ABSTRACT
Anaerobic soil disinfestation (ASD) and mustard seed meal (MSM) appear to be promising non-fumigant alternatives for soilborne pathogens control. However, studies of their effect on charcoal rot caused by Macrophomina phaseolina in California strawberry are limited. A demonstration field trial with ASD with rice bran 20 t ha–1 (ASD-RB), MSM 4.5 t ha–1 (MSM), and ASD with rice bran 6.7 t ha–1 + MSM 4.5 t ha–1 (ASD-RB+MSM) treatments was conducted in a M. phaseolina-infested organic field in Oxnard, CA. A doubling of fruit yields relative to the grower standard, and a reduction in plant mortality by M. phaseolina, was achieved by ASD-RB. MSM failed to control M. phaseolina and resulted in fruit yields that were more than 20% below the ASD-RB yield. Fruit yield in the ASD-RB+MSM plot was intermediate between ASD-RB and MSM. Treatment effects on soil pH, EC, inorganic N dynamics, and soil microbial communities were also examined.
Introduction
Since the 1950s, pre-plant soil fumigation has been the core technology for soilborne disease and weed control in California strawberry (Wilhelm and Paulus, Citation1980). Due to the environmental and human health concerns (CDPR, Citation2009; Gemmill et al., Citation2013; UNEP, Citation1997) and resulting stringent regulations, however, future availability of fumigants is increasingly uncertain making research on non-fumigant alternatives a priority (CDPR, Citation2013; Legard, Citation2011). Anaerobic soil disinfestation (ASD) was developed in the Netherlands (Blok et al., Citation2000; Lamers et al., Citation2010) and Japan (Momma et al., Citation2013; Shinmura, Citation2000) as a biological alternative to fumigants. In California, ASD has been shown to be effective in reducing Verticillium dahliae microsclerotia in soil by 80% to 100% and to be able to provide marketable fruit yields comparable to fumigation in strawberry systems (Shennan et. al., Citation2014). The relative marketable yield of strawberries in ASD plots to fumigant controls in 10 replicated-field trials across the California coast averaged 99% when 20 t ha–1 of rice bran was used as the carbon source for ASD (Daugovish et al., Citation2013; Shennan et al., Citation2012, Citation2014; Zavatta et al., Citation2014a, Citation2014b). In response, California berry growers started to adopt ASD at a commercial scale; ASD acreage has rapidly increased from 2 ha to over 400 ha in the last 4 years (Farm Fuel Inc., personal communication). Mustard seed meal (MSM) has been successfully used for controlling the replant disease complex of apples, and providing similar or superior yield compared to fumigant controls (Mazzola and Brown, Citation2010; Mazzola et al., Citation2015). It has also been evaluated in strawberry systems and showed neutral to positive effects on fruit production and disease suppression when used alone or in combination with other non-fumigant approaches, such as ASD (Shennan et al., Citation2013) and steam (Fennimore et al., Citation2014).
Charcoal rot caused by Macrophomina phaseolina is an emergent lethal disease of California strawberries (Koike, Citation2008). ASD and a similar method called biosolarization was effective in controlling this disease in strawberries in warmer regions, such as Florida (Rosskopf et al., Citation2014) and Spain (Chamorro et al., Citation2015). However, studies on the effect of ASD on this disease in California strawberries has been limited (Daugovish et al., Citation2013). Here, we report results from a large-scale non-fumigant demonstration trial at a M. phaseolina-infected organic field in Oxnard, CA. The goals of the project were: (1) to conduct a large-scale on-farm demonstration for two non-fumigant alternatives: ASD and MSM, (2) to test efficacy of ASD and MSM on charcoal rot infection of strawberry, and (3) to examine the effects of ASD and MSM on selected soil chemical and biological characteristics.
