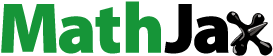
ABSTRACT
The aim of this research was to evaluate the effect of exogenous jasmonic acid and abscisic acid, at different salinity regimes, on primary and secondary metabolic changes in ʻKurdistanʼ and ʻQueen Elisaʼ strawberry leaves. To this end, strawberry plants were induced by gradual NaCl salinity (10 mmol•L−1) and shock salinity. The plants were sprayed with ABA (40 mmol•L−1) and JA treatments up to 40 mmol•L−1. In both cultivars, salt shock stress significantly enhanced the total antioxidant capacity, though the JA treatment outperformed the ABA treatment. Increasing JA level in the presence of ABA resulted in downswing in total phenolic content (6.42% in ʻKurdistanʼ and 23.13% in ʻQueen Elisaʼ) and antioxidant capacity (12.9% in ʻKurdistanʼ and 17.7% in ʻQueen Elisaʼ) at non-saline conditions. Adding both hormonesresulted in a 20.82% increase in total phenolic content and a 17.86% increase in total antioxidant capacity in ‘Kurdistan’ at gradual salt stress; however, in ʻQueen Elisaʼ, the same treatment resulted in 21.98% increase in total phenol and 11.92% increase in total antioxidant capacity at salt shock. Salt shock induced tyrosine increase and both hormones enhanced asparagine, alanine, histidine, and GABA contents in ʻQueen Elisaʼ. The study reveals that the applation of abscisic acid along with different jasmonic acid concentrations activates the protective mechanism of the strawberry plant against NaCl stress. Further studies are needed to compare the exact salt stress tolerance mechanisms for these cultivars.
Abbreviations: JA: Jasmonic acid; ABA: Abscisic Acid; GABA: γ-Aminobutyric acid
Introduction
Salt stress is one of the most serious environmental threats to crop production. According to an estimation, by the middle of the twenty first century, up to 50% of arable lands will have become uncultivable due to high salinity of soil and irrigation water (Mahajan and Tuteja, Citation2005). Plant metabolism can be severely affected by high salinity of soil and/or irrigation water. The effect of salt stress on plants depends on salt concentration and exposure time, plant genotype and environmental factors, such as high temperature and light conditions (Hasanuzzaman et al. Citation2013; Ivanov, Citation2010).
Strawberry plants are considered as salinity sensitive species, although there are differences in their sensitivity to salinity among cultivars. Adverse effects of salinity on strawberry plant growth, fruit yield and productivity have been reported in several studies (e.g. D’ Anna et al., Citation2003; Kaya et al., Citation2002; Keutgen and Pawelzik, Citation2009). An increase in polyphenol content in different tissues under increasing salinity was reported for different plants (Parida and Das, Citation2005). In one study, moderate salinity of about 40 mmol•L−1 increased both antioxidant capacity and phenol content in ʻKoronaʼ strawberries (Keutgen and Pawelzik, Citation2007a). Salinity in nutrient solution was reported to decrease total soluble protein content in strawberry leaves, while total and specific peroxidase activities increased compared with control treatments (Gulen et al., Citation2006). In two studies, salinity levels of 40 and 80 mmol•L−1 enhanced ascorbic acid as well as contents of total and free amino acids including proline, asparagine and glutamine (Keutgen and Pawelzik, Citation2008a, Citation2008b).
In two recent studies, the response of plants to salt stress was found to be influenced by phytohormones such as methyl jasmonate, abscisic acid (ABA) and cytokinin (Yoon et al., 2009; Parida and Das, Citation2005). Moreover, abscisic acid was found to be distinguished at several extracellular and intracellular sites (Liu et al., Citation2007). Kempa et al. (Citation2008) reported that high salinity and ABA had an effect on carbohydrate metabolism and that ABA stimulated the initial steps of carbon metabolism. Other researchers reported that in Arabidopsis thaliana, leucine, isoleucine and valine accumulated in response to salt treatment. Nonetheless, Abscisic acid deficiency in plants resulted in lower accumulation of amino acids (Huang and Jender, Citation2017). Jasmonic acid (JA) and its methyl esters have hormone properties, which play a remarkable role in the adaptation of plants to biotic and abiotic stress factors (Wasternach and Hause, Citation2003). Accelerating activities of antioxidant enzymes and enhancing the concentration of anti-oxidative compounds, JA could eliminate the adverse effects of salt stress in wheat seedlings (Qui et al, Citation2014).
Probably the regulation of plant water balance and osmotic stress tolerance are the main functions of ABA at salt stress conditions (Tuteja, Citation2007). In one experiment Jasmonic acid and/or ABA controlled leaf growth and acidification of the leaf apoplast in maize genotypes during the first phase of salt stress (Shahzad, Citation2011). On the other hand, JA interaction with ABA regulated plant responses to water stress conditions in Arabidopsis, where a complex JA-ABA crosstalk was found (Ollas et al., Citation2015). Nonetheless, the impact of stress hormones, especially of JA, on metabolic characteristics in strawberry cultivars under salt stress has so far been little investigated. . Additionally, to the best of researchers’ knowledge, there are no data comparing strawberry plants under gradual and shock salt stress, exposed to JA and ABA.
Saadati and Moallemi (Citation2012) reported that 30 mM NaCl stress reduced leaf number and area, fresh and dry weight of root and shoot, yield indexes, chlorophyll content, and leaf relative water content in ʻKurdistanʼ and ʻQueen Elisaʼ strawberry by 67.97% and 45.88% respectively. The higher salinity resistance of ‘Kurdistan’ might be due to higher relative water content and higher activity of enzymes (Ghaderi et al., Citation2018). Faghih et al. (Citation2018) reported that when strawberry plants were induced by salicylic acid and methyl jasmonate, the undesirable effects of salinity were eliminated. Methyl jasmonate effect was also studied in tomato plants by altering biochemical and physiological changes (Manan et al., Citation2016), However, the interaction of ABA and JA on metabolic characteristics of the forenamed cultivars under salt stress is still ambiguous. ‘Kurdistan’ is a high quality short day native strawberry cultivar with small fruit sizecommonly grown in Sanandaj area in Kurdistan, Iran. ʻQueen Elisaʼ is a short day cultivar originally grown in Italy, which is also grown regularly in Iran. Given the afore-mentioned research gaps, the present study investigated the effects of exogenous JA and ABA exposure, at different salinity regimes, on primary and secondary metabolites in the leaves of ‘Kurdistan’ and ‘Queen Elisa’ cultivars.
