ABSTRACT
Secondary somatic embryogenesis was observed in the suspension culture of bract-derived banana calli in the presence of liquid Murashige and Skoog medium with additives such as glutamine (0.68–34.21 µM), biotin (0.40–20.46 µM), or ascorbic acid (0.56–28.38 µM) along with malt extract (100 mg/l). After the initiation of homogenous cell suspension, fully developed somatic embryos were observed after fifth subculture in the four cultivars. Somatic embryos were successfully regenerated in basal MS medium and were transplanted after fifth week. After fifth subculture, the frequency of somatic embryos increased in each culture flask. Maximum number of somatic embryos were observed in cv. Sannachenkadali (46.12 ± 0.85a) when cultured in liquid MS medium supplemented with biotin (8.18 µM) along with malt extract (100 mg/l), in followed by cv. Matti (44.75 ± 1.19a) having (AA) genome. SEM analysis revealed the presence of secondary somatic embryos on the surface of primary somatic embryos in each culture and it attributed a high frequency of somatic embryogenesis. The addition of biotin along with malt extract increased the frequency of secondary somatic embryogenesis in the diploid banana cultivars.
Introduction
Banana is one of the most important tropical fruits in the world trade. India has been the largest producer of banana worldwide since the past one decade (FAO, Citation2010). It is largely cultivated in India accounting for about 33.4% of the total fruit production. India ranks first both in terms of area and production of banana in the world contributing around 15% of the total global area under banana and about 29% of the total world’s production (Nayak and Singh, Citation2018). In India, banana is the second most important fruit crop and has dominated the fruit industry contributing the highest GDP of 1.99% to agricultural sector. Though India is a leading country in global banana production, the total production is largely consumed domestically and provides only a negligible share in the global trade. In Kerala, the cultivation for local consumption is based on a large number of cultivars adapted to domestic cultivation as well as the varied use and taste of local consumers (Aravindakshan and Pushkaran, Citation1996). In Kerala cv. viz., Njalipooovan, Matti, Sannachenkadali, Palayamcodan, and Nendran are widely cultivated. Most of the edible bananas are sterile polyploids and must be propagated vegetatively. The materials used for conventional propagation include corms, large and small suckers, and sword suckers and are not the ideal propagule, because they carry weevils, fungal pathogens, nematodes, and viruses (Arias, Citation1992) and also suffer from slow multiplication, bulkiness, and poor phytosanitary quality and genetic improvement of this plant through cross breeding is a difficult task. In vitro culture of banana has been extensively used to quickly propagate vegetative clones of many genotypes. Somatic embryogenesis, the process whereby a single somatic cell developed into somatic embryos is widely applicable in case of banana.
Somatic embryogenesis and plant regeneration in suspension culture of dessert banana (AA and AAA) and cooking (ABB) bananas (Musa sp.) were reported by Novak et al. (Citation1989). Grapin et al. (Citation2000) established embryogenic callus initiation and regeneration of embryogenic cell suspensions from female and male immature flowers of Musa (AAB). Cote et al. (Citation2000) studied the field performance of embryogenic cell suspension – derived banana plants (Musa AAA, cv. Grand Naine) and reported two types of variants with ‘variegated or deformed leaves’ and ‘fascinated-leafed plants.’ Regeneration of banana (Musa spp. AAB cv. Dwarf Brazilian) via secondary somatic embryogenesis was reported by Khalil et al. (Citation2002) In their study primary somatic embryos were produced from explants of immature male flower buds and morphologically normal plants developed from all of the regenerated plantlets. Kosky et al. (Citation2002) initiated cell suspensions of the hybrid cultivar FHIA-18 (AAAB) which were established from sections of embryogenic tissue derived from male flowers. Xu et al. (Citation2005) established embryogenic cell suspensions and plant regeneration of the dessert banana ‘Williams’ (Musa AAA, group). Morias - Lino et al. (Citation2008) reported cell suspension culture and plant regeneration of a Brazilian plantain, cultivar ‘Terra’ from immature male flowers, and secondary somatic embryos were reported from the suspension culture. Molecular genetics studies have also shown that ectopic expression of a specific embryo – and meristem-expressed transcription factors or loss of certain chromatin-modifying proteins induces spontaneous somatic embryogenesis (Horstman et al., Citation2017). The literature review revealed that there are many reports on somatic embryogenesis in triploid bananas since most of the commercial cultivation belongs to triploid cultivars; however, in this study, we describe an efficient and reproducible protocol with high-frequency regeneration of secondary somatic embryogenesis in four diploid banana cultivars viz, Musa acuminata cv. Matti (AA), Sannachenkadali (AA), Chingan (AB), and Njalipoovan (AB). These cultivars are common in Kerala under domestic cultivation and may have the potential for nonconventional banana breeding programs.
