ABSTRACT
The present study evaluated the effect of pruning according to different tree forms on yield, fruit size, and leaf starch reserves of young ‘Nadorcott’ mandarin trees planted at high density. Trees were selectively pruned by hand either according to form (pyramid or open center) by removing either lateral or central branches, or were left unpruned until trees touched each other in the row with minimal pruning thereafter (control). Form pruning, in particular the pyramid form, resulted in higher amount of biomass removal compared with the control treatment, affecting canopy volume and trunk cross-sectional area (TCSA). This led to a yield reduction in the first year, but steady yield increases in subsequent years. Fruit size was significantly larger in pyramid and open-center-shaped trees compared with the control treatment, but this difference declined as trees got older. Production efficiency (yield/canopy volume) of the pyramid form was significantly higher after the second year of pruning compared with the other treatments. Pruning did not influence leaf starch content, but indirectly affected crop load and subsequently the following year’s crop size. Judicial removal of lateral branches, e.g. in pyramid-shaped trees, appeared to be the most beneficial tree form for ‘Nadorcott’ mandarin as it is closest to its natural growth habit and promotes sustainable yields with good marketable fruit size.
Introduction
Pruning is an important canopy management strategy to achieve and maintain optimal production with marketable fruit size and good fruit quality (Krajewski et al., Citation2021). For citrus, there are many different pruning strategies with equally different responses depending on species, tree age, tree vigor, fruiting habit, climatic conditions, and production practices (Tucker et al., Citation1994; Van der Merwe, Citation2012). The goal of pruning is to remove weak, dead, unproductive, and dense branches in order to improve light penetration, to develop and maintain a well-balanced tree structure with strong bearing branches that can support heavy crops of optimum-sized, high-quality fruit, and to optimize other cultural practices, such as pest and disease control, fertilization, servicing of irrigation systems, weed control, orchard sanitation, and harvest (Krajewski, Citation1996; Krajewski et al., Citation2021; Tucker et al., Citation1994). These goals can be achieved by pruning trees at the correct time (generally after harvest) and managing the vegetative regrowth. In addition, fruit thinning through pruning may add to the overall benefits of pruning (Krajewski et al., Citation2021). The increase in light interception and the associated beneficial changes in carbohydrate assimilation and microclimate are crucial aspects of pruning as these influence the emerging time and strength of vegetative shoots, flower intensity, as well as crop load and fruit quality parameters, such as fruit color and size (Davenport, Citation1990; Khurshid and Krajewski, Citation2010; Krajewski et al., Citation2021; Suzuki et al., Citation1973; Tucker et al., Citation1994; Verreynne et al., Citation2004). Tree form as well as pruning severity are crucial in this regard (Krajewski and Pittaway, Citation2000; Krajewski et al., Citation2021; Van der Merwe, Citation2012).
In order to be sustainable, the pruning method should fit the natural growth habit of the tree. In recent years, the late maturing mandarin cultivar, ‘Nadorcott’, has become very popular in South Africa because of its good eating quality and excellent bearing capacity. Additionally, ‘Nadorcott’ orchards are generally planted at high densities to maximize production. Particularly in the warm subtropical areas of South Africa, its natural growth habit is characterized by vigorous growth, the development of long, upright growing shoots, which have the tendency to hang over and restrict light in the lower canopy area, as well as a strong alternate bearing pattern (Van der Merwe, Citation2012). In high-density plantings, such growth habits make it challenging to maintain a balance between vegetative and reproductive growth (Krajewski, Citation1996; Krajewski et al., Citation2021; Tucker et al., Citation1994). In general, good yields are achieved in high-density orchards during the initial years, but early overcrowding restricts sustainable production in the long term (Tucker et al., Citation1994). Therefore, pruning of trees planted at high density should preferably start from a young age in order to avoid crop losses through overcrowding, which then necessitates heavy pruning to regain tree size, form, and productivity (Krajewski, Citation1996; Tucker et al., Citation1994).
So far, only limited research has been done on pruning of ‘Nadorcott’ mandarin grown under high density. Van der Merwe (Citation2012) studied the regrowth management of ‘Nadorcott’ trees planted at a high-density spacing of 5 × 2 m in South Africa by comparing pruning at flowering with summer pruning, while Mesejo et al. (Citation2020) evaluated mechanical pruning at flowering for ‘Nadorcott’ trees planted 5 × 7 m apart in Spain. However, both studies only looked at pruning strategies for mature ‘Nadorcott’ trees older than 10 years, and no studies could be found on pruning of young ‘Nadorcott’ trees. Due to the poor knowledge about adequate pruning strategies for young ‘Nadorcott’ mandarin, the current study was initiated. The aim of the study was to find the optimum tree form and suitable tree age when pruning should commence for young ‘Nadorcott’ trees grown under high density in order to maintain optimum fruit production, i.e. high yields with good, marketable fruit size.
