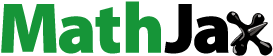
ABSTRACT
In Iran, the most pistachio orchards are located in semi-arid regions and irrigated with saline water which influences their growth and yield. The present investigation was conducted to evaluate the effects of salinity on growth, and morpho-physiological parameters of six pistachio genotypes as rootstock (ʽAkbariʼ, ʽAhmad-Aghaiʼ, ʽUCB-1ʼ, ʽItalyayiʼ, ʽGhazviniʼ and ʽBadamiʼ). Treatments were different salinity concentrations using NaCl at 0.5, 12 and 18 dSm-1 on six genotypes of pistachio. According to the results, by increasing salinity, the FW, DW, number of growing leaves, SPAD index of leaves, chlorophyll content and stomatal conductance were reduced, but electrolyte leakage of leaf and root tissues, soluble sugars and antioxidant capacity were increased. ʽUCB-1ʼ, ʽAkbariʼ and ʽAhmad-Aghaiʼ had the highest leaf area compared to other genotypes under salinity conditions. The lowest leaf abscission was belonged to ʽUCB-1ʼ and its difference was significant with other genotypes. The WUE of ʽUCB-1ʼ reduced and it was higher than ʽAkbariʼ. The growth of ʽUCB-1ʼ significantly declined up to 79.6% under salinity stress. Salinity stress had the lowest adverse effect on the growth of ʽAkbariʼ and ʽAhmad-Aghaiʼ genotypes. There was no significant difference among genotypes in regard to RWC under salinity stress. It can be concluded that ʽUCB-1ʼ, ʽAkbariʼ and to some extent ʽAhmad-Aghaiʼ were tolerant to salinity stress and can be recommended to use as rootstock under moderate salinity conditions.
Introduction
In the large agricultural area of Iran, pistachio is the most grown fruit tree and adapted to semi- and -arid regions, while still producing adequate yield. Most of Iranian’s pistachio orchards are located in the dry and warm regions (Panahi et al., Citation2002), which affects the productivity of the trees. In recent years, prolonged drought, low rainfall, high temperatures and inadequate irrigation have increased the soil salinity. In addition, they can induce serious damage to pistachio orchards that are irrigated with low-quality water (Karimi et al., Citation2012). It has been revealed that the growth rate of pistachio trees decreased by increasing sodium chloride concentration in soil (Karimi and Kuhbanani, Citation2015). Osmotic stress and toxicity are the most adverse effects of salinity stress (Munns and Tester, Citation2008). The reduction of leaf area, leaf abscission, increase in leaf thickness, necrosis in shoots and roots and reduction of internode length are some common responses to salinity stress (Parida and Das, Citation2005). The plants growing under salinity conditions can uptake the Na+ and Cl− ions leads to an increase of osmotic pressure in the cytosol. Cell hemostasis is maintained by synthesis of organic osmoprotectants and these compounds accumulate to high concentrations without interfering in plant cells metabolism (Munns, Citation2002). It has been reported that compatible osmoprotectants such as proline, glycinebetain and soluble sugars are produced to protect the cells against the harmful effect of salinity stress (Ghanaatiyan and Sadeghi, Citation2017; Sairam and Tyagi, Citation2004). At the present time, soil salinity is a major problem in pistachio production, which consequently affects the export of pistachio. Therefore, selecting salt-tolerant rootstocks play an important role in pistachio germplasm in the world (Aliakbarkhani et al., Citation2015; Talebi et al., Citation2016). Akbari et al. (Citation2018) studied the mechanism of salinity tolerance in five pistachio rootstocks (ʽUCB-1ʼ, ʽBadamiʼ, ʽGhzviniʼ, ʽKale-Ghouchiʼ, ʽAkbariʼ) and reported that ʽUCB-1ʼ was the most tolerant rootstock among them. In another study, Rahneshan et al. (Citation2018) evaluated the effects of salinity on two pistachio cultivars (Badami-Riz and Badami-e-Sefid) and reported that Badami-Riz exhibits more tolerance to salinity stress than Badami-e-Sefid. Despite of many reports on evaluating the tolerance of pistachio rootstocks to salinity stress, there is not enough information on morphological and physiological responses of pistachio to salinity stress for selecting and introducing more tolerant rootstock for orchards with soil and/or water source salinity. Therefore, the purpose of the present study was to compare morpho-physiological responses of six pistachio rootstocks to salinity stress conditions for selecting the best one.
Materials and Methods
Plant Materials and Salinity Treatments
This study was conducted as a pot experiment in a plastic greenhouse (with the average temperature 27–30°C and 70–75% relative humidity) of the Department of Horticultural Science, School of Agriculture, Shiraz University, Shiraz, Iran. The seeds of ʽUCB-1ʼ pistachio rootstock (P. atlantica × P. integerrima) and five P. vera L. genotypes including, ʽAkbariʼ, Ahmad Aghaee, Italiyayi, ʽGhazviniʼand ʽBadamiʼ were obtained from Pistachio Research Center, Rafsanjan, Kerman, Iran. Seeds were disinfected with Captan solution (1:1000), then incubated at 25°C in a growth chamber to germinate under dark condition. Germinated seeds were sown in black round pots (top diameter, 31 cm; height 24 cm) filled with sandy loam soil. Pots were transferred to the greenhouse with the following conditions, 32/26°C: day/night, 14/10 (h): light/dark, and 70% RH. Fifteen days after sowing, plants were thinned out to one per pot. The 8-month old seedlings of different genotypes and ʽUCB-1ʼ were subjected to salinity stress by three levels of saline water including 0.5 (control), 12 and 18 dS m−1 for 75 days. The concentration of NaCl increased gradually within 2 weeks to avoid the salinity shock. Irrigation treatments were applied at 3-day intervals. For every time irrigation, the soil moisture content was increased to field capacity level. After this period, plants were harvested, and separated to different organs (root, shoot and leaves).
