ABSTRACT
To find out more about the biochemical aspects of seed-softness trait in pomegranate (Punica granatum L.), some of the phenylpropanoid pathway enzymes as well as their products were evaluated in the various soft- and hard-seeded genotypes during fruit development. Results showed that peroxidase (POD) activity decreased in all studied genotypes during fruit growth, while increasing at the last stages only in the hard-seeded genotypes. Although laccase (LAC) activity did not show high differences during fruit ripening and in different genotypes, but its general trend was increasing. Phenylalanine ammonia-lyase (PAL) activity decreased during the fruit development and approximately diminished for the hard-seeded genotypes. All genotypes had lignin components in their seeds and amount of lignin increased during the growth season. Total phenolic decreased drastically and antioxidant capacity increased gradually showing an opposite trend and probable competitive effect for the same substrate during fruit development. Considerable differences that were observed in the POD activity at the last sampling stage, and higher activity that was observed in POD than LAC, may indicate that POD is more important for polymerization of lignin in the pomegranate seed and this enzyme might have some role in the development of seed softness trait in pomegranate. Also, this is the first report about lignin detection in the seeds of soft-seeded pomegranate genotypes, and represents a different mechanism (such as monolignol conformation in the final polymer) from lignin content for development of the soft-seeded pomegranate genotype.
Introduction
Pomegranate (Punica granatum L.) is a subtropical fruit tree that has been cultivated from old times. In addition to its superior consumer preference, pomegranate is an extremely healthful food choice because of its elevated amount of human health-bioactive compounds. Most of the researchers believe that this fruit tree is native to Persia, where wild forests of the tree exist, and was spread by humans to a much broader area in prehistory (Mars, Citation2000; Stover and Mercure, Citation2007). Regarding the wide areas of pomegranate cultivation, Iran has many different genotypes that contain various noticeable traits. One of the desirable fruit traits in pomegranate is seed softness. Similar to the hard-seeded pomegranate genotypes, soft-seeded ones have the entire arils component, but their integument covers (testa) remain soft and the arils are completely soft. Because of its useful seed reservoirs especially the unsaturated fatty acids and the anticancer properties, seed-softness pomegranate genotypes are more nutritive and also more palatable for the consumers (Sarkhosh et al., Citation2009).
The integument of pomegranate seeds is mostly believed to be composed of lignin polymers (Dalimov et al., Citation2003). Lignin is a phenolic plant polymer that imparts strength to the plant secondary cell wall and is essential for mechanical support, water transport, and defense in vascular terrestrial plants. Lignin polymer is consisted from three hydroxycinnamyl alcohol subunits, namely, p-coumaryl, coniferyl, and sinapyl alcohol resulting in hydroxyphenyl (H), guaiacyl (G), and syringyl (S) types of lignin, respectively (Boerjan et al., Citation2003).
Precursors for lignin biosynthesis are synthesized from L-phenylalanine via the phenylpropanoid pathway. Therefore, the first step of monolignol synthesis is catalysis by phenylalanine ammonia-lyase (PAL) (Elkind et al., Citation1990). After monolignols construction, they will be connected in an irregular manner to organize the lignin polymer.
Lignin polymerization is a coupling reaction between two radicals and formation of the radicals is catalyzed by H2O2 dependent peroxidase (POD) and/or O2 dependent laccase (LAC) (Harkin and Obst, Citation1973; Savidge and Udagama-Randeniya, Citation1992).
PODs are currently considered more important for lignin polymerization. Besides their role in lignification, these enzymes have been involved in a broad range of physiological processes, such as senescence, auxin metabolism, the cross-linking of cell wall proteins, defense against pathogenic attack, and a variety of abiotic stress tolerances (Passardi et al., Citation2005).
LACs are multi-copper-containing glycoproteins and have been found to be widespread in higher plants, both in monocots and dicots. Several authors reported that these enzymes are associated with lignification and isolated these enzymes from lignified tissues (Cai et al., Citation2006; Gavnholt and Larsen, Citation2002; Sterjiades et al., Citation1992). Plant LACs were also reported to play a role in iron metabolism, formation of proanthocyanidin or tannin, as well as responses to the stress, and are essential for normal cell wall structure and integrity (Pourcel et al., Citation2005; Ranocha et al., Citation2002).
Antioxidant and phenolic compounds are two major groups in the phenylpropanoid pathway that branch out from the pathway; hence, better understanding of their content in relation to other substances, such as lignin, may be useful for better elucidation of the seed-softness trait in the pomegranate arils. In fact, a better understanding of the phenylpropanoid pathway will be applicable to understand a general mechanism of seed coat hardening in pomegranate arils.
With regards to the importance of seed-softness, no investigation is available about the biochemical features of the arils and their differences among the hard- and soft-seeded genotypes. In fact, analysis of different biochemical substances among the soft- and hard-seeded pomegranate genotypes, especially at different developmental stages with different degrees of hardness, is useful to elucidate the distinctions of these genotypes and could be the starting point for revealing how this trait is developed. It is clear that a better understanding of this trait will improve our knowledge about the mechanism of seed-softness formation. This information would be beneficial for identification of the genetic basis of seed-softness in pomegranate and, hence, might be applicable for breeding programs to improve the quality of pomegranate genotypes.
