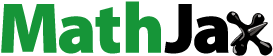
ABSTRACT
Dewatering of fruit juice through forward osmosis (FO) has attracted great attention in recent years; however, its application for fruit juice concentration through FO is limited due to high fouling rate, low flux, and expensive nature of membranes. Inorganic membranes are expensive due to heat-intensive steps and expensive raw materials that are involved in its synthesis, while geopolymerization technique for inorganic membrane synthesis reduced the cost due to its sintering-free steps and using fly ashes as a source material. In this research work, we report sintering-free economical and eco-friendly geopolymeric membrane in FO pilot-scale plant for orange juice concentration. However, the low flux of geopolymeric membrane was enhanced through coating of graphene oxide (GO) layer for high hydrophilicity. In this work, water flux through GO-based coated and uncoated geopolymeric membrane for concentrating sugar solution through FO was investigated. Prepared GO was coated on geopolymeric substrate through spin technique. The pore size of both geopolymeric substrate and GO was 1.8 and 5 µm, while porosity was 51.09% through the gravimetric method for both active and supportive layer. The orange juice solution was concentrated to 26.1 ± 0.5° and 21.87° Brix from 15.2 ± 0.5° Brix reaching flux of 0.405 and 0.360 kg.m−2.h−1 at a temperature of 30°C using sodium chloride salt solution (10 M) by coated and uncoated geopolymeric membranes, respectively. This study indicates that GO-based coated geopolymeric substrate is an eco-friendly and cost-effective feasible approach for fruit juice concentration through FO application.
Introduction
Membrane-based separation has emerged as a substitute for conventional juice clarification and concentration processes as they require less energy, reduced operating cost, and low temperature (Akther et al., Citation2015; Rastogi, Citation2016). The advantages of these membrane processes over conventional methods are lower thermal cracking to product, high changes in aroma retention, less operational consumption, and lower equipment capital costs (Alaswad et al., Citation2018; Chun et al., Citation2017). Fruit juice concentration through membrane not only provides microbiological stability but also saves cost in packaging and distribution of the finished product due to a reduction in bulk weight and volume (Rehman et al., Citation2019). Alongside their intrinsic characteristics of efficiency and operational simplicity, the high selectivity and permeability for the transport of specific components increased their importance in process industries. Furthermore membrane compatibilities in integrated systems, low energetic requirement, good stability under operating conditions and eco-friendly, easy wash-up and scale-up and large operational flexibility, membrane operations represent an interesting answer to all rationalized chemical productions (Naveed et al., Citation2017a). Similarly, membrane setup holds a great potential in fruit juice concentration. Juice concentration is one of the important processes during food manufacturing and processing. It is responsible for the dewatering of juice products, in order to reduce and ensure their preservation stability (Kim et al., Citation2019). However, the conventional techniques that have been used for concentrating juice include thermal processes, such as evaporation. These techniques demand energy and high-temperature process which evaporates important compositions of juice (Neilly, Citation2014).
Forward osmosis (FO) is an emerging membrane-based technology used for fruit juice concentration and desalination. FO-based semi-permeable membrane separation has attracted the attention of researchers and industrial development due to its technical advantages over conventional processes (Kim et al., Citation2017). In FO technology, the net water movement occurs from fruit juice (feed solution) of low concentration through a semipermeable membrane to high concentration (draw solution) under an osmotic pressure gradient. Furthermore, other classical techniques such as reverse osmosis (RO) cannot concentrate beyond 25% to 30% due to osmotic and hydraulic pressure limitations (Rehman et al., Citation2019). Concentration is a vital parameter which can directly affect the quality and taste of juice during the production process. Membrane-based separations such as ultrafiltration and FO have been widely applied in the field of juice processing (Garcia-Castello and McCutcheon, Citation2011; Kim et al., Citation2019). However, the low flux and separation performance limit the further development and caused high expenses (Neilly, Citation2014). Meanwhile, the commercial- and market-based membranes suffer serious biofouling after a long-time operation, which is still an operational issue that needs to be solved. For conventional membrane separation, the performance (including the flux and rejection) deeply depends on the thickness and porous structure of membrane. It is difficult to obtain a free-standing functional layer with high physical, chemical, and mechanical stabilities. The normal polymer substrates are flexible. With the repeated bending, some cracks will be inside the upper functional layer and result in failure. Ceramic membrane is a kind of well-developed inorganic membrane which shows high tremendous potential value on gas phase or liquid phase separation. As a stable substrate, ceramic membrane can well support and protect functional layer even at a quite low thickness. Ceramic membrane has a controllable porous structure (ordered and disordered) and pore size (micro-, meso- and macro-) to meet different application requirements, but the expensive raw material for synthesis and high sintering with calcination temperature makes the application of ceramic membrane limited (Naveed et al., Citation2017b). There is a need of sintering-free and low-cost membrane for economic commercial application. Naveed et al. (Citation2019) used geopolymeric membrane with pore size of 1.77 μm and compressive strength of 14.09 MPa with 51.09% porosity for household wastewater treatment through dead-end filtration.