Materials and methods
Demonstration trial
A non-fumigant demonstration trial was established in an organic field with Pico sandy loam soil (coarse-loamy, mixed, thermic Fluventic Haploxerolls) in Oxnard, CA in Aug. 2013. The field had been managed organically for 8 years before establishment of the trial; the last strawberry was grown 3 years ago and had some die-out, followed by vegetables, such as celery, chards, and spinach. Immediately prior to the trial setup, Sudan/sorghum hybrid was sawn on 16 June at 112 kg ha–1 seeding rate and mowed and incorporated at the end of July 2013. The demonstration trial had four treatments in adjacent plots; MSM (Brassica juncea: Sinapis alba = 1:1 wt/wt) 4.5 t ha–1, ASD with rice bran 6.7 t ha–1 + MSM 4.5 t ha–1 (ASD-MSM+RB), ASD rice bran 20 t ha–1 (ASD-RB), and the grower standard (GS). Each plot was 0.4 ha without replication but each plot was divided into four equal-sized sections, which were used as pseudo-replicates.
MSM and RB were broadcast to assigned plots and incorporated into 0–15-cm soil depth by a spring-tooth harrow passing two to three times from different directions on 31 Aug. 2013. On the same day, beds were listed, pre-plant fertilizer (1122 kg ha–1 of True organic 12-12-2) was band applied into beds of all plots, and drip tapes and tarp (black TIF) were applied. The first drip irrigation of 34 mm was applied to ASD-MSM+RB, ASD-RB, and GS plots on 3 Sept. Then 14–21 mm of drip irrigation and 1.5 mm of sprinkler irrigation to seal the soil surface of the furrow were added approximately every 3 days across the three plots until 30 Sept. (total 220 mm of drip and 25 mm of sprinkler irrigation) whereas MSM plots only received 21 mm of drip irrigation on 30 Sept. During ASD treatment, soil Eh, an indicator of the level of anaerobisis, at 15-cm depth in the ASD-MSM+RB and ASD-RB plots was monitored daily with an oxidation reduction potential (ORP) sensor (S500CD-ORP, Sensorex, Garden Grove, CA, USA) installed in each section of the plots. A hand-held ORP meter was used to record ORP mV values. Eh mV values were obtained by adding 199 mV to ORP mV (Patrick et al., Citation1996). To express intensity of anaerobic condition during ASD treatment, cumulative Eh mV hours below 200 mV (cum. Eh) was also calculated. Soil temperature at 15-cm depth was continuously monitored for the ASD plots using two soil temperature/moisture/EC sensors (5-TE, Decagon Devices Inc., Pullman, WA, USA) per plot connected to a data logger (Em50, Decagon Devices Inc.). Planting holes were cut in the tarp during the first week of October and strawberry plants (Fragaria ananassa) cv. PSI 1975, a cultivar known to be susceptible to charcoal rot and Fusarium wilt, was planted at the density of 71,784 plants per ha on 14 Oct. 2013. In-season liquid fertilizer was applied to all plots 13 times from Oct. 2013 to May 2014 for a total rate of 108 kg-N ha–1, 14 kg-P2O5 ha–1, and 14 kg-K2O ha–1.
Soil analysis
From each section of each treatment plot, soil samples (composite of 20 sub-samples at 0–15-cm depth) were taken pre-treatment (30 Aug. 2013), post-treatment (3 Oct. 2013), and then monthly throughout the growth period. Fresh soil samples were kept on ice in an ice chest in the field, brought or overnight shipped to the Agroecology lab at UCSC, and extracted for inorganic N using 2M KCl within 24 h from the sampling. Filtrate of the extracts were kept frozen until analysis and the remainder of the soils were stored in a 4 °C refrigerator until further analysis. Ammonium-N and nitrate + nitrite-N in 2M KCl filtrates were determined by Lachat flow injection analyzer (Lachat Instruments, Citation1993a, Citation1993b). Soil moisture content was determined gravitationally, and fresh soil pH (1:1 w/w) and EC (1:1 w/w) were determined using a bench-top pH meter (pH 700 benchtop meter, Oakton, Vermon Hills, IL, USA) and conductivity meter (pH/CON 700 benchtop meter, Oakton), respectively. Post-treatment soil samples were also overnight shipped on the day of sampling to the USDA-ARS Tree Fruit Research Laboratory, Wenatchee, WA. DNA was extracted from 5 g of each soil sample using the PowerMax soil DNA isolation kit (MO BIO Laboratories Inc., Carlsbad, CA, USA). Similarity in soil bacterial and fungal community composition among soil treatments was assessed using terminal restriction fragment length polymorphism (T-RFLP) analysis. The analyses were conducted essentially as described by Weerakoon et al. (Citation2012). Effect of soil treatments on densities of total bacteria and fungi, Pythium spp., Fusarium spp., and fluorescent Pseudomonas spp., were determined using standard soil dilution methods and cultivation on semi-selective media (Mazzola et al., Citation2001).