To find out more about pomegranate seed hardening, in the present study, some of the phenylpropanoid pathway enzymes and products were analyzed in the arils and seeds of six pomegranate genotypes during different developmental stages from fruit set to ripening.
Materials and methods
Six pomegranate genotypes () were selected from National Pomegranate Collection of Yazd city (longitude 54.16° E, latitude 31.55° N, and altitude 1200 m) located in central Iran with hot and dry climate condition in 2013. These genotypes were 24 years old and selected according to preliminary morphological studies and had different degrees of seed hardness. Fruits were harvested at different sampling times from fruit set to ripening. Sampling times were as follows: 20 days after fruit set (DAF) (10 June), 60 DAF (21 July), 80 DAF (11 Aug.), 105 DAF (6 Sept.), and 130 DAF (2 Oct.). Arils were immediately frozen using liquid nitrogen and stored at –80 °C until analysis. Regarding the differences in the seed covering and to obtain a uniform powder in the soft and hard seed genotype, the fleshy part of the arils was grounded for antioxidant, total phenolic content, as well as enzyme activity assay, and seeds (without fleshy parts) were used for lignin analysis.
Table 1. Studied genotypes and some of their general attributes.
Chemicals are listed as follows: sodium phosphate, polyvinylpolypyrrolidone (PVPP), ethylenediaminetetraacetic acid (EDTA), guaiacol, L-phenylalanine, Tris-HCl, NaOH, gallic acid, HCl, 2,2-diphenyl-1-pic-rylhydrazyl (DPPH), and Folin–Ciocalteu (Merck, Darmstadt, Germany), β-mercaptoethanol, H2O2, and thioglycolic acid (Sigma Aldrich, St. Louis, MO, USA).
Enzyme assay
POD was extracted from 350 mg of powdered tissues from arils by using 1.5 ml of sodium phosphate buffer 100 mM (pH 7.0) containing 2% PVPP and 1.4 mM EDTA, and was assayed spectrophotometrically using a guaiacol substrate as described by Chance and Maehly (Citation1955). The reaction mixture consisted of 150 µl supernatant in 600 µl buffer sodium phosphate 100 mM, with 270 µl guaiacol 2% and 170 µl H2O2 1%. The increase in absorbance at 470 nm at 30 °C was recorded for 3 min and enzyme activity was expressed as kkat/ml.
LAC activity was measured based on guaiacol oxidation using the procedure of Erkurt et al. (Citation2007). For this purpose, 1.5 ml of sodium phosphate buffer 100 mM (pH 6.0) was added to 350 mg of grounded aril tissues and centrifuged at 20,000 g for 15 min. The reaction mixture contained 300 µl supernatant, 600 µl sodium phosphate buffer 100 mM (pH 6.0), and 100 µl guaiacol 200 mM. This mixture was incubated at 30 °C for 60 min and increase in absorbance at 465 nm was measured using the spectrophotometer and enzyme activity was expressed as kat/ml.
PAL activity was assayed based on the ability of this enzyme to convert l-phenylalanine to the trans-cinnamic acid, according to the method described by Olsen et al. (Citation2008) with some modification. To extract the PAL enzyme, 140 mg of grounded aril tissues was thoroughly homogenized with 2 ml of extraction buffer containing 100 mM Tris-HCl (pH 8.8), 12 mM β-mercaptoethanol, and 1% PVPP. After centrifugation at 20,000 g for 20 min at 4 °C the supernatant was used for PAL assay. The reaction mixture consisted of 400 µl enzyme extract, 500 µl 100 mM Tris-HCl buffer (pH 8.8), 100 µl 100 mM l-phenylalanine. This mixture was incubated at 40 °C for 2 h and the reaction was terminated by adding 100 µl 5 M HCl. Control reactions were run in the same way as the assays, only 100 µl 5 M HCl was added before l-phenylalanine. The differences between assay and control absorbance in 290 nm was recorded. PAL activity was expressed as kkat trans-cinnamic acid formed per ml extract per hour.
Catalase (CAT) activity was determined according to Aebi (Citation1984). To the 350 mg of grounded tissues from arils was added 1.5 ml of sodium phosphate buffer 100 mM (pH 7.0) containing 2% PVPP and 1.4 mM EDTA. After centrifugation at 20,000 g for 15 min at 4 °C, the supernatant was used for enzymatic assay. The reaction mixture contained 30 mM H2O2 in a 50 mM phosphate buffer (pH 7.0), and 100 µl aril extract in a total volume of 1 ml. Catalase (CAT) activity was estimated spectrophotometrically by decrease in absorbance of H2O2 at 240 nm and was expressed as kkat/ml.