Materials and Methods
Experimental Site and Design
The study was conducted on a commercial citrus farm in the warm subtropical production area near Nelspruit (Mpumalanga Province, South Africa; 31°E, 25°S; altitude 718 m; summer rainfall area) between 2014 and 2017. ‘Nadorcott’ mandarin trees (Citrus reticulata Blanco) grafted on ‘Carrizo Citrange’ (C. sinensis x Poncirus trifoliata) rootstock, which is the industry standard for ‘Nadorcott’ mandarin in South Africa, were used for the experiment. In order to prevent early overcrowding, 3-year-old trees in their first year of bearing were chosen for the current experiment, following the suggestion by Tucker et al. (Citation1994) and Krajewski (Citation1996) to start pruning trees in high-density orchards as early as possible. The trees were planted in a north–south orientation with a planting distance of 1.5 m × 5 m. All experimental trees were selected for uniformity of tree size and vigor, and subjected to the same conventional farming practices, i.e. adequate fertilizer, irrigation (drip irrigation), and pest and disease management.
Three treatments were applied immediately after harvest (July) and followed by regrowth management (water shoot thinning) in summer (January). The treatments were as follows:
Control (dome-like form; ): No pruning during the first experimental year. Removal of no more than three overhanging lateral branches during the second and third experimental year. This is consistent with current commercial pruning practices to avoid total overcrowding due to high density.
Open Center (open vase-like form; ): Removal of selected (up to three) upright inner branches and strong water shoots in all three experimental years to form and maintain an open center tree structure.
Pyramid (pyramid-like form; ): Removal of selected (up to three) lateral branches, poorly positioned branches, and strong water shoots in all three experimental years to form and maintain a pyramid shaped tree structure with a central leader.
The experiment was laid out in a randomized complete block design with 3 treatments and 10 blocks (i.e. 10 experimental units per treatment). Each experimental unit consisted of three trees, of which the middle tree was used for data collection. Each experimental unit was separated by buffer trees. The same trees were used every year.
Data Collection and Analysis
Following pruning and water shoot thinning (July and January, respectively), the weight of the removed branches was recorded using an electronic digital field scale. Pruning severity was quantified as the ratio of cumulative fresh weight of removed branches per unit of final trunk cross-sectional area (TCSA) as given by Robinson et al. (Citation1993) and was determined at the end of the trial period. Trunk circumference was measured at harvest 10 cm above the graft union. The TCSA was determined using the formula (TCSA = Girth2/4π) provided by Dalal and Brar (Citation2012). Canopy dimensions were determined at harvest by measuring tree height and canopy diameter. Canopy volume for the Control and Open Center trees was calculated using the equation suggested by Castle (Citation1983): Volume = 0.5236 x canopy height x (canopy diameter)2. Canopy volume for the pyramid-shaped trees was calculated using the equation for a pyramid: Volume = (in-row canopy length x between-row canopy width x canopy height)/3. At commercial maturity, fruits of each individual tree were harvested and weighed with an electronic digital field scale to determine yield. Due to the importance of fruit size for the market value of ‘Nadorcott’, only fruit size but no other quality parameters were determined. The fruit equatorial diameter of up to 100 fruits per tree, where available, was determined with an electronic digital caliper to determine fruit size. Production Efficiency (PE) for each treatment was calculated as: 1) the ratio of fruit yield per TCSA (PE1) (Robinson et al., Citation1993), and 2) the ratio of fruit yield per canopy volume (PE2) (Fallahi et al., Citation1990).
For the determination of leaf starch reserves, 30 randomly distributed leaves from vegetative shoots from the previous spring flush were collected per tree in the early morning, as described by Van der Merwe (Citation2012), at harvest (July), full bloom (September), fruit set (October), after fruit drop (November), after water shoot thinning in summer (January) and at flower induction (April). After sampling, the leaves were washed, oven dried (60°C), and ground to a fine powder. The samples were then analyzed for starch content using the iodine colorimetric method by Xu et al. (Citation1998).
Statistical Analysis
All data were subjected to analysis of variance (ANOVA) using the General Linear Models Procedure (PROC GLM) of SAS software (Version 9.4; SAS Institute Inc, Cary, USA). Where repeated measurements on the same experimental unit were taken, a split-plot analysis of variance with year as sub-plot factor was performed according to the methodology described by Montgomery (Citation2005) to compare treatment differences over time. The Shapiro–Wilk test on the standardized residuals from the model verified normality (Shapiro and Wilk, Citation1965). Levene’s test verified homogeneity of treatment variances (Levene, Citation1960). Tukey’s Studentized Range (HSD) was calculated at the 5% significance level to compare treatment means (Ott and Longnecker, Citation2010). Pearson product-moment correlation was performed using the correlation procedure (PROC CORR) of SAS software (Version 9.4; SAS Institute Inc, Cary, USA). Correlations with r-values higher than 0.5 (r > 0.5) were regarded as physiologically meaningful according to Verreynne (Citation2005).