Materials and Methods
Male flower bunches obtained from adult field – grown banana maintained at garden, Department of Botany, University of Kerala, Kariavattom. Male flowers of four cultivars were collected at the end of the emergence of the last batch of fruits after sixth week. The bracts with associated hands of male flowers were removed in a step-wise manner until they become too small to be removed by hand. The remaining portion having an approximate size of 4–5 cm length was immersed in 1% (v/v) Labolene® for 6 min and kept under running tap water for 30 min. The explants were surface sterilized in 0.1% (w/v) mercuric chloride for 4 min followed by three rinses in autoclaved double-distilled water, 5 min for each rinse. Two or three outer protective bracts and corresponding groups of male flowers were sequentially discarded. Here, ~1 cm square pieces from the basal region of inner bracts were excised, removed the male flowers with a single cut and the bract was inoculated on Murashige and Skoog medium supplemented with Thidiazuron (0.045–9.00 µM), 30 gl−l sucrose, 0.7 gl−l agar and the pH of the media was adjusted to 5.8 before autoclaving at 120°C for 18 min. The cultures were maintained at a temperature of 25 ± 2°C with a photoperiod of 16 h/day under 50 µmol m−2s−1 light intensity provided by fluorescent lamps. Primary explants were transferred to fresh media every 3 weeks. The darkened tissue was removed and the remaining tissue was transferred to fresh medium containing the same hormonal concentrations. After sixth week, embryogenic calli were initiated and cell suspension cultures were initiated by placing 100 mg fresh weight of embryogenic callus into 20 ml of MS liquid medium with additives such as glutamine (0.68–34.21 µM), biotin (0.40–20.46 µM) or ascorbic acid (0.56–28.38 µM) along with malt extract (100 mg/l) and were dispensed in 100 ml Erlenmeyer flasks. The cultures were maintained at 80 rpm. Subculturing was done at the end of every third week. The cultures were maintained at 25 ± 2°C temperature with a photoperiod of 16 h/day under 50 µM m−2 s−1 light intensity provided by fluorescent lamps. Fully developed embryos were isolated by sieving through a stainless steel filter (450 µM) and placed in liquid MS basal or half basal medium for germination. Germinated somatic embryos were transferred to the MS basal or half basal medium for rooting. Rooted plantlets were transferred to greenhouse condition for 2 weeks for acclimatization and were successfully transferred to field conditions. Secondary somatic embryos were more frequently observed after fifth subculture. The presence of secondary somatic embryos was studied under scanning electron microscope (SEM; JEOL JSM). Samples were dehydrated in ethanol series (30–100% v/v) and dehydrated tissues were then critical point dried, mounted on metal blocks, and sputter coated with gold (JOEL JFC 1200 Fine coater). The gold coated samples were observed and photographed under SEM operating at 10 kv.
Statistical Analysis
Statistical analysis was performed with the software SPSS/PC Version 4.0 (SPSS Inc., Chicago, USA). Mean and SE were calculated and differences between means were tested using Duncan’s Multiple Range Test at the level of P < .05.
