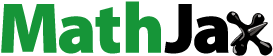
ABSTRACT
Lygus spp. are major pests of the California strawberry industry and have prompted growers to purchase and use machines called “bug vacuums” to mitigate damage throughout the production season. The aim of this work was to design, develop and evaluate a vacuum to mechanically remove and eliminate Lygus spp. from California strawberry fields at higher rates than existing vacuum systems. Field tests of the prototype vacuum were conducted in Guadalupe, California on raised strawberry beds with fully developed canopies. Results indicated that the prototype vacuum significantly increased removal of Lygus spp. compared with a conventional vacuum. The average number of Lygus spp. removed on each pass by the prototype vacuum was 2.2 times greater than that of the conventional vacuum. The use of cylindrical ducts and two fans per bed increased airflow and resultant Lygus spp. removal. The elimination baffle located at the outlet of the conventional vacuum was observed to significantly reduce air speed and raising it 30.2 cm above the outlet increased air speed by 17% (3.0 m/s) at the vacuum inlet. A validation study of a computational fluid dynamics model suggested that simulations could serve as a useful tool for bug vacuum design.
Introduction
Lygus hesperus Knight (Hemiptera: Miridae) and Lygus elisus Van Duzee (Hemiptera: Miridae) are frequently found in California strawberry fields. California strawberries account for almost 90% of total U.S. production and generated 2.3 USD billion in 2018 (U.S. Department of Agriculture, NASS, Citation2018). Lygus spp. feed on the seeds of developing fruit which can cause a deformity commonly known as ‘cat-facing’ (Strand, Citation2008). The deformed strawberries are undesirable for fresh market and are discarded or harvested for processing. Strawberry growers in the Watsonville, Santa Maria and Oxnard growing districts in California incur financial losses from Lygus spp. damage which generally increases during the summer months (Joseph and Bolda, Citation2016).
Tractor mounted vacuums for Lygus spp. management have been adopted by growers in all major California strawberry growing districts despite mixed results from studies concerning their efficacy. The near-complete adoption of bug vacuums by the California strawberry industry is largely because insecticides registered for use in California strawberries fail to consistently control Lygus spp. populations (Pickel et al., Citation1994). Growers have also attempted to mitigate damages from Lygus spp. with techniques such as alfalfa trap-cropping, braconid wasp release and reflective mulch (Joseph and Bolda, Citation2016).
Vincent and Chagnon (Citation2000) studied Lygus lineolaris P.de. B (Hemiptera: Miridae) behavior in the laboratory and found that most individuals which were still present in the plant canopy after a vacuum pass had not moved from their position prior to the pass. Of the individuals that did move, 86% moved vertically with most of that movement being downward in the canopy. Field studies by Vincent and Lachance (Citation1993), Pickel et al. (Citation1994) and Rancourt et al. (Citation2003) compared sampled numbers of Lygus spp. in a test plot before and after vacuuming to determine vacuum efficacy and these studies found that Lygus spp. populations were not consistently and significantly reduced. Studies performed by Thomas et al. (Citation2014) and Thomas et al. (Citation2015) compared direct uptake counts to estimated field populations determined by pre-treatment sampling. After several minor modifications to a commercially available vacuum, Thomas et al. (Citation2015) found that the vacuum removed an average of 16–18% of the estimated population with each pass. One such modification was the replacement of the originally solid elimination baffle with one made from perforated plate. The elimination baffle is affixed to the outlet of the vacuum such that when Lygus spp. are vacuumed off the plants, most are killed upon impact. Bug vacuums have also been used in fields infested by the Colorado potato beetle. Studies by Misener and Boiteau (Citation1992, Citation1993) measured the beetle’s aerodynamic properties and grip strength to conclude that air speed required to remove a beetle gripping the plant would be considerably larger than its suspension velocity. No such studies have been published on Lygus spp.
Computational fluid dynamics (CFD) is a popular tool for modeling fluid behavior for research and development purposes. Ponpesh and Giles (Citation2008) used CFD to model airflow and discrete phase modeling to track particle distribution through an almond harvester. There are no known studies related to the use of CFD to predict the performance of a bug vacuum. Discrete phase modeling is added to a CFD model to simulate the interaction of a fluid and discrete particles. An understanding of the aerodynamic properties of Lygus spp. and the strawberry canopy, combined with Lygus spp. grip strength, would improve the reliability of CFD predictions regarding vacuum efficacy.