Geopolymerization concept which was introduced by Davidovits in 1978 is an innovative technology of solid aluminosilicate oxide and high alkaline silicate solution (Davidovits et al., Citation2012). Zhang et al. (Citation2014) prepared self-supported zeolite membrane using geopolymerization technique for the separation of the mixture of alcohol/water. As the synthesis of membranes through geopolymerization does not need sintering heat and can use waste ashes as raw material, it has therefore attracted the focus of researchers working on inorganic membrane synthesis to use this technique for synthesis of membranes (Xu et al., Citation2013). Currently, Geopolymer membranes have been recognized for their potential, owing to their easy synthesis, low cost, free-sintering and alkali resistance, and high strength (Xu et al., Citation2019). Geopolymerization technique for inorganic membrane synthesis reduced the cost due to its sintering-free steps and fly ashes as source materials. Xu et al. (Citation2013) prepared a geopolymeric membrane for produced water treatment and recommended that geopolymerization technique for membrane synthesis is preferable due to its survival in harsh and corrosive environment (Xu et al., Citation2013). However, geopolymeric membranes have low flux which can be increased through hydrophilic group coating and enhancing porosity using foaming agents.
Graphene oxide (GO) has been introduced into filtration for liquid phase separation as active layer in recent years (Ma et al., Citation2017). The 2D nanosheets with relatively large size can stack together to form a membrane easily. The functional groups (carboxyl, hydroxyl, and epoxide) in oxygenated graphene nanosheets exhibit a great potential for the synthesis of nanocomposite materials coupled with strong hydrophilicity and chemical and structural stability (Shokrgozar Eslah et al., Citation2018). Moreover, it has high structural stability and mechanical strength because of the hydrogen bonding between GO nano-sheets (Algamdi et al., Citation2019). In other words, the surface oxygen-containing groups result in high hydrophilicity and enhanced the relative flux. The pores on nano-sheets can shorten the water transfer routes to improve the water flux without decline in rejection because of the controllable pore size which ranges from tens of nanometers to several micrometers (Qin et al., Citation2017; Shokrgozar Eslah et al., Citation2018). Graphene-based geopolymeric membrane can be the best choice for concentrating fruit juice through FO. Membranes used in FO are expensive, so we need sintering-free membrane with high hydrophilicity for high flux and better rejection. To the best of our knowledge in membrane-based separation, there is no geopolymeric membrane used in FO for fruit juice concentration. The basic novelty of this research work is filling the research gap of using geopolymeric membrane for fruit juice concentration through FO. However, using GO for enhancing hydrophilicity of geopolymeric membrane for high water flux in FO application is the prime objective of this research work.
In this research, we fabricated a pilot-scale plant using geopolymeric membrane with GO layer on both sides for fruit juice concentration through FO. Flux, rejection and concentration performance were analyzed for both of the prepared membranes. Surface and cross-sectional morphology of coated and uncoated membranes were observed through scanning electron microscopy (JSM5910, JEOL, Japan).
Materials and Methods
Preparation of Feed and Draw Solutions
In this research work, a novel kind of geopolymeric membrane with bi-interception layer of GO was developed for orange juice concentration through FO techniques. Orange juice was collected from local market with ingredient of 15° Brix as shown in . Sodium chloride coarse salt (NaCl) of 10 M was prepared using magnetic stirrer to stir the mixture for 30 min at 60 rpm in distilled water of 1 L. TDS and pH of distilled water was 0.003 and 7.02, respectively. Prepared draw solutions were purified through filter paper and then introduced into the system as draw solution.