Results
Friable embryogenic calli initiated from bract explants inoculated on MS medium supplemented with TDZ (4.5 µM) were selected for the initiation of embryogenic cell suspension (). MS medium supplemented with (0. 45 µM) TDZ produced off-white friable calli while (3.6 µM) and (9.00 µM) TDZ initiated yellow compact calli. Influence of TDZ on callus induction from bract explants of diploid banana cultivars and frequency of somatic embryo formation after second subculture were summarized in . After synchronization of suspension cultures by filtration over the course of more than 4 months, stable and homogenous embryogenic cell suspension cultures were established. Round or oval-shaped viable single cells were observed during the initial stages of cell suspension (). Embryogenic cells and embryos of different stages were also observed during the period of the first subculture (). After 4–5 months, embryogenic cell suspension reached the phase of synchronized growth in the medium supplemented with additives. Further subculture in the same hormonal concentration enhanced the development of heterogeneous embryo culture with various developmental stages (). Cell suspensions were sieved and washed thoroughly and plated on petri plate containing basal MS medium and were incubated in dark for 1 week. Somatic embryos were germinated within 2–3 weeks and basal MS medium showed better growth of somatic embryos in all four cultivars. Synchronous development of root and shoot observed in basal medium. Full strength MS basal medium found to be effective for cv. Matti and cv. Sannachenkadali and was germinated after 2 weeks while somatic embryos of cv. Chingan and cv. Njalipoovan germinated after third week. Then, the cultures were transferred to a 16-h photoperiod in the culture room and green shoots were developed (). Globular embryos were transferred individually or in small groups to 100 ml Erlenmeyer flask containing basal MS medium or one-half basal MS medium also showed shoot regeneration. In suspension culture development of clusters of somatic embryos from the existing embryos was frequently observed after fifth subculture. For SEM analysis, secondary somatic embryos were observed on the surface of primary embryos. Frequency of somatic embryos bearing secondary somatic embryos after fifth subculture was summarized in . The maximum frequency of secondary somatic embryos was observed in the presence of 8.18 µM biotin along with malt extract (100 mg/l) in cv. Matti (44.75 ± 1.19a) and cv. Sannachenkadali (46.12 ± 0.85a). While 16.37 µM biotin along with malt extract (100 mg/l) results in maximum frequency of secondary somatic embryos in cv. Chingan (34.62 ± 0.77a) and cv. Njalipoovan (33.50 ± 0.96a) Glutamine (6.84 µM) along with malt extract (100 mg/l) produced a maximum number of secondary somatic embryos from cv. Sannachenkadali (38.12 ± 0.63a) while ascorbic acid (11.35 µM) along with malt extract (100 mg/l) produced a maximum number of somatic embryos in cv. Matti (27.00 ± 0.65a). Secondary somatic embryos typically entered the globular stage directly from primary somatic embryos and occurred in clusters, initially appearing as small protuberances on the surface of primary somatic embryos (). SEM analysis revealed the initial development of somatic embryos and clusters of secondary embryo, on higher magnification tertiary somatic embryos were also observed. In the clusters of somatic embryos, several developmental stages were observed, revealing non-synchronized development and the somatic embryos had a broad basal area fused to the maternal tissue. Once somatic embryogenesis was triggered, continual development of somatic embryos was again observed, resulting in the development of clusters of secondary and tertiary somatic embryos.
Table 1. Influence of TDZ on embryogenic induction from bract explants of diploid banana cultivars
Table 2. Influence of additives with malt extract on the production of secondary somatic embryos in diploid Musa acuminata cultivars
Figure 1. (a) Calli forming from male immature banana flowers. (b) Round or oval-shaped viable single cells observed during the initial stages of cell suspension. (c) Formation of somatic embryos at various stages of development. (d) Culture in liquid medium containing globular somatic embryos. € Green shoot development from the embryo. (f) Secondary somatic embryos appearing as small protuberances on the surface of primary somatic embryos
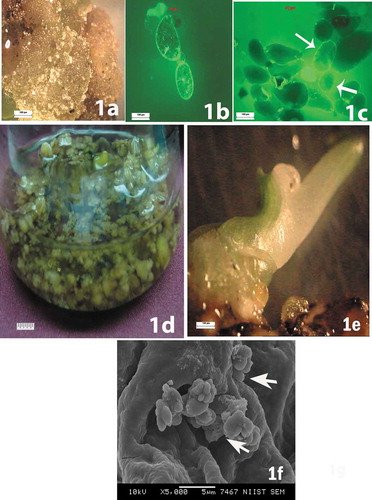
Discussion
The phenomenon of somatic embryogenesis was exploited to regenerate whole plants of agronomically and horticulturally important species, since the discovery of this process for the first time by Steward et al. (Citation1958) in callus cultures of carrot. Horticulture industry realized the utility of this process for studying early events regulating embryogenesis, in large-scale production of propagules, respectively, with low labor inputs by combining somatic embryogenesis with mechanized automated culture systems. Embryogenic culture system with reliable regeneration efficiency from important varieties of banana is a prerequisite for realizing the potential of cellular and molecular tools of crop improvement. Toward this goal, studies were made to develop protocols for somatic embryogenesis and plant regeneration from different banana cultivars. In banana, the limited choice of explants, lengthy preparation phase, low and variable embryogenic response have been reported from various studies of somatic embryogenesis (Khalil, Citation2002). The capacity for totipotent growth reaches its maximum potential during in vitro tissue culture, where an even wider range of explants can be induced to undergo embryogenesis, including haploid cells of the male and female gametophyte (Soriano et al., Citation2013) and vegetative cells of the sporophyte (Elhiti et al., Citation2013).
