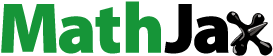
ABSTRACT
Currently, there is a growing market demand for dehydrated green mango powder for the development of value-added products. Preservation of green mangoes through drying is a good option. In this study, the influence of pretreatments and drying temperatures on drying kinetics and physicochemical properties of dried product was investigated. The sliced (4 mm thickness) green mango samples were pretreated with hot water blanching at 98°C for 3 min, 3% (w/v) ascorbic acid, and 3% (w/v) calcium chloride solutions for 1 h at room temperature (30°C). The pretreated and untreated samples were dried at 50, 60, and 70°C with an air velocity of 2.25 m/s. The dried products were ground to powder and evaluated physicochemical quality in terms of bulk and tapped density, flowability, water solubility index, rehydration capacity, total soluble solids, pH and ascorbic acid. It was observed that the moisture removal rate increased while increasing the drying temperature, and pretreated (blanching) samples dried faster than untreated samples. The experimental data were fitted to four models and evaluated for their ability to describe the drying behavior and predict the drying kinetics, of which the Logarithmic model was found to be the best. Effective moisture diffusivity and activation energy were estimated using Fick’s second law of moisture diffusion and the Arrhenius-type equation, respectively, and corresponding values were 1.209 × 10−7 to 1.566 × 10−7 m2/s and 43.873 to 44.403 kJ/mol. The samples treated with blanching and ascorbic acid showed higher physical properties and ascorbic acid content, respectively.
Introduction
Mango (Mangifera Indica L.), family Anacardiaceae, is a highly nutritious and economically important fruit crop grown in the tropical and subtropical regions of the world. India is a leading producer of mango, sharing 40% of the global production, about 55.4 million tonnes (Altendorf, Citation2019). Mangoes are rich in vitamins (A, C, E, K, B1, B2, B3, B5 and B6), minerals (potassium, magnesium, calcium and sodium), phytonutrients (α, β-carotene, and crypto-xanthin), carbohydrates, calorie, while low in protein, fat and dietary fiber (Zafar and Sidhu, Citation2017). Though mangoes are rich in antioxidants and phytonutrients, they have a short shelf life due to high moisture content (80% w.b.) (Dereje and Abera, Citation2020), as mangoes are seasonal crop, overproduction during peak season and scarcity during offseason. Moreover, studies have reported that annual postharvest losses for mangoes in India are about 12.74% (Narayana et al., Citation2014).
Preservation of green mango is one of the best options to reduce postharvest losses. Green mango has several medicinal properties, including cure scurvy, stomach troubles, promote liver and heart health, and prevent body dehydration (Lauricella et al., Citation2017); and widely used for the preparation of pickle, chutney, sauce, green mango drink, and a dehydrated powder called as amchoor (Zafar and Sidhu, Citation2017). Amchoor is used in culinary preparation, categorized under spices for different chat foods, used as an acidulant instead of tamarind, and popular in the preparation of pulihora mix that utilized for making instant rice-based food. There is a growing market demand for the dehydrated powder to prepare value-added products (Prabhakararao et al., Citation2012). Traditionally, the sun drying method is commonly used for drying green mango to produce amchoor. There are some significant issues associated with sun drying, such as long drying time, uneven drying, inferior quality product, high spoilage due to microbes, birds, and rodents (Goyal et al., Citation2006). To overcome these issues and to fulfill market demand, there is a need for improved drying techniques. Convective hot air drying has been recognized as the cost-effective food preservation method commonly used in the food industry, mainly for drying fruits (Taşeri et al., Citation2018).
Mechanical driers are more efficient in fast and uniform drying, hygienic dried product, prolong the shelf life of the dried products by reducing the destructive potential of microbial and enzymatic activities (Afolabi et al., Citation2015; Olanipekun et al., Citation2015). Although the mechanical dryers have several advantages, some demerits escort mainly degradation of nutritional quality and changes in physicochemical properties (Dereje and Abera, Citation2020; Nyangena et al., Citation2019). Pretreatment can minimize the adverse changes in color, texture hardness and shrinkage, and functional properties during drying. Besides, it significantly affects structural softening, inactivating enzymes, destroying microorganisms, preventing browning, improving dried product quality, facilitating moisture removal, and enhancing the drying process (Aral and Bese, Citation2016; Deng et al., Citation2019; Junqueira et al., Citation2017). Pretreatment also has a great potential in reducing the degradation of vitamins, pigments, flavonoids and phenolic acids during drying (Vardin and Yılmaz, Citation2018). Recently, hot water blanching and chemical pretreatments have been frequently used for drying fruits in the hot-air convective dryer. Dinrifo (Citation2012) used hot water blanching at 100°C for 2 min and dipping in 0.01% sodium metabisulfite for sweet potato slices, Garba et al. (Citation2015) used hot water blanching at 98°C for 3 and 6 min for carrot shreds, Afolabi et al. (Citation2015) applied hot water blanching at 100°C for 5 min and soaking in 0.2% sodium metabisulphite for 5 min for cocoyam slices, Adepoju and Osunde (Citation2017) reported steam blanching at 120°C for 2 min, dipping in 31% ascorbic acid and 21% honey solutions for 4 min for mango fruit, Vardin and Yılmaz (Citation2018) applied blanching of pomegranate arils by dipping into hot water with 0.1% citric acid at 80 ± 2°C for 2 min, Nyangena et al. (Citation2019) stated steam blanching for 3 min, dipping in 1% citric acid and 0.5% lemon juice for 40 min for mango slices, Dereje and Abera (Citation2020) recommended hot water blanching at 90°C for 1 min and dipping in 0.5% lemon juice for 10 min for mango fruit slices, and (Brar et al. (Citation2020) used to tip in 1% ascorbic acid, citric acid, and potassium metabisulfite solution at 40°C for 1 min for yellow European plums.
Drying is a simultaneous heat and mass transfer process in which diffusion of moisture occurs from inside to outside due to water vapor pressure difference (Chakraverty and Singh, Citation2014). The knowledge of heat and mass transfer mechanism (moisture diffusivity, activation energy, and energy consumption) is necessary for designing the dryer and quality of the dried product (Aral and Bese, Citation2016). According to Onwude et al. (Citation2016), the thin-layer drying method is most widely used to determine the drying kinetics of fruits and vegetables. Drying kinetics is an effective tool to describe the drying behavior and drying mechanism, optimize the process parameters, predict and simulate the drying data, analyze the heat and mass transfer rate during drying, determine the moisture diffusivity and activation energy, and design of ideal dryer (Da Silva et al., Citation2014; Kumar et al., Citation2012; Malaikritsanachalee et al., Citation2018; Olanipekun et al., Citation2015).
Recently, there have been several researches on mathematical modeling of thin-layer drying characteristics of various fruits and vegetables, such as kiwifruit (Orikasa et al., Citation2008), banana slices (Doymaz, Citation2010), jackfruit leather (Chowdhury et al., Citation2011), carrot pomace (Kumar et al., Citation2012), apple slice (Rayaguru and Routray, Citation2012), passion fruit (Bezerra et al., Citation2015), cocoyam slices (Afolabi et al., Citation2015), pineapple (Olanipekun et al., Citation2015), carrot shreds (Garba et al., Citation2015), hawthorn fruit (Aral and Bese, Citation2016), pomegranate arils (Mazandarani et al., Citation2017; Vardin and Yılmaz, Citation2018), gooseberry (Junqueira et al., Citation2017). However, studies on thin-layer drying of green mango slices in the literature were very few (Corzo et al., Citation2011; Goyal et al., Citation2006). Besides, no study on physicochemical properties such as bulk and tapped density, flowability, water-solubility, rehydration capacity, pH, total soluble solids and ascorbic acid of dried green mango powder was reported. The main objectives of this investigation were (i) to study the effects of hot water blanching and chemical pretreatments and drying temperature on the drying characteristics of green mango slices; (ii) to fit the experimental data to four thin layer drying kinetics; (iii) to determine the effective moisture diffusivity and activation energy for drying of green mango slices; (iv) to evaluate the physicochemical properties of the dried green mango powder.