Material and Methods
Plant Material and Condition of Growth
Strawberry plants (Fragaria × ananassa Duch. cvs. ‘Queen Elisa’ and ‘Kurdistan’) were singly planted in 2 L pots filled with a mixture of perlite and cocopeat (1:1).Then, they were fed continuously with 250 mL of half strength Hoagland nutrient solution [Macronutrients: NH4H2PO4 (115.03 g•L−1), KNO3 (101.10 g•L−1), Ca(NO3)2.4H2O (236.15 g•L−1), MgSO4.7H2O (246.47 g•L−1), Micronutrients: KCl (3.728 g•L−1), H3BO3 (1.546 g•L−1), MnSO4.H2O (0.845 g•L−1), ZnSO4.7H2O (0.575 g•L−1), CuSO4.5H2O (0.125 g•L−1), H3MoO4.H2O (0.09 g•L−1), FeEDDHA (9.31 g•L−1)]. Final EC for the nutrient solution recorded was 0.92 ds•m−1. The plants were placed randomly in a greenhouse with day/night temperature of 25/16°C, relative humidity of 75-80% and light conditions of 12h light/12h dark with 600 lux light intensity.
Experimental Design
The experiment was carried out in a fully randomized three factorial design (3 factors: salt stress regimes, ABA and JA treatments) with three replicates per treatment and three plants per replicate. To induce salt stress, salinity was increased by adding sodium chloride (NaCl) to the nutrient solution. Rooted runners at 4–6 leaves growth stage were used to start the treatments. Gradual NaCl stress treatment was performed with up to 10 mmol•L−1 over 5 weeks as follows: first week: 0 mmol•L−1 NaCl, second week: 2 mmol•L−1 NaCl, third week: 4 mmol•L−1 NaCl, fourth week: 8 mmol•L−1 NaCl and fifth week: 10 mmol•L−1 NaCl. For shock NaCl stress, treatment plants were subjected to 10 mmol•L−1 starting four weeks after planting when plants were well established. Final EC for the nutrient solution after NaCl application (gradual salinity and salt shock), reached 1.94 ds•m−1.Control treatments were not exposed to any salt stress (0.92 ds•m−1). All treatments were performed three times a week, and double distilled water was added once a week to all the pots to prevent salt deposition in the culture media. This strategy was chosen to reduce the effect of water deficit and to focus on the salt-specific impact of NaCl stress at the levels used in the experiment. Foliar application of both hormones was done once with ABA and JA, 24 hours after the first salt treatment. All treatments were sprayed with 0 or 40 µmol•L−1 ABA, respectively. To analyze the effect of different JA concentrations, all ABA+, as well as all ABA- treatments were sprayed with 0, 5, 10, 20 and 40 μmol•L−1 JA solutions, respectively. Control plants were sprayed with ethanol 5% and 0.05% Tween 20.
Sampling
Four weeks after the termination of gradual and shock NaCl stress treatments, at the first week of the flowering stage, while the plants were kept under salt stress, six leaves (two leaves from each of the young, average and old ones) were selected as samples from each replication. All samples were grind into flour by applying liquid nitrogen and dried under vacuum. Then the samples were powdered by a mill (Retsch-MM 400, Germany) and stored at room temperature until further analysis.
Leaf Relative Water Content, Electrolyte Leakage and Leaf Area
Leaf relative water content, electrolyte leakage and leaf area were measured four weeks after the beginning of the experiment. Three leaf samples were randomly selected in each plant and immersed in distilled water for 24 hours. Turgid leaves were weighed and after being dried in the oven in 80ºC, the leaf dry weight was calculated as follows:
In order to determine leaf electrolyte leakage, properly cleaned leaves were sliced to 1 cm2 and put into test tubes containing 10 ml deionized water and shaken on a shaker (80 rpm) for 24 hours. Electrolyte leakage was measured with EC meter (inLab-720 Germany) (EC1). The samples were kept in autoclave at 121ºC for 20 minutes, before electrolyte leakage was measured again (EC2). Leaf electrolyte leakage was calculated as EC1/EC2 percentage (Lutts et al., Citation1995). Leaf area was measured with digital pictures via Image J-1.46r software.
Endogenous ABA and JA Content
Four weeks after the inception of treatments, leaf samples were harvested and extracted with sodium 0.5 mg•L−1 ascorbate. The extraction procedure was carried out in 16 hours at 4ºC in darkness. The residual was filtered by Whatman #1 filter paper and methanol evaporation at 35ºC. Then, equal volume of 0.5 mol•L−1 phosphate buffer (8 gr NaCl, 0.2 gr KH2PO4, 9.6 grL−1 KH2PO4.H2O) was added to the residual. Next, liquid phase acidity was adjusted to 2.5. The residual water was removed, after a separation process with equal volume of ethyl acetate was carried out and the unbounded MBA in the solution was collected. Then, acidic phase in ethyl acetate evaporated, and the remnants of the material were immediately dissolved in 2 ml methanol. The samples were then filtered with 0.45 mm tetrafluoroethylene and injected to HPLC (High performance liquid chromatography, Unicam, Netherlands) equipped with Dimonsic-C18 (250*4.6 mm and 5 µm). Holding mobile phase, 0.1 N acetic acid and 80% methanol (50:50), ABA standard solution was prepared and chromatography was performed to determine the retention time. Finally the ABA content was expressed by nmol•g−1 DW.
In order to determine the endogenous content of JA, 2g of leaf tissue was immersed in a mixture containing 50 ml NaCl saturated solution, 2.5 ml of 1mol•L−1 acetic acid and 50 ml diethyl ether having 10 mg butylated hydroxyl toluene as an antioxidant. Ether phase was discontinued after 10 minutes in the centrifuge (2000 rpm). Residual dry materials were saluted in 5 ml of n-hexane and passed through silicagel column (60 Fluka, Steinheim, Germany). The samples were washed with 7 ml n-hexane/ether (v/v, 2:1) and dried with nitrogen evaporation system. Dried samples were saluted again in 50 ml n-hexane/ether (v/v 2:1), and 1 ml of the sample was injected to a gas chromatography equipped with flame ionization detector (Hewlett Packard 5890series, CA) and capillary tube (DB5MS, J & W Scientific, Folsom, CA, USA) with 0.33 µm film diameter, internal diameter of 0.3 mm and 50 m length. Injector temperature was adjusted to 250ºC and column temperature maintained 1 minute in 100ºC, then it was increased to 190ºC with a rate of 5ºC per minute. Afterward, it reached 200ºC and remained fixed for 2 minutes. Again, it was raised to 280ºC with a rate of 15ºC per minute and the final detector temperature reached 290ºC. Hydrogen was used as a carrier gas. Jasmonic acid was identified by retention time. Using internal standards, hormone content was calculated and expressed as ng•DW−1 (Fan et al., Citation1998; Kando et al., Citation2000).