Although most vacuums used in industrial and consumer applications utilize centrifugal type impellers, conventional bug vacuums use axial fans. Centrifugal impellers create high suction and can lift relatively dense objects, but the effective use of most vacuums is typically dependent on the objects being close to the inlet or residing in a sealed region where high suction can be maintained. In the strawberry field, Lygus spp. in the canopy range from the top of the canopy to the surface of the bed, which is about 30.5 cm below optimal inlet height. Because the plant canopy is a relatively open environment, suction decreases rapidly as distance from the inlet is increased. Thus, a bug vacuum must rely primarily on drag forces to remove Lygus spp. from the lower portions of the canopy. Drag forces are caused by air moving past a particle with the force being a function of the shape and size of the particle, the density of the fluid and proportional to the square of velocity. Thus, the high flow capabilities of axial fans are desirable. Axial fans efficiently produce high airflow at low static pressure in a cylindrical duct of slightly larger diameter than the fan itself; efficiency is negatively affected by increases in static pressure which are a result of reductions in duct size, outlet restrictions and changes in direction. (BASF, Citation2003).
The purpose of this study was to design, develop and evaluate a vacuum to remove Lygus spp. from California strawberry fields at higher rates than existing vacuum systems. Computational fluid dynamics was investigated as a design tool and field studies were performed to assess vacuum performance.
Materials and Methods
Vacuum Model Validation
Laboratory experiments and computer simulations were conducted to determine the reliability of simulations for predicting vacuum performance. Air speed and the response of a test particle was recorded at 48 known locations. The test particles were 2.4 mm diameter nylon balls which weighed 8 mg and had a theoretical suspension velocity of 7.8 m/s. Lygus hesperus adults are typically between 5.3–6.5 mm long and 2.3–2.8 mm wide (Kelton, Citation1982). Laboratory reared Lygus hesperus and Lygus elisus were found to weigh an average of 4.9 and 5.7 mg, respectively (Wells unpublished). The test particles were held in a device made from half of a stainless-steel 32 mesh tea ball strainer (Fu Store, Amazon.com) affixed to a rod.
The vacuum was turned on and the fan was spun between 2700–2800 RPM. Measurements were taken along two radii with six measurement locations on each radius; the measurements started at the outside edge of the fan diameter and moved toward the center in 5.1 cm increments. Measurements were taken in planes located 0, 7.6, 15.2 and 30.5 cm away from the inlet in the downward direction. The air speed at each location was recorded with a hot-wire anemometer (Testo, amazon.com) and the particle was recorded as removed or not removed after being held in position for 5–10 seconds. This procedure was performed twice at each measurement location. The experimental data was plotted such that six data markers in each plane represent the average of four air speed measurements at the corresponding distance from the outside edge of the fan diameter.
A single fan stationary vacuum was designed and built for testing in the laboratory. A simplified 3D model of the vacuum was created with the 3D drafting software Solidworks for use in a CFD simulation (). Power transmission components, structural members and parts exterior to the cylindrical duct were neglected for simplicity. The cylindrical duct, fan blades and a large exterior cylinder were included in the simulation geometry. The exterior cylinder served as the open-air environment surrounding the vacuum. The geometry was then imported into the simulation software ANSYS. In the geometry setup, the duct and fan blade were designated as solid objects. After the geometry was prepared for the simulation, a meshing tool was used to break down the geometry into discrete cells for calculation. A body sizing was applied to the fan while the remainder of the geometry meshed automatically. The mesh was limited to 512,000 cells per the ANSYS academic license limit. The mesh was loaded in the CFD software ANSYS Fluent. The bottom face of the exterior cylinder represented the ground and was designated a ‘wall’ while cylinder walls and the top face were designated ‘pressure-outlets’ at 101.325 kPa to represent the open-air environment. The k-epsilon turbulence model was used. Discrete phase modeling (DPM) was enabled and test particles were created with the same physical properties as those used in the laboratory experiment. DPM calculates particle behavior with a Lagrangian formulation and was appropriate in this simulation because the total volume of the solid particles was less than 10% of the fluid volume (ANSYS, Citation2019). Particles were placed in 48 locations with coordinates matching that of the measurement locations from the laboratory experiment. The fan blade was set to spin at a constant speed of 2750 RPM, and the simulation was run as a transient case with timesteps of 0.001 seconds. 2500 timesteps were calculated for a total flow time of 2.5 seconds and the particles were placed into the simulation after 0.5 seconds of flow time. The results of the particle removal study were collected via an animation of the simulation results for each timestep. The simulation was not repeated, so the particle response at each location was counted twice for comparison to the data collected from the laboratory experiment (). In post-processing, 50 uniformly spaced air speed values were plotted along the paths where the particles had been placed into the simulation. The simulation data was plotted on top of the experimental data and started and ended at the same locations as the experimental measurements.