Materials and Methods
Sample Preparation
Mango cultivar “Dasheri” with a maturity level of stage-1 free from damage was purchased from a local farmer’s field nearby SHUATS, Allahabad (India) and immediately transported to the laboratory. The maturity stages of mango can be characterized into stage-1 (dark olive green), stage-2 (olive), stage-3 (apple green), stage-4 (brown), stage-5 (saddle brown) and stage-6 (dark goldenrod color) based on physical and chemical attributes such as color, flesh firmness, moisture, solids, sugar and acid contents (Mim et al., Citation2018). The mangoes were washed in running water to remove dirt and foreign matters and peeled manually using stainless steel blade peeler. The edible portion around the pit was sliced at a thickness of 4 mm using a food-grade stainless steel slicer; however, other dimensions (length and width) were not constant. The mango slices were washed in water and removed excess moisture on the surface of the slices by spreading them in blotting paper. The sliced samples were stored in a domestic refrigerator until preparation for pretreatments. The initial moisture content of the green mango (507.91% (d.b.)) was estimated using the hot-air oven at 105°C for 24 h (AOAC, Citation2000).
Pretreatments
Three different pretreatments, such as hot water blanching (T2), ascorbic acid (T3) and calcium chloride (T4), were tested, and untreated (T1) was performed as a control. The selection of treatment methods, procedure, temperature, time, and chemical concentration was based on the information available in the published literature. Deng et al. (Citation2019) reviewed that blanching increases the structure softening, cell wall destruction, and drying rate and reduces moisture movement resistance during drying. Brar et al. (Citation2020) stated that ascorbic acid is an enzyme inhibitor, bleaching and reducing agents. Udomkun et al. (Citation2014) reported that calcium is widely used to decrease physiological disorders, delaying membrane lipid catabolism, polyphenol oxidase induces browning, and improving firmness. Therefore, these treatment methods were chosen. The sliced green mango samples were divided into different groups, each 250 g.
For hot water blanching, the sliced green mango samples were immersed in the water bath at 98°C for 3 min, and drained excess water and cooled immediately to prevent overcooking (Adepoju and Osunde, Citation2017; Dinrifo, Citation2012; Garba et al., Citation2015; Vardin and Yılmaz, Citation2018). For ascorbic acid pretreatment, 3% (w/v) ascorbic acid solution was prepared by dissolving 30 g ascorbic acid powder in 1000 mL distilled water, and the samples were immersed in the solution for 1 h at room temperature (approximately 30°C) (Adepoju and Osunde, Citation2017; Brar et al., Citation2020). For calcium chloride pretreatment, prepared a calcium chloride solution of 3% (w/v) by dissolving 30 g anhydrous calcium chloride in 1000 mL distilled water. Then, samples were soaked in the solution for 1 h at room temperature (Udomkun et al., Citation2014). The pretreated samples of each group were allowed to drip excess water for 20 min and spread on the blotting paper to remove surface wetness before drying. The experiments were triplicated.
Drying Process
The treated and untreated green mango samples were dried using a laboratory-scale cabinet hot-air dryer (Kilburn, India) at 50, 60, and 70°C. As per the manufacturer's specifications, the outer dimension of the dryer is 110 × 80 × 90 cm (length × width × height). The stainless steel trays (size: 90 × 40 cm) with round perforations (diameter = 1 cm) are placed in the drying chamber. Two heat blower fans fixed at the bottom of the drying chamber blows air at a constant speed of 2.25 m/s, but an air-flow regulator not provided. Two ventilation holes, each 5 cm diameter, are provided at the top of the dryer to escape the exhaust air. A microcontroller-based temperature controller is installed to control the temperature. The desired temperature can be set by pressing the SET button (to increase or decrease the temperature) of the digital indicator cum controller and act to supply or cut off the power to the heating coil. shows the photograph of the convective hot-air dryer. The dryer was operated for 30 min at the required temperature to stabilize the drying chamber’s temperature before starting the experiment. The samples were spread in a single layer on the trays and positioned in the dryer. The hot-air direction is from the bottom of the trays through the perforations and horizontal over the product surface. The samples were weighed at a time interval of 60 min using a digital electronic balance in the measurement range of 0–400 g with an accuracy of ± 0.001 g. The drying process terminated when consecutive readings become constant. All the experiments were carried out in triplicates.
Moisture Content Measurement
The initial moisture content, moisture content during the drying process, and equilibrium moisture content of the samples were determined using Eqs. (Equation1(1)
(1) ), (Equation2
(2)
(2) ) and (Equation3
(3)
(3) ) respectively (Malaikritsanachalee et al., Citation2018).
To attain equilibrium moisture content (Me), the samples in all conditions were dried until no considerable weight loss.
Drying Rate Calculation
Drying rate (DR) is the amount of water removed from the unit weight of food material per unit time (Chakraverty and Singh, Citation2014) and expressed by g water/g dry matter/min. The DR was estimated by Eq. (Equation4(4)
(4) ):
Determination of Moisture Ratio
The thin layer drying action can be represented based on Newton’s law for heating or cooling of solids, assuming no resistance to moisture movement from the center to the surface of the material (Chakraverty and Singh, Citation2014). The rate of change in moisture content to time can be expressed by Eq. (Equation5(5)
(5) ):
The moisture ratio (MR) was obtained by integrating and simplifying Eq. (Equation5(5)
(5) ) with suitable limits M (r, i) = Mi and M (r0, t) = Me. The MR was determined by Eq. (Equation6
(6)
(6) ):
The experimental MR values were estimated by substituting the experimental moisture content data in Eq. (Equation6(6)
(6) ), and curve fitting were done between the experimental MR data and drying time.
Evaluation of Thin-layer Drying Kinetics
Several thin-layer drying kinetics have been proposed to demonstrate the drying characteristics of fruits and vegetables based on Fick’s second law of moisture diffusion (Onwude et al., Citation2016). Among popular models, Page, Modified Page, Henderson and Pabis, and Logarithmic models, as shown in , were tested for selecting the appropriate model that can describe the suitability of the drying process of green mango slices. The experimental data were fitted in these normalized models and evaluated model constants using the non-linear regression procedure of XLSTAT 2016. The goodness-of-fit of the models was assessed based on the statistical parameters such as correlation coefficient (R2), sum square error (SSE), and root mean square error (RMSE), and calculated corresponding values through the Eqs. (Equation7(7)
(7) ), (Equation8
(8)
(8) ) and (Equation9
(9)
(9) ), respectively (Malaikritsanachalee et al., Citation2018; Mazandarani et al., Citation2017; Olanipekun et al., Citation2015).