Lignin assay and seed hardness
Lignin was extracted with thioglycolic acid and measured spectrophotometrically according to the method of Bruce and West (Citation1989). To start, 50 mg of grounded pomegranate seeds (without fleshy part) were homogenized in 2 ml of 99.5% ethanol and centrifuged at 20,000 g for 20 min. The supernatant was discarded and the pellet was re-suspended in 2 ml of 99.5% methanol then centrifuged at 20,000 g for 20 min. After discarding the supernatant, the pellet was allowed to dry overnight. Next, 1800 µl of 2 M HCl and 200 µl of thioglycolic acid were added to the pellet and then the tubes were sealed using parafilm and incubated at 100 °C for 8 h and vortexed several times during incubation. After centrifugation at 20,000 g for 20 min at 4 °C, the pellet was washed twice with distilled water, and then 1400 µl of 1 M NaOH was added and incubated in a shaker at 25 °C for 18 h. The tubes were centrifuged at 20,000 g for 20 min at 4 °C, then the supernatant was transferred to the test tube and 240 µl of concentrated HCl was added and incubated at 4 °C for 4 h. After centrifugation at 20,000 g for 15 min at 4 °C and discarding the supernatant, the pellet was dissolved in 1 ml of 1 M NaOH. The absorbance was measured against a NaOH blank at 280 nm, and data were expressed as equivalent of mg lignin in ml. Seed hardness was evaluated using the method previously described by Zarei et al. (Citation2013).
Total phenolic content
The content of total phenols was determined by using the Folin–Ciocalteu colorimetric method, based on the reaction of the reagent with the functional hydroxyl groups of phenols according to the method of Velioglu et al. (Citation1998). To the 500 mg grounded aril tissues, 5 ml of 80% methanol was added. This mixture was incubated at room temperature on a shaker in dark conditions for 24 h and then was filtered using No. 1 Whatman filter paper. To measure the total phenolic content, 150 µl of filtered extract was added to the 1500 µl Folin–Ciocalteu (previously diluted ten-fold with distilled water) and was allowed to stand at room temperature for 1 min. Following the addition of 1500 µl of 6% sodium carbonate, total polyphenols were determined after 1 h of incubation at room temperature using a spectrophotometer at 725 nm. A standard curve was depicted using a different concentration of gallic acid and the total phenolics were expressed as mg/100 g gallic acid equivalents (GAE).
Antioxidant activity
Antioxidant activity was measured using 2,2-diphenyl-1-pic-rylhydrazyl (DPPH) radical as described by Moon and Terao (Citation1998). Initially, 100 µl of aril juice was mixed with 900 µl buffer Tris-HCl (pH 7.4) and 1000 µl of 500 µM DPPH was added. The reaction mixture was incubated at 25 °C for 30 min and absorbance was measured at 517 nm. The absorbance of each reaction mixture without DPPH was used as control and the antioxidant activity was calculated using the following equation:
where A is absorbance.
All of the photometry analyses were performed using a UV-spectrophotometer (Lambda EZ201, Perkin Elmer, Waltham, MA, USA).
Statistical analysis
SAS software ver. 9.1.3 (SAS Institute Inc., Cary, NC, USA) was used to analyze the data as a factorial experiment. Data were represented as mean of triplicates ± SE. Duncan’s multiple range test was employed to determine the statistical significance of the differences among the mean values.
Results and discussion
Analysis of variance showed that the measured biochemical factors were significantly (P < 0.01) different for different genotypes and for different developmental stages ().
Table 2. Analysis of variance (mean squares) for the measured biochemical factors in different pomegranate genotypes at different developmental stages.
POD activity
POD activity showed significant differences during the growth season (). At the first stage of sampling, POD activity was relatively high in all genotypes and its activity was higher in the hard seed genotypes. At 60 DAF its activity decreased in all genotypes and in ‘Torshe-Zabol’ was higher than other genotypes. It is noteworthy that this genotype had the hardest seed among the studied genotypes.
Figure 1. Change in activity of POD (A), LAC (B), PAL (C), and CAT (D) enzymes in various hard- and soft-seeded genotypes during different sampling date. (Data are mean of three replications ± SE.). DAF: Day after fruit set.
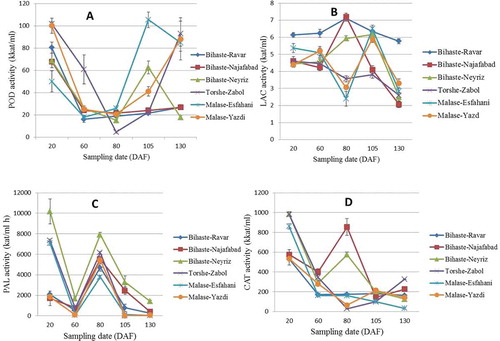
Except for the ‘Torshe-Zabol’ that showed a tangible decrease compare to the previous stage, at 80 DAF POD activity did not show significant differences compared with 60 DAF, and at this stage POD activity of all genotypes was minimal. POD activity was increased in the hard-seeded genotypes and a soft-seeded genotype (‘Bihaste-Neyriz’) at 105 DAF but in two other soft-seeded ones (‘Bihaste-Ravar’ and ‘Bihaste-Najafabad’) did not show significant differences. The activity of this enzyme showed a noticeable increase in the hard-seeded genotypes and reached more than four times that of the soft-seeded ones at 130 DAF.