Figure 1. (a) 3-D model of the single fan vacuum used in the laboratory experiment. (b) Simplified 3-D model used in the simulation
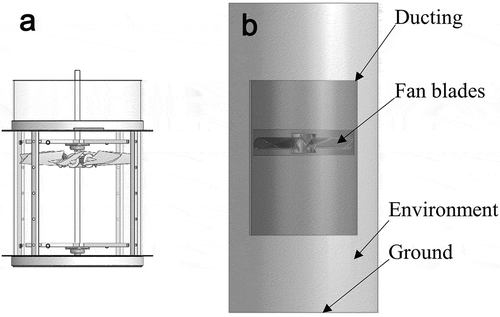
Figure 3. (a) Side view of prototype vacuum in a Guadalupe, CA strawberry field. (b) Top view schematic shows the lateral overlap and lengthwise spacing of the fans
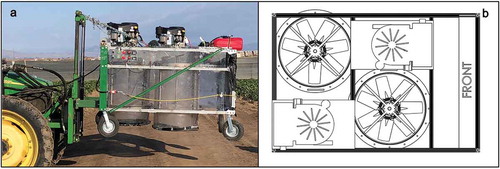
An analysis of the sensitivity and specificity of the model was performed to evaluate its practicality as a predictor of vacuum efficacy. The sensitivity is the probability that a nylon ball at a given location was correctly predicted to be removed by the simulation. In contrast, the specificity is the probability that the non-removal of a nylon ball was correctly predicted by the simulation. Mathematically:
Prototype Vacuum Design
A prototype vacuum was designed and built in the summer of 2018. The vacuum consisted of two 61 cm axial fans each driven by a 708cc gasoline engine rated for a maximum of 22 horsepower (Predator, harborfreight.com). The prototype vacuum was built to cover one strawberry bed typical of the Santa Maria, California growing region. The two fans were overlapped laterally to ensure a coverage depth of at least 25 cm along the 107 cm bed top. The ducts were spaced lengthwise in relation to the path of travel to accommodate this overlapping design (). Each 61 cm fan blade resided in a 122 cm long duct with an inside diameter of 65 cm. A belt and pulley were used on each fan such that the motors were mounted without obstructing airflow. Throttle cables and tachometers were installed on each motor for precise control of fan speed. A 26.5-degree fan was installed in the front duct and was selected to work at a relatively high static pressure (7.5 mmHg); A 30-degree fan was installed in the rear and was selected for a relatively low static pressure (1.8 mmHg). The front and rear fans spun at 3200–3300 RPM and 3000–3100 RPM, respectively. Each fan had seven blades and was of the 5ZR type manufactured by Multi-Wing America.
Field Trials-2018
The prototype and conventional vacuums were compared on six days between 16–31 Oct. 2018 in Guadalupe, California strawberry fields. The field trials followed a protocol developed by the California Strawberry Commission’s Lygus Management Program (Thomas et al., Citation2015). The fields had fully developed plant canopies of the Monterey cultivar. A 20-plant beating tray sample was taken on each side of a vacuumed bed on each date to verify the presence and estimate the abundance of Lygus spp. in the experimental plot before vacuuming. Beds were sampled such that each vacuumed bed was interior to two sampled beds and there was a buffer bed between each sampled and vacuumed bed. For a trial date with four replicates and two vacuums, nine beds were sampled prior to vacuuming. The trays used were clear plastic storage containers measuring 43 × 29 x 15 cm (Sterilite, Walmart.com).