Table 1. Thin-layer drying models used to describe the drying of green mango slices
These parameters can be used to evaluate the linear relationship between experimental MR and predicted MR. The model that has the highest value of R2 and lowest values of SSE and RMSE would be the best goodness-of-fit (Chowdhury et al., Citation2011; Kumar et al., Citation2012; Olanipekun et al., Citation2015). A model is the best fit when the R2 value closer to 1 (Chen et al., Citation2013) and a prediction is nearer to the experimental data when the SSE and RMSE approach zero (Garba et al., Citation2015).
Estimation of Effective Moisture Diffusivity
In the drying of biological material, complete drying occurs in the falling-rate period of the drying process, and Fick’s second law of diffusion can describe the moisture movement phenomenon within the material. Based on this law, Crank (Citation1975) developed a diffusion model (Eq. Equation10(10)
(10) ) to estimate the effective moisture diffusivity (Deff) with the following assumptions: (i) uniform initial moisture distribution (ii) constant moisture diffusivity and temperature (iii) negligible shrinkage and external resistance (iv) material with slab geometry.
Drying of green mango slices took place in the falling-rate phase. For long drying time, the first term of the series alone can be considered (Aral and Bese, Citation2016; Garba et al., Citation2015; Kumar et al., Citation2012), and other terms in the series are neglected (n = 0) then the Eq. (Equation10(10)
(10) ) was re-written as follows after taking natural logarithm from both sides:
Graphs were plotted between experimental ln(MR) and drying time. The slope value was calculated from the slope of the straight line using Eq. (Equation12(12)
(12) )
In this study, the effective moisture diffusivity (Deff) was estimated using Eq. (Equation14(14)
(14) ).
Determination of Activation Energy
The Arrhenius-type equation for diffusion is assumed to describe the relationship between the effective moisture diffusivity and drying temperature (Doymaz, Citation2010).
To obtain the Arrhenius plot, Eq.Equation15(15)
(15) was linearized by applying a natural logarithm at both sides.
A slope of the Arrhenius plot of ln(Deff) against reciprocal absolute temperature (1/T) plotted. The activation energy and Arrhenius constant were estimated from the fitted slope and the y-intercept, respectively.
Evaluation of Physicochemical Quality of Powder
The dried green mango slices were allowed to cool at room temperature and ground to a size of 250 μm using a mixer grinder (Icon Superb 1000 W, Morphy Richards, Mumbai, India). The powder was packed in polyethene bags of 75 μm thick and sealed to prevent caking. shows the photograph of the dried green mango powder. The physicochemical quality parameters were evaluated for moisture content, bulk and tapped density, flowability, water-solubility, rehydration capacity, pH, total soluble solids, and ascorbic acid.
Bulk Density
The powder sample of 5 g was gently introduced without compacting into a dry graduated cylinder of 50 mL and measured the apparent unsettled volume to the nearest graduated unit (Chegini and Ghobadian, Citation2005). The bulk density was calculated using the following formula:
Tapped Bulk Density
Five gram of powder sample was taken in a 50 mL graduated measuring cylinder. After observing the initial powder volume, the cylinder was gently tapped 10–15 times onto a rubber mat from a height of a maximum of 15 cm. It measured the final volume of the powder sample after tapping (Dehghannya et al., Citation2019). The tapped bulk density was determined as given below:
Flowability
The flowability of the powder was estimated in terms of the Carr index and the Hausner ratio. The bulk and tapped density terms used in the determination of the Carr index and Hausner ratio as described by Dehghannya et al. (Citation2019):
Water Solubility Index
The water solubility index (WSI) is an important physical property of the food powders that measures the rate of dispersing ability of powder in a liquid medium (Grabowski et al., Citation2006). The powder sample of 5 g was thoroughly dissolved with 50 mL distilled water using a magnetic stirrer and centrifuged at 5000 rpm for 10 min using a digital centrifuge machine (LI-GEN-DIGI, Labard Instruchem Private Limited, Kolkata, India). The supernatant was taken in a petri dish and dried at 130°C using a hot air oven and weighed the dried supernatant’s solids (Kandasamy et al., Citation2019). The following formula estimated the WSI:
Rehydration Capacity
Five gram of the powder sample was dissolved in 100 mL distilled water using a magnetic stirrer at room temperature (28°C) and filtered the solution using Whatman filter paper No1. The resulted filtrate was weighed, and rehydration capacity was estimated using a given formula (Ramallo and Mascheroni, Citation2012):
Total Soluble Solids, pH and Ascorbic Acid
The powder sample was reconstituted in distilled water in the ratio of 1:20 w/v (powder: water) and centrifuged at a speed of 5000 rpm for 10 min using a digital centrifuge machine. The total soluble solids (TSS) were determined by placing a drop of the supernatant on the refractometer’s prism (Master-53a, Atago, Japan) and expressed as degree Brix. The pH level was measured using a digital pH meter (Type-335, Systronics India Ltd, Ahmedabad, India). The ascorbic acid was estimated by the 2, 6-Dichlorophenol-Indophenol visual titration method (Ranganna, Citation1986).
Statistical Analysis
Each experiment was carried out in triplicate, and mean values with standard deviation were reported. The obtained data were analyzed with the completely randomized design using the SPSS software (version 16.0.0 software, SPSS Inc., Chicago, USA), and defined the significance at a 5% level.
Results and Discussion
Drying Characteristics
The effect of pretreatments and drying temperatures on variation in moisture content of green mango is presented in ). The drying curves demonstrated that the drying time decreased as the drying temperature increases. Obviously, while increasing the drying temperature speeds up the drying process, consequently reduced the time of drying. It can also be observed from the Figure that the moisture content decreased over the drying time. For instance, at 50°C, moisture content of the hot-water blanched and chemically treated samples reached 16.23 and 17.45% (db), respectively, by 540 min from an initial moisture content of about 507.91% (db) whereas, for untreated sample, it reached 24.43% (db) within the same time. Similar drying behavior took place at 60°C, there, 480 min spent to reach 14.65% (db) and 16.39% (db) for hot-water blanched and chemically treated samples, respectively, but the moisture content of untreated samples was 21.07% (db). At 70°C, pretreated samples’ moisture content reached the range of 11.37–12.52% (db) within 420 min. The reduction of drying time with pretreatment and increasing temperature may be due to an increase in water vapor pressure within the pretreated products, which resulted in speeds up diffusion of moisture to the product surface (Afolabi et al., Citation2015).
Figure 3. Drying curves for untreated (T1), hot water blanching (T2), ascorbic acid (T3), and calcium chloride (T4) pretreated green mango slices dried at (a) 50°C (b) 60°C and (c) 70°C. Error bars with 5% value. Data are presented in mean ± standard deviation, n = 3, p < .05
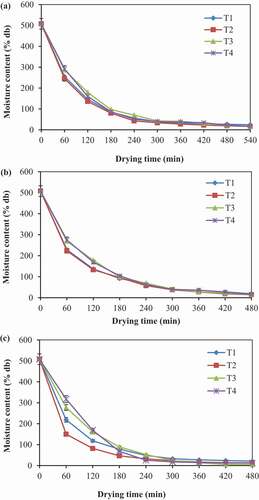
Besides, the moisture content was marginally varied for the different pretreatment methods. The hot water blanching reduces the resistance to moisture diffusion due to cell wall destruction and softening of structure, ascorbic acid accelerates water removal as pectin loosening in an acidic environment, and calcium chloride prevents the loss of firmness (Deng et al., Citation2019). Kumar et al. (Citation2012) reported similar findings during thin-layer drying of carrot pomace in a forced convection hot air dryer, where moisture reduction from an initial moisture content 830 ± 1% (db) to 7.68, 6.64, 5.68, and 4.07% (db) at 60, 65, 70, and 75°C, respectively, was observed. A reduction in drying time 10, 6.67, and 4.76% in each temperature interval also observed. Similar drying behavior for the influence of drying temperature on drying time has been reported for yellow European plums (Brar et al., Citation2020), cocoyam slices (Afolabi et al., Citation2015), and stone apple slices (Rayaguru and Routray, Citation2012). The present study results were in good agreement with the findings of Vardin and Yılmaz (Citation2018), who reported that the moisture content of hot-water blanched pomegranate arils reached 16% (wb) in 168, 204 and 237 min at 75, 65, and 55°C, respectively. For untreated samples, the values were 244, 448, 945 min at the same temperature in a hot-air cabinet dryer. Garba et al. (Citation2015) also reported similar trends for hot water blanched and unblanched carrot shreds at 40, 50, and 60°C in a laboratory-scale tray dryer.