In the present study friable embryogenic calli obtained from bract explants was the potential source for secondary embryogenesis. Grapin et al. (Citation2000) reported that the embryogenic and regenerative capacity of suspension cultures of banana cultivars Currare and Currare Enano retained for 18 months. The cell suspensions with high regeneration capacity were commonly used for mass clonal propagation and these cells were also the only source to regenerate plant materials for inducing mutations and genetic engineering (Strosse et al., Citation2006). In situ gene expression analysis resolved the contradictory relationship between expression of developmental marker genes and cell fate in different culture systems (Miguel and Marum, Citation2011) and provided a more exact description of when and how embryogenic cells are formed in culture (Soriano et al., Citation2014). In the present investigation, secondary somatic embryos were observed during SEM studies of embryogenic callus. A much higher efficiency of secondary somatic embryos over primary somatic embryos has been reported in many plant species (Raemakers et al., Citation1995). Khalil et al. (Citation2002) reported regeneration of banana via secondary somatic embryogenesis in Musa spp. AAB cv. Dwarf Brazilian. In their reports primary somatic embryos were produced when explants of immature male flower buds were cultured on Murashige and Skoog medium plus 1 mg/l biotin, 100 mg/l malt extract, 100 mg/l glutamine, 4 mg/l 2,4-dichlorophenoxyacetic acid, 1 mg/l indole-3-acetic acid, 1 mg/l α-naphthaleneacetic acid, 30 g/l sucrose, and 2.6 g/l phytagel and then transferred to m1 medium plus 200 mg/l casein hydrolyzate and 2 mg/l proline. Subsequent transfer to MS supplemented with 10% coconut water produced rapidly proliferating embryogenic callus that developed into secondary somatic embryos. Some cultures were able to retain their competence for secondary somatic embryogenesis for many years and thus provide useful material for various studies, as described for Vitis ruperis (Martinelli et al., Citation2001). Secondary embryo development frequently occurred in embryogenic cultures at the late stages of embryogenesis. Possibly somatic embryos that failed to convert into plantlets were inclined to produce secondary embryos (Ammirato, Citation1987). The occurrence of secondary somatic embryos in primary culture condition was observed in many other monocot species including Hemerocallis sp, Dactylis glomerata, Oryza sativa, Panicum maximum, Pennisetum americanum, Triticum aestivum, and Zea mays (Martinelli et al., Citation2001; Raemakers et al., Citation1995).