Aydin and Kadioglu (Citation2001) analyzed the POD activity during the development of medlar fruits and observed the same activity pattern. These authors reported that POD activity was higher in the beginning of fruit development as well as during the final stage of ripening and attributed this higher activity to the higher metabolic activity during these developmental stages, especially anthocyanin metabolism as well as reducing the astringent taste of the fruit at ripening. By study on enzymes activity during tomato growth, Thompson et al. (Citation1998) reported that POD activity dramatically increased as fruit expansion generally ceased. Study on POD activity during date palm growth revealed that activity of this enzyme was low at the beginning and increased drastically during development of various cultivars, so that the maximum activity was observed at the Bisir, Kimri, and Rutab stages (up to cultivar), afterwards decreased to the maturity (Awad et al., Citation2011). Also, POD activity increased during papaya fruit development (Silva et al., Citation1990), while its activity decreased in strawberry (Civello et al., Citation1995).
In all cited investigations, increase or decrease in POD activity showed a similar trend in all studied cultivars, but in the present study results of POD activity revealed a different trend in the soft- and hard-seeded genotypes at least at the final stages of sampling. Also, hard-seeded genotypes showed higher activity compared to the soft-seeded ones during the fruit development.
POD is one of the key antioxidant enzymes in which its activity increases in response to the various stresses to protect the plant against unfavorable conditions (Ara et al., Citation2013).
It is noteworthy that these plants have been cultivated together and the same horticultural practices were performed on them, also sampling and the laboratory conditions were the same; therefore, it is unlikely that higher activity of POD observed in the hard-seeded genotypes have been triggered as a result of response to the various stresses. To identify the amount of the POD activity that may have been caused by a response to the stress, CAT (another important antioxidant enzyme) activity was measured simultaneously. Results indicated there are no significant differences among the studied genotypes, confirming that these genotypes had not been under severe stresses. Based on these observations and regarding the higher POD activity in the hard-seeded genotypes at ripening stage, it might be concluded that POD activity may have an effect on the seed-softness trait in pomegranate. It should be considered that differences between hardness in the seeds of soft- and hard-seeded pomegranate genotypes were recognizable at 60 DAF.
LAC activity
LAC activity was relatively low in pomegranate arils and although statistically significant, this enzyme did not show much difference during the development of pomegranate fruit (). However, generally speaking, activity of this enzyme showed a decreasing trend during the growth season. At the first stage of sampling, ‘Bihaste-Ravar’ (a soft-seeded genotype) showed the highest activity and afterwards ‘Malase-Esfahani’ (a hard-seeded genotype) had higher activity compared to the other genotypes. Studied genotypes did not show significant differences at 60 DAF compared with the previous stage. LAC activity increased a little in the soft-seeded genotypes and decreased in the hard-seeded ones at the third stage of sampling. At the fourth stage, LAC activity did not show a uniform trend for all genotypes and increased in some and decreased in some others. At the last sampling, the activity decreased in all genotypes except the ‘Bihaste-Ravar’ that still had relatively high activity.
Harel et al. (Citation1970) studied the LAC activity of peach fruit during fruit growth and reported that this enzyme had much less activity compared to the catechol oxidase, which was undetectable at the beginning stages and increased during the fruit development. Popescu et al. (Citation2009) followed the physico-chemical changes during the maturation of five white grapes and observed the same trend of activity in all studied genotypes. These authors reported that LAC activity increased during the berry development and reached the highest value at full maturity, and then decreased till harvesting. Also, in the present work, LAC activity decreased at the last sampling in most of the studied genotypes.
Although in this study some differences was observed in LAC activity among different pomegranate genotypes, these differences showed no correlation with seed-softness, and it is unlikely that this enzyme plays a crucial role in the pomegranate seed hardness and its activity may implicate the role in cell wall formation and not necessarily in the lignification process (Mayer and Staples, Citation2002).
PAL activity
PAL is the first enzyme in the phenylpropanoid pathway and plays a key role in the biosynthesis of phenolic compounds in plants (Tomas-Barberan and Espin, Citation2001). PAL activity was the greatest at the early stage of fruit growth for some genotypes, and the highest activity was recorded for ‘Bihaste-Neyriz’ (a soft-seeded genotype) (). In the next stage PAL activity decreased rapidly for all genotypes, and then increased at the third sampling date. For the two last sampling dates, the activity decreased for all studied genotypes and diminished for the hard-seeded genotypes to an undetectable level. This trend is consistent with reported results from strawberry (Cheng and Breen, Citation1991), loquat (Ding et al., Citation2001), litchi (Sun et al., Citation2009), and longan (Yu et al., Citation2010) fruits. Interestingly, at 105 and 130 DAF, hard-seeded genotypes showed significantly lower PAL activity compared to the soft-seeded ones, this may indicate a role for this enzyme to influence the development of the seed-softness trait in pomegranate.
Generally, the synthesis of phenolic compounds in the plant tissues is largely related to the PAL activity (Jones, Citation1984); results of the present study showed that the trend of PAL activity is similar to content of total phenolics during fruit development.