Drying Rate
) shows drying rate versus drying time at 50, 60, and 70°C. It is clear from the Figure that the drying rates decreased continuously with drying time. Although it declined constantly, complete drying took place in the falling rate period at all drying conditions and did not observe the constant rate period of drying. Moisture movement phenomena can describe it; there, the moisture diffusion from the interior to the outer surface is due to moisture concentration differences (Chakraverty and Singh, Citation2014). The decreasing trend in drying rate over the drying progression is mainly due to reduced moisture content. The drying rate was higher at the beginning of drying than at the end because the moisture diffusion rate decreases at the final stage of drying (Kumar et al., Citation2012). Moreover, the drying rate increased as the drying temperature increases and higher values observed at 70°C as compared to other drying temperatures. As expected, a higher drying temperature leads to a greater moisture evaporation rate that induces moisture to diffuse from inside to outside (Garba et al., Citation2015). The drying rate behavior for pretreated and untreated samples was parallel. Among pretreatments, the hot water blanched treatment showed a marginally higher drying rate. Similar behavior in drying rate has reported for hawthorn fruit (Aral and Bese, Citation2016), cocoyam slices (Afolabi et al., Citation2015) and banana (Da Silva et al., Citation2014).
Moisture Ratio
) shows the moisture ratio changes with drying time at 50, 60, and 70°C. It is clear from the Figure that the moisture ratio decreased continuously for all treatments as the drying advances. This decrease in moisture ratio shows the diffusion of the internal mass transfer due to the water vapor pressure difference between the center and surface of the product. Moreover, the moisture ratio decreased rapidly at the beginning of the drying process than at the final stage of drying because of the slower diffusion of residual moisture. The values of the final moisture ratio observed in the range of 0.03–0.05. Moreover, the ascorbic acid treatment showed a marginally higher moisture ratio than hot water blanching, calcium chloride pretreatments and control in all drying conditions. A similar decreasing trend in moisture ratio over the drying time have reported for thin-layer drying of cocoyam slices (Afolabi et al., Citation2015), pineapple (Agarry et al., Citation2013) and carrot pomace (Kumar et al., Citation2012).
Figure 5. Graphs of moisture ratio versus drying time for untreated (T1), hot water blanching (T2), ascorbic acid (T3), calcium chloride (T4) pretreated green mango slices dried at (a) 50°C (b) 60°C and (c) 70°C. Error bars with 5% value. Data are presented in mean ± standard deviation, n = 3, p < .05
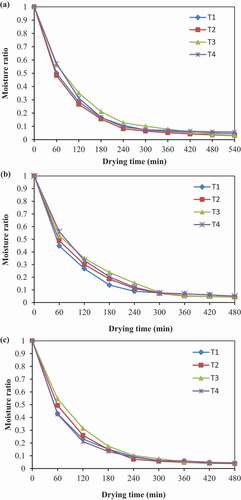
Modeling of Thin-layer Drying Kinetics
Moisture ratios (MR) were calculated from the experimental data and fitted into the thin layer drying models listed in . The statistical parameters such as correlation coefficient (R2), sum square error (SSE), and root-mean-square error (RMSE) estimated to verify the goodness-of-fit of each model and estimated the drying model constants. The results of statistical parameters and drying constants are presented in . The R2, SSE, and RMSE values were compared and evaluated the performance of the models. The best model was chosen based on the highest R2 and lowest SSE and RMSE values with reference to previous thin layer drying studies (Chen et al., Citation2013; Kumar et al., Citation2012; Mazandarani et al., Citation2017; Olanipekun et al., Citation2015).
Table 2. Experimental constants and statistical parameters of different thin-layer drying models for drying of green mango slices
In the present study, R2 values varied in the range of 0.801–0.998, SSE and RMSE values varied from 0.00015 to 0.00092 and 0.00253 to 0.00922, respectively. Among the thin layer drying models, the Logarithmic model was performed well in fitting experimental data during the drying process. Therefore, the Logarithmic model was chosen as the best descriptive model (), as proposed by the highest average value of R2 (0.993), the lowest average values of SSE (0.00027) and RMSE (0.00388). Besides, the best treatment was selected within the Logarithmic model using the same procedure. Of these, hot water blanching treatment (T2) showed the highest value of R2, the lowest values of SSE and RMSE (). At 50°C, R2 = 0.998, SSE = 0.00014 and RMSE = 0.00136, at 60°C, R2 = 0.996, SSE = 0.00022 and RMSE = 0.00282) and at 70°C, R2 = 0.995, SSE = 0.00017 and RMSE = 0.00281).
The values of Logarithmic model constants (k, a, and c) for hot water blanching treatment (T2) dried at 50, 60, and 70°C are presented in . To calculate the predicted moisture ratio performed Logarithmic regression analysis and validated the selected model by comparing the predicted moisture ratio with the experimental moisture ratio (). It is clear from the Figure that the data points moving close to the straight line showing R2 value of 0.967, 0.982 and 0.966 for 50°C, 60°C, and 70°C, respectively, which indicates that the logarithmic model could be suitable to describe the thin-layer drying behavior of the green mango slices. Similarly, studies have reported that the suitability of the Logarithmic model in describing the thin-layer drying characteristics of cocoyam (Agarry et al., Citation2013), stone apple (Rayaguru and Routray, Citation2012), banana (Doymaz, Citation2010) and apple pomace (Wang et al., Citation2007).
Effective Moisture Diffusivity
Effective moisture diffusivity (Deff) used to describe the theory of moisture movement within the food material during drying. Deff values of green mango slices during drying were estimated using the slope method, as shown in Eq. (Equation14(14)
(14) ). The slope value was calculated using the slope of the graph, which plotted between natural logarithmic moisture ratio and drying time (). The effect of pretreatments and drying temperatures on Deff is presented in . The results showed that the Deff increased with an increase in drying temperature as the moisture diffusion is temperature-dependent. Rapid moisture diffusion occurs at higher drying temperatures due to the rise in water vapor pressure gradient between inside and outside the sample. In the present study, Deff values were varied from 1.209 × 10−7 to 1.382 × 10−7 m2/s for untreated and from 1.291 × 10−7 to 1.566 × 10−7 m2/s for pretreated green mango slices over the drying temperatures (). The Deff rate under thin-layer drying of food materials lies within 10−7 to 10−12 m2/s (Aral and Bese, Citation2016; Bezerra et al., Citation2015; Kumar et al., Citation2012; Malaikritsanachalee et al., Citation2018; Olanipekun et al., Citation2015). Besides, pretreatments also had a significant effect on the effective diffusivity. The hot water blanching treatment showed significantly higher Deff as compared to other treatments. Agarry et al. (Citation2013) reported the blanching treatment enhanced the rate of Deff of pineapple. In contrast, Garba et al. (Citation2015) noticed a decreasing trend in Deff for blanched black carrot shreds compared to unblanched.