Total Phenolic Content and Total Antioxidant Capacity Assay
Dried leaf tissues of ʻQueen Elisaʼ and ʻKurdistanʼ were homogenized in an extraction solution (70 mL of 80% methanol, 30 mL H2O and 0.1 mL of concentrated (36%) HCl) at a ratio of 1:5 and were transferred to 5 ml plastic tubes. After centrifugation at 13000 × g for 10 minutes at 4°C, supernatants were transferred to the new clean tubes.
The method used for the analysis of total phenolic content was Folin-Ciocalteu’s spectrophotometeric assay (Lamuela-Raventos, Citation2018). Calibration curve was created using different concentrations (0, 0.5, 1, 1.5 and 2 gallic acid eq g−1•FW) of standard gallic acid solutions. To analyze the samples, 50 μL of each sample were mixed with 475 μL of 0.2 mol•L−1 sodium carbonate solution, respectively and were incubated for 60 minutes at constant room temperature. The absorbance of 200 μL of standard solution, as well as that of the samples was obtained at 724 nm. Results were expressed on the basis of mM gallic acid eq g−1•FW. The total antioxidant capacity was determined following the ABTS (2,2-azino-bis-3-ethylbenzothiazoline-6-sulfonic acid diammonium salt) method (Arnao et al., Citation1999). This compound was chosen because the enzyme, horseradish peroxidase (HRP, Sigma Aldrich, EC 1.11.1.17) facilitates reaction with hydrogen peroxidase, turning it into a green end product (ABTS•+). The reaction was determined spectrophotometrically (Hewlett Packard Agilent 8453, Germany) at 730 nm for 5 minutes.
Standard curve for total antioxidant capacity: In the 1 mL cuvette, the reaction mixture consisted of 980 μL of ABTS-buffer solution, 1.55 μL H2O2, and 10 μL HRP until a stable absorbance was obtained due to ABTS radical formation. Afterward, per 10 μL of different concentrations (0.1–0.8 mmol•L−1) of ascorbic acid (AA) were added for a standard curve set up.
Spectrophotometeric analysis: Adding per 10 μL of methanolic extracts of plant tissue to the reaction mixture instead of ascorbic acid standards resulted in the absorbance decreasing as a consequence of ABTS radical depletion. Results were expressed as mM AA eq g−1•FW (Maksimovic and Zivanovic, Citation2012).
Total Soluble Proteins
To isolate fractions of soluble proteins, 0.25 g milled freeze-dried material of ʻQueen Elisaʼand ʻKurdistanʼ leaves was vortexed and homogenized using a shaker for 30 minutes (Edmund Buhler, KL-2, Germany) at 100 rpm, and centrifuged in 25 mL of 1 M NaCl solution. The absorbance was measured by spectrophotometer (Hewlett Packard Agilent 8453, Germany) at 595 nm. The protein concentration was determined using the BioRad protein assay with bovine serum albumin (BSA) as the standard (Biorad Serum Albumin) (Bradford, Citation1976).
Free Amino Acids
To measure free amino acids including aspartic acid, asparagine, alanine, tyrosine and GABA (γ-aminobutyric acid) in ʻQueen Elisa’ leaves, 125 mg of each freeze-dried sample was extracted using 2 mL of 0.1 M HCl. The samples were mixed by a shaker for 30 minutes (Edmund Buhler, KL-2, Germany) at 100 rpm, and were then centrifuged at 10000 × g for 20 minutes (Eppendorf, 5804 R, Germany). Next, the supernatants were transferred to 5 mL flasks, and 1.5 mL of 0.1 M HCL were added to the 2mL microtubes. This extraction procedure with HCl was repeated twice more. Afterward, the supernatants for each sample were collected, filled up to 50 mL by adding 0.1 M HCL and shaken at 80 rpm for 30 minutes. The extracts were then filtered by a cellulose membrane-filter (0.45 μm, Millipore, Merck, Germany).The separation of diluted derivatives of amino acids was carried out after the injection of 20 μL of each sample to the reversed-phase high-performance liquid chromatography (RP-HPLC). A pre-column (LichroCART 4–4 Purospher STAR RP-18 5 μm) and a separation column (LiChroCART 250–3 Purospher STAR RP-18 5 μm; Merck KGaA) were used for this purpose. 50 mmol•L−1 sodium acetate-buffer, pH 7.0 (solvent A) and methanol (solvent B) were used for HPLC separation by a linear gradient from a solvent ratio of 71/29 (v/v) at the beginning to 20/80 (v/v) over 25 minutes. Amino acids were detected by a florescence detector at wavelengths of Ex 330/Em 450 nm. The flow rate of 0.65 mL•min−1 at a temperature of 35°C was performed for the separation procedure. The detection range of the amino acids in our experiment was between 0.25 and 2.5 μM (Keutgen and Pawelzik, Citation2008a).
Statistics
The statistical analysis was carried out by multifactorial analysis of variance (ANOVA) with SAS 9.1.3 software. The mean values were calculated according to Duncan’s Multiple Range Test (P > .01).
Results
In the initial experiment, after exposing the samples to salt stress, a significant decrease in leaf relative water content and an increase in electrolyte leakage was observed. Leaf relative water content decrease was detected at both cultivars. Higher levels of NaCl showed a more significant reduction in ʻQueen Elisaʼ. Minimum leaf area was recorded at the highest level of NaCl in ʻKurdistanʼ (). Similarly, strawberry leaf area was reduced more noticeably in ʻElsantaʼ than in ʻKoronaʼ at salt stress conditions (Keutgen and Pawelzik, Citation2009). Electrolyte leakage in ʻQueen Elisaʼ at 5 and 10 mmol L−1 NaCl was significantly higher than that in ʻKurdistanʼ (). Electrolyte leakage could be a proper criterion to evaluate cell membrane disruption in plants exposed to salt stress. Maintaining cell membrane permeability at salinity conditions is the main result of stress resistance (Stevens et al., Citation2006). By increasing NaCl concentration, endogenous leaf ABA and JA showed an increase in both cultivars (). Similar results have been reported by Hamayun et al. (Citation2010), who exposed ABA content raised in soybean leaves to salt stress (Hamayun et al., Citation2010). Other researchers have found that ABA could remarkably improve water permeability and the ABA induced proteins protect plant cell from water deficit (Moons et al., Citation1997). One study found that endogenous JA level in salinity resistant cultivars of tomato showed an increase, and that a significant reduction in susceptible cultivars was observed after salt treatments (Kang et al., Citation2005). JA role in response to abiotic stress apparently depends on antioxidant route induction. Another study has found similar results with respect to ascorbate, glutathione, cysteine (Sasaki-sekimo et al., Citation2005). To sum up, it can be concluded from the review of literature that the higher levels of ABA and JA would be effective responses at salinity conditions.