Growth Parameters
At the beginning and the end of the experiment period, the number of leaves, stem height (cm), and stem diameter (mm) were measured. Stem diameter was measured at fourth node using a Vernier caliper. Stem height was measured from the crown up to the highest point of the plants. At the completion of the salinity treatments period, roots and stems were rinsed with distilled water to wash the soil from. To measure fresh weight (FW) of roots, leaves and stems, these organs were placed in separate envelopes and dried in an oven at 70°C for 72 h and then the dry weight (DW) was measured using a digital balance with 0.01 g accuracy.
Stomatal Conductance
Stomatal conductance was measured using a leaf porometer (SC-1; Decagan Devices, Inc.) at end of the experiment between 10 and 12 am (Jafari et al., Citation2018).
Leaf Area
Leaf area was determined using a leaf area meter (CRLA1 Devices, Iran).
Relative Water Content (RWC)
Leaf RWC was measured in 10 uniform discs of fully developed leaves based on fresh weight (FW), turgid weight (TW – after re-hydration for 24 h at 4°C in darkness), and dry weight (DW – after oven-drying in 75°C for 72 h) and calculated with following formula (Mirfattahi et al., Citation2017):
Water Use Efficiency (WUE)
To measure the WUE (mg ml−1), the total water consumption for each pot during the plant growth period measured and also the total dry weight of plant (biomass) determined and calculated with the following equation (Karimi et al., Citation2016):
WUE (mg ml−1) = dry weight of final biomass−dry weight of initial biomass/total water consumed
Electrolytic Conductivity (EC)
Ionic leakage of leaves was measured as described by Bartolozzi and Fontanszza (Citation1999). Ten leaf discs (10 mm diameter) were washed with distilled water and placed in a test tube with 5 ml distilled water, then shaked for 24 h. Electrolytic conductivity (EC1) was measured using a digital conductivity meter (Model HI8633, USA). Samples were then autoclaved at 121°C for 20 min. Then, solutions were cooled, and conductance was measured again (EC2). Ionic leakage (EC %) was expressed as the percentage of final reading (EC% = EC1/EC2 × 100).
Total Chlorophyll and Greening Index
Total chlorophyll content of leaves were determined based on the method described by Arnon (Citation1949) and DMSO was used as the extraction solvent for pigment. The absorbance of the extractions was recorded at 663 and 645 nm by a spectrophotometer (Epoch, Bio Tek©, United States). SPAD index was determined using a Chlorophyll Meter SPAD-503 (Japan) in SPAD units.
Total Soluble Carbohydrate and Starch Concentrations
To determine soluble sugar concentration, 0.1 g of samples was extracted twice with ethanol (80%). The samples were centrifuged at 5000 rpm for 20 min and the supernatant was adjusted to 10 ml. Soluble sugar content was measured according to the method described by Fox and Robyt (Citation1991). Starch concentration of samples was measured using the anthrone reagent (McCready et al., Citation1950).
Statistical Analysis
This study was conducted in a completely randomized design (CRD) with factorial arrangement and four replications. Data were analyzed using one-way analysis of variance (ANOVA). Means were compared with Duncan’s multiple range test (DMRT) at p ≤ 0.05. The SAS software version 9. 1 (SAS Institute Inc., Cary, NC) was used for all the analyses.
Results
Effect of Salinity on Growth Parameters
Growth parameters significantly decreased in six pistachio genotypes under different NaCl concentrations (0.5, 12 and 18 dSm−1) after 90 days (). In this case, average fresh weight (FW) and dry weight (DW) of leaf, stem and root were significantly reduced by increasing the salinity levels from 12 to 18 dSm−1 (). The maximum reduction in FW of leaves was belonged to 12 and 18 dSm−1 salinity compared to control that were 41.30% and 62.24%, respectively, while the minimum reduction in FW of root was belonged to in comparison with control that were 21.75% and 31.62%, respectively. The highest reduction in FW and DW of leaves, stems and roots were observed in ʽBadamiʼ, ʽGhazviniʼ and ʽItalyayiʼ genotypes under salinity stress. The lowest FW and DW of leaves were observed in ʽBadamiʼ genotype at a salinity of 18 dSm−1, the reduction was 74.63% compared to 75.47% in control. Furthermore, this genotype had the highest reduction in FW and DW of root at 18 dSm−1 treatment by 47.31% compared to 61.39% in control, respectively. ʽGhazviniʼhad the lowest stem weight with 71.12% and 79.31% reduction in FW and DW of stem at 18 dSm−1 salinity level. ʽAkbariʼ, ʽAhmad-Aghaiʼ, and ʽUCB-1ʼ had no significant difference in FW and DW of leaves under salinity conditions, while, ʽUCB-1ʼ significantly showed higher FW and DW than ʽAkbariʼ and ʽAhmad-Aghaiʼ. After the end of experiment, ʽUCB-1ʼ was very vigorous under normal condition (without salinity treatments), but its growth rate reduced in comparison with ʽAkbariʼ and ʽAhmad-Aghaiʼunder salinity conditions (). Furthermore, stem FW decreased at a salinity of 12 and 18 dSm−1 by 29.57 compared to 40.58% in control (). Stem FW of ʽAhmad-Aghaiʼ and ʽAkbariʼ were decreased at 12 and 18 dSm−1 in comparison with control (31.35% and 41.18%) and (29.50% and 43.27%), respectively (). The same trend was observed in root DW under salinity conditions. ʽAkbariʼ and ʽAhmad-Aghaiʼ had the lowest reduction in FW and DW of root under salinity stress. Reduction of root FW in ʽAkbariʼ at a salinity of 12 and 18 dSm−1 was 15.2% and 18.45%, while in ʽAhmad-Aghaiʼ was 8.6% and 10.8%, and in ʽUCB-1ʼ was 32.5% and 33.79% compared to control, respectively ().