Yield and mortality monitoring and disease diagnosis
Marketable fruit yield was monitored as number of flats harvested for fresh market from each plot by the grower and expressed as relative % of the grower standard plot. Plant mortality was surveyed in each plot on 31 Mar. and 15 May 2014 by counting the number of dead plants in the entire area of each plot. At the end of the harvest season in late May, two healthy plants and two symptomatic plants were excavated to confirm the presence of pathogens from each section of each plot (n = 8 for each of healthy and sick plants per treatment). DNA was extracted from 50-mg crown or root tissue using the PowerPlant Pro DNA Isolation Kit (MO BIO Laboratories, Carlsbad, CA, USA), and quantity of M. phaseolina and Verticillium dahliae DNA in tissue extracts was determined by real-time PCR. Four segments of crown tissue from each plant were plated onto 1/10th-strength potato dextrose agar amended with rifampicin (10 μg ml–1) and streptomycin (75 μg ml–1). Mycelium emerging from crown tissue was examined microscopically to determine identity.
Since this is a non-replicated trial, soil and plant analysis data are shown as mean and standard error of mean (SEM) of pseudo-replicates for each treatment without analysis of variance (ANOVA) and mean separations.
Results
Strawberry growth, disease, and yield
The incorporation of rice bran using a spring-tooth harrow resulted in somewhat uneven distribution of rice bran across the bed profiles ( and ). During ASD treatment, strong anaerobic conditions in both ASD-RB (161,000 cum. Eh mV h) and ASD-MSM+RB (92,700 cum. Eh mV h) plots were developed and soil temperature at 15-cm depth averaged 28 °C. Cumulative hours that soil temperature exceeded 30 °C during the ASD treatment were 253 h (ASD-RB) to 262 h (ASD-RB+MSM). In Nov. 2013, strawberry plants showed some phytotoxicity in the MSM plot. Also, some salt damage was observed in the MSM and ASD-MSM+RB plots in Dec. 2013 to Feb. 2014. Plant size was largest in ASD-RB, followed by ASD-MSM+RB and MSM, and smallest in GS throughout the growing season ().
Figure 1. Rice bran distribution across the bed profiles. Relatively well-mixed profile (A) and unevenly mixed profile where rice bran is concentrated in the middle of the bed and no bran exists towards both shoulders (B).
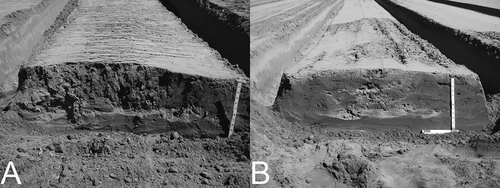
Figure 2. Strawberry plants of each treatment at the Oxnard demonstration site at the end of the season. May 29, 2014. A; mustard seed meal (MSM) 4.5 ton ha-1, B; anaerobic soil disinfestation (ASD) using rice bran 6.7 ton ha-1 + MSM 4.5 ton ha-1, C; ASD using rice bran 20 ton ha-1, and D; the grower standard.
