adams , R.P. and Rieseberg , L.H. 1998 . The effects of non-homology in RAPD bands on similarity and multivariate statistical ordination in Brassica and Helianthus. . Theor. Appl. Genet. , 97 : 323 – 326 .
- Archak , S. , Gaikwad , A.B. , Gautam , D. , Rao , E.V.V.B. , Swamy , K.R.M. and Karihaloo , J.L. 2003 . DNA fingerprinting of Indian cashew (Anacardium occidentale L.) varieties using RAPD and ISSR techniques . Euphytica , 130 : 397 – 404 .
- Arnau , G. , Lallemand , J. and Bourgoin , M. 2002 . Fast and reliable strawberry cultivar identification using inter simple sequence repeat (ISSR) amplification . Euphytica , 129 : 69 – 79 .
- Atila , S.P. and Agaoglu , Y.S. 2006 . Developments in raspberry breeding . Res. J. Agr. Biol. Sci. , 2 : 171 – 177 .
- Casasoli , M. , Mattioni , C. , Cherubini , M. and Villani , F. 2001 . A genetic linkage map of European chestnut (Castanea sative Mill.) based on RAPD, ISSR and isozyme markers . Theor. Appl. Genet. , 102 : 1190 – 1199 .
- Cousineau , J.C. and Donnelly , D.J. 1992 . Use of isoenzyme analysis to characterize raspberry cultivars and detect cultivar mislabeling . HortScience , 27 : 1023 – 1025 .
- Dale , A. , Moore , P.P. , McNicol , R.J. and Sjulin , T.M. 1989 . Pedigree analysis of red raspberry . Acta Hort. , 262 : 35 – 39 .
- Dale , A. , Moore , P.P. , McNicol , R.J. , Sjulin , T.M. and Burmistrov , L.A. 1993 . Genetic diversity of red raspberry varieties throughout the world . J. Amer. Soc. Hort. Sci. , 118 : 119 – 129 .
- De Ancos , B. , Gonza´les , E.M. and Cano , M.P. 2000 . Ellagic acid, vitamin C, and total phenolic contents and radical scavenging capacity affected by freezing and frozen storage in raspberry fruit . J. Agr. Food Chem. , 48 : 4565 – 4570 .
- Debnath , S.C. 2007 . Inter-simple sequence repeat (ISSR)-PCR analysis to assess the genetic diversity in a collection of wild cloudberry (Rubus chamaemorus L.) clones . J. Hort. Sci. Biotechnol. , 82 : 727 – 732 .
- Degani , C. , Rowland , L.J. , Saunders , J.A. , Hokanson , S.C. , Ogden , E.L. , Golan-Goldhirsh , A. and Galletta , G.J. 2001 . A comparison of genetic relationship measures in strawberry (Fragaria x ananassa Duch.) based on AFLPs, RAPDs, and pedigree data . Euphytica , 117 : 1 – 12 .
- Excoffier , L. , Smouse , P. and Quattro , J. 1992 . Analysis of molecular variance inferred from metric distances among DNA haplotypes: Application to human mitocondrial DNA restriction data . Genetics , 131 : 479 – 491 .
- Falconer , D.S. and Mackay , T.F.C. 1996 . Introduction to quantitative genetics. , 4th , Harlow, , UK : Prentice Hall .
- Fang , D.Q. and Roose , M.L. 1997 . Identification of closely related citrus cultivars with inter-simple sequence repeat markers . Theor. Appl. Genet. , 95 : 408 – 417 .
- Gardner , N. and Hokanson , S.C. 2005 . Intersimple sequence repeat fingerprinting and genetic variation of Clematis cultivars and commercial germplasm . HortScience , 40 : 1982 – 1987 .
- Gilbert , J.E. , Lewis , R.V. , Wilkinson , M.J. and Caligari , P.D.S. 1999 . Developing an appropriate strategy to assess genetic variability in plant germplasm collections . Theor. Appl. Genet. , 98 : 1125 – 1131 .
- Gower , J.C. 1966 . Some distance properties of latent root and vector methods used in multivariate analysis . Biometrika , 53 : 325 – 338 .
- Graham , J. and McNicol , R.J. 1995 . An examination of the ability of RAPD markers to determine the relationships within and between Rubus species . Theor. Appl. Genet. , 90 : 1128 – 1132 .
- Graham , J. , McNicol , R.J. , Greig , K. and van de Ven , W.T.G. 1994 . Identification of red raspberry cultivars and an assessment of their relatedness using fingerprints produced by random primers . J. Hort. Sci. , 69 : 123 – 130 .
- Graham , J. , Squire , G.R. , Marshall , B. and Harrison , R.E. 1997 . Spatially dependent genetic diversity within and between colonies of wild raspberry Rubus idaeus detected using RAPD markers . Mol. Ecol. , 6 : 1001 – 1008 .