Table 1. The effects of different salinity levels and genotypes on some characteristics of pistachio genotypes
Stem diameter, number of growing leaves, leaf abscission and leaf area were studied to assess the adverse effects of high salinity on the growth of pistachio rootstocks (). Salinity levels significantly reduced the stem diameter at 12 and 18 dSm−1 in comparison with control by 59.88%. The uppermost adverse effects of salinity stress on stem diameter were observed in ʽBadamiʼ genotype at 12 and 18 dSm−1 by 59.32% and 66.44%, respectively (). After 75 days, the average number of growing leaves significantly reduced via salinity stress at 12 and 18 dSm−1 by 62.54% and 73.90%, respectively (). The uppermost negative effects of salinity stress at 12 and 18 dSm−1 on a number of growing leaves were observed in ʽBadamiʼ genotype by 80.64% and 82.25% reduction, respectively. ʽAkbariʼ with average growing leaves of 10.5% significantly had more growing leaves than ʽUCB-1ʼ at 12 dSm−1 salinity stress. Increasing salinity up to 12 and 18 dSm−1 significantly increased leaf abscission by 98.76% and 98.93%, respectively. Under salinity stress, the lowest leaf abscission was observed in ʽUCB-1ʼ and the difference was significant with other genotypes. Thereafter, the lowest leaf abscission was observed in ʽAkbariʼ and ʽAhmad-Aghaiʼ genotypes. Leaf area was also affected by increasing the salinity levels (). Salinity stress significantly reduced the average leaf area at 12 and 18 dSm−1 by 24.46% and 41.33%, respectively. Compared to other genotypes, ʽUCB-1ʼ, ʽAkbariʼ and ʽAhmad-Aghaiʼ had the highest leaf area during growing period under salinity conditions. Salinity-reduced leaf area in ʽUCB-1ʼ at 12 dSm−1 by 44.70%, while it was 3.14% in ʽAkbariʼ and 9.49% in ʽAmad-Aghaiʼ.
Table 2. The effects of different salinity levels and genotypes on some characteristics of pistachio genotypes
Growth rate was also evaluated in the study to show the adverse effects of salinity treatments on the growth of pistachio genotypes (). The interaction of genotypes and salinity levels on growth rate are shown in . ʽUCB-1ʼ significantly lost the growth up to 79.6% under salinity stress. Salinity stress had lesser negative effects on growth of ʽAkbariʼ (43.1%) and ʽAhmad-Aghaiʼ (58.85%) genotypes.
Figure 2. The effects of different salinity treatments on growth rate of the genotypes tested, AK: ʽAkbariʼ, AH: ʽAhmad-Aghaiʼ, IT: ʽItalyayiʼ, GH: ʽGhazviniʼ, BA: ʽBadamiʼ. Means with the same letter(s) are not significantly different at 5% level using DMRT and error bars are Standard Errors
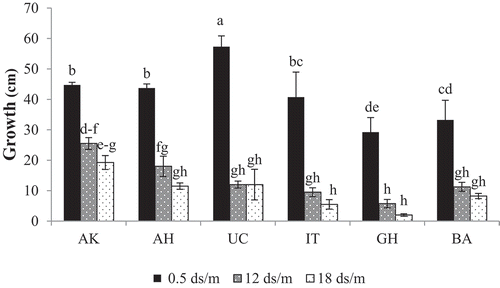
In this study, the growth of ʽAkbariʼ genotype at 12 dSm−1 salinity stress was more than ʽUCB-1ʼ. The highest and the lowest growth rate at 18 dSm−1 of NaCl treatment were observed in ʽAkbariʼ (19 cm) and ʽGhazviniʼ (2 cm) genotypes, respectively ().
Physiological Parameters
Water Use Efficiency (WUE)
Results showed that the main effects of genotype and salinity levels and also their interaction were significant at 1% level of probability (). WUE generally decreased by increasing NaCl concentrations (). The WUE of ʽBadamiʼ, ʽGhazviniʼand ʽItalyayiʼ significantly reduced under salinity stress, although WUE of the ʽItalyayiʼ genotype at 12 dSm−1 salinity level was more than the others. Our results showed that ʽUCB-1ʼ had the highest WUE and better growth rate under normal condition and produced more dry matter. The reduction of WUE was obvious at 12 and 18 dSm−1 salinity and plants were not able to keep their growth and dry weight production. The WUE of ʽUCB-1ʼ at 12 dSm−1 salinity reduced by 26.12%, although it was higher than ʽAkbariʼ (22.47%).