Table 3. Effective moisture diffusivity and activation energy at different pretreatments and drying temperatures
Figure 7. Natural logarithmic of moisture ratio versus drying time for estimation of the effective moisture diffusivity of untreated (T1) and treated with hot water blanching (T2), ascorbic acid (T3), calcium chloride (T4) green mango slices dried at (a) 50°C (b) 60°C and (c) 70°C. Error bars with 5% value
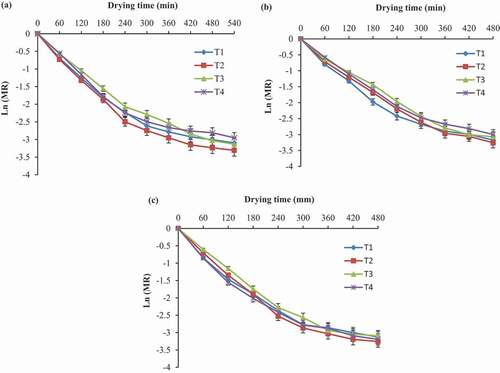
Activation Energy
The importance of activation energy is to study the energy required to initiate the moisture transfer from the interior to the surface of the food materials during the drying process. The activation energy for most fruits and vegetables within the range of 12.7–110 kJ/mol and lower activation energy indicates an ease diffusion of moisture (Aral and Bese, Citation2016; Olanipekun et al., Citation2015). In the present study, a slope of the graph of ln(Deff) against inverse absolute temperature (1/T) was plotted (). The activation energy (Ea) and Arrhenius constant (D0) were estimated using fitted slope (Eq.Equation17(17)
(17) ) and y-intercept, respectively. The values of D0 and Ea are presented in . The values of D0 were calculated in the range of 1.131 × 10−7-1.259 × 10−7 m2/s. Similarly, the values of D0 in the range of 1.59 × 10−7-2.314 × 10−7 m2/s for potato have reported by Senadeera et al. (Citation2003). The values of Ea were found to be in the range of 43.873–44.403 kJ/mol over the treatments. On the other hand, no significant variation in Ea between pretreated and untreated samples. These values are within the general range of 12.7–110 kJ/mol for fruits and vegetables (Aral and Bese, Citation2016). Studies have reported that the activation energy for drying of dika nuts (Aregbesola et al., Citation2015), kiwi fruit (Orikasa et al., Citation2008), carrot shreds (Garba et al., Citation2015) were 37.019, 38.6, and 26.0–34.6 kJ/mol, respectively.
Physicochemical Quality Parameters
Moisture Content
Moisture content plays a vital role in determining the functional properties and predicting the shelf life of the dried products. The moisture content of the green mango powder treated with hot water blanching, ascorbic acid solution, and calcium chloride solutions in the range of 16.23–17.45% (db) dried at 50°C, 14.65–16.39% (db) at 60°C, 11.37–12.52% (db) at 70°C, and for untreated 21.07–24.43% (db) (). This variation in moisture content between pretreated and untreated samples may be due to pretreatment speeds up moisture diffusion during the drying process. Garba et al. (Citation2015) reported similar trends in drying of blanched and unblanched carrot shreds at 40, 50, and 60°C. Kumar et al. (Citation2012) also demonstrated that the final moisture content of dried carrot pomace was 7.68, 6.64, 5.68, and 4.07% (db) at 60, 65, 70, and 75°C, respectively, during forced convective drying.
Bulk and Tapped Density
The bulking properties are measures of the ability of the powder to flow, which is influenced mainly by the inter-particulate interactions. These interactions are directly affected by moisture content, particle size distribution, pores space and compaction profile. Comparing the bulk and tapped density is generally used as an index of the ability of the powder to flow. Such interactions are less significant in a free-flowing powder. Thus, the difference between bulk and tapped densities will be closer, and these values more in a poorer flowing powder as the more significant interactions. These differences reflected in the Carr Index and Hausner ratio (Dehghannya et al., Citation2019); accordingly, they have classified the flowability of the food powders ().
Table 4. Powder flowability based on Carr index and Hausner ratio values, Dehghannya et al. (Citation2019)
Table 5. Physicochemical characteristics of the green mango powder at different pretreatments and drying conditions
The bulk and tapped density of the green mango powder are presented in . The result showed that the drying temperature and pretreatments significantly affected the bulk and tapped density. An increasing trend in bulk and tapped density observed as the drying temperature increased from 50 to 70°C. The changes in bulk and tapped density of pretreated powder were in the range of 0.7–0.77 and 0.82–0.89 g/cm3, respectively, whereas untreated powder values were 0.55–0.63 and 0.67–0.75 g/cm3, respectively. The bulk and tapped densities increased as high as 40% and 32.84%, respectively. This increase may be due to pretreatments as well as increasing the drying temperature. The difference between bulk and tapped density was found closer in value in all drying conditions. Moreover, the bulk and tapped density of untreated powders significantly lower as compared to the pretreated powders. The lower value may be due to the higher moisture content in untreated powders.
Chegini and Ghobadian (Citation2005) stated that the bulk and tapped density of the powdered material decreased at higher moisture due to particles’ tendency to adhere to each other, creating more pores within them increase in the volume of powder. Consequently, an increase of powder’s density at higher temperature is associated with higher moisture removal. The bulk density of pretreated green and ripe mango flour dried in a hot-air dryer at 60°C was 0.69 and 0.68 g/ml, respectively (Aziz et al., Citation2012). Loose and packed bulk density values of Tommy Atkin mango powder dried in a hot-air cabinet dryer at 60 ± 2°C were 0.44 and 0.63 g/ml, wb, respectively (Sogi et al., Citation2015). The bulk and tapped density of Amropali mango powder dried in a laboratory-scale cabinet dryer at 70°C were 0.50 and 0.61, respectively (Akther et al., Citation2020). The bulk and tapped densities in the present study were in good agreement with those reported previously.
Flowability
Carr index (CI) and Hausner ratio (HR) values of the green mango powder are given in . The results showed that the pretreatments and drying temperature had a significant effect on the CI and HR of the powder. The CI and HR values decreased while increasing the drying temperature. Besides, the flowability of powder improved by increasing the drying temperature. The CI and HR values of the pretreated powder were recorded in the range of 13.48–14.63% and 1.15–1.17, respectively, whereas their values of untreated powder were 16–17.91% and 1.19–1.22, respectively. The CI values characterize the flowability properties of powders, and high CI values exhibit a food powder has poor flowability (Yılmaz and Bastıoğlu, Citation2020). As the CI and HR values of pretreated green mango powder were within the general range of 11–15% and 1.12–1.18, respectively (), the powders had excellent flowability. On the other hand, the flowability of the untreated green mango powder fetched fair as the CI and HR values were within the range of 16–20% and 1.19–1.25, respectively. The results confirmed the findings of Akther et al. (Citation2020), who reported that the mango powder dried in a laboratory-scale cabinet dryer at 70°C had good to fair flowability as the HR and CI were 1.22 and 18.03, respectively.