Table 1. The effect of sodium chloride (mmol• L−1) on leaf relative water content (%), leaf electrolyte leakage (%) and leaf area (cm2) in two strawberry cultivars, ʻKurdistanʼ and ʻQueen Elisaʼ
Table 2. The effect of sodium chloride (mmol• L−1) on ABA (nm• g−1 FW) and JA (ng• g−1 FW) in two strawberry cultivars, ʻKurdistanʼ and ʻQueen Elisaʼ
In our experiment, JA supply at different concentrations contingent on NaCl stress gradient and ABA application showed distinct changes in some primary and secondary metabolites of strawberry leaves. The following paragraphs will describe such changes in detail.
Total Phenolic Content and Antioxidant Capacity
In ‘Kurdistan’ leaves, a significant increase in total phenolic content was recorded at both NaCl treatments in comparison with control, but there were no significant differences in total antioxidant capacity between NaCl stress regimes (gradual and shock NaCl stress) and control ( and ). In leaves of ʻQueen Elisaʼ, total phenolic content increased significantly, mostly at gradual NaCl stress compared with the control. The total antioxidant capacity showed a distinct increase at gradual NaCl stress compared with control ( and ). In ʻKurdistanʼ, total phenolic content was significantly increased at gradual NaCl stress under ABA treatment without JA application compared with control and shock NaCl stress conditions. Nonetheless, total antioxidant capacity significantly decreased at gradual and shock NaCl stress conditions compared with control at ABA treatments without JA ( and ). After treatment with ABA without JA, in ‘Queen Elisa’, total phenolic content showed a significant decrease at shock NaCl stress condition compared with control. Meanwhile, a significant decrease was also noticed at both NaCl treatments compared with control at ABA treatments without JA in this cultivar ( and ).
Figure 1. (a) Effect of different salt stress regimes and ABA application on total phenolic content in strawberry leaves of ʻKurdistanʼ. Vertical bars indicate standard errors of means. The mean data are resulted from three replicates per treatment and three plants per replicate. Different letters indicate statistically significant differences between mean values for – ABA and +ABA, according the Duncanʼs Multiple Range Test (p > .01). (b) Effect of different salt regimes on total phenolic content in strawberry leaves of ʻQueen Elisaʼ, with or without ABA. Vertical bars indicate standard errors of means. The mean data are resulted from three replicates per treatment and three plants per replicate. Different letters indicate statistically significant differences between mean values for – ABA and +ABA, according the Duncanʼs Multiple Range Test (p > .01)
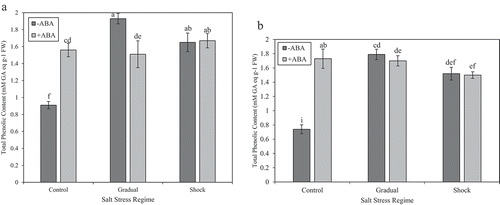
Figure 2. (a) Effect of different salt stress regimes and ABA application on total antioxidant capacity in strawberry leaves of ʻKurdistanʼ. Vertical bars indicate standard errors of means. The mean data are resulted from three replicates per treatment and three plants per replicate. Different letters indicate statistically significant differences between mean values for – ABA and +ABA, according the Duncanʼs Multiple Range Test (p > .01). (b) Effect of different salt stress regimes and ABA application on total antioxidant capacity in strawberry leaves of ʻQueen Elisaʼ. Vertical bars indicate standard errors of means. The mean data are resulted from three replicates per treatment and three plants per replicate. Different letters indicate statistically significant differences between mean values for – ABA and +ABA, according the Duncanʼs Multiple Range Test (p > .01)
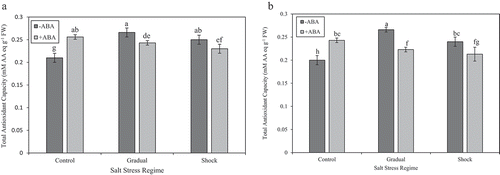
A significant increase in total phenolic content (7.1%) was recorded after the application of 5 µM JA in ‘Kurdistan’. Nonetheless with increased JA level, the rate decreased to 6.4% (). By adding JA in the presence of ABA, regardless of saline condition, total phenolic content in ‘Queen Elisa’ and the total antioxidant capacity in ‘Kurdistan’ and ‘Queen Elisa’ decreased 12.9% and 17.7%, respectively ( and ).
Total Soluble Protein Concentration
Both gradual and shock NaCl stress significantly decreased total soluble protein content compared with control in ‘Kurdistan’ strawberry leaves. In the presence of ABA, the total soluble proteins increased significantly in ʻKurdistanʼ and ʻQueen Elisaʼ leaves at both gradual NaCl stress and control treatment of about 12.22 ± 14 mg • g−1 DW and 12.61 ± 0.31 mg • g−1 DW, respectively ( and ). The application of the two hormones under non saline conditions, increased protein content in both cultivars significantly with a rate of 3.3% in ‘Kurdistan’ and 7.9% in ‘Queen Elisa’ respectively ().
Figure 5. (a) Effect of different salt stress regimes and ABA application on total soluble protein in strawberry leaves of ʻKurdistanʼ. Vertical bars indicate standard errors of means. The mean data are resulted from three replicates per treatment and three plants per replicate. Different letters indicate statistically significant differences between mean values for – ABA and +ABA, according the Duncanʼs Multiple Range Test (p > .01). (b) Effect of different salt stress regimes and ABA application on total soluble protein in strawberry leaves of ʻQueen Elisaʼ. Vertical bars indicate standard errors of means. The mean data are resulted from three replicates per treatment and three plants per replicate. Different letters indicate statistically significant differences between mean values for – ABA and +ABA, according the Duncanʼs Multiple Range Test (p > .01)
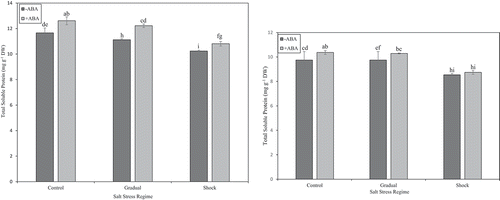
Free Amino Acids Concentration
NaCl shock significantly increased aspartic acid, histidine and asparagine content compared with control. A significant increase in tyrosine content was noticed at both NaCl treatments compared with control. No significant differences were found for alanine and GABA content ().