Figure 3. The effects of different salinity treatments on water use efficiency of the genotypes tested, AK: ʽAkbariʼ, AH: ʽAhmad-Aghaiʼ, IT: ʽItalyayiʼ, GH: ʽGhazviniʼ, BA: ʽBadamiʼ. Means with the same letter(s) are not significantly different at 5% level using DMRT and error bars are Standard Errors
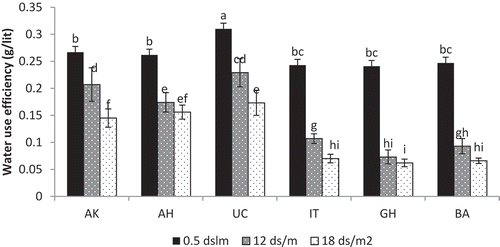
Relative Water Content (RWC)
The results of this study showed that salinity significantly reduced RWC of genotypes at 12 and 18 dSm−1 by 9.2% and 17.4%, respectively. There was no significant difference among genotypes for RWC under salinity stress.
Electrolyte Leakage (EL)
Leaf
EL was affected by genotypes and salinity levels (). EL increased at 12 and 18 dSm−1 of salinity by 76.75% and 124.42%, respectively. ʽBadamiʼ genotype had the highest EL (69.25%), at salinity of 18 dSm−1 but ʽUCB-1ʼ had the lowest EL and the difference was not significant with ʽAkbariʼ, ʽAhmad-Aghaiʼ and ʽItalyayiʼ genotypes.
Table 3. The effects of different salinity levels and genotypes on some characteristics of pistachio genotypes
Root
In the roots, the same as leaves, by increasing the salinity levels the EL increased and the effect of genotype on EL was significant. Root EL in ʽBadamiʼ genotype at salinity of 18 dSm−1 was 62.20% and it was 116% more than control treatment (). The lowest EL was observed in ʽUCB-1ʼ (29.25%) and had no significant difference with ʽAkbariʼ genotype (32.7%).
Stomatal Conductance (SC)
The SC was decreased by increasing the salinity levels. SC decreased at salinity levels of 12 and 18 dSm−1 by 32.83% and 42.25%, respectively, and there was no significant difference among genotypes under salinity conditions ().
SPAD Index
SPAD index significantly reduced in six genotypes under salinity stress. SPAD index reduced at 12 and 18 dSm−1 of salinity by 16.22% and 28.43%, respectively (). ʽGhazviniʼand ʽBadamiʼ genotypes had the lowest SPAD index. ʽBadamiʼ showed 38.82 reduction in SPAD index at highest salinity level (18 dSm−1) and the uppermost SPAD index was observed in ʽAhmad-Aghaiʼgenotype ().
Table 4. The effects of different salinity levels and genotypes on some characteristics of pistachio genotypes
Total Chlorophyll
Salinity stress significantly reduced the total chlorophyll content. Salinity at 12 and 18 dSm−1 reduced total chlorophyll content by 30.58% and 51.94%, respectively. ʽAkbariʼ had the highest and ʽBadamiʼ had the lowest leaf chlorophyll content under salinity stress ().
Total Soluble Carbohydrates (TSCs)
Salinity and genotypes had significant effects on TSCs content of leaves (). The TSCs significantly augmented by increasing the salinity levels from 0.5 to 12 dSm−1. The TSCs increased 18.36% by increasing the salinity to 12 dSm−1 and it was significantly different with control. ʽGhazviniʼ, ʽBadamiʼ and ʽItalyayiʼ genotypes had lower TSCs content than other genotypes.
ʽItalyayiʼ had the lowest TSCs content at salinity of 18 dSm−1, but ʽUCB-1ʼ had the highest TSCs content at this level of salinity (), although it was not significantly different with ʽAkbariʼ and ʽAhmad-Aghaiʼ.
Starch Content of Leaf
Salinity levels and genotypes had significant effects on starch content of leaves. Salinity stress reduced starch content at 12 and 18 dSm−1 by 25.40% and 22.28%, respectively (). The uppermost starch content under normal condition (without salinity stress) were observed in ʽUCB-1ʼ and ʽBadamiʼ, respectively. ʽAkbariʼ had the highest starch content at salinity stress of 12 and 18 dSm−1. ʽGhazviniʼ had the lowest starch content at salinity level of 18 dSm−1 ().
Discussion
Growth Parameters
Pistachio has been considered as a salt tolerant plant by many authors (Sepaskhah and Maftoon, Citation1981; Behboudian et al., Citation1986; Picchioni et al, Citation1990; Ferguson et al., Citation2002; Karimi et al., Citation2009a; Hajiboland et al., Citation2014). According to previous reports, salinity reduced the rate of photosynthesis, water uptake and growth and development of pistachio trees (ʽAkbariʼ et al., Citation2018). In the present study, FW and DW of leaves, stem, and root decreased and the greatest reduction was observed in the leaves. In this study, FW and DW of leaves under 12 and 18 dSm−1 salinity were decreased by 41.30% and 62.24% but at the same salinity levels, FW and DW of roots were decreased 21.75% and 31.62%, respectively. In spite of the direct exposure of roots to salinity, their growth is less vulnerable to salt than shoots (Munns, Citation2002). Accumulation of Na+ in the roots is an adaptive response in many woody plants to avoid its toxicity in the shoots (Guccia and Tattini, Citation1997; Picchioni et al., Citation1990). Picchioni and Miyamato (1990) reported that by increasing salinity levels the root to shoot ratio was augmented in all pistachio rootstocks. In the present study, ʽBadamiʼ, ʽGhazviniʼ and ʽItalyayiʼ genotypes were less tolerant to salinity compared to ʽAkbariʼ, ʽAhmad-Aghaiʼand ʽUCB-1ʼ. This is in agreement with Mirfattahi et al. (Citation2017) results. They reported that ʽAkbariʼ was more tolerant to salinity than ʽGhazviniʼ. On the contrary, Akbari et al. (Citation2018) found that ʽAkbariʼ had the lowest tolerance to salinity stress.