Somatic embryos are used for studying the regulation of embryo development, but also as a tool for large-scale vegetative propagation. In some cases, somatic embryogenesis is favored over other methods of vegetative propagation because of the possibility to scale up the propagation by using bioreactors (Zhong et al., Citation2017). The embryogenic-callus-induced rate of the immature male florescence cultured on the media containing the recombinant protein Arg9-NLSWIND1 was significantly higher than that of the immature male florescence cultured on the control media. (Chang et al., Citation2018). Genetic analysis showed that AIL proteins interact with auxin pathways throughout plant development (Horstman et al., Citation2014) and BBM (BABY BOOM) binds to auxin biosynthesis genes (TAA1, YUC3, and YUC8) in somatic embryo tissue (Horstman et al., Citation2018). SE-inducing AIL gene has been investigated in the Arabidopsis (Su et al., Citation2015). Differential expression pattern of SE related genes in different developmental stages of cell suspensions (ECS and NECS) suggested that MaPIN1 played a crucial role in the initiation and maintenance of embryogenic capacity of ECS in banana (Shivani et al., Citation2018). In the present study, the biotin requirement for somatic embryogenesis was different with respect to their genomes. The maximum frequency of secondary somatic embryos was observed in the presence of 8.18 µM biotin along with malt extract in cv. Matti (AA) and cv. Sannachenkadali (AA), while 16.37 µM biotin along with malt extract (100 mg/l) produced a maximum frequency of secondary somatic embryos in cv. Chingan (AB) and cv. Njalipoovan (AB). This indicates the potential of these cultivars for further studies in genome analysis and the protocol developed in the present study may be used for plant propagation and genetic transformation studies.
As somatic embryos arise from single cells seems to produce fewer rates of somaclonal variations and due to the cellular totipotency somatic cells under appropriate conditions are able to develop a complete functional embryo. When integrated with conventional breeding programs and molecular and cell biological techniques, somatic embryogenesis provides a valuable tool to enhance the pace of genetic improvement of commercial crop species.
References
- Ammirato, P.V. 1987. Organizational events during somatic embryogenesis, p. 57–81. In: C.E. Green, D.A. Somers, W.P. Hackett, and D.D. Biesboer (eds.). Plant tissue and cell culture. Alan R, Liss, New York.
- Aravindakshan, M., and K. Pushkaran. 1996. Banana compendium, p. 1–8. Kerala Agriculture University, Thrissur, Kerala, India.
- Arias, O. 1992. Commercial micropropagation of banana, p. 139–142. In: Biotechnology applications for banana and plantain improvement. INIBAP, San Jose, Costa Rica.
- Chang, S., Z. Wu, W. Sun, F. Lin, Q. Zeng, J. Zhang, L. Qiao, and H. Shu. 2018. A method to improve the embryogenesis rate of banana somatic cell embryogenesis. Am. J. Plant Sci. 9:531–541.
- Cote, F.X., M. Folliot, R. Domergue, and C. Dubois. 2000. Field performance of embryogenic cell suspension derived banana plants (Musa AAA, cv. Grand nain). Euphytica 112:245–251.
- Elhiti, M., C. Stasolla, and A.M. Wang. 2013. Molecular regulation of plant somatic embryogenesis. In Vitro Cell Dev. Biol. Plant 49:631–642. doi: 10.1007/s11627-013-9547-3.
- FAO. 2010. The second report on the state of the World’s plant genetic resources for food and agriculture, p. 511. Food and Agriculture Organization, Rome, Italy.
- Grapin, A., J.L. Ortiz, T. Lescot, N. Ferriere, and F.X. Cote. 2000. Recovery and regeneration of embryogenic cultures from female flower of false horn plantain (Musa AAB). Plant Cell. Tissue Org. Cult. 61:237–244. doi: 10.1023/A:1006423304033.
- Horstman, A., Bermer M., and Boutilier K.. 2017. A transcriptonal view on somatic embryogenesis. regeneration (OXF) E. collection. 5:4 (4) 201–216. doi: 10.1002/REG2.91.
- Horstman, A., A. Bemer, and K. Boutilier. 2018. A transcriptional view on somatic embryogenesis Wiley regeneration. doi: 10.1002/reg2.91.
- Horstman, A., V. Willemsen, K. Boutilier, and R. Heidstra. 2014. Aintegumenta-like proteins: Hubs in a plethora of networks. Trends Plant Sci. 19:146–157. doi: 10.1016/j.tplants.2013.10.010.
- Khalil, S.M., K.T. Cheah, E.A. Perez, D.A. Gaskill, and J.S. Hu. 2002. Regeneration of banana (Musa spp. AAB cv. Dwarf Brazilian) via secondary somatic embryogenesis. Plant Cell Rep. 20:1128–1134. doi: 10.1007/s00299-002-0461-0.