Table 3. The contribution of ABA (40 µ M) and JA (µ M) at control, gradual NaCl stress and shock NaCl stress on free amino acids content (mg•g−1 DW) of ʻQueen Elisaʼ strawberry leaves
After adding ABA without JA, aspartic acid and tyrosine content significantly increased at both NaCl stress regimes compared with control. Histidine and GABA showed a significant increase by adding ABA without JA at shock NaCl stress compared with gradual NaCl stress and non-saline conditions. Shock NaCl stress significantly increased alanine content compared with non-saline condition at ABA treatment ().
Aspartic acid was the most abundant amino acid in the leaves, followed by GABA, histidine and tyrosine. The highest amino acid concentrations, e.g. for asparagine and tyrosine, were mainly found at salt stress regimes after the application of 20 and 40 µM JA in the presence of ABA. Maximum aspartic acid concentrations of leaves treated with 10 and 20 μM JA plus ABA amounted with 14.70 ± 0.67 mg•g−1 DW and 15.92 ± 1.42 mg • g−1 DW, respectively. However, the highest alanine content of 2.97 ± 0.16 mg • g−1 DW was detected in leaves at salt shock regime treated with 40 μmol•L−1 JA plus ABA. The application of 10, 20 and 40 μM JA in the presence of ABA showed a significant increase in GABA compared with the same treatments without ABA. γ-aminobutyric acid concentrations were the highest at 40 μ mol•L−1 JA plus ABA under non-saline and salt stress regimes. Salt shock and ABA application caused an increase in histidine concentration. Nonetheless, the application of both hormones didn’t show any significant effect. The data of free amino acids concentration is mentioned just for ʻQueen Elisaʼ as the quality of the ʻKurdistanʼ extracts was not suitable for this purpose ().
Discussion
Involvement of total phenolic content and total antioxidant capacity in NaCl stress defense in the presence or absence of ABA/JA or by ABA application along with different JA concentrations
By increasing JA level in the presence of ABA, a decrease in total phenolic content and antioxidant capacity was recorded. It confirms that JA may not play an important role in inducing salt stress defense by an increase of total phenolic content and total antioxidant capacity. The following studies are in line with the results we reported above. In strawberry fruits, 40 mmol•L−1 NaCl stress resulted in a cultivar-specific higher antioxidant capacity (Keutgen and Pawelzik, Citation2007b). For cv. Elsanta, which is salt-sensitive, this increase was even more significant (14% and 23%) compared with cv. Korona. Furthermore, the mean initial level of phenolics in less sensitive cv. Korona was higher when compared with cv. Elsanta (Keutgen and Pawelzik, Citation2008b). An increase in total phenolics was also obtained in leaves of six Thai rice cultivars (Daiponmak et al., Citation2010). The various levels of salt stress have a different effect on the phenolic antioxidant system in plants. Salinity enhanced the secondary metabolism in strawberry, enabling roots and leaves to respond to salinity for defense requirements such as increase in antioxidant capacity (Neocleous et al., Citation2012). The higher phenolic level for the stressed plants might result from a higher overall amount of the metabolites. The amount of extra carbon and proportional allotment of available carbon partly depends on environmental conditions such as salt stress (Valifard et al. Citation2014). It is noteworthy that in our experiment, total phenolic content and total antioxidant capacity increased in the absence of ABA only after JA application in both cultivars. Hence, JA and ABA might induce a protective mechanism against salt stress by contributing to total phenolic content enhancement under different salt stress regimes. In addition, Jasmonic acid amplified the adverse effect of salt shock by increasing total phenolic content and total antioxidant capacity. Some researchers have come to similar conclusions: the increase in total phenolic content and antioxidant activity exhibits a protective mechanism against oxidative damages of the cellular structures caused by salinity (Valifardm et al., Citation2014).
Involvement of total soluble protein and free amino acids concentration in NaCl stress defense in the presence or absence of ABA/JA or by ABA application along with different JA concentrations
In our experiment, ABA treatment showed significant higher levels of total soluble proteins in both cultivars, but the relative increase of total soluble proteins was higher after applying both hormones in ʻQueen Elisaʼ (). Similar results have been reported in the literature: enhancing soluble proteins content was reported after the application of 0.5 mM JA in soybean leaves at saline conditions (Farhangi-Abriz and Ghassemi-Golezani, Citation2018). In contrast, treatment with JA reduced seed protein content in soybean plants. Lysine, methionine, phenylalanine, threonine, aspartic acid, glutamic acid, proline and tyrosine contents increased, whereas leucine and valine contents decreased with enhanced salinity (Farhangi-Abriz and Ghassemi-Golezani, Citation2016). In our experiment, by adding the hormones, highest concentrations of asparagines and tyrosine were observed (). Other researchers have reported similar results: Gulen et al. (Citation2006) observed a decrease in total soluble protein content in strawberry leaves of the cultivars ‘Tioga’ and ‘Chandler’ caused by salinity, while it was almost unchanged in ‘Camarosa’ . A higher content of soluble proteins has been found in salt tolerant cultivars of barley, sunflower and rice (Parvaiz and Satyawati, Citation2008). Also in our experiment, total soluble protein concentration decreased significantly under NaCl stress regimes in both cultivars. Other researchers have reported various results: Ashraf and Harris (Citation2004) reported that the proteins produced under salinity, cannot always be considered as an salt tolerance index, because it strongly depends on the natural habitat of the plant species or cultivar. The protein degradation in saline environments has been attributed to the decrease in protein synthesis, accelerated proteolysis, decreased availability of amino acids and denaturation of enzymes involved in the protein synthesis (Jaleel, et al., Citation2007; Lakhdar et al., Citation2008). One characteristic of NaCl stress is the removal of potassium ions from root cells, which causes a physiological imbalance as potassium is needed for protein synthesis (Chen et al., Citation2007). On the other hand, to survive under saline conditions, plants accumulate proteins that protect cells from salt stress effects (Wang et al., Citation2003). According to Keutgen and Pawelzik (Citation2008a), high concentration