The uptake from each prototype vacuum pass was collected with screens fashioned from the rims of 208.2 L circular container lids and 32-mesh stainless steel sheets with an opening size of 0.0635 cm and 63% open area. The screens were affixed to the inlet side of each duct with elastic cords. When the vacuum was running the screen formed a complete seal across the inlet. The tractor operator then drove the vacuum down a single bed. After the tractor exited the field, a separate large mesh bag was placed around each inlet and screen. With the bags in place, the vacuum was turned off and the debris from the screens was collected. The bags were emptied into separate large, clear, plastic containers. The Lygus spp. individuals collected from each vacuum pass and beating tray sample were counted and categorized as ‘small,’ ‘large’ and ‘adult,’ where ‘small’ approximately represented the 1st-4th instar and large represented the 5th instar. For the conventional vacuum, the same screen material was affixed to a rectangular frame that was fastened to the underside of the inlet with elastic cords. The mesh formed a complete seal around the inlet when the vacuum was running. When the operator reached the end of the row, a large plastic container was placed beneath the vacuum. The operator then turned off the vacuum and the screen and debris fell into the container. Simultaneously, a lid was placed over the container. The collected Lygus spp. were assessed in the manner described above.
This procedure was replicated for both the conventional and prototype vacuum three times on October 16 and October 24 and four times on October 17, 25, 30 and 31. Trials were performed in two different fields: October 16 and 17 in one field and the remaining trials in another field. The same beds were never used in multiple trials. Each vacuum traveled at the same speed through the field and the inlets were kept at the top of the canopy. A two-way analysis of variance (ANOVA) was performed between the treatment and trial date to determine the effect each parameter had on Lygus spp. removal.
Elimination Baffle Optimization
Four elimination baffle designs were tested and characterized according to their effect on air speed. The standard elimination baffle consists of six pieces of perforated plate welded at a 22.5-degree angle relative to the vacuum outlet. The perforations were 0.318 cm in diameter with a center-to-center distance of 0.478 cm. The sheets had approximately 40% open area. The standard elimination baffle was placed on threaded rods such that it could be raised at different heights relative to the outlet of the vacuum.
Tests were conducted with the elimination baffle mounted directly on top of the outlet, with the elimination baffle removed, and raised 5.1, 10.2 and 15.2 cm above the outlet. Nine uniformly spaced air speed measurements were taken along the edges and at the center of the inlet. A propeller-type anemometer (WeatherFlow, amazon.com) was used to record the average air speed of a 10-second reading at each location. The measurements were taken at distances of 0, 15.2 and 30.5 cm below the inlet. This procedure was repeated for each baffle configuration, and all measurements were repeated once. Measurements at a given distance from inlet and elimination baffle configuration were averaged with their corresponding repeated measurements for analysis.
Further testing was performed to determine the mortality rate of Lygus spp. when the baffle was raised above the outlet. The 15.2-cm raised configuration was tested because it appeared to have the highest probability of allowing Lygus spp. to escape. Groups of ten adults were anesthetized and placed in the mesh semi-sphere discussed previously. A large sewn fabric bag was affixed to the top of the vacuum outlet to collect the output (). With the vacuum running, a lid was placed over semi-sphere and the device was held 30.5 cm below the inlet. The lid was removed from the semi-sphere and the device was held in place until all 10 individuals were removed. The output was collected from the bag and placed in a vial for examination. The underside of the elimination baffle was inspected for remains after each test. A total of 50 Lygus spp. were released into the vacuum. All elimination baffle testing was performed using a conventional bug vacuum.
Results
Vacuum Model Validation
The simulation showed significant error in certain areas of the geometry. The average simulated air speed at the 0, 7.6, 15.2 and 30.5 cm distances from the inlet differed from the average measured air speed by +5%, +4%, −27% and −17%, respectively (). Analysis of the vacuum simulation yielded 46 removed and 50 not removed particles, while the laboratory experiment resulted in 67 removed and 29 not removed particles. The sensitivity and specificity analysis indicated that the model has a sensitivity of 61.3% and a specificity of 100% ().
Table 1. Sensitivity-specificity table comparing model predictions and experimental results
Field Trials-2018
Pre-sampling on each trial date indicated that there were Lygus spp. in the field at greater than one individual per 20 plants. The prototype vacuum removed significantly more Lygus spp. than the conventional vacuum (F1,5 = 44.5; P < .001). The prototype and conventional vacuums removed an average of 216.8 and 100.8 Lygus spp. per pass, respectively. The prototype vacuum removed between 81 and 151 more Lygus spp. than the conventional vacuum on each pass.