Water Solubility Index
The water solubility index (WSI) is an important physical property of the powder that measures the ability to dissolve in water and the amount of soluble solids released from the powder to form a homogeneous, stable solution (Jafari et al., Citation2017). A perfect powder characterized by its rapid wettability completely sinks into the water and dissolves without lumps and cake formation (Dehghannya et al., Citation2019). Water absorption, distribution and dispersion ability of powder are influenced mainly by the drying conditions (Kandasamy et al., Citation2019). In the present study, the WSI of the green mango powder increased significantly by increasing the drying temperature from 50 to 70°C, and pretreatments also considerably influenced the WSI. The WSI variations were in the range of 78.94–79.52% on pretreated powder but 78.45–79.25% on untreated powder (). A significant reduction in WSI observed in untreated powder as compared to the pretreated powder within the drying temperature. This reduction may be due to particle size distribution in high moisture powder. The fine particles have a remarkable ability to dissolve in water than coarse particles. The solubility values in the present study are comparable with the findings of Sogi et al. (Citation2015), who reported the solubility of 78.69 g/100 g for Tommy Atkin mango powder dried in a hot-air cabinet dryer at 60 ± 2°C. On the other hand, Akther et al. (Citation2020) reported a higher solubility of 98.2% for Amropali mango powder dried in a cabinet dryer at 70°C. The difference in solubility may be due to different ripening stage and cultivar. Akther et al. (Citation2020) used full ripe mangoes of ‘Amropali’ cultivar, whereas the present study used green mangoes of ‘Dasheri’ cultivar.
Rehydration Capacity
The water absorption potential of the powder is termed as rehydration capacity (RC). Rehydration is a multi-stage process in which water absorption by powder, transfer to the porous network, distribution within the solid matrix, swelling of the solid matrix and dissolving the solids into the aqueous medium take place (Bhandari et al., Citation2013). In the present study, the drying temperature and pretreatments significantly affected the RC of the green mango powder (). The changes in RC of the pretreated powder were in the range of 4.12–4.58 while untreated powder 3.83–3.91. A significant increase in the RC observed while increasing drying temperature from 50 to 70°C than untreated powder in all the drying conditions. The powder’s rehydration capacity is influenced mainly by its physical characteristics such as particle size distribution, bulk density, porosity, and internal molecular structure. The results corroborated with Ramallo and Mascheroni (Citation2012) findings, who reported that the rehydration ratio increased with increasing the drying temperature from 45 to 75°C in convective hot-air drying of the pineapple.
pH
pH is one of the important chemical parameters in enhancing the post-harvest life of the fruits and vegetables. The pH values of the green mango powder were found in the range of 3.25–3.38 (). The drying temperature had no significant effect on pH value, whereas, among the pretreatments, ascorbic acid-treated powder showed significant higher pH value as compared to other treatments. This increase may be due to the diffusion of ascorbic acid into the green mango slices during pretreatment. The pH values were in good agreement with the value of 3.72 reported by Sogi et al. (Citation2015) for Tommy Atkin mango powder dried in a hot-air cabinet dryer at 60 ± 2°C, and 3.33 for mango slices dried in a tray dryer at 70°C (Dereje and Abera, Citation2020). However, Akther et al. (Citation2020) observed the pH value of 5.22 for Amropali mango powder dried in a cabinet dryer at 70°C. The pH values play an important role in shelf stability against microbial spoilage. It is directly associated with the concentration of organic acids, and changes in pH may occur during drying due to the removal of water and the degradation of organic acids (Dereje and Abera, Citation2020).
Total Soluble Solids
TSS content of dried green mango powder was found as high as 16.34 ºBrix as compared to fresh green mango slices (5.83 ºBrix). This increase in TSS due to a reduction in slice moisture during drying. No significant change in TSS content of pretreated green mango powder dried at 50, 60, and 70°C (), which indicates that pretreatment reduces TSS loss by inactivating the enzymatic activities. TSS is a primary dry matter component, indicating maximum sugar concentration and mainly composed of carbohydrates, organic acids, amino acids, and mineral salts (Dereje and Abera, Citation2020). The present study results were in confirmation with the findings of Dereje and Abera (Citation2020). They reported that the TSS content of mango slices, which untreated and treated with lemon juice, hot water blanching, and salt solution, and then dried in a tray dryer at 70°C reached 69.3, 69.27, 69.37, and 69.5 ºBrix, respectively, from an initial value of 11.59 ºBrix for fresh ripe mango slices. The TSS value of green mango powder in the present work lower than the findings of the literature. This reduction may be due to different cultivar and ripening stage; Dereje and Abera (Citation2020) used full ripe mangoes of ‘Keitt’ cultivar, whilst the present study used green mangoes of ‘Dasheri’ cultivar.
Ascorbic Acid
The green mango powder was analyzed for ascorbic acid as affected by pretreatments and drying conditions, and the findings are presented in . The result shows a significant reduction in ascorbic acid content while increasing drying temperature from 50 to 70°C. The ascorbic acid content recorded in the range of 34.17–34.68 mg/100 g at 50°C, 32.31–33.19 mg/100 g at 60°C and 29.81–31.16 mg/100 g at 70°C. This decreasing trend may be due to the oxidation and heat-sensitive nature of ascorbic acid (Brar et al., Citation2020). Besides, pretreatments had a significant effect on changes in ascorbic acid content. Under this drying condition, ascorbic acid pretreated powder showed significant higher ascorbic acid content (31.16 to 34.68 mg/100 g) as compared to blanching (30.02 to 34.24 mg/100 g) and calcium chloride (29.93 to 34.39 mg/100 g) pretreatments and control (29.81 to 34.17 mg/100 g). The diffusion of ascorbic acid into the green mango slices during ascorbic acid pretreatment may be the reason for this augmentation.
Similarly, a decrease in ascorbic acid for treated and untreated Yellow European plums drying at higher temperature (70°C) has reported by Brar et al. (Citation2020). Besides, ascorbic acid pretreated plum samples showed a higher ascorbic acid content as compared to citric acid and potassium metabisulfite pretreatments. Nyangena et al. (Citation2019) reported a significant increase in loss of vitamin C content of dried mango slices while increasing drying temperature from 50 to 65°C, and citric acid-treated samples retained more vitamin C than blanching and lemon juice treated sample and control.
Conclusion
The influence of pretreatments on drying kinetics and physicochemical properties of dried green mango powder was investigated. The sliced samples were treated with hot water blanching at 98°C for 3 min, immersed in 3% ascorbic acid and 3% calcium chloride solutions for 1 h at room temperature (30°C) dried in a hot air dryer at 50, 60, and 70°C. The drying process took place in the falling rate period, and the drying rate increased as the drying temperature increases. We tested four thin layer drying models (Page, Modified Page, Henderson and Pabis, and Logarithmic models) for their goodness of fit to describe the drying kinetics of green mango slices. The Logarithmic model found to be the best. The effective moisture diffusivities varied between 1.209 × 10−7 and 1.566 × 10−7 m2/s, and activation energies were in the range of 43.873–44.403 kJ/mol. The pretreatments improved the values of physical properties (bulk and tapped density, flowability, water solubility index, rehydration capacity) of the green mango powder, and drying temperature also significantly affected the physical properties. Pretreatments and the drying temperature did not affect pH and TSS. An increase in ascorbic acid content observed for samples treated with ascorbic acid, and samples treated with hot water blanching showed superior physical properties, whereas untreated samples exhibited inferior physicochemical quality.