of NaCl around the root caused an increase in total and free amino acids in strawberry fruits. Asparagine and glycine accumulation were more obvious than the other free amino acids. Debouba et al. (Citation2010) found that high salinity levels induced an increase in total amino acids in leaves and roots of tomato. Furthermore, they found a relationship between serine and proline enhancement and GABA and asparagine accumulation at saline conditions. Kovacs et al. (Citation2012) reported that glutamine, glycine, aspartic acid, threonine, alanine and tyrosine rapidly increased three days after osmotic stress. Further increase was observed on the day seven. Under osmotic stress, transcription of the genes encoding the enzymes involved in arginine biosynthesis was activated and an increase in expression of the genes related to asparagine synthase was found; therefore, the role of arginine and asparagine in response to osmotic stress could be confirmed (Herrera-Rodriguez et al., Citation2007; Wang, et al., Citation2005). Under saline conditions, carbon flow will increase and may create a resource for the photosynthetic electron transfer, which may facilitate the production of carbon containing compounds such as osmolytes mostly found in the cytosol (Martino et al., Citation2003). In our experiment, aspartic acid, histidine, asparagine, and tyrosine increased under salt stress regimes (). This might be due to the production of more osmolytes under salt stress condition and the involvement of genes responsible for amino acid biosynthesis. Other researcher have come to various conclusions: γ-aminobutyric acid can serve as a stress indicator (Bouche and Fromm, Citation2004; Wang et al., Citation2014). Accumulation of GABA occurs in response to several environmental stresses as it is involved in osmotic and pH regulation (Akcay et al., Citation2012). A functional GABA shunt is important for stress tolerance (Wang et al., Citation2016). Environmental stress enhances the activity of enzymes involved in GABA metabolism (Krasensky and Jonak, Citation2012; Wang, et al., Citation2017). Having a prominent role in stress defense, GABA clearly increases. Probably because of acceleration in protein destructive metabolism, an increase in valine, tyrosine, serine, phenylalanine, glycine, glutamine and glutamic acid was also recorded (Kempa et al., Citation2008). In our study, in the absence of ABA, JA did not show a strong impact on total soluble protein content in ‘Kurdistan’. This shows that the two hormones are more effective when used together under gradual NaCl stress. Further, aspartic acid and GABA concentrations significantly increased after the application of JA in the absence of ABA at gradual NaCl stress. Significant increase in asparagine, alanine and tyrosine levels were observed after adding JA without ABA but under both NaCl stress regimes ().
To sum up, JA and ABA treatments at gradual salt regime resulted in aspartic acid increment and enhanced asparagine, alanine, histidine, and GABA content/concentration (). As reported in the literature the application of JA increased leaf calcium, magnesium and potassium content affected leaf water potential and photosynthetic rate positively (Kang et al., Citation2005).
Conclusion
After the application of both hormones, the total phenolic content and antioxidant capacity increased noticeably in both cultivars. It can be concluded that ABA might activate the non-enzymatic defensive mechanisms against salt stress in the plant cells. Jasmonic acid could effectively protect the strawberry plants from salt damage by enhancing total antioxidant capacity and phenolic compounds to quench the excessive reactive oxygen species caused by salt stress. Jasmonic acid amplified the adverse effect of salt shock by increasing total phenolic content and total antioxidant capacity. Generally, we were able to show how application of ABA along with different JA concentrations activates the protective mechanism of the strawberry plant against salt stress. By adding ABA, gradual salt stress treatment showed an obvious enhancement of total phenolic content and total antioxidant capacity in ʻKurdistanʼ. In ʻQueen Elisaʼ also at gradual stress, elimination of undesirable effects of salinity is facilitated. The fact that the application of the two hormones under study resulted in a decrease in total soluble proteins in ʻQueen Elisaʼ shows that possibly other metabolites are involved in determination of salt stress compatibility. Aspartic acid, asparagine, alanine, histidine and GABA were the amino acids that increased as a result of the interaction of JA and ABA at gradual salt stress in this cultivar. Certainly further studies are essential to compare the exact salt stress tolerance mechanisms for these cultivars.
References
- Akcay, N., M. Bor, T. Kerabudak, F. Ozdemir, and I. Turkan. 2012. Contribution of gamma amino butyric acid (GABA) to salt stress responses of Nicotinia sylvestris CMII mutant and wild type plants. J. Plant Physiol. 169(5):452–458. doi: 10.1016/j.jplph.2011.11.006.
- Arnao, M.B., A. Cano, and M. Acosta. 1999. Methods to measure the antioxidant activity in plant material. A comparative discussion. Free Radic. Res. 31:89–96. doi: 10.1080/10715769900301371.
- Ashraf, M., and P.J.C. Harris. 2004. Potential biochemical indicators of salinity tolerance in plants. Plant Sci. 166:3–16. doi: 10.1016/j.plantsci.2003.10.024.
- Bouche, N., and H. Fromm. 2004. GABA in plants: Just a metabolite? Trends. Plant Sci. 9:110–115. doi: 10.1016/j.tplants.2004.01.006.
- Bradford, M.M. 1976. A rapid and sensitive method for the quantification of microgram quantities of protein utilizing the principle of protein-dye binding. Anal. Biochem. 72:248–254. doi: 10.1016/0003-2697(76)90527-3.
- Chen, Z., T.A. Cuin, M. Zhou, A. Twomey, B.P. Naidu, and S. Shabala. 2007. Compatible solute accumulation and stress-mitigating effects in barley genotypes contrasting in their salt tolerance. J. Exp Bot. 58(15–16):4245–4255. doi: 10.1093/jxb/erm284.
- D’Anna, F.D., G. Incalcaterra, A. Moncad, and A. Miceli. 2003. Effects of different electrical conductivity levels on strawberry grown in soilless culture. Acta Hort. 609:355–360. doi: 10.17660/ActaHortic.2003.609.53.
- Daiponmak, W., P. Theerakulpisut, P. Thanonkao, A. Vanavichit, and P. Prathepa. 2010. Changes of anthocyanin cyanidine-3-glucoside content and antioxidant activity in Thai rice varieties under salinity stress. Sci. Asia. 286–291. doi: 10.2306/scienceasia1513-1874.2010.36.286.
- Debouba, M., A. Suzuki, S. Boutet, M.H. Ghorbel, and H. Gouia. 2010. Amino acid changes in leaves and roots of tomato (Solanumlycopersicon) during salt stress. Acta Bot. Gall. 157(2):255–264. doi: 10.1080/12538078.2010.10516203.