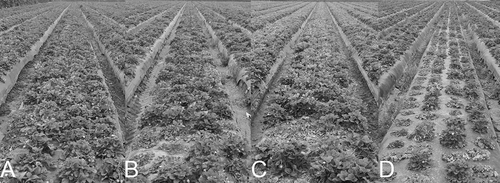
Plants began to show severe wilt in mid-March. The main causal pathogen was identified as M. phaseolina though F. oxysporum was also found to a lesser degree. At the end of the season in late May, mortality was 16% in ASD-RB and 26% to 29% in all other plots (). Crowns of diseased plants were primarily infested by M. phaseolina while Cylindrocarpon spp. and Fusarium spp. were the primary fungi isolated from crowns of healthy plants. When healthy crowns were examined for the presence of M. phaseolina using qPCR, the fungus was not detected in any plants from the ASD or ASD-RB+MSM treatment and was detected in all crowns from GS treatment but only one crown from the MSM alone treatment. When roots were assessed for the presence of this pathogen, however, MSM and ASD-RB possessed lower quantity of M. phaseolina DNA than the GS treatment as determined by qPCR (). This finding is important as the quantity of the pathogen in root tissue may be predictive of spread to crown tissue, and subsequent wilt development, later in the growing season.
Figure 3. Mortality of strawberry plants (A) and cumulative marketable fruit yield (relative % to the grower standard yield on May 23, 2014) (B) at the Oxnard demonstration site. MSM; mustard seed meal 4.5 ton ha-1, ASD-RB+MSM; anaerobic soil disinfestation (ASD) using rice bran 6.7 ton ha-1 + MSM 4.5 ton ha-1, ASD-RB; ASD using rice bran 20 ton ha-1, and GS; the grower standard.
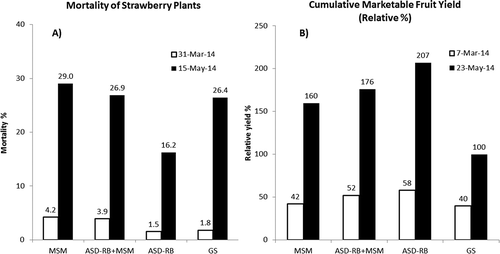
Figure 4. Effect of soil treatments on abundance of Macrophomina phaseolina detected in strawberry root DNA extracts as determined by real-time PCR. Bars represent one standard error of the mean. Treatments: ASD = ASD-RB, ASD+MSM = ASD-RB+MSM. See ’s caption for more information on legends.
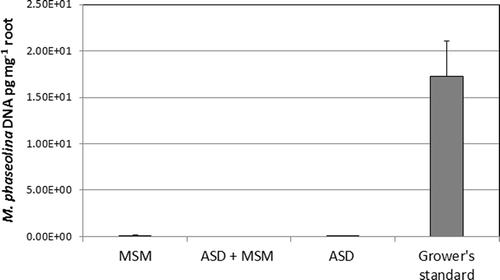
Absolute yield data are not available (proprietary information). Relative cumulative marketable fruit yields were highest for ASD-RB (207%), followed by ASD-MSM+RB (176%), MSM (160%), and the lowest for the GS (100%) ().
Soil characteristics
Soil pH (1:1) was reduced by all treatments but more clearly by ASD treatments (). Especially, ASD-RB treatment lowered soil pH about 1 unit more than the GS in Dec. 2013 and maintained the low pH until the end of the season. Soil EC (1:1) increased to 1.4 mS cm–1 in MSM plots post-treatment and remained above the salt damage threshold of 0.9 mS cm–1 for salt sensitive crop including strawberry (USDA Soil Quality Institute, Citation1998) until Feb. 2014 (). In the ASD-RB plots in comparison, EC was relatively low (0.8–0.9 mS cm–1) until Jan. 2014, increased to 1.3 mS cm–1 in February, and then dropped to 0.6 mS cm–1. GS had low EC of 0.5 to 0.6 mS cm–1 throughout the growth period. Immediately post-treatment, ammonium was the major form of soil inorganic N in ASD-RB and ASD-MSM+RB due to the anaerobic conditions during the treatment, though it was mostly nitrified by December ().