In our study, ʽUCB-1ʼ had the highest FW and DW of leaves under salinity stress that is in agreement with the report of Akbari et al. (Citation2018). In fact, there are still varying reports on the degree of salt tolerance in pistachio species and/or cultivars. These variations might be due to the different environmental conditions, inter- and intra-specific variations, and soil type. The results of the present study are in agreement with the reports of Sepaskhah et al. (Citation1985), and Hokmabadi et al. (Citation2005). They reported that relative growth rate (RGR) and net assimilation rate (NAR) decreased with increasing NaCl concentrations.
Stem diameter, number of growing leaves, number of leaves abscised and leaf area are morphological indices that decreased by increasing NaCl concentrations. The stem height and diameter are indices of pistachio growth. The results of this study showed that salinity at 12 and 18 dSm−1 significantly reduced the stem diameter of ʽBadamiʼ genotype by 59.32% and 66.44%, respectively. This is in agreement with the report of Mirfattahi et al. (Citation2017). They reported that salinity stress reduced plant biomass, height, stem diameter and leaf number in pistachio genotypes. Decrease in stem diameter under salinity stress in pecan (Miyamoto et al., Citation1986) and pistachio (Ferguson et al., Citation2002; Yaron et al., Citation1969) was primarily due to leaf abscission. It was also reported that the decrease in stem diameter under salinity stress was due to the reduction in turgor potential and cell division (Ashraf and Sarwar, Citation2002). Escalona et al. (Citation2002) reported that the main reasons for reduction in stem diameter of pistachio seedlings were the limitation of photoassimilates accumulation and root function in water transport under salinity stress.
In present study, ʽAkbariʼ at 12 and 18 dSm−1 salinity had higher growing leaf number than ʽUCB-1ʼ and their difference was significant at 1% level of probability. This finding was in agreement with the results of Mirfattahi et al. (Citation2017). They reported that under salinity stress, the growth of ʽAkbariʼ seedling was higher than the other genotypes. ʽUCB-1ʼ and ʽAkbariʼ had the lowest leaf abscission and the highest leaf area under salinity stress than the other genotypes that was similar to the results of Mirfattahi et al. (Citation2017). Moreover, our study confirmed the results of Karimi et al. (Citation2009b) concerning leaf abscission in pistachio seedlings under high salinity stress. This decrease was due to accumulation of Na+ in the leaf of seedlings and following leaf senescence and abscission. However, decreasing the leaf number might be due to the reduction in roots growth, suggesting that sink in leaves and stem strongly inhibited under salinity stress by reduction in plant photosynthesis (Karimi et al., Citation2009b). Increasing salinity up to 12 and 18 dSm−1 significantly increased leaf abscission by 98.76% and 98.93%, respectively. ʽUCB-1ʼ had no leaf abscission under high salinity stress.
Leaf area was affected by increasing salinity levels in different pistachio genotypes. ʽUCB-1ʼ, ʽAkbariʼ and ʽAhmad-Aghaiʼ compared to other genotypes had the highest leaf area under salinity conditions. Salinity at 12 dSm−1 significantly reduced leaf area in ʽUCB-1ʼ (4.7%) in comparison with ʽAkbariʼ and ʽAhmad-Aghaiʼ.
The present study showed that salinity stress-induced leaf abscission, decreased number of growing leaves and leaf area that is in agreement with the previous reports (Rahneshan et al., Citation2018; Mirfattahi et al., Citation2017; Karimi et al., Citation2009b; Karimi and Nasrolahpour, Citation2016). Leaves of six genotypes showed different responses to salinity stress. ʽGhazviniʼ, ʽBadamiʼ and ʽItalyayiʼ genotypes had significantly lower leaf area index under salinity stress. It seems that lower growth and thickness of shoots were caused by higher leaf abscission, lower leaf production and higher reduction in leaf area under high salinity levels. ʽUCB-1ʼ, ʽAkbariʼ and ʽAhmad-Aghaiʼ produced more leaves and showed a lower reduction of leaf area under salinity stress. ʽAkbariʼ at salinity of 18 dSm−1 showed lowest leaf area reduction that probably was due to the reduction in cell division and cell elongation of this genotype under salinity conditions. ʽUCB-1ʼ had no leaf abscission under salinity stress but its leaf area significantly reduced compared to control. Reduction in leaf area under salinity stress, can cause limited synthesis and accumulation of carbohydrates and then reduced plant growth under this condition. With concerning the growth parameters ʽBadamiʼ, ʽGhazviniʼ and ʽItalyayiʼ genotypes showed uppermost growth reduction under salinity stress. Therefore, these genotypes are very sensitive to high salinity levels. ʽUCB-1ʼ, ʽAkbariʼ and ʽAhmad-Aghaiʼ genotypes had a better growth rate under the same conditions.
Growth rate was studied in this experiment to find out the adverse effects of salinity stress on growth and development of pistachio genotypes. Salinity stress had lesser adverse effects on growth rate of ʽAkbariʼ and ʽAhmad-Aghaiʼ, but significantly reduced the growth rate of ʽUCB-1ʼ. ʽAkbariʼ genotype at higher salinity levels was more tolerant than ʽUCB-1ʼ. ʽGhazviniʼ genotype had the lowest growth rate under high salinity levels.
Our results are in agreement with the reports of Karimi et al. (Citation2014) and Rahneshan et al. (Citation2018) in pistachio rootstocks. Previous reports indicated that the reduction of PGRs in pistachio genotypes has been attributed to a decrease in carbon assimilation due to the limitation of stomatal conductance and/or impairment (Hajiboland et al., Citation2014). Furthermore, the reduction in PGRs under salinity stress has been attributed to the inhibition of cell division and enlargement (Munns and Tester, Citation2008; Zhu, Citation2001).