Table 1. Ingredients of orange juice as feed solution
Synthesis of Flat Geopolymeric Membrane
X-ray fluorescence (Model: XRF-1800, Manufacturer: Shimadzu, Japan) and XRD (machine model: JDX3532, X-ray tube: Cu-Ka) analysis shows that fly ash of Lakhra power plant (Sindh, Pakistan) has an optimum ratio of silica and alumina (Si/Al = 2.80) and amorphous nature of mullite, respectively (Xu et al., Citation2013). The compressive strength, reaction time, and pore size are parameters that are dependent on the optimum ratio of silica and alumina and amorphous nature of fly ash. Fly ash of power plant was chemically activated by the high molar alkaline silicate of 15 M NaOH and Na2SiO3 solutions. Concentrated solution of 15 M was prepared in deionized water (98% purity), while the compositions of sodium silicate were found to be 15.8% of Na2O, 55.0%, SiO2 = 30.4% and 60.0% by mass. The ratios of chemical activators (Na2SiO3/NaOH = 2.5, 15 M) of sodium hydroxide and sodium silicate solutions were kept constant during the formation of geopolymeric paste. Sodium hydroxide and sodium silicate were purchased from international market [Nobel Chemical Limited, Pakistan] and were mixed for 30 min at 60 rpm. Dissolution occurs when fly ashes were mixed at a high speed of 120 rpm for 24 h at 25°C with the prepared alkali activators. Hydrogen peroxide as a foaming agent was added after the dissolution of silica and alumina in chemical activator in the volume ratio of 10% at 60 rpm to enhance the porosity of geopolymeric membrane. Poly-condensation of prepared foaming agent-based geopolymeric slurry was carried out in the rectangular mold of (Length: 51.8 mm, width: 72.7 mm, thickness: 2 um) at 60°C for 12 h as shown in .
Synthesis and Coating of GO
In the present study, a synthesized foaming agent-based geopolymeric membrane of 2 µm thickness was coated with bi-interception layer of GO. GO was prepared in the laboratory and characterized through modified Hummer’s method. In this technique, GO is prepared by oxidizing graphite without using NaNO3, which proved that H2SO4 and KMnO4 are capable of oxidizing graphite (Dikin et al., Citation2007). Dark black solution was obtained by mixing graphite powder of 1 g with 25 ml of sulfuric acid (H2SO4) for 2 h. Dark green solution was obtained when 3 g of potassium permanganate (KMnO4) was slowly added at 20ºC. The prepared solutions were oxidized through 100 ml of deionized water while hydrogen peroxide was added to stop the reaction. Prepared GOs were washed with hydrochloric acid (HCl) and deionized water solutions several times to remove the Mn2+ ions and other impurities. GO was dried at 90ºC for 24 h. Prepared GO was deposited through geopolymeric substrate by spin coating technique as shown in . Coated GO worked as active layer in enhancing hydrophilicity.
FO Step and Experimental Procedure
Experimental rig for FO system was constructed for orange juice concentration using geopolymeric membrane. In this system, a semi-permeable geopolymeric membrane was placed between orange juice of a low osmotic pressure and sodium chloride of high osmotic pressure and the driving force was osmotic pressure due to concentration differences. Peristaltic pumps (Category: Lab V, 0.00016–570 mL/min) were used for circulating of orange juice and draw solutions as shown in . The flow rate of 150 mL/min draw and orange juice solution was kept constant during the 7.5 h concentration processes.
Results and Discussion
XRD of Prepared GO
XRD analysis of prepared GO was done to analyze its fingerprints (Qin et al., Citation2015). From XRD pattern, it was found that prepared GO through Hummer method has the same characteristic with commercially purchased GO as shown in . The major peak in the graph shows the crystalline structure of both GO. Dikin et al. (Citation2007) found the same peaks during his preparation and characterization of GO sheets. Small peaks were observed due to minor impurities in GO as shown in . Prepared GO layer was coated on geopolymeric substrate which plays the role of selective barrier and enhancing hydrophilicity in membrane transportation.
Porosity and Surface Electron Microscope
Overall porosity (ε) of the geopolymeric membranes of active and supportive layer was determined by gravimetric method through EquationEquation (1)(1)
(1) using distilled water (Li et al., Citation2019).
ε is the porosity of coated geopolymeric membrane, m1 is the weight of wet membrane, m2 is the weight of dry membrane, A is the effective surface area of membrane, Dm is the cross-sectional thickness of membrane, and dw is the density of distilled water (0.98 g/cm3). Yu and Lencki (Citation2004) used the same methodology for finding the porosity of laminar MoS2 nanosheet for FO applications (Yu and Lencki, Citation2004). The porosity of both the membrane with coated GO layers and without coated geopolymeric substrate was observed to be 51.09% through gravimetric method.