- Jennings , D.L. 1988 . Raspberries and blackberries: Their breeding, diseases and growth , Academic Press : London .
- Jennings , D.L. , Daubeny , H.A. and Moore , J.N. 1990 . Blackberries and raspberries . Acta Hort , 290 : 329 – 391 .
- Kollmann , J. , Steinger , T. and Roy , B.A. 2000 . Evidence of sexuality in European Rubus (Rosaceae) species based on AFLP and allozyme analysis . Amer. J. Bot. , 87 : 1592 – 1598 .
- Messmer , M.M. , Melchinger , A.E. , Hermann , R.E. and Boppenmaier , J. 1993 . Relationships among European maize inbreds: II. Comparison of pedigree and RFLP data . Crop Sci. , 33 : 944 – 950 .
- Nei , M. and Li , W.H. 1979 . Mathematical model for studying genetic variation in terms of restriction endonucleases . Proc. Natl. Acad. Sci. USA , 76 : 5269 – 5273 .
- Nybom , H. 1995 . Evaluation of interspecific crossing experiments in facultatively apomictic blackberries (Rubus subgen. Rubus) using DNA fingerprinting . Hereditas , 122 : 57 – 65 .
- Parent , J.G. , Fortin , M.G. and Page , D. 1993 . Identification of raspberry cultivars by random amplified polymorphic DNA (RAPD) analysis . Can. J. Plant Sci. , 73 : 1115 – 1122 .
- Prevost , A. and Wilkinson , M.J. 1999 . A new system of comparing PCR primers applied to ISSR fingerprinting of potato cultivars . Theor. Appl. Genet. , 98 : 107 – 112 .
- Qian , W. , Ge , S. and Hong , D.Y. 2001 . Genetic variation within and among populations of a wild rice Oryza granulate from China detected by RAPD and ISSR markers . Theor. Appl. Genet. , 102 : 440 – 449 .
- Reddy , P.M. , Sarla , N. and Siddiq , E.A. 2002 . Inter simple sequence repeat (ISSR) polymorphism and its application in plant breeding . Euphytica , 128 : 9 – 17 .
- Rohlf , F.J. 1998 . “ NTSYS-pc. Numerical taxonomy and multivariate analysis system, version 2.0. ” . In Exeter software , New York : Setauket .
- Schneider , S. , Roessli , D. and Excoffier , L. 2000 . “ Arlequin, version 2.000 ” . In A software for population genetics data analysis , Switzerland : Genetics and Biometry Laboratory, University of Geneva .
- Schut , J.W. , Qi , X. and Stam , P. 1997 . Association between relationship measures based on AFLP markers, pedigree data and morphological traits in barley . Theor. Appl. Genet. , 95 : 1161 – 1168 .
- Sneath , P.H.A. and Sokal , R.R. 1973 . “ Numerical taxonomy ” . In The principles and practice of numerical classification , San Francisco, California : W.H. Freeman and Company .
- Souza , E. and Sorells , M.E. 1989 . Pedigree analysis of North American oat cultivars released from 1951 to 1985 . Crop Sci. , 29 : 595 – 601 .
- Trople , D.D. and Moore , P.P. 1999 . Taxonomic relationships in Rubus based on RAPD analysis . Acta Hort. , 505 : 373 – 378 .
- Van Berloo , R. and Hutten , R.C.B. 2005 . Peditree: Pedigree database analysis and visualization for breeding and science . J. Hered. , 96 : 465 – 468 .
- Vijayan , K. and Chatterjee , S.N. 2003 . ISSR profiling of Indian cultivars of mulberry (Morus spp.) and its relevance to breeding programs . Euphytica , 131 : 53 – 63 .
- Wang , Z. , Weber , J.L. , Zhong , G. and Tanksley , S.D. 1994 . Survey of plant short random DNA repeats . Theor. Appl. Genet. , 88 : 1 – 6 .
- Waugh , R. , van de Ven , W.T.G. , Phillips , M.S. and Powell , W. 1990 . Chloroplast DNA diversity in the genus Rubus (Rosaceae) revealed by Southern hybridization . Plant Syst. Evol. , 172 : 65 – 75 .
- Weber , C.A. 2003 . Genetic diversity in black raspberry (Rubus occidentalis L.) detected by RAPD markers . HortScience , 38 : 269 – 272 .
- Weising , K. , Nybom , H. , Wolff , K. and Meyer , W. 1995 . DNA fingerprinting in plants and fungi , Boca Raton, , Florida : CRC Press, Inc .
- Zietkiewicz , E. , Rafalski , A. and Labuda , D. 1994 . Genome fingerprinting by simple sequence repeat (SSR)-anchored polymerase chain reaction amplification . Genomics , 20 : 176 – 183 .