Figure 5. Changes in soil pH (1:1) (A) and soil EC (1:1) (B) (0-15 cm depth) at the Oxnard demonstration site. See ’s caption for legends.
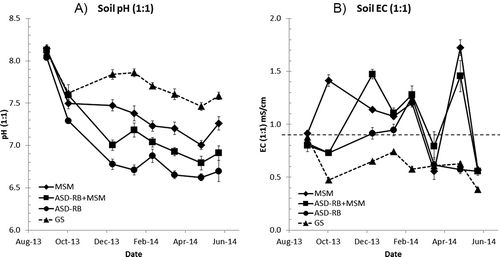
Figure 6. Changes in soil inorganic N (0-15 cm depth) at the Oxnard demonstration site. See ’s caption for legends.
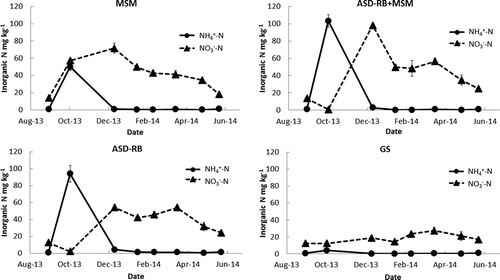
Based upon the characterization of similarity through analysis of T-RFLP data, all soil treatments dramatically and consistently altered bacterial () and fungal composition (). Such alterations in community composition were previously shown to be associated with efficacy of ASD-RB in improving strawberry yields at multiple California field locations (Shennan et al., Citation2013). ASD alone increased total bacterial, total fungal, and fluorescent Pseudomonas spp. populations. ASD treatment with or without MSM increased Pythium spp. populations, while MSM decreased Pythium soil densities. ASD alone was the only treatment that significantly reduced Fusarium spp. populations (data not shown).
Figure 7. Soil bacterial (A) and fungal (B) community analysis by T-RFLP analysis at post-treatment (October 3, 2013) at the Oxnard demonstration site. 0 to 15 cm depth. ASD = ASD-RB, ASD MSM = ASD-RB+MSM. See ’s caption for more information on legends. Number after treatment indicates section number of each treatment.
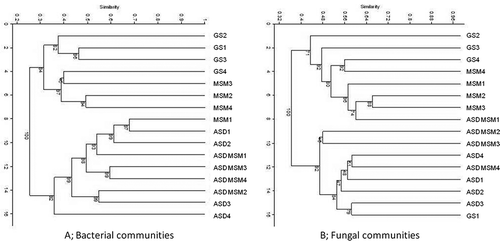
Discussion
A doubling of yields relative to the grower standard, as well as a reduction in the incidence of disease caused primarily by M. phaseolina, was achieved by using ASD-RB at this organic site. Complete disease control was not achieved, however, and this may be related to areas where the RB was not evenly distributed within the bed (). Studies show that the environmental threshold under ASD varies depending on the target pathogen. For example, Shennan et al. (Citation2009) found that, in the incubation experiments, over 90% of Verticillium dahliae microsclerotia became below the detection limit at 25 °C when cumulative Eh below 200 mV reached 50,000 mV hours. For Fusarium oxysporum f. sp. fragariae, however, Yonemoto et al. (Citation2006) found that approximately 280–300 h above 30 °C at 20-cm soil depth were necessary to consistently reduce the pathogen population below the detection limit. Although no threshold for controlling M. phaseolina by ASD has been established, a higher temperature than the one for V. dahliae may be necessary (Chamorro et al., Citation2015; Rosskopf et al., Citation2014).