CAT activity
CAT activity showed the highest activity at 20 DAF for most genotypes, and then decreased gradually to the ripening stages (). Only two soft-seeded genotypes (‘Bihaste-Najafabad’ and ‘Bihaste-Neyriz’) showed somewhat of an increase in the CAT activity at 80 DAF, indicating these that two genotypes were exposed to the stresses at this stage. Therefore, some of the increases recorded in the POD activity about ‘Bihaste-Neyriz’ could be influenced by stresses. For the other stages there was no significant difference between soft- and hard-seeded genotypes.
Seed hardness and lignin measurement
Quantitative results of required force to rapture seed during fruit and seed development showed that at first sampling date all of the genotypes had soft seeds, and then seed hardness increased in the hard-seeded genotypes while remaining low in the soft-seeded ones (). According to the seed hardness evaluation test, at 60 DAF this difference was obvious between hard- and soft-seeded genotypes.
Figure 2. Change in lignin content (A), total phenolic (B), antioxidant activity (C), and seed hardness (D) in various hard- and soft-seeded genotypes during different sampling date. (Data are mean of three replications ± SE.) DAF: Day after fruit set; N: force needed to rupture seed in Newton.
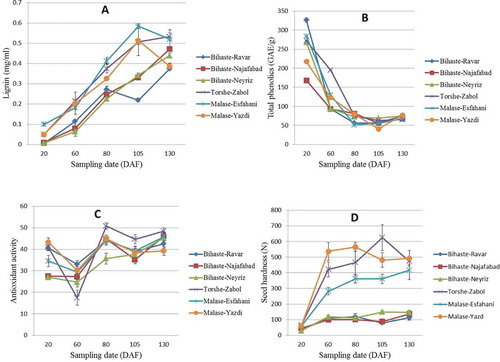
Quantification of lignin in pomegranate seeds during fruit development revealed that soft-seeded genotypes showed to have remarkable lignin derivatives, but their lignin content was a little less compared to the hard-seeded genotypes at all stages (). Lignin content showed an increasing trend during the growth season (except for ‘Malase-Esfahani’ that showed the highest value at 105 DAF). The lowest amount of lignin was recorded at 20 DAF for all genotypes.
Although lignin content was different among the various soft- and hard-seeded genotypes, these differences, especially at the last stages of sampling, were not to a certain extent that the seed hardness could be attributed to it. Therefore, it is unlikely that differences in the seed covering of the hard- and soft-seeded genotypes can be ascribed to the lignin content.
Although spectrophotometric methods have been widely used to study the lignin content of various plants, it should be noted that in this method the assay is based on the aromatic ring of phenolic compound; therefore, it is probable that some of the final absorbance may be due to the monomers, which were not omitted during the washing steps. Therefore, photometric absorbance that was recorded in the soft-seeded genotypes may be as a result of the lignin monomers that were not converted to its polymer form. However, other compounds, such as phenolic acids, can be partially incorporated into the lignin polymer too (Ralph, Citation2010). Also, it is possible that the level of polymerization in the soft-seeded genotypes is not to the degree that makes them hard, or the conformation of lignin polymerization in the soft-seeded genotypes would be different from the hard-seed ones. Also, it is more probable that the differences of soft- and hard-seeded genotypes might be due to the differences in the contribution of each lignin monomer in the final polymer. Boerjan et al. (Citation2003) reported that a proportion of monomers vary among taxa, cell types, and individual cell wall layers, and give different properties to the final lignin polymer. In fact, the ratio of S/G is an important factor, having a major impact on lignin polymer structure and dictating the degree of lignin condensation by allowing for different types of polymeric linkages and G content leads to more highly condensed lignin (Guo et al., Citation2001). Therefore, it is probable that S unit constitutes the main part of lignin polymer in the soft-seeded pomegranate genotype, which subsequently affects the mechanical strength of seed cover in this type of genotype (this is in consistence with our unpublished results from qPCR experiment).
Total phenolic content
The total phenolic content of pomegranate arils was the greatest at the beginning of fruit development, then decreased quickly during the second stage and remained low until ripening (). The highest value of phenolic (325.76 mg GAE/g) was recorded for ‘Bihaste-Ravar’ (a soft-seeded genotype) at 20 DAF and the lowest value was (40.91 mg GAE/g) recorded for ‘Malase-Yazdi’ (a hard-seeded genotype) at 105 DAF. Also, results showed that total phenolic content has no significant correlation with seed-softness trait in pomegranate.
Decreasing trends in the total phenolic content have been reported in other pomegranate cultivars such as Ganesh (Kulkarni and Aradhya, Citation2005) and 121-2 (Shwartz et al., Citation2009), but in the cultivar 101-2 there was no significant difference during fruit development (Shwartz et al., Citation2009). Also, the same trend has been reported for other fruits, such as pear (Amiot et al., Citation1995), strawberry (Wang and Lin, Citation2000), guava (Bashir et al., Citation2003) as well as flaxseed (Herchi, Citation2011). The decreasing trend of total phenolic may be due to the oxidation of phenolic content by polyphenol oxidase, which is one of the characteristics of fruit maturity (Amiot et al., Citation1995). On the one hand, reduction in the phenolic content during fruit ripening seems to be a natural process, because phenolic compound and especially hydrolysable tannins are the main components of stringency taste of the fruit and decline in these compounds during the fruit ripening making the edible parts of the fruit more palatable for the consumers and, hence, facilitating the spread of seeds and survive their species.