Surface morphology of coated and uncoated prepared membranes was carried out with the help of SEM analysis to investigate pore size, pores density, and pinhole crack. The average pore diameter was measured from SEM analysis by plotting the standard scale on pore of membrane. Average was taken on a specific-selected area during pore size calculation. (a) shows the pore size of geopolymeric substrate which was measured through standard scale with magnification and was found to be 1.8 µm which can be used for fruit juice concentration through RO and has shown good results. Ur Rehman et al. (Citation2019) used the same pore size of membrane contactor for fruit juice concentration through osmotic distillation (Rehman et al., Citation2019). (b) shows that apparently there is no major pinhole crack in the membrane surface that causes reverse flux during FO application. Cracks on the membrane surface also caused the penetration of coating fluid inside the membrane which blocks the cross-sectional channels of geopolymeric membrane. Furthermore, it also shows that pore density is uniform throughout the geopolymeric membrane. (c) demonstrates the cross-sectional morphology of geopolymeric membrane which determines if the pore channels are open or block. Major and minor channels of different width clearly show that large particle size will effectively affect the inner membrane of geopolymeric substrate and water molecules can easily infiltrate into the pores. Similarly, (d) shows the thickness and asymmetric nature of the geopolymeric membrane. (a) shows the pores surface nature of GO which are open toward geopolymeric substrate. Furthermore, SEM analysis also shows a smooth uniform coating with surface thickness of 1 µm of porous GO. Similarly, (b) shows the size and structure of pore coated GO. It was found that average pore size of coated GO was 5 µm. In this study, the main objective of GO coating was to enhance the surface hydrophilicity of geopolymeric substrate.
Performance Evaluation
Membrane performance of coated and uncoated geopolymeric membranes was tested through experimental rig for FO as shown in . The water flux from orange juice to the draw solution across the membrane was analyzed using sodium hydroxide (10 M NaCl) as to draw solution and 15.2 ± 0.5° Brix of orange juice as feed solution in the decrease in weight of orange juice and increase in weight of orange juice for a particular period of time using EquationEquation 2(2)
(2) (Babu et al., Citation2008).
where J is the overall flux across the membrane from orange juice to the draw solution, Δm is mass (kg) of the permeated water, A is the overall membrane effective area (m2), and Δt is the period of operation (h). However, the reverse flux of draw solution to the orange juice was measured with the conductivity meter using EquationEquation 3(3)
(3) (Babu et al., Citation2008):
where Δc*V is the change in concentration (kg/m3) of draw solution with volume V (m3). shows the flux of both GO-based coated and uncoated geopolymeric membrane for 7.5 h without cleaning. It has been demonstrated that the value of flux increased from 0.205 to 0.405 kg.m−2.h−1 which can be attributed to the hydrophilic nature of coated GO layer on the geopolymeric substrate. The increase in the value of orange juice concentration from 15.2 ± 0.5° Brix to 21.87 ± 0.5° Brix and 26.1 ± 0.5° sBrix of uncoated and coated geopolymeric substrate is due to dewatering of water content while the decrease in the water flux is due to accumulation of organic component inside the juice on membrane surface. It was observed that GO-based geopolymeric membrane has high flux and better performance in FO applications. Furthermore, due to high hydrophilicity, graphene-based geopolymeric membrane has low fouling profile. The same finding was observed in thin-film nanocomposite (TFN) FO membrane, where water flux increases with increase in GO concentration from 0.05% to 0.1%. This can be attributed to the existence of functional groups on GO nanosheets and increase in membrane surface area (Shokrgozar Eslah et al., Citation2018). Nevertheless, the overloading of GO can lead to water flux reduction and therefore it is recommended to find the optimum load of GO (Shokrgozar Eslah et al., Citation2018). Another study examined the effect of thickness layer of geopolymer composite membrane on the performance of filtration process. It was found that the membrane resistance increases with increase in membrane dense layer from 150 to 600 μm, which led to decrease of water flux from 5.34 to 0.405 kg.m−2 h−1, respectively (Xu et al., Citation2019). The GO-based geopolymeric membrane and geopolymeric membrane exhibited a gradual decline in water flux with respect to process operating time, as shown in . Such behavior can be interpreted to be the effect of membrane fouling that occurred as a result of the concentrated feed solution (orange juice). The fouling layer formed on the surface of the layer facing feed solution hinders water permeation through the membrane and therefore lower water flux (Bhattacharjee et al., Citation2017). Membrane fouling can be reduced and the water flux of the membrane can be returned to the original state after applying back flushing method (Babu et al., Citation2008; Xu et al., Citation2019). Another valid reason is related to the effect of internal concentration polarization (ICP) that occurs within the layer that faces the DS (NaCl), which became gradually diluted throughout process duration (Bush et al., Citation2016; Cath et al., Citation2006). This occurs as a result of the decrease of osmotic pressure difference across the membrane, which is due to the continuous concentration of feed solution.