Physiological Parameters
Water Use Efficiency (WUE)
This study showed that the WUE of ʽBadamiʼ, ʽGhazviniʼ and ʽItalyayiʼ significantly reduced under salinity stress. Data showed that ʽUCB-1ʼ had the highest WUE. This finding is in agreement with the results of Karimi et al (Citation2016) that reported decreased WUE in female seedlings of ʽBadami-Riz-e-Zarandʼ under salinity stress, whereas was not affected in male seedlings. In this study, decreasing of WUE was more abundant in ʽBadamiʼ, ʽGhazviniʼ and ʽItalyayiʼ seedlings and ʽUCB-1ʼ seedlings had more WUE than those genotypes under 12 and 18 dSm−1 salinity levels. The results indicated that ʽUCB-1ʼ could use the transferred water to the shoot more effectively and store more biomass in the shoot.
Relative Water Content (RWC)
Relative water content (RWC) significantly reduced at salinity of 12 and 18 dSm−1 by 4.2% and 17.4%, respectively, and there were no significant difference among the genotypes. It seems that RWC can not to be a sole parameter and other physiological responses of plant should be considered as well. Mirfattahi et al. (Citation2017) reported that ʽAkbariʼ and ʽGhazviniʼ× ʽAkbariʼ seedlings were able to preserve leaf RWC with lower proline content in their leaves and suggested that other mechanisms were more important than osmoregulation which can help pistachio rootstocks to prevent leaf water loss under salinity stress.
Electrolyte Leakage (EL)
In this study, genotypes and salinity levels were effective on EL. Membrane permeability is determined by measuring EL. The high salinity treatments of 12 and 18 dSm−1 significantly increased the EL by 76.75% and 124.42%, respectively. EL of leaf and root in ʽBadamiʼ genotype was the highest, but in contrast, the EL of these tissues in ʽUCB-1ʼ under high salinity of 18 dSm−1 were lower compared to control plants. Tavallali et al. (Citation2008) reported that high salt concentration (150 mM NaCl) significantly increased EL in ʽGhazviniʼ and ʽBadami-e- Zarandʼ compared to control plants. High salinity stress could damage the integrity of cellular membrane as well as cellular components such as lipids and proteins. Maintaining the integrity of cellular membrane under salinity stress is considered as integral part of salinity tolerance mechanism (Steven et al., Citation2006).
Stomatal Conductance (SC)
The SC was decreased by increasing the salinity levels. The results of this study are in agreement with previous report on growth inhibition under severe salinity in pistachio that has been related to a decrease in carbon assimilation due to limitation of stomatal conductance (Hajiboland et al., Citation2014). Photosynthetic activity can be inferred by modifying either stomatal conductance or mesophyll capacity to assimilation (Forquhr and Sharkey, Citation1982). ʽGhazviniʼ genotype showed a significant reduction in photosynthetic rates under salinity conditions (Hajiboland et al., Citation2014). ʽGhazviniʼ has large stomata (Arzani et al., Citation2013) and inefficiency of stomatal regulation in its leaves is expected (Bosabalidis and Kofidis, Citation2002).
SPAD Index
Salinity at 12 and 18 dSm−1 reduced SPAD index of leaves by 16.22% and 24.8%, respectively. ʽGhazviniʼ and ʽBadamiʼ genotypes had the lowest SPAD index, and the highest was observed in ʽAhmad-Aghaiʼ. Our results are in agreement with the report of Karimi and Sadeghi-Seresht (Citation2018) that by increasing salinity to 120 mM, SPAD index declined in Banebaghi (Pistacia sp.) as a rootstock for pistachio. Decline in SPAD index in pistachio seedlings was due to chlorophyll degradation by free radicals produced under salinity conditions (Desingh and Kanagraj, Citation2007).
Total Chlorophyll
Salinity stress significantly reduced total chlorophyll content. Our results supported Tavallali et al. (Citation2008) report that ʽBadami-e-Zarandʼ had more chlorophyll content than ʽGhazviniʼ under salinity stress conditions. Decrease in chlorophyll index under salinity condition can be related to destruction of chloroplast, and inhibition of biosynthesis of new chlorophylls (Karimi and Nasrolahpour-Moghadam, Citation2016).
Total Soluble Carbohydrates (TSCs)
The TSCs were significantly increased by raising the salinity levels from 0.5 to 12 dSm−1. ʽUCB-1ʼ had the highest TSCs at higher salinity level and the lowest was observed in ʽItalyayiʼ genotype. The results of this study were in agreement with the previous report by Akbari et al. (Citation2018). They found that maximum increase of TSCs was belonged to UCB-1 and accumulated more in both leaves and roots than other rootstocks. Accumulation of carbohydrates probably improve the plants’ ability to avoid tissue death under salinity stress by increasing osmotic adjustment, protecting molecules and balancing accumulated ions in vacuoles (Tang et al., Citation2013).
Starch Content of Leaf
Salinity treatments at 12 and 18 dSm−1 reduced starch content of the leaves. ʽAkbariʼ had the highest starch content at high salinity levels and the lowest was observed in ʽGhazviniʼ. It might reveal that reduction in plants’ photosynthesis by salinity declined NAR and starch content in the leaves.