Nomenclature
Acknowledgments
The authors wish to thank the Department of Food Process Engineering, Vaugh School of Agricultural Engineering and Technology, Sam Higginbottom University of Agriculture, Technology and Sciences, Uttar Pradesh, India and Department of Agricultural Engineering, Visva-Bharati University, West Bengal, India, for providing laboratory facilities and immense technical supports.
Disclosure Statement
The authors declare that they have no conflict of interest.
References
- Adepoju, L.A., and Z.D. Osunde. 2017. Effect of pretreatments and drying methods on some qualities of dried mango (Mangifera indica) fruit. Agric. Eng. Int: CIGR J 19(1):187–194.
- Afolabi, T.J., T.Y. Tunde-Akintunde, and J.A. Adeyanju. 2015. Mathematical modeling of drying kinetics of untreated and pretreated cocoyam slices. J. Food Sci. Technol 52(5):2731–2740. doi: https://doi.org/10.1007/s13197-014-1365-z.
- Agarry, S.E., A.O. Ajani, and M.O. Aremu. 2013. Thin layer drying kinetics of pineapple: Effect of blanching temperature-time combination. Nig. J. Basic Appl. Sci 21(1):1–10. doi: https://doi.org/10.4314/njbas.v21i1.1.
- Akther, S., A. Sultana, M.R. Badsha, M.M. Rahman, M.A. Alim, and A.M. Amin. 2020. Physicochemical properties of mango (Amropali cultivar) powder and its reconstituted product as affected by drying methods. Int. J. Food Prop. 23(1):2201–2216. doi: https://doi.org/10.1080/10942912.2020.1849278.
- Altendorf, S. 2019. Major tropical fruits market review 2017. FAO, Rome. 10 pp. Licence: CC BY-NC-SA 3.0 IGO.
- AOAC. 2000. Official methods of analysis. The Association of Official Analytical Chemists. 17th. Method 925.10. Gaithersburg, Md.: AOAC International, Maryland, USA.
- Aral, S., and A. Bese. 2016. Convective drying of hawthorn fruit (Crataegus spp.): Effect of experimental parameters on drying kinetics, color, shrinkage and rehydration capacity. Food Chem. 210:577–584. doi: https://doi.org/10.1016/j.foodchem.2016.04.128.
- Aregbesola, O.A., B.S. Ogunsina, A.E. Sofolahan, and N.N. Chime. 2015. Mathematical modeling of thin-layer drying characteristics of dika (Irvingia gabonensis) nuts and kernels. Niger. Food J. 33:83–89. doi: https://doi.org/10.1016/j.nifoj.2015.04.012.
- Aziz, N.A.A., L.M. Wong, R. Bhat, and L.H. Cheng. 2012. Evaluation of processed green and ripe mango peel and pulp flours (Mangifera indica var. Chokanan) in term of chemical composition, antioxidant compounds and functional properties. J. Sci. Food Agric. 92(3):557–563. doi: https://doi.org/10.1002/jsfa.4606.
- Bezerra, C., L. Silva, D. Correa, and A.A. Rodrigues. 2015. Modeling study for moisture diffusivities and moisture transfer coefficient in drying of passion fruit peel. Int. J. Heat Mass Tran. 85:750–755. doi: https://doi.org/10.1016/j.ijheatmasstransfer.2015.02.027.
- Bhandari, B., N. Bansal, M. Zhang, and P. Schuck. 2013. Handbook of food powders: Processes and properties. Woodhead publishing, Cambridge, UK.
- Brar, H.S., P. Kaur, J. Subramanian, G.R. Nair, and A. Singh. 2020. Effect of chemical pretreatment on drying kinetics and physicochemical characteristics of yellow European plums. Int. J. Fruit Sci 20(sup2):S252–S279. doi: https://doi.org/10.1080/15538362.2020.1717403.
- Chakraverty, A., and R.P. Singh. 2014. Postharvest technology and food process engineering. CRC Press, New York.
- Chegini, G.R., and B. Ghobadian. 2005. Effect of spray-drying conditions on physical properties of orange juice powder. Drying Technol. 23:657–668. doi: https://doi.org/10.1081/DRT-200054161.
- Chen, J., Y. Zhou, S. Fang, Y. Meng, X. Kang, X. Xu, and X. Zuo. 2013. Mathematical modelling of hot air drying kinetics of Momordica charantia slices and its color change. Adv. J. Food Sci. Technol 5(9):1214–1219. doi: https://doi.org/10.19026/ajfst.5.3085.
- Chowdhury, M.M.I., B.K. Bala, and M.A. Haque. 2011. Mathematical modeling of thin-layer drying of jackfruit leather. J. Food Process. Preserv 35(6):797–805. doi: https://doi.org/10.1111/j.1745-4549.2011.00531.x.
- Corzo, O., N. Bracho, and C. Alvarez. 2011. Determination of suitable thin layer model for air-drying of mango slices (Mangifera indica L.) at different air temperatures and velocities. J. Food Process Eng. 34:332–350. doi: https://doi.org/10.1111/j.1745-4530.2009.00360.x.
- Crank, J. 1975. The mathematics of diffusion. 2nd ed. Clarendon Press, Oxford, UK.
- Da Silva, W.P., C.M.D.P.S. E Silva, F.J.A. Gama, and J.P. Gomes. 2014. Mathematical models to describe thin-layer drying and to determine the drying rate of whole bananas. J. Saudi Soc. Agric. Sci. 13:67–74. doi: https://doi.org/10.1016/j.jssas.2013.01.003.
- Dehghannya, J., M. Pourahmad, B. Ghanbarzadeh, and H. Ghaffari. 2019. Heat and mass transfer enhancement during the foam-mat drying process of lime juice: Impact of convective hot air temperature. Int. J. Therm. Sci. 135:30–43. doi: https://doi.org/10.1016/j.ijthermalsci.2018.07.023.
- Deng, L.-Z., A.S. Mujumdar, Q. Zhang, X.-H. Yang, J. Wang, Z. Zheng, Z.-J. Gao, and H.-W. Xiao. 2019. Chemical and physical pretreatments of fruits and vegetables: Effects on drying characteristics and quality attributes - a comprehensive review. Crit. Rev. Food Sci. Nutr 59(9):1408–1432. doi: https://doi.org/10.1080/10408398.2017.1409192.
- Dereje, B., and S. Abera. 2020. Effect of pretreatments and drying methods on the quality of dried mango (Mangifera Indica L.). Slices. Cogent Food Agric. 6:1747961. doi: https://doi.org/10.1080/23311932.2020.1747961.
- Dinrifo, R.R. 2012. Effects of pre-treatments on drying kinetics of sweet potato slices. Agric. Eng. Int. CIGR J. 14:136–145.
- Doymaz, I. 2010. Evaluation of mathematical models for prediction of thin-layer drying of banana slices. Int. J. Food Prop. 13(3):486–497. doi: https://doi.org/10.1080/10942910802650424.
- Garba, U., S. Kaur, S. Gurumayum, and P. Rasane. 2015. Effect of hot water blanching time and drying temperature on the thin layer drying kinetics and anthocyanin degradation in black carrot (Daucus carota L.) shreds. Food Technol. Biotech 53(3):324–330. doi: https://doi.org/10.17113/ftb.53.03.15.3830.
- Goyal, R.K., A.R.P. Kingsly, M.R. Manikantan, and S.M. Ilyas. 2006. Thin-layer drying kinetics of raw mango slices. Biosyst. Eng. 95(1):43–49. doi: https://doi.org/10.1016/j.biosystemseng.2006.05.001.