Anaerobic soil disinfestation lowered the soil pH to more optimal levels for strawberry growth relative to other treatments due likely to organic acid production during the anaerobic decomposition process of the added carbon source (Momma et al., Citation2006) as well as nitrate formation during the post-treatment period (). The pH reduction by ASD worked positively for this high pH field, but for acidic fields it can be of concern and adjustment by liming may be necessary. The EC changes may be mostly explained by soil nitrate dynamics [EC (1:1) = 0.00942 × NO3-N + 0.544; R2 = 0.337 (n = 143, P < 0.0001)] suggesting that the high soil nitrate may be responsible for the salinity damage observed in the MSM and ASD-MSM+RB plots from Dec. 2013 to Jan. 2014. Salt index value, an indicator of osmotic pressure increase by fertilizer, of potassium nitrate is 1.580, which is greater than 0.853 of potassium sulfate and lower than 1.936 of potassium chloride when values are expressed as per pound of K2O (Rader et al., Citation1943).
In contrast, MSM applied at 4.5 t ha–1 failed to control M. phaseolina and resulted in yields that were more than 20% below the ASD-RB yields, although still substantially higher than GS. A 5-week period after 3% MSM application was needed to avoid reduction in seed emergence of lettuce (Rice et al., Citation2007). For strawberries, a 3-week plant back time is empirically suggested to avoid MSM phytotoxicity (Farm Fuel Inc., personal communication). Further, an addition of sufficient water is important to stimulate isothiocyanate releases from MSM (Rice et al., Citation2007). In our trial, the first drip irrigation at the MSM plot was delayed until 2 weeks before transplanting, which is likely to be the reason of observed phytotoxicity. Thus, the yield reduction in the MSM treatment might be attributed to a combination of the phytotoxicity and successive salinity injury both in the early growth stage of strawberries. As previously observed for other locations, application of ASD with RB or use of MSM led to distinctly different soil microbial community profiles, which may be important for short- to long-term pathogen suppression (Mazzola et al., Citation2015).
In the upcoming season the grower has decided to increase the acreage under ASD from 5 acres to 80 acres based on these results. The grower also has agreed to repeat the demonstration with some modifications for the upcoming season, with ASD-RB, MSM, and grower standard treatments repeated in the same locations, and the ASD-RB+MSM treatment replaced with ASD with rice bran 13.4 ton ha–1. A longer plant back period may be justified based upon findings in other plant production systems (Handiseni et al., Citation2013; Mazzola et al., Citation2015) and will be employed following MSM application. In this way we will be able to document the effects of multiple years of treatment on disease incidence and crop production.
A higher amount of water was used for ASD in the demonstration than typically recommended, and this is of concern given the current restrictions on water availability due to prolonged drought. In retrospect, we probably could have saved water by applying it for a shorter time (1–2 h instead of 3 h) at each drip irrigation (approximately every 3 d). This modification will be made this coming season and Eh closely monitored to ensure adequate anaerobic conditions are still created.
In summary, there is evidence that ASD with rice bran 20 ton ha–1 as a carbon source shows promise for reducing M. phaseolina, but were not able to provide complete control. The trial is being repeated with refinements at the same location to see if greater pathogen control can be achieved.
Acknowledgments
We thank our collaborators and cooperators, including Will Doyle of WD Farms; Mark Edsall and Daniel Legard of the California Strawberry Commission; and staff, students, and volunteers of the Shennan lab, UC Santa Cruz.
Funding
This project was funded by the California Strawberry Commission ST13-25 and ST14-10 and USDA NIFA Methyl Bromide Transition Program 2012-51102-20294.
Additional information
Funding
Literature cited
- Blok, W.J., J.G. Lamers, A.J. Termorshuizen, and G.J. Bollen. 2000. Control of soilborne plant pathogens by incorporating fresh organic amendments followed by tarping. Phytopathology 90:253–259.
- California Department of Pesticide Regulation (CDPR). 2009. Reducing smog-producing emission from field fumigants: September 2009 update. http://www.cdpr.ca.gov/docs/dept/factshts/voc_rules_11_08.pdf. Accessed 1 July 2016.
- California Department of Pesticide Regulation (CDPR). 2013. Nonfumigant Strawberry Production Working Group Action Plan. http://www.cdpr.ca.gov/docs/pestmgt/strawberry/work_group/action_plan.pdf. Accessed 1 July 2016.