Conclusion
In conclusion, our findings revealed that ʽAkbariʼ, ʽAhmad-Aghaiʼ and ʽUCB-1ʼ had the lowest reduction in morphological and growth parameters under salinity stress. Moreover, these genotypes had the highest chlorophyll content, SPAD index and soluble sugar content under salinity conditions. It seems that these rootstocks by increasing osmoregulation, protector substances and balancing of toxic ions had better growth and morphological characteristics under salinity stress. We recommend using these genotypes as rootstock for commercial pistachio cultivars under moderate salinity conditions.
References
- Akbari, M., N. Mahna, K. Ramesh, A. Bandehagh, and S. Mazzuca. 2018. Ion homeostasis, osmoregulation, and physiological changes in the roots and leaves of pistachio rootstocks in response to salinity. Protoplasma 255(5):1349–1362. doi: 10.1007/s00709-018-1235-z.
- Aliakbarkhani, S.T., M. Akbari, A. Hassankhah, A. Talaie, and M.F. Moghadam. 2015. Phenotypic and genotypic variation in Iranian Pistachios. J. Genet. Engineer. Biotech. 13(2):235–241. doi: 10.1016/j.jgeb.2015.05.003.
- Arnon, D.I. 1949. Copper enzymes in isolated chloroplasts. Polyphenoloxidase in Beta vulgaris. Plant P 24(1):1. doi: 10.1104/pp.24.1.1.
- Arzani, K., M. Ghasemi, A. Yadollahi, and H. Hokmabadi. 2013. Study of foliar epidermal anatomy of four pistachio rootstocks under water stress. Idesia 31(1):101–108. doi: 10.4067/S0718-34292013000100012.
- Ashraf, M.Y., and G. Sarwar. 2002. Salt tolerance potential in some members of Brassicaceae physiological studies on water relations and mineral contents, p. 237–245. In: Ahmad R., Malik K.A. (eds) Prospects for saline Agriculture. Springer, Dordrecht.
- Bartolozzi, F., and G. Fontanazza. 1999. Assessment of frost tolerance in olive (Olea europaea L.). Sci. Hort. 81(3):309–319. doi: 10.1016/S0304-4238(99)00019-9.
- Behboudian, M.H., R.R. Walker, and E. Törökfalvy. 1986. Effects of water stress and salinity on photosynthesis of pistachio. Sci. Hort. 29(3):251–261. doi: 10.1016/0304-4238(86)90068-3.
- Bosabalidis, A.M., and G. Kofidis. 2002. Comparative effects of drought stress on leaf anatomy of two olive cultivars. Plant Sci. 163(2):375–379. doi: 10.1016/S0168-9452(02)00135-8.
- Desingh, R., and G. Kanagaraj. 2007. Influence of salinity stress on photosynthesis and antioxidative systems in two cotton varieties. Gen. Appl. Plant Physiol. 33(3–4):221–234.
- Escalona, J., J. Flexas, and H. Medrano. 2002. Drought effects on water flow, photosynthesis and growth of potted grapevines. VITIS 41(2):57–62.
- Farquhar, G.D., and T.D. Sharkey. 1982. Stomatal conductance and photosynthesis. Annu. Rev. Plant Physiol. 33(1):317–345. doi: 10.1146/annurev.pp.33.060182.001533.
- Ferguson, L., J.A. Poss, S.R. Grattan, C.M. Grieve, D. Wang, C. Wilson, and C.T. Chao. 2002. Pistachio rootstocks influence scion growth and ion relations under salinity and boron stress. J. Am. Soc. Hortic. Sci. 127(2):194–199. doi: 10.21273/JASHS.127.2.194.
- Fox, J.D., and J.F. Robyt. 1991. Miniaturization of three carbohydrate analyses using a microsample plate reader. Anal. Biochem. 195(1):93–96. doi: 10.1016/0003-2697(91)90300-I.
- Ghanaatiyan, K., and H. Sadeghi. 2017. Differential responses of chicory ecotypes exposed to drought stress in relation to enzymatic and non-enzymatic antioxidants as well as ABA concentration. J. Hortic. Sci. Biotech. 92(4):404–410.
- Gucci, R., and M. Tattini. 1997. Salinity tolerance in olive. Hortic. Rev. 21:177–214.
- Hajiboland, R., F. Norouzi, and C. Poschenrieder. 2014. Growth, physiological, biochemical and ionic responses of pistachio seedlings to mild and high salinity. Trees 28(4):1065–1078. doi: 10.1007/s00468-014-1018-x.
- Hokmabadi, H., K. Arzani, and P.F. Grierson. 2005. Growth, chemical composition, and carbon isotope discrimination of pistachio (Pistacia vera L.) rootstock seedlings in response to salinity. Aust. J. Agric. Res 56(2):135–144. doi: 10.1071/AR04088.
- Jafari, M., M. Rahemi, and A.A.K. Haghighi. 2018. Role of fig rootstock on changes of water status and nutrient concentrations in ‘Sabz’cultivar under drought stress condition. Sci. Hort. 230, 56–61
- Karimi, H.R., and A.M. Kuhbanani. 2015. The evaluation of inter-specific hybrid of P. atlantica× P. vera cv.‘Badami Zarand’as a pistachio rootstock to salinity stress. J. Nuts 6(2):113–122.
- Karimi, H.R., and E. Sadeghi-Seresht. 2018. Effects of salinity stress on growth indices, physiological parameters and element concentration in Banebaghi (Pistacia sp.) as rootstock for pistachio. J. Plant Nutr. 41(9):1094–1103. doi: 10.1080/01904167.2017.1392572.