- Grabowski, J., V.D. Truong, and C. Daubert. 2006. Spray-drying of amylase hydrolyzed sweet potato puree and physicochemical properties of the powder. J. Food Sci. 71(5):E209–E217. doi: https://doi.org/10.1111/j.1750-3841.2006.00036.x.
- Jafari, S.M., M.G. Ghalenoei, and D. Dehnad. 2017. Influence of spray drying on water solubility index, apparent density, and anthocyanin content of pomegranate juice powder. Powder Technol. 311:59–65. doi: https://doi.org/10.1016/j.powtec.2017.01.070.
- Junqueira, J.R.J., J.L.G. Correa, H.M. De Oliveira, R.I.S. Avelar, and L.A.S. Pio. 2017. Convective drying of cape gooseberry fruits: Effect of pretreatments on kinetics and quality parameters. LWT-Food Sci. Technol. 82:404–410. doi: https://doi.org/10.1016/j.lwt.2017.04.072.
- Kandasamy, P., N. Varadharaju, D.S. Dhakre, and S. Smritikana. 2019. Assessment of physicochemical and sensory characteristics of foam-mat dried papaya fruit powder. Int. Food Res. J. 26(3):819–829.
- Kumar, N., B.C. Sarkar, and H.K. Sharma. 2012. Mathematical modeling of thin layer hot air drying of carrot pomace. J. Food Sci. Technol 49(1):33–41. doi: https://doi.org/10.1007/s13197-011-0266-7.
- Lauricella, M., S. Emanuele, G. Calvaruso, M. Giuliano, and A. D’Anneo. 2017. Multifaceted health benefits of Mangifera indica L. (Mango): The inestimable value of orchards recently planted in sicilian rural areas. Nutrients. 9:525. doi: https://doi.org/10.3390/nu9050525.
- Malaikritsanachalee, P., W. Choosri, and T. Choosri. 2018. Study on kinetics of flow characteristics in hot air drying of pineapple. Food Sci. Biotechnol 27(4):1047–1055. doi: https://doi.org/10.1007/s10068-018-0357-6.
- Mazandarani, Z., N. Aghajani, A.D. Garmakhany, M.J.B. Ardalan, and M. Nouri. 2017. Mathematical modeling of thin-layer drying of pomegranate (Punica granatum L.) arils: Various drying methods. J. Agric. Sci. Technol 19:1527–1537.
- Mim, F.S., S.M. Galib, M.F. Hasan, and S.A. Jerin. 2018. Automatic detection of mango ripening stages: An application of information technology to botany. Sci. Hortic. 237:156–163. doi: https://doi.org/10.1016/j.scienta.2018.03.057.
- Narayana, C.K., B.K. Pandey, S.K. Malhotra, and V. Pandey. 2014. Technical bulletin on postharvest losses in selected fruits and vegetables in India (A compilation). ICAR-Indian Institute of Horticultural Research, Bengaluru, India. 25 Sep. 2019. https://iihr.res.in/sites/default/files/post%20harvestfinalcolour_0.pdf
- Nyangena, I.O., W.O. Owino, S. Imathiu, and J. Ambuko. 2019. Effect of pretreatments prior to drying on antioxidant properties of dried mango slices. Scientific African. 6:e00148. doi: https://doi.org/10.1016/j.sciaf.2019.e00148.
- Olanipekun, B.F., T.Y. Tunde-Akintunde, O.J. Oyelade, M.G. Adebisi, and T.A. Adenaya. 2015. Mathematical modelling of thin-Layer pineapple drying. J. Food Process. Preserv 39(6):1431–1441. doi: https://doi.org/10.1111/jfpp.12362.
- Onwude, D.I., N. Hashim, N.B. Janius, N.M. Nawi, and K. Abdan. 2016. Modeling the thin-layer drying of fruits and vegetables: A review. Compr. Rev. Food Sci. Food Saf. 15:599–618. doi: https://doi.org/10.1111/1541-4337.12196.
- Orikasa, T., L. Wu, T. Shiina, and A. Tagawa. 2008. Drying characteristics of kiwifruit during hot air drying. J. Food Eng. 85:303–308. doi: https://doi.org/10.1016/j.jfoodeng.2007.07.005.
- Prabhakararao, P.G., G. Narsingrao, A. Nagender, T. Jyothirmayi, and A. Satyanarayana. 2012. Standardization, chemical characterization and storage studies of an instant Pulihora mix based on raw mango. Indian J. Tradit. Know 11(1):90–95.
- Ramallo, L.A., and R.H. Mascheroni. 2012. Quality evaluation of pineapple fruit during the drying process. Food Bioprod Process. 90:275–283. doi: https://doi.org/10.1016/j.fbp.2011.06.001.
- Ranganna, S. 1986. Handbook of analysis and quality control for fruit and vegetable products. 2nd ed. Tata McGraw-Hill publishing company limited New Delhi, India.
- Rayaguru, K., and W. Routray. 2012. Mathematical modeling of thin-layer drying kinetics of stone apple slices. Int. Food Res. J. 19(4):1503–1510.
- Senadeera, W., B.R. Bhandari, G. Young, and B. Wijesinghe. 2003. Influence of shapes of selected vegetable materials on drying kinetics during fluidized bed drying. J. Food Eng. 58:277–283. doi: https://doi.org/10.1016/S0260-8774(02)00386-2.
- Sogi, D.S., M. Siddiq, and K.D. Dolan. 2015. Total phenolics, carotenoids and antioxidant properties of Tommy Atkin mango cubes as affected by drying techniques. LWT-Food Sci. Technol 62(1):564–568. doi: https://doi.org/10.1016/j.lwt.2014.04.015.
- Taşeri, L., M. Aktaş, S. Şevik, M. Gülcü, G.U. Seçkin, and B. Aktekeli. 2018. Determination of drying kinetics and quality parameters of grape pomace dried with a heat pump dryer. Food Chem. 260:152–159. doi: https://doi.org/10.1016/j.foodchem.2018.03.122.
- Udomkun, P., B. Mahayothee, M. Nagle, and J. Müller. 2014. Effects of calcium chloride and calcium lactate applications with osmotic pretreatment on physicochemical aspects and consumer acceptances of dried papaya. Int. J. Food Sci. Technol 49(4):1122–1131. doi: https://doi.org/10.1111/ijfs.12408.
- Vardin, H., and F.M. Yılmaz. 2018. The effect of blanching pre-treatment on the drying kinetics, thermal degradation of phenolic compounds and hydroxymethyl furfural formation in pomegranate arils. Ital. J. Food Sci. 30(1):156–169. doi: https://doi.org/10.14674/IJFS-947.
- Wang, Z., J. Sun, X. Liao, F. Chen, G. Zhao, J. Wu, and X. Hu. 2007. Mathematical modeling on hot air drying of thin layer apple pomace. Food Res. Int. 40:39–46. doi: https://doi.org/10.1016/j.foodres.2006.07.017.
- Yılmaz, F.M., and A.Z. Bastıoğlu. 2020. Production of phenolic enriched mushroom powder as affected by impregnation method and air drying temperature. LWT-Food Sci. Technol. 122:109036. doi: https://doi.org/10.1016/j.lwt.2020.109036.
- Zafar, T.A., and J.S. Sidhu. 2017. Composition and nutritional properties of mangoes, p. 217–236. In: M. Siddiq, J.K. Brecht, and J.S. Sidhu (eds.). Handbook of mango fruit: Production, postharvest science, processing technology and nutrition. John Wiley & Sons, New Jersey.