- Faghih, S., A. Zarei, and C. Ghabadi. 2018. Positive effects of plant growth regulators on physiology responses of Fragaria × ananassa cv. Camarosa under salt stress. Int J. Fruit Sci. doi: 10.1080/15538362.2018.146229.
- Fan, X., J.P. Mattheis, and J.K. Fellman. 1998. A role of jasmonates in climacteric fruit ripening. Planta 204:444–449. doi: 10.1007/s004250050278.
- Farhangi-Abriz, S., and K. Ghasemi-Golezani. 2016. Improving amino acid composition of soybean under salt stress by salicylic acid and jasmonic acid. J. Appl Bot. Food Qual. 89:243–248.
- Ghaderi, N., M.R. Hatami, A.A. Mozafari, and A. Siosemardeh. 2018. Physiol. Mol. Biol. (N.Y.). doi: 10.1007/s12298-018-0535-2.
- Gulen, H.E., E. Turhan, and A. Eris. 2006. Changes in peroxidase activities and soluble proteins in strawberry varieties under salt stress. Acta Physiol. Plant. 28(2):109–116. doi: 10.1007/s11738-006-0037-7.
- Hamayun, M., S. Afzal Khan, A. Latif Khan, Z.K. Shinwari, J. Hussain, E.-Y. Sohn, S.-M. Kang, Y.-H. Kim, M. Ajmal Khan, and I.-J. Lee. 2010. Effects of salt stress on growth attributes and endogenous growth hormones of soybean cultivar Hwangkeumkong. Pak J. Bot. 45(2):3103–3112.
- Hassanuzzaman, M., K. Nahar, and M. Fujita. 2013. Plant responses to salt stress and role of exogenous protectants to mitigate salt stress damages, p. 25–87. In: Ahmad P., Azooz M., Prasad M. (eds.), Ecophysiology and responses of plants under salt stress. Springer.
- Herrera-Rodriguez, M.B., R. Perez-Vicente, and M. Maldonado. 2007. Expression of asparagines synthetase genes in sunflower (Helianthus annus) under various environmental stresses. Plant Physiol. Biochem. 45(1):33–38. doi: 10.1016/j.plaphy.2006.12.002.
- Huang, T., and G. Jander. 2017. Abscisic acid-regulated protein degradation causes osmotic stress-induced accumulation of branched-chain amino acids in Arabidopsis thaliana. Planta 246(4):737–747. doi: 10.1007/s00425-017-2727-3.
- Ivanov, A.A. 2010. Effect of light conditions of wheat growing on sensitivity of photosynthetic machinery to salt stress. Russ J. Plant Physiol. 57(6):770–777. doi: 10.1134/S102144371006004X.
- Jaleel, C.A., P. Manivannan, G.M.A. Lakshmanan, R. Sridharan, and R. Panneerselvam. 2007. NaCl as a physiological modulator of proline metabolism and antioxidant potential in Phyllanthusamarus. C R: Biol 330:806–813. doi: 10.1016/j.crvi.2007.08.009.
- Kando, S., A. Tomiyama, and H. Seto. 2000. Changes of endogenous jasmonic acid and methyl-jasmonate in apples and sweet cherries during fruit development. J. Ameriacn Soc. Hortic. Sci. 125:282–287. doi: 10.21273/JASHS.125.3.282.
- Kang, D.J., Y.J. Seo, L.D. Lee, R. Ishii, K.U. Kim, D.H. Shin, S.K. Park, S.W. Jang, and I.J. Lee. 2005. Jasmonic acid differentially affects growth, ion uptake and abscisic acid concentration in salt-tolerant and salt-sensitive rice cultivars. J Agron. Crop Sci. 191:273–282. doi: 10.1111/j.1439-037X.2005.00153.x.
- Kaya, C., H. Kirnak, H. Higgs, and K. Saltali. 2002. Supplementary calcium enhances plant growth and fruit yield in strawberry cultivars grown at high (NaCl) salinity. Sci. Hort. 93:65–72. doi: 10.1016/S0304-4238(01)00313-2.
- Kempa, S., J. Krasensky, S. Dal Santo, J. Kopka, and C. Jonak. 2008. A central role of abscisic acid in stress regulated carbohydrate metabolism. PLoS ONE 3:e3935. doi: 10.1371/journal.pone.0003935.
- Keutgen, A., and E. Pawelzik. 2007a. Modification of taste relevant compounds in strawberry fruit under NaCl salinity. Food Chem 105:1487–1494. doi: 10.1016/j.foodchem.2007.05.033.
- Keutgen, A., and E. Pawelzik. 2007b. Modifications of strawberry fruit antioxidant pools and fruit quality under NaCl stress. J. Agr. Food Chem. 55(10):4066–4072. doi: 10.1021/jf070010k.
- Keutgen, A., and E. Pawelzik. 2008a. Contribution of amino acids to strawberry fruit quality and their relevance as stress indicators under NaCl salinity. Food Chem 111:642–647. doi: 10.1016/j.foodchem.2008.04.032.
- Keutgen, A., and E. Pawelzik. 2008b. Quality and nutritional value of strawberry fruit under long term salt stress. Food Chem 107:1413–1420. doi: 10.1016/j.foodchem.2007.09.071.
- Keutgen, A., and E. Pawelzik. 2009. Impacts of NaCl stress on plant growth and mineral nutrient assimilation in two strawberry cultivars of strawberry. Environ. Exp. Bot. 65:170–176. doi: 10.1016/j.envexpbot.2008.08.002.
- Kovacs, Z., L. Simon-Sarkadi, I. Vashegyi, and K. Gabor. 2012. Different accumulation of free amino acids during short-and long term osmotic stress in wheat. Sci. World J. doi: 10.1100.2012.216521.
- Krasensky, J., and C. Jonak. 2012. Drought, salt and temperature stress-induced metabolic rearrangements and regulatory networks. J. Exp. Bot. doi: 10.1093/jxb/err460.
- Lakhdar, A., C. Hafsi, M. Rahbi, A. Debez, F. Montemurro, C. Abdell, N. Jeddi, and Z. Oureghi. 2008. Application of municipal solid waste compost reduces the negative effects of saline water in (Hordeummaritimum L.). Bioresour. Technol. 99:7160–7167. doi: 10.1016/j.biortech.2007.12.071.
- Lamuela-Raventos, R.M. 2018. Folin-Ciocalteu method for the measurement of total phenolic content and antioxidant capacity: Recent trends and applications, p. 337. New York: John Wiley and Sons Ltd.