- Chamorro, M., L. Miranda, P. Dominguez, J.J. Medina, C. Soria, F. Romero, J.M. Lopez-Aranda, and B. de los Santos. 2015. Evaluation of biosolarization for the control of charcoal rot disease (Macrophomina phaseolina) in strawberry. Crop Protection 67:279–286.
- Daugovish, O., S. Fennimore, T.R. Gordon, S.T. Koike, J. Muramoto, C. Shennan, and K.V. Subbarao. 2013. Non-fumigant treatments for management of Fusarium oxysporum and Macrophomina phaseolina on strawberry cultivar San Andreas. 2013 Annu. Intl. Res. Conf. Methyl Bromide Altern. Emissions Reduct., San Diego, CA, 4–6 Nov. 2013.
- Fennimore, S.A., F.N. Martin, T.C. Miller, J.C. Broome, N. Dorn, and I. Greene. 2014. Evaluation of a mobile steam applicator for soil disinfestation in California strawberry. HortScience 49:1542–1549.
- Gemmill, A., R. Gunier, A. Bradman, B. Eskenazi, and K. Harley. 2013. Residential proximity to methyl bromide use and birth outcomes in an agricultural population in California. Environ. Health Perspect. 121:737–743.
- Handiseni, M., J. Brown, R. Zemetra, and M. Mazzola. 2013. Effect of Brassicaceae seed meals with different glucosinolate profiles on Rhizoctonia root rot in wheat. Crop Protection 48:1–5.
- Koike, S.T. 2008. Crown rot of strawberry caused by Macrophomina phaseolina in California. Plant Dis. 92:1253–1253.
- Lachat Instruments. 1993a. Ammonia (Salicylate) in 2M KCl soil extracts: QuikChem Method 12-107-06-2-A. Lachat Instruments, Milwaukee, WI.
- Lachat Instruments. 1993b. Nitrate in 2M KCl soil extracts: QuikChem Method 12-107-04-1-B. Lachat Instruments, Milwaukee, WI.
- Lamers, J.G., W.T. Runia, L.P.G. Molendijk, and P.O. Bleeker. 2010. Perspectives of anaerobic soil disinfestation. Acta Hort. 883:277–283.
- Legard, D.E. 2011. Developing non-fumigant based strawberry production system for California. 2011 Annu. Intl. Res. Conf. Methyl Bromide Altern. Emissions Reduct., San Diego, CA, 31 Oct.– 2 Nov. 2011.
- Mazzola, M., and J. Brown. 2010. Efficacy of brassicaceous seed meal formulations for the control of apple replant disease in organic and conventional orchard production systems. Plant Dis. 94:835–842.
- Mazzola, M., D.M. Granatstein, D.C. Elfving, and K. Mullinix. 2001. Suppression of specific apple root by Brassica napus seed meal amendment regardless of glucosinolate content. Phytopathology 91:673–679.
- Mazzola, M., S.S. Hewavitharana, and S.L. Strauss. 2015. Brassica seed meal amendments transform the rhizosphere microbiome and improve apple production through resistance to pathogen reinfestation. Phytopathology 105:460–469.
- Momma, N., Y. Kobara, S. Uematsu, N. Kita, and A. Shinmura. 2013. Development of biological soil disinfestations in Japan. Appl. Microbiol. Biotechnol. 97:3801–3809.
- Momma, N., K. Yamamoto, P. Simandi, and M. Shishido. 2006. Role of organic acids in the mechanisms of biological soil disinfestation (BSD). J. Gen. Plant Pathol. 72:247–252.
- Patrick, W.H., R.P. Gambrell, and S.P. Faulkner. 1996. Redox measurements of soils, p. 1255–1273. In: D.L. Sparks, A.L. Page, P.A. Helmke, and R.H. Loeppert (eds.). Methods of soil analysis. Part 3—Chemical methods. Soil Science Society of America, Inc., Madison, WI.
- Rader, L.F., L.M. White, and C.W. Whittaker. 1943. The salt index—A measure of the effect of fertilizers on the concentration of the soil solution. Soil Sci. 55:201–218.