Antioxidant activity
Although antioxidant capacity did not show high differences during the fruit development, the overall trend was increasing during the growth season and its mean increased from 35.67% (at 20 DAF) to 44.6% (at 130 DAF) (). Antioxidant activity decreased a little at 60 DAF, then raised to a higher level at 80 DAF and remains constant till ripening. The average value of antioxidant activity was a little higher for the hard-seeded genotypes than the soft-seeded ones at 20 DAF, then the soft-seeded genotypes showed higher antioxidant capacity at the next stage. However, at three last samplings, the activity in the soft- and hard-seeded genotypes did not show significant differences. According to the results there were no significant differences in antioxidant capacity among the soft- and hard-seeded genotypes.
Jiang et al. (Citation2006) studied antioxidant activities of four apple cultivars and reported that in some cultivars the activity increased while some others showed irregular trends. Kulkarni and Aradhya (Citation2005) observed a similar trend in the pomegranate cv. Ganesh. These authors recorded the lowest antioxidant activity at 60 DAF and after some increase to the next stage, did not observe significant differences till the ripening. While studying the two pomegranate cultivars, Shwartz et al. (Citation2009) observed that, after a slight reduction in the antioxidant capacity at the beginning stage of fruit development, these cultivars showed no significant differences until maturity.
Although some of the studies regarding the antioxidant and total phenol indicate the similar trend between these two components (Drogoudi et al., Citation2008; Yuri et al., Citation2012), some others represented a completely opposite trend, namely, “object and its reflection in the mirror” (Fernandez-Orozco et al., Citation2011; Miletic et al., Citation2012), while some others reported lack of a particular trend (Tlili et al., Citation2011; Kristl et al., Citation2011). As observed in the present study, especially during the last stages of sampling, total phenolic content decreased drastically, while total antioxidant activity increase gradually; therefore, there was a little contrary trend between these two factors.
Conclusion
Although LAC activity was low and did not show remarkable differences between soft- and hard-seeded genotypes, POD activity was high and showed a distinctive pattern at ripening stages. Therefore, POD might be a more powerful candidate than LAC in the lignin polymerization phase in pomegranate seeds. Also, PAL activity decreased in all genotypes, but it’s decreases were more obvious in the hard-seeded genotypes. However, this is the first report indicating the existing of lignin polymers in the seeds of soft-seeded pomegranate genotypes. Lignin detection in the soft-seeded pomegranate genotypes revealed that the differences between soft- and hard-seeded genotypes might be due to the differences in the percentage of monolignol monomer, polymerization degree and or polymer structure which needs to be investigated further. Decrease in the PAL activity was followed by decrease in the total phenolic content during the growth season, confirming the role of PAL in the production of phenolic compounds. Also, antioxidant activity increased a little and showed an opposite trend with phenolic content during fruit development indicating that there may be some competitive effects for the same substrates.
Based on the results of the present study more investigations on molecular aspect of seed-softness with emphasis on POD and PAL enzymes as well as different monolignol specific enzymes are recommended to identify the molecular basis of this trait. Further, a more in-depth analysis of lignin constitute is needed to reveal the lignin differences between soft- and hard-seeds in pomegranate.
Literature cited
- Aebi, H. 1984. Catalase, p. 121–126. In: L. Packer (ed.). Methods in enzymology, vol. 105. Academic Press, Orlando, FL.
- Amiot, J.M., M. Tacchini, S.Y. Aubert, and W. Oleszek. 1995. Influence of cultivar, maturity stage and storage conditions on phenolic composition and enzymatic browning of pear fruit. J. Agr. Food Chem. 43:1132–1137.
- Ara, N., K. Nakhanong, W. Lv, L. Yang, Z. Hu, and M. Zhang. 2013. Antioxidant enzymatic activities and gene expression associated with heat tolerance in the stems and roots of two cucurbit species (“Cucurbita maxima” and “Cucurbita moschata”) and their interspecific inbred line “Maxchata”. Intl. J. Mol. Sci. 14:24008–24028.
- Awad, M.A., A.D. Al-Qurashia, and S.A. Mohamed. 2011. Antioxidant capacity, antioxidant compounds and antioxidant enzyme activities in five date cultivars during development and ripening. Sci. Hort. 129:688–693.
- Aydin, N. and A. Kadioglu. 2001. Changes in the chemical composition, polyphenol oxidase and peroxidase activities during development and ripening of medlar fruits (Mespilus germanica L.). Bul. J. Plant Phys. 27:85–92.
- Bashir, H.A., A. Abu-Bakr, and A. Abu-Goukh. 2003. Compositional changes during guava fruit ripening. Food Chem. 80:557–563.
- Boerjan, W., J. Ralph, and M. Baucher. 2003. Lignin biosynthesis. Annu. Rev. Plant Biol. 54:519–546.