- Liu, X., Y. Yue, Y. Nie, Y. Nie, and W. Li. 2007. A G protein-coupled receptor for the plant hormone abscisic acid. Sci. 315:1712–1716. doi: 10.1126/science.1135882.
- Lutts, S., J.M. Kinet, and J. Bouharmont. 1995. Changes in plant response to NaCl during development of rice varieties differing in salinity resistance. J. Exp. Bot. 46:1843–1852. doi: 10.1093/jxb/46.12.1843.
- Maksimovic, J.J.D., and B.D. Zivanovic. 2012. Quantification of the antioxidant activity in salt-stressed tissues, p. 237–250. In: S. Shabala and T.A. Cuin eds.. Plant Salt Tolerance: Methods and Protocols. Methods in Molecular Biology. Vol. 913. Humana Press. Totowa. NJ.
- Manan, A., C.M. Ayyub, and R. Aslam Pervez, Ahmad. 2016. Methyl jasmonate brings about resistance against salinity stressed tomato plants by altering biochemical and physiological processes. Pak. J. Agri. Sci. 53(1):35–41.
- Martino, C.D., S. Delfine, R. Pizzuto, F. Loreto, and A. Fuggi. 2003. Free amino acids and glycine betaine in leaf osmoregulation of spinach responding to increasing salt stress. New Phytol. 158:455–463. doi: 10.1046/j.1469-8137.2003.00770.x.
- Moalemi, N., and S. Saadati. 2012. Effect of Zink foliar application on growth and yield of strawberry plant under saline condition.7th International Strawberry Symposium. 18–22 Feb.
- Moons, A., E. Prisen, G. Bauw, and M.V. Montagu. 1997. Antagonistic effects of abscisic acid and jasmonates on salt-inducible transcripts in rice roots. Plant Cell 92:243–259.
- Muhajan, S., and N. Tuteja. 2005. Cold, salinity and drought stresses: An overview. Arch Biochem. Biophys. 444:139–158. doi: 10.1016/j.abb.2005.10.018.
- Neocleous, D., V. Ziogas, and M. Vasilakakis. 2012. Antioxidant response of strawberry plants under stress conditions. Acta Hort.. doi: 10.17660/ActaHortic.2012.926.47.
- Ollas, C., V. Arbona, and A. Gomez-Cadenas. 2015. Jasmonic acid interacts with abscisic acid to regulate plant responses to water stress conditions. Plant Signal Behav 10(12):e1078953. doi: 10.1080/15592324.2015.1078953.
- Parida, A.K., and A.B. Das. 2005. Salt tolerance and salinity effects on plants: A review. Exotoxicol. Environ Saf. 60:324–349. doi: 10.1016/j.ecoenv.2004.06.010.
- Parvaiz, A., and S. Satyawat. 2008. Salt stress and phyto-biochemical responses of plants: A review. Plant Soil Environ. 54(3):89–99. doi: 10.17221/2774-PSE.
- Qiu, Z., J. Guo, A. Zhu, L. Zhang, and M. Zhang. 2014. Exogenous jasmonic acid can enhance tolerance of wheat seedlings to salt stress. Exotoxicol Environ. Saf. 104:202–208. doi: 10.1016/j.ecoenv.2014.03.014.
- Sasaki-Sekimoto, Y., N. Taki, T. Obayashi, M. Aono, F. Matsumoto, N. Sakurai, H. Suzuki, M.Y. Hirai, M. Noji, K. Saito, et al. 2005. Coordinated activation of metabolic pathways for antioxidants and defense compounds by jasmonates and their roles in stress tolerance in Arabidopsis. Plant J. 44:653–668. doi: 10.1111/j.1365-313X.2005.02560.x.
- Shahzad, A.N. 2011. The role of jasmonic acid (JA) and abscisic acid (ABA) in salt resistance of maize (Zea mays L.), Justus Liebig University Giessen.VVB LAUFERSWEILER VERLAG, Doctoral thesis, p. 96. Printed in Germany. ISBN: 978-3-8359-5829-6. Justus Liebig University Giessen.
- Stevens, J., T. Senaratna, and K. Sivasithamparam. 2006. Salicylic acid induces salinity tolerance in tomato (Lycopersicon esculentum cv. Ruma): Associated changes in gas exchange, water relations and membrane stabilization. Plant Growth Regul. 49:77–83.
- Tuteja, N. 2007. Abscisic acid and abitic stress signaling. Plant Signal Behav 2(3):135–138. doi: 10.4161/psb.2.3.4156.
- Valifardm, M., S. Mohsenzadeh, B. Kholdebarin, and V. Rowshan. 2014. Effects of salt stress on volatile compounds, total phenolic content and antioxidant activities of Salvia mizayanii. S Afr J. Bot. 93:92–97. doi: 10.1016/j.sajb.2014.04.002.
- Wang, W., B. Vinocur, and A. Altman. 2003. Plant responses to drought, salinity and extreme temperatures: Toward genetic engineering for stress tolerance. Planta 218(1):1–14. doi: 10.1007/s00425-003-1105-5.
- Wang, Y., Z. Luo, X. Huang, K. Yang, and S. Gao. 2014. Effect of exogenous γ-aminobutyric acid (GABA) treatment on chilling injury and antioxidant capacity in banana. Sci. Hort. 168:132–137. doi: 10.1016/j.scienta.2014.01.022.
- Wang, Y., Z. Luo, M. Linchun, and Y. Tiejin. 2016. Contribution of polyamines metabolism and GABA shunt to chilling tolerance induced by nitric oxide in cold-stored banana fruit. Food Chem 197:333–339. doi: 10.1016/j.foodchem.2015.10.118.
- Wang, D., L. Li, Y. Xu, J. Limwachiranon, D. Li, Z. Ban, and Z. Luo. 2017. Effect of exogenous nitro oxide on chilling tolerance, polyamine, proline and gamma-aminobutyric acid in bamboo shoots (Phyllostachys praecox f. prevernalis). J. Agr. Food Chem. 65:5607–5613. doi: 10.1021/acs.jafc.7b02091.
- Wang, H., D. Liu, J. Sun, and A. Zhang. 2005. Asparagine synthetase gene TaASN1 from wheat is upregulated by salt stress, osmotic stress and ABA. J. Plant Physiol. 162(1):81–89. doi: 10.1016/j.jplph.2004.07.006.
- Wasternack, C., and B. Hause. 2003. Jasmonates and octadecanoids: Signals in plant stress responses and development. Prog. Nucleic Acid Res. Mol Biol. 72:165–221.