Conclusion
Successful synthesis of GO-based geopolymeric membrane for fruit juice concentration through FO was carried out through geopolymerization technique. Spin coating of GO and addition of foaming agents increased the water flux and porosity within a reasonable range. Addition of hydrogen peroxide as foaming agent in geopolymeric paste increases the porosity of prepared membrane after forced evaporation. Coated thin and dense layer of GO on geopolymeric membrane shows the hydrophilic nature of water, high retention of solute and enhanced the membrane transportation. Results show that GO enhanced water flux up to 1.053 kg.m−2.h−1 and increase orange juice concentration from 15.2 ± 0.5° Brix to 26.1 ± 0.5° Brix at a temperature of 30°C using sodium chloride salt solution (10 M). GO-based coated geopolymeric membrane is one of the cost-effective and promising technologies for the application of ; however, lower water flux in comparison to the conventional membrane remains a challenge.
Additional information
Funding
References
- Akther, N., A. Sodiq, A. Giwa, S. Daer, H.A. Arafat, and S.W. Hasan. 2015. Recent advancements in forward osmosis desalination: A review. Chem. Eng. J. 281:502–522. doi: 10.1016/j.cej.2015.05.080.
- Alaswad, S.O., S. Al-aibi, E. Alpay, and A.O. Sharif. 2018. Efficiency of organic draw solutions in a forward osmosis process using nano-filtration flat sheet membrane. J. Chem. Eng. Process Technol. 9(1):1–10. doi: 10.4172/2157-7048.1000370.
- Algamdi, M.S., I.H. Alsohaimi, J. Lawler, H.M. Ali, A.M. Aldawsari, and H.M.A. Hassan. 2019. Fabrication of graphene oxide incorporated polyethersulfone hybrid ultrafiltration membranes for humic acid removal. Sep. Purif. Technol. 223:17–23. doi: 10.1016/j.seppur.2019.04.057.
- Babu, B.R., N. Rastogi, and K. Raghavarao. 2008. Concentration and temperature polarization effects during osmotic membrane distillation. J Membrane Sci. 322:146–153. doi: 10.1016/j.memsci.2008.05.041.
- Bhattacharjee, C., V. Saxena, and S. Dutta. 2017. Fruit juice processing using membrane technology: A review. Innov. Food Sci. Emerg. Technol. 43:136–153. doi: 10.1016/j.ifset.2017.08.002.
- Bush, J.A., J. Vanneste, and T.Y. Cath. 2016. Membrane distillation for concentration of hypersaline brines from the Great Salt Lake: Effects of scaling and fouling on performance, efficiency, and salt rejection. Sep Purif Technol. 170:78–91. doi: 10.1016/j.seppur.2016.06.028.
- Cath, T.Y., A.E. Childress, and M. Elimelech. 2006. Forward osmosis: Principles,applications, and recent developments. J Membrane Sci 281:70–87.
- Chun, Y., D. Mulcahy, L. Zou, and I.S. Kim. 2017. A short review of membrane fouling in forward osmosis processes. Membranes 7(2):1–23. doi: 10.3390/membranes7020030.
- Davidovits, J., R. Davidovits, and M. Davidovits, Geopolymeric cement based on fly ash and harmless to use, US 8,202,362 B2 Patents, 2012.
- Dikin, D.A., S. Stankovich, E.J. Zimney, R.D. Piner, G.H. Dommett, G. Evmenenko, S.T. Nguyen, and R.S. Ruoff. 2007. Preparation and characterization of graphene oxide paper. Letter 448:457.
- Garcia-Castello, E.M., and J.R. McCutcheon. 2011. Dewatering press liquor derived from orange production by forward osmosis. J. Membr. Sci. 372(1–2):97–101. doi: 10.1016/j.memsci.2011.01.048.