- Karimi, H.R., and S. Nasrolahpour-Moghadam. 2016. Study of sex-related differences in growth indices and eco-physiological parameters of pistachio seedlings (Pistacia vera cv. Badami-Riz-e-Zarand) under salinity stress. Sci. Hort. 202:165–172. doi: 10.1016/j.scienta.2016.03.003.
- Karimi, H.R., and Z. Hasanpour. 2014. Effects of salinity and water stress on growth and macro nutrients concentration of pomegranate (Punica granatum L.). J. Plant Nutr. 37(12):1937–1951. doi: 10.1080/01904167.2014.920363.
- Karimi, H.R., Z. Zamani, A. Ebadi, and M.R. Fatahi. 2009a. Morphological diversity of Pistacia species in Iran. Genet. Resour. Crop Evol. 56(4):561–571. doi: 10.1007/s10722-008-9386-y.
- Karimi, H.R., Z. Zamani, A. Ebadi, and R. Fatahi. 2012. Effects of water salinity on growth indices and physiological parameters in some wild pistachio. J. Nuts. 3:41–48.
- Karimi, S., M. Rahemi, M. Maftoun, and V. Tavallali. 2009b. Effects of long-term salinity on growth and performance of two pistachio (Pistacia L.). Rootstocks. Aust. J. Basic Appl. Sci. 3(3):1630–1639.
- McCready, R.M., J. Guggolz, V. Silviera, and H.S. Owens. 1950. Determination of starch and amylose in vegetables. Anal. Chem. 22(9):1156–1158. doi: 10.1021/ac60045a016.
- Mirfattahi, Z., S. Karimi, and M.R. Roozban. 2017. Salinity induced changes in water relations, oxidative damage and morpho-physiological adaptations of pistachio genotypes in soilless culture. Acta Agric. Slov 109(2):291–302. doi: 10.14720/aas.2017.109.2.12.
- Miyamoto, S., T. Riley, G. Gobran, and J. Petticrew. 1986. Effects of saline water irrigation on soil salinity, pecan tree growth and nut production. Irrig. Sci. 7(2):83–95. doi: 10.1007/BF00259425.
- Munns, R. 2002. Comparative physiology of salt and water stress. Plant Cell Environ. 25(2):239–250. doi: 10.1046/j.0016-8025.2001.00808.x.
- Munns, R., and M. Tester. 2008. Mechanisms of salinity tolerance. Annu. Rev. Plant Biol. 59:651–681. doi: 10.1146/annurev.arplant.59.032607.092911.
- Panahi, B., A. Ismail-Poor, F. Farbood, M. Moazin-Poor, and H. Farivar-Mahin. 2002. Pistachio Handbook (planting, processing and harvesting). 149. Agricultural Training Publication, Tehran, Iran. In Persian.
- Parida, A.K., and A.B. Das. 2005. Salt tolerance and salinity effects on plants: A review. Ecotoxicol. Environ. Saf. 60:324–349. doi: 10.1016/j.ecoenv.2004.06.010.
- Picchioni, G.A., S. Miyamoto, and J.B. Storey. 1990. Salt effects on growth and ion uptake of pistachio rootstock seedlings. J. Am. Soc. Hortic. Sci. 115(4):647–653. doi: 10.21273/JASHS.115.4.647.
- Rahneshan, Z., F. Nasibi, and A.A. Moghadam. 2018. Effects of salinity stress on some growth, physiological, biochemical parameters and nutrients in two pistachio (Pistacia vera L.) rootstocks. J. Plant Interact’ 13(1):73–82. doi: 10.1080/17429145.2018.1424355.
- Sairam, R.K., and A. Tyagi. 2004. Physiology and molecular biology of salinity stress tolerance in plants. Curr. Sci. 86(3): 407–421.
- Sepaskhah, A.R., and M. Maftoun. 1981. Growth and chemical composition of pistachio cultivars as influenced by irrigation regimes and salinity levels of irrigation water. 1. Growth. HortScience 56(4):277–284.
- Sepaskhah, A.R., M. Maftoun, and N. Karimian. 1985. Growth and chemical composition of pistachio as affected by salinity and applied iron. HortScience 60(1):115–121.
- Stevens, J., T. Senaratna, and K. Sivasithamparam. 2006. Salicylic acid induces salinity tolerance in tomato (Lycopersicon esculentum cv. Roma): Associated changes in gas exchange, water relations and membrane stabilisation. Plant Growth Regul. 49(1):77–83.
- Talebi, M., M. Akbari, M. Zamani, and B.E. Sayed-Tabatabaei. 2016. Molecular polymorphism in Pistacia vera L. using non-coding regions of chloroplast DNA. J. Genet. Engineer. Biotech 14(1):31–37. doi: 10.1016/j.jgeb.2016.04.002.
- Tang, J., J.J. Camberato, X. Yu, N. Luo, S. Bian, and Y. Jiang. 2013. Growth response, carbohydrate and ion accumulation of diverse perennial ryegrass accessions to increasing salinity. Sci. Hort. 154:73–81. doi: 10.1016/j.scienta.2013.02.021.
- Tavallali, V., M. Rahemi, and B. Panahi. 2008. Calcium induces salinity tolerance in pistachio rootstocks. Fruits 63(5):285–296. doi: 10.1051/fruits:2008024.
- Yaron, B., N. Zieslin, and A.H. Halevy. 1969. Response of Baccara roses to saline irrigation. J Amer. Soc. Hort. Sci. 94:481–484.
- Zhu, J.K. 2001. Plant salt tolerance. Trends Plant Sci. 6(2):66–71. doi: 10.1016/S1360-1385(00)01838-0.