- Bruce, R.J. and C.A. West. 1989. Elicitation of lignin biosynthesis and isoperoxidase activity by pectic fragments in suspension cultures of castor bean. Plant Phys. 91:889–897.
- Cai, X., E.J. Davis, J. Ballif, M. Liang, E. Bushman, V. Haroldsen, J. Torabinejad, and Y. Wu. 2006. Mutant identification and characterization of the laccase gene family in Arabidopsis. J. Exp. Bot. 57:2563–2569.
- Chance, B. and A.C. Maehly. 1955. Assay of catalases and peroxidases, p. 764–775. In: S.P. Colowick and N.O. Kaplan (eds.). Methods in enzymology. Academic Press, New York.
- Cheng, G.W. and P.J. Breen. 1991. Activity of phenylalanine ammonia-lyase (PAL) and concentrations of anthocyanins and phenolics in developing strawberry fruit. J. Amer. Soc. Hort. Sci. 116:865–869.
- Civello, P.M., G.A. Martınez, A.R. Chaves, and M.C. Anon. 1995. Peroxidase from strawberry fruit (Fragaria ananassa Duch.): Partial purification and determination of some properties. J. Agr. Food Chem. 43:2596–2601.
- Dalimov, D.N., G.N. Dalimova, and M. Bhatt. 2003. Chemical composition and lignins of tomato and pomegranate seeds. Chem. Nat. Compounds 39:37–40.
- Ding, C.K., K. Chachin, Y. Ueda, Y. Imahori, and C.V. Wang. 2001. Metabolism of phenolic compounds during loquat fruit development. J. Agr. Food Chem. 49:2883−2888.
- Drogoudi, P.D., Z. Michailidis, and G. Pantelidis. 2008. Peel and flesh antioxidant content and harvest quality characteristics of seven apple cultivars. Sci. Hort. 115:149–153.
- Elkind, Y., R. Edwards, M. Mavandad, S.A. Hedrick, O. Ribak, R.A. Dixon, and C. Lamb. 1990. Abnormal plant development and down-regulation of phenylpropanoid biosynthesis in transgenic tobacco containing a heterologous phenylalanine ammonia-lyase gene. Proc. Natl. Acad. Sci. U.S.A. 87:9057–9061.
- Erkurt, E.A., A. Unyayar, and H. Kumbur. 2007. Decolorization of synthetic dyes by white rot fungi, involving laccase enzyme in the process. Process Biochem. 42:1429–1435.
- Fernandez-Orozco, R., M. Roca, B. Gandul-Rojas, and L. Gallardo-Guerrero. 2011. DPPH-scavenging capacity of chloroplastic pigments and phenolic compounds of olive fruits (cv. Arbequina) during ripening. J. Food Compos. Anal. 24:858–864.
- Gavnholt, B., and K. Larsen. 2002. Molecular biology of plant laccases in relation to lignin formation. Physiol. Plant 116:273–280.
- Guo, D., F. Chen, K. Inoue, J.W. Blount, and R.A. Dixon. 2001. Down-regulation of caffeic acid 3-O-methyltransferase and caffeoyl CoA 3-O-methyltransferase in transgenic alfalfa: Impacts on lignin structure and implications for the biosynthesis of G and S lignin. Plant Cell 13:73–88.
- Harel, E., A.M. Mayer, and H.R. Lerner. 1970. Changes in the levels of catechol oxidase and laccase activity in developing peaches. J. Sci. Food Agr. 21:542–544.
- Harkin, J.M. and T.R. Obst. 1973. Lignification in trees: Indication of exclusive peroxidase participation. Science 180:296–297.
- Herchi, W., F. Sakouhi, D. Arraez-Roman, A. Segura-Carretero, S. Boukhchina, H. Kallel, and A. Fernandez-Gutierrez. 2011. Changes in the content of phenolic compounds in flaxseed oil during development. J. Amer. Oil Chem. 88:1135–1142.
- Jiang, H., B. Ji, J. Liang, F. Zhou, Z. Yang, and G. Zhang. 2006. Changes of contents and antioxidant activities of polyphenols during fruit development of four apple cultivars. Eur. Food Res. Technol. 223:743–748.
- Jones, D. 1984. Phenylalanine ammonia-lyase: Regulation of its induction, and its role in plant development. Phytochemistry 23:1349–1359.
- Kristl, J., M. Slekovec, S. Tojnko, and T. Unuk. 2011. Extractable antioxidants and non-extractable phenolics in the total antioxidant activity of selected plum cultivars (Prunus domestica L.): Evolution during on-tree ripening. Food Chem. 125:29–34.
- Kulkarni, A.P. and S.M. Aradhya. 2005. Chemical changes and antioxidant activity in pomegranate arils during fruit development. Food Chem. 93:319–324.
- Mars, M. 2000. Pomegranate plant material: Genetic resources and breeding, a review. Options Mediterranean 42:55–62.
- Mayer, A.M. and R.C. Staples. 2002. Laccase: New functions for an old enzyme. Phytochemistry 60:551–565.
- Miletić, N., B. Popović, O. Mitrović, and M. Kandić. 2012. Phenolic content and antioxidant capacity of fruits of plum cv. ‘Stanley’ (Prunus domestica L.) as influenced by maturity stage and on-tree ripening. Austral. J. Crop Sci. 6:681–687.