- Kim, B., G. Gwak, and S. Hong. 2017. Review on methodology for determining forward osmosis (FO) membrane characteristics: Water permeability (A), solute permeability (B), and structural parameter (S). Desalination. 422:5–16. doi: 10.1016/j.desal.2017.08.006.
- Kim, D.I., G. Gwak, M. Zhan, and S. Hong. 2019. Sustainable dewatering of grapefruit juice through forward osmosis: Improving membrane performance, fouling control, and product quality. J. Membr. Sci. 578:53–60. doi: 10.1016/j.memsci.2019.02.031.
- Li, Y., I. Nulens, R. Verbeke, H. Mariën, T. Koschine, M. Dickmann, W. Egger, and I. Vankelecom. 2019. Tuning the porosity of asymmetric membranes via simple post-synthesis solvent-treatment for non-aqueous applications. Sep. Purif. Technol. 217:147–153. doi: 10.1016/j.seppur.2019.02.012.
- Ma, J., D. Ping, and X. Dong. 2017. Recent developments of graphene oxide-based membranes: A review. Membrane 7:52.
- Naveed, A., S. Gul, N.U. Aminb, M. Younasa, and N. Ullaha. 2017a. Synthesis and characterization of inorganic microfiltration membrane through geopolymerization. Desalination Water Treat. 66:203–209. doi: 10.5004/dwt.2017.20212.
- Naveed, A., F. Saeed, and S. Hassan. 2017b. Geopolymerization: A green & sustainable approach for inorganic membrane synthesis, ICSP-Proceedings, 42. Lahore, Pakistan.
- Naveed, A., F. Saeed, M. Khraisheh, M. Al Bakri, and S. Gul. 2019. Porosity control of self-supported geopolymeric membrane through hydrogen peroxide and starch additives. Desalination Water Treat. 152:11–15. doi: 10.5004/dwt.2019.23895.
- Neilly, A.G. 2014. Forward osmosis: The relationships between draw solution, membrane orientation and water flux. James Cook University, USA.
- Qin, W., K. Guan, B. Lei, Y. Liu, C. Peng, and J. Wu. 2015. One-step coating and characterization of α-Al2O3 microfiltration membrane. J. Membr. Sci. 490:160–168. doi: 10.1016/j.memsci.2015.05.003.
- Qin, Z., L. Wang, W. Zhang, and K. Pan. 2017. A novel surface cross-linked GO-based membrane with superior separation performance. RSC Adv 7(85):54213–54221. doi: 10.1039/C7RA11088J.
- Rastogi, N.K. 2016. Nutrition, opportunities and challenges in application of forward osmosis in food processing. Crit. Rev. Food Sci.Nutr. 56:266–291. doi: 10.1080/10408398.2012.724734.
- Rehman, W.U., A. Muhammad, Q.A. Khan, M. Younas, and M. Rezakazemi. 2019. Pomegranate juice concentration using osmotic distillation with membrane contactor. Sep. Purif. Technol. 7:32.
- Shokrgozar Eslah, S., S. Shokrollahzadeh, O. Moini Jazani, and A. Samimi. 2018. Technology, forward osmosis water desalination: Fabrication of graphene oxide-polyamide/polysulfone thin-film nanocomposite membrane with high water flux and low reverse salt diffusion. Sep. Sci.Technol 53(3):573–583. doi: 10.1080/01496395.2017.1398261.
- Xu, M.-X., Y. He, Z.-H. Liu, Z.-F. Tong, and X.-M. Cui. 2019. Preparation of geopolymer inorganic membrane and purification of pulp-paper making green liquor. Appl. Clay Sci. 168:269–275. doi: 10.1016/j.clay.2018.11.024.
- Xu, M.-X., Y. He, C.-Q. Wang, X.-F. He, X.-Q. He, J. Liu, and X.-M. Cui. 2013. Preparation and characterization of a self-supporting inorganic membrane based on metakaolin-based geopolymers. J. Membr. Sci. 447:66–72. doi: 10.1016/j.memsci.2013.07.027.
- Yu, J., and R. Lencki. 2004. Effect of enzyme treatments on the fouling behavior of apple juice during microfiltration. J. Food Eng. 63(4):413–423. doi: 10.1016/j.jfoodeng.2003.08.013.
- Zhang, J., Y. He, Y.-P. Wang, J. Mao, and X.-M. Cui. 2014. Synthesis of a self-supporting faujasite zeolite membrane using geopolymer gel for separation of alcohol/water mixture. Mater. Lett. 116:167–170. doi: 10.1016/j.matlet.2013.11.008.