- Kosky, R.G., M.F. Silva, L.P. Perez, T. Gilliard, F.B. Martinez, M.R. Vega, R.C. Milian, and E.G. Mendoza. 2002. Somatic embryogenesis of the banana cultivar FHIA-18 (AAAB) in liquid medium and scaled up in a bioreactor. Plant Cell Tissue Org. Cult. 68:21–26. doi: 10.1023/A:1012905825307.
- Martinelli, L., E. Candioli, D. Costa, and V. Poletti. 2001. Morphogenic competence of Vitis ruperis S. secondary somatic embryos with a long culture history. Plant Cell Rep. 20:279–284. doi: 10.1007/s002990100339.
- Miguel, C., and L. Marum. 2011. An epigenetic view of plant cells cultured in vitro: Somaclonal variation and beyond. J. Exp. Bot. 62:3713–3725. doi: 10.1093/jxb/err155.
- Morias - Lino, S.L., A.J. Santos - Serejo, S. Oliveira E Silva, F.R.J. Santana, and K.A. Kobayashi. 2008. Cell suspension culture and plant regeneration of a Brazilian plantain, cultivar Terra. Presq. Agropec. Bras. 43(10):1325–1330. doi: 10.1590/S0100-204X2008001000010.
- Nayak, K.A., and N. Singh. 2018. Agricultural situation in India: Growth, instability and export performance of banana in India – An economic analysis, p. 25–33.
- Novak, F.J., R. Afza, M. Van Duren, M. Parea - Dallos, B.V. Conger, and T. Xiaolang. 1989. Somatic embryogenesis and plant regeneration in suspension cultures of dessert (AA and AAA) and cooking (ABB) bananas (Musa sp.). Biotechnology 7:154–159.
- Raemakers, C.J.I.M., E. Jacobsen, and R.C.F. Visser. 1995. Secondary somatic embryogenesis and application in plant breeding. Euphytica 81:93–107. doi: 10.1007/BF00022463.
- Shivani, N.K., A. Praveen, and T. Siddharth. 2018. Identification and expression analysis of genes involved in somatic embryogenesis of banana. Acta Physiol. Plant. 40:139. doi: 10.1007/s11738-018-2714-8.
- Soriano, M., H. Li, and K. Boutilier. 2013. Microspore embryogenesis: Establishment of embryo identity and pattern in culture. Plant Reprod. 26:181–196. doi: 10.1007/s00497-013-0226-7.
- Soriano, M., H. Li, C. Jacquard, G.C. Angenent, J. Krochko, R. Offringa, and K. Boutilier. 2014. Plasticity in cell division patterns and auxin transport dependency during in vitro embryogenesis in Brassica napus. Plant Cell 26:2568–2581. doi: 10.1105/tpc.114.126300.
- Steward, F.C., M.O. Mapes, and K. Mears. 1958. Growth and organized development of cultured cells 11: Organization in cultures grown from freely suspended cells. Am. J. Bot. 45:705–708.
- Strosse, H., H. Schoofs, B. Panis, E. Andre, K. Reyniers, and R. Swennen. 2006. Development of embryogenic cell suspensions from shoot meristematic tissue in bananas and plantains (Musa spp.). Plant Sci. 170:104–112. doi: 10.1016/j.plantsci.2005.08.007.
- Su, Y.H., Y.B. Liu, B. Bai, and X.S. Zhang. 2015. Establishment of embryonic shoot-root axis is involved in auxin and cytokinin response during Arabidopsis somatic embryogenesis. Front. Plant Sci. 5:792. doi: 10.3389/fpls.2014.00792.
- Xu, C.X., B. Panis, H. Strosse, H.P. Li, H.G. Xiao, H.Z. Fan, and R. Swennen. 2005. Establishment of embryogenic cell suspensions and plant regeneration of the dessert banana ’Williams’ (Musa AAA group). J. Hortic. Sci. Biotechnol. 80(50):523–528.
- Zhong, L., E. Liu, C. Yang, S. Jin, Y. Diao, and Z. Hu. 2017. High embryogenic ability and regeneration from floral axis of Amorphophallus konjac (Araceae). Open Life Sci. 12:34–41.