- Moon, J.H. and J. Terao. 1998. Antioxidant activity of caffeic acid and dihydrocaffeic acid in lard and human low-density lipoprotein. J. Agr. Food Chem. 46:5062–5065.
- Olsen, K.M., U.S. Lea, R. Slimestad, M. Verheul, and C. Lillo. 2008. Differential expression of four Arabidopsis PAL genes: PAL1 and PAL2 have functional specialization in abiotic environmental-triggered flavonoid synthesis. J. Plant Phys. 165:1491–1499.
- Passardi, F., C. Cosio, C. Penel, and C. Dunand. 2005. Peroxidases have more functions than a Swiss army knife. Plant Cell Rep. 24:255–265.
- Popescu, C., E. Postolache, G. Rapeanu, M. Bulancea, and T. Hopulele. 2009. Change of the physico-chemical indices and the oxidative enzymatic activities during the white grapes ripening. Food Technol. 13:70–76.
- Pourcel, L., J. Routaboul, and L. Kerhoas. 2005. Transparent testa10 encodes a laccase-like enzyme involved in oxidative polymerization of flavonoids in Arabidopsis seed coat. Plant Cell 17:2966–2980.
- Ralph, J. 2010. Hydroxycinnamates in lignification. Phytochem. Rev. 9:65–83.
- Ranocha, P., M. Chabannes, S. Chamayou, S. Danoun, A. Jauneau, A.M. Boudet, and D. Goffner. 2002. Laccase down-regulation causes alterations in phenolic metabolism and cell wall structure in poplar. Plant Phys. 129:145–155.
- Sarkhosh, A., Z. Zamani, R. Fatahi, and H. Ranjbar. 2009. Evaluation of genetic diversity among Iranian soft-seed pomegranate accessions by fruit characteristics and RAPD markers. Sci. Hort. 121:313–319.
- Savidge, R.A. and P.V. Udagama-Randeniya. 1992. Cell wall-bound coniferyl alcohol oxidase associated with lignification in conifers. Phytochemistry 31:2959–2966.
- Shwartz, E., I. Glazer, I. Bar-Ya’akov, I. Matityahu, I. Bar-Ilan, I. Holland, and R. Amir. 2009. Changes in chemical constituents during the maturation and ripening of two commercially important pomegranate accessions. Food Chem. 115:965–973.
- Silva, E., E.J. Lourencuo, and V.A. Neves. 1990. Soluble and bound peroxidases from papaya fruit. Phytochemistry 29:1051–1056.
- Sterjiades, R., J.F.D. Dean, and K.E.L. Eriksson. 1992. Laccase from sycamore maple (Acer pseudoplatanus) polymerizes monolignols. Plant Phys. 99:1162–1168.
- Stover, E. and E.W. Mercure. 2007. The pomegranate: A new look at the fruit of paradise. HortScience 45:1088–1092.
- Sun, J., X. Xiang, C. Yu, J. Shi, H. Peng, B. Yang, S. Yang, E. Yang, and Y. Jiang. 2009. Variations in contents of browning substrates and activities of some related enzymes during litchi fruit development. Sci. Hort. 120:555–559.
- Thompson, D.S., W.J. Davies, and L.C. Ho. 1998. Regulation of tomato fruit growth by epidermal cell wall enzymes. Plant Cell Environ. 21:589–599.
- Tlili, I., C. Hdider, M.S. Lenucci, R. Ilahy, H. Jebari, and G. Dalessandro. 2011. Bioactive compounds and antioxidant activities during fruit ripening of watermelon cultivars. J. Food Compos. Anal. 24:923–928.
- Tomas-Barberan, F.A. and J.C. Espin. 2001. Phenolic compounds and related enzyme and determinants of quality in fruits and vegetables. J. Sci. Food Agr. 81:853–876.
- Velioglu, Y.S., G. Mazza, L. Gao, and B.D. Oomah. 1998. Antioxidant activity and total phenolics in selected fruits, vegetables, and grain products. J. Agr. Food Chem. 46:4113−4117.
- Wang, S.Y. and H.S. Lin. 2000. Antioxidant activity in fruits and leaves of blackberry, raspberry, and strawberry varies with cultivar and developmental stage. J. Agr. Food Chem. 48:140–146.
- Yu, C., J. Sun, X. Xiang, B. Yang, and Y. Jiang. 2010. Variations in contents of (−)-epicatechin and activities of phenylalanine ammonia lyase and polyphenol oxidase of longan fruit during development. Sci. Hort. 125:230–232.
- Yuri, J.A., F.J. Maldonado, Y. Razmilic, A. Neira, A. Quilodran, and I. Palomo. 2012. Concentrations of total phenols and antioxidant activity in apple do not differ between conventional and organic orchard management. J. Food Agr. Environ. 10:207–216.
- Zarei, A., Z. Zamani, R. Fatahi, A. Mousavi, and S.A. Salami. 2013. A mechanical method of determining seed-hardness in pomegranate. J. Crop Improve. 27(4):444–459.