Elimination Baffle Optimization
Air speed was significantly higher when the elimination baffle was raised above the outlet. Each incremental increase in the distance between the outlet and elimination baffle resulted in an additional increase in air speed. The 15.2 cm raised configuration yielded a 17, 44 and 59% increase in the air speed at the 0, 15.2 and 30.5 cm distances from the inlet, respectively, compared to the elimination baffle at standard height ().
Figure 6. The effect of the fan exhaust’s elimination baffle position on the average wind speeds at three distances from the fan ducting’s inlet
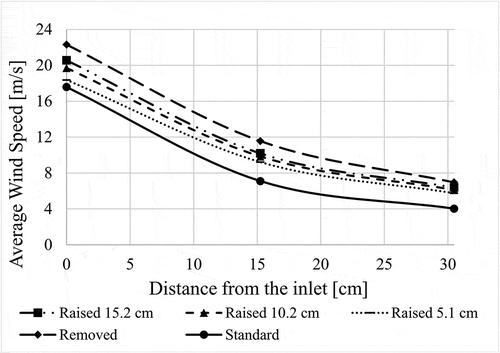
From the five mortality tests of ten individuals each, one live Lygus spp. was captured in the mesh bag on the outlet. Seven of the fifty Lygus spp. individuals were not found in the bag after the test.
Discussion
After initial calibration to determine the appropriate fan type and speed, simulation predictions agreed well with the experimental measurements in select locations. The differences are likely due to a combination of measurement error and the simplifications of the 3D model’s geometry in comparison to the single fan vacuum. In the particle removal study, the high specificity of the simulation result indicates that the model can reliably predict at what air speed a nylon ball of similar size and weight to an adult Lygus spp. will not be removed. It should be noted that increasing the specificity is considered a higher priority than increasing the sensitivity because false positives lead to an overestimation of vacuum performance and greater numbers of Lygus spp. remaining in the field. The low sensitivity of the simulation may be due to the experimental test particles being held in position for 5–10 seconds whereas the particles in the simulation immediately begin falling to the ground if the drag force is not greater than the gravitational force. Additionally, the airflow of the single fan vacuum is highly turbulent and because the particle remains in position for 5–10 seconds, there is a greater chance that a high-speed gust of air could give the particles an initial lift. Once the particle is moving it requires less force to maintain that movement and it is closer to the inlet where the air speed is higher. This may explain why all incorrect predictions were false negatives. Increasing the number of measurement planes would increase the resolution of the study and allow for better identification of removal thresholds for air speed and particle distance from the inlet. The simulation was determined to be a useful tool for characterizing vacuum airflow and particle behavior.
The prototype vacuum outperformed the conventional vacuum in field studies. Moving larger volumes of air at similar speeds appeared to increase vacuum efficacy. The larger coverage of the prototype vacuum exposed each point on the plant canopy to suction for a longer time in comparison with the conventional vacuum. Both the prototype and conventional vacuums were operated without the elimination baffle, which is used in regular field operation, but with sampling screens, which are not used during typical operation. As each component negatively affects Lygus spp. removal, we expect the field performance of each vacuum to be representative of the tested results.
Significant increases in air speed were observed with the raised-baffle designs. The 98% mortality rate of the collected Lygus spp. is adequate for field use. A conservative estimate that assumes all the missing Lygus spp. were survivors places the mortality rate at 84%. However, with the testing system shown in , there is a low probability that a live Lygus spp. could have escaped the collection bag. Most likely, the missing individuals were destroyed beyond recognition. Depending on the increase in removal associated with the greater air speed, it is possible that a lower mortality rate would still yield an overall increase in vacuum efficacy. This study neglected to test the survivability of immature Lygus spp. primarily because of the increased difficulty in the handling of the smaller Lygus spp. and identifying the remains. Future field research will be directed at finding a survival rate for both adult and immature Lygus spp.