- Rice, A.R., J.L. Johnson-Maynard, D.C. Thill, and M.J. Morra. 2007. Vegetable crop emergence and weed control following amendment with different Brassicaceae seed meals. Renew. Agr. Food Syst. 22:204–212.
- Rosskopf, E.N., N. Kokalis-Burelle, J. Hong, D.M. Butler, J.W. Noling, Z. He, B. Booker, and F. Sances. 2014. Comparison of anaerobic soil disinfestation and drip-applied organic acids for raised-bed specialty crop production in Florida. Acta Hort. 1044:221–228.
- Shennan, C., J. Muramoto, G. Baird, S.T. Koike, M.P. Bolda, and M. Mazzola. 2013. Optimizing anaerobic soil disinfestation for soilborne disease control. 2013 Annu. Intl. Res. Conf. Methyl Bromide Altern. Emission Reduct., San Diego, CA, 4–6 Nov. 2013.
- Shennan, C., J. Muramoto, M. Bolda, S.T. Koike, and O. Daugovish. 2009. Optimizing anaerobic soil disinfestation for non-fumigated strawberry production in California. 2009 Annu. Intl. Res. Conf. Methyl Bromide Altern. Emissions Reduct., San Diego, CA, 29 Oct.–1 Nov. 2009.
- Shennan, C., J. Muramoto, S.T. Koike, and O. Daugovish. 2012. Optimizing anaerobic soil disinfestation for non-fumigated strawberry production in California. California Strawberry Commission Annual Production Research Report 2010–2011. California Strawberry Commission.
- Shennan, C., J. Muramoto, J. Lamers, M. Mazzola, E.N. Rosskopf, N. Kokalis-Burelle, N. Momma, D.M. Butler, and Y. Kobara. 2014. Anaerobic soil disinfestation for soil borne disease control in strawberry and vegetable systems: Current knowledge and future directions. Acta Hort. 1044:165–175.
- Shinmura, A. 2000. Causal agent and control of root rot of welsh onion. PSJ Soilborne Dis. Workshop Rep. 20: 133–143 (in Japanese with English summary).
- United Nations Environment Programme (UNEP). 1997. Report of the ninth meeting of the parties to the Montreal Protocol on substances that deplete the ozone layer. http://ozone.unep.org/Meeting_Documents/mop/09mop/9mop-12.e.pdf. Accessed 1 July 2016.
- USDA Soil Quality Institute. 1998. Soil quality test kit guide. http://www.nrcs.usda.gov/wps/portal/nrcs/detail/soils/health/assessment/?cid=nrcs142p2_053873. Accessed 1 July 2016.
- Weerakoon, D.M.N., C.L. Reardon, T.C. Paulitz, A.D. Izzo, and M. Mazzola. 2012. Long-term suppression of Pythium abappressorium induced by Brassica juncea seed meal amendment is biologically mediated. Soil Biol. Biochem. 51:44–52.
- Wilhelm, S., and A.O. Paulus. 1980. How soil fumigation benefits the California strawberry industry. Plant Dis. 64:264–270.
- Yonemoto, K., K. Hirota, S. Mizuguchi, and K. Sakaguchi. 2006. Utilization of the sterilization by soil reduction in an open air field and its efficacy against Fusarium wilt of strawberry. Proc. Assoc. Pl. Protection Shikoku 41:15–24(in Japanese with English summary).
- Zavatta, M., C. Shennan, J. Muramoto, G. Baird, S.T. Koike, M.P. Bolda, and K. Klonsky. 2014a. Integrated rotation systems for soilborne disease, weed and fertility management in strawberry/vegetable production. Acta Hort. 1044:269–274.
- Zavatta, M., C. Shennan, J. Muramoto, and M. Mazzola. 2014b. Evaluating C-sources for anaerobic soil disinfestation. 2014 Annu. Intl. Res. Conf. Methyl Bromide Altern. Emissions Reduct. 12-1–2-4, Maitland, FL, 4–6 Nov. 2014.