The two gasoline engines allowed the vacuum to operate with or without a tractor but added complexity to the development and usage of the vacuum. Future designs will utilize hydraulic power and eliminate many of the components required by the gasoline engines. Hydraulic motors have a smaller footprint than the gasoline engines and can be placed directly into the duct with minimal restriction to airflow. This would eliminate the belts, pulleys and bearing assemblies required for the prototype and decrease flow restriction. The cylindrical duct design increased the total system efficiency by reducing the static pressure in the duct in comparison to the narrowed duct typical of conventional vacuums. The selected axial fans required about 15 horsepower each and produced an average air speed of approximately 20 m/s across the inlet. The prototype vacuum used nearly twice the power of the conventional vacuum which is likely a factor in the improved performance of the prototype. Future designs will seek to reduce the total power requirement such that the vacuum can cover three beds and be installed on tractors of similar size to those already in use by California strawberry growers. Overall, the prototype vacuum was found to be more effective than the conventional vacuum. This result should be further validated against other vacuum systems in the industry and after further refinement, this prototype vacuum design has the potential for commercialization.
Acknowledgments
We thank the Cal Poly Strawberry Center, the California Strawberry Commission and several California strawberry growers for assisting us. We also thank the technical assistance of Matt Haberland, Guy W. Bates, Garret Forbes and the California Polytechnic State University San Luis Obispo BioResource and Agricultural Engineering senior design class of 2019.
Additional information
Funding
Literature cited
- ANSYS. 2019. Fluent R1 theory guide. ansyshelp.ansys.com.
- BASF. 2003. Basic guidelines for plastic conversion of metal axial flow fans.
- Joseph, S.V., and M. Bolda. 2016. Efficacy of insecticides against lygus hesperus knight (Hemiptera: Miridae) in the california’s central coast strawberry. Int. J. Fruit Sci. 16(S1):178–187. doi: 10.1080/15538362.2016.1219293.
- Kelton, L.A. 1982. Plant bugs on fruit crops in Canada Heteroptera: Miridae. Research Branch Agriculture Canada, Ottawa, Canada.
- Misener, G.C., and G. Boiteau. 1992. Suspension velocity of the Colorado potato beetle in free fall. Am Potato J 70(4):309–316. doi: 10.1007/BF02851424.
- Misener, G.C., and G. Boiteau. 1993. Holding capacity of the Colorado potato beetle to potato leaves and plastic surfaces. Can. Agric. Eng. 35(1):27–31.
- Pickel, C., F.G. Zalom, D.B. Walsh, and N.C. Welch. 1994. Efficacy of vacuum machines for lygus hesperus (Hemiptera: Miridae) control in coastal California strawberries. J. Econ. Entomol. 87(6):1636–1640. doi: 10.1093/jee/87.6.1636.
- Ponpesh, P.O., and D.K. Giles. 2008. Modeling turbulent flow for design of almond harvesters with low power demand and reduced emission of particulate material. American Society of Agricultural and Biological Engineers, Davis, CA.
- Rancourt, B., C. Vincent, and D.D. Oliveira. 2003. Field evaluation of efficacy and persistence of an insect vacuum device against the tarnished plant bug (hemiptera: Miridae) in a day-neutral strawberry field. J. Econ. Entomol. 96(2):401–406. doi: 10.1093/jee/96.2.401.
- Strand, L. 2008. Integrated pest management for strawberries. 2nd ed. University of California Statewide Integrated Pest Management Program, Agriculture and Natural Resources Publication 3351, Oakland, CA.
- Thomas, H.Q., D. Legard, and M. Edsall. 2014. 2014 lygus and mite management program. California Strawberry Commission, Watsonville, CA.
- Thomas, H.Q., D. Legard, M. Edsall, and D. Olivier. 2015. 2015 California strawberry commission lygus management program. California Strawberry Commission, Watsonville, CA.
- U.S. Department of Agriculture, NASS. 2018. United States department of agriculture nation agricultural statistics service. https://www.nass.usda.gov/.
- Vincent, C., and R. Chagnon. 2000. Vacuuming tarnished plant bug on strawberry: A bench study of operational parameters versus insect behavior. Entomol. Exp. Appl. 97:347–354. doi: 10.1046/j.1570-7458.2000.00749.x.
- Vincent, C., and P. Lachance. 1993. Evaluation of a tractor-propelled vacuum device for the management of tarnished plant bug populations in strawberry plantations. Environ. Entomol. 22(5):1103–1107. doi: 10.1093/ee/22.5.1103.