Results and Discussion
Effect of Pruning on Regrowth, TCSA, and Canopy Volume
The total amount of biomass (June pruning and January water shoot removal combined) removed from the Open Center and Pyramid trees was higher than in the Control trees for the first 2 years as no or limited pruning was done on the Control trees. However, similar amounts of biomass were removed in all three treatments in the third year (). The 3-year mean for biomass removal indicated significantly higher biomass removal in the Pyramid trees compared with the Control trees, but showed similar (p > .05) biomass removal compared with the Open Center trees. These differences in pruning severity could be confirmed by the index for pruning severity, which was 0.30, 0.27, and 0.17 kg/cm2 final TCSA (p = .0007) for the Pyramid, Open Center, and Control treatment, respectively.
Table 1. Effect of pruning treatments on removed biomass, trunk cross-sectional area (TCSA), and canopy volume of young ‘Nadorcott’ trees between 2014 and 2017
Table 2. Effect of pruning treatments on yield, production efficiency (PE) and fruit diameter of young ‘Nadorcott’ trees between 2014 and 2017
Form pruning had an impact on vegetative regrowth and was expressed as removed biomass in January in . The Open Center and Pyramid treatments showed stronger regrowth responses due to more severe pruning compared with the Control. However, while yearly regrowth remained rather stable in the Pyramid trees despite increasing biomass removal after each harvest, regrowth in the Open Center trees increased year by year despite the removed biomass after harvest being lower in 2014 and 2016, compared with 2015. Similarly, the amount of regrowth in the Control trees increased each year and proportionally to the amount of biomass removed after harvest. This interaction between biomass removal and regrowth was also reflected in the correlation between biomass removal and canopy volume, which was significantly positive for the Control and Open Vase trees but lacking for the Pyramid trees (). Nevertheless, the Control trees showed the least regrowth in all years compared with the other treatments ( and B). The intensity of regrowth response in the present study may be due to a combination of natural growth habit of the ’Nadorcott’, the pruning cut position and its resulting changes in light distribution. By opening up the tree center, the regrowth response of the Open Vase treatment was mainly observed in the center, which intensified with time as branching complexity and density in the canopy periphery increased (Krajewski, Citation1996). In contrast, the Pyramid treatment focused on the removal of lateral branches thus allowing the light to penetrate the canopy at an angle, promoting the growth of lateral bearing branches and maintaining regrowth at a similar level throughout the trial period ( and B). Although light interception could not be measured, removal of lateral branches as done in the Control and Pyramid treatments appeared to accommodate the natural growth habit of ‘Nadorcott’ trees better under high-density conditions compared with open center pruning. This was in contrast to the growth habits of other citrus species, e.g. sweet orange, which benefited more from the removal of central leader branches to form an open-center tree (Ghosh and Bera, Citation2014).
Table 3. Pearson’s correlation coefficients (r-values) for removed biomass and canopy volume correlated with various yield-related parameters (3 years of data combined)
Figure 2. Effect of pruning treatments on biomass removal of young ‘Nadorcott’ trees for the entire trial period for: A) pruning after harvest in July and B) water shoot thinning in January. Bars indicate mean values ± SE (n = 10). Different letters above columns indicate significant differences among treatments and pruning times at p = .05 according to Tukey’s Studentized Range test
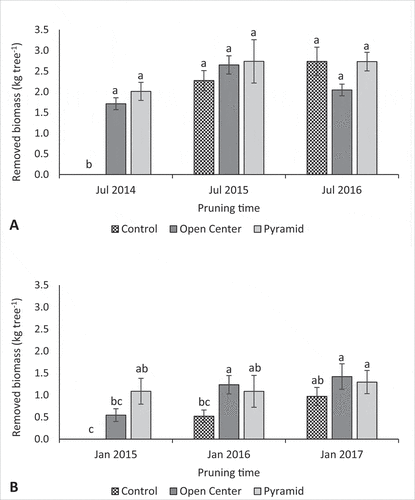
As expected, TCSA increased each year for all treatments as the trees grew bigger. This was also confirmed with highly significant and positive correlations between canopy volume and TCSA for all treatments across the years () and confirms a well-established relationship between efficient water and nutrient supply for canopy growth and fruit production, which can be found in many tree species (Dalal and Brar, Citation2012; Robinson et al., Citation1993; Westwood and Roberts, Citation1970; Wright et al., Citation2006). Although no significant treatment effects and year × treatment interactions in TCSA were found, TCSA were overall smaller in the Pyramid and Open Center trees compared with the Control trees (). TCSA increases were significantly affected by year across all treatments (2015–16: 28.3%; 2016–17: 10.8%; p < .05) and revealed that tree growth slowed down as the trial continued. The total TCSA increase (2015–17) was considerably smaller for the Pyramid treatment with 36.1%, compared with 45.4% in the Control treatment (p < .1) and 45.1% in the Open Vase treatment (p > .1). This indicates that severe pruning, like in the Pyramid treatment, suppresses tree trunk growth compared with lighter pruning. Similar trends in TCSA were found in studies with different pruning severities in cherry and peach (Ikinci et al., Citation2014; Kappel et al., Citation1997). Contrary to this, Pearson product-moment correlation performed on the individual treatments revealed highly significant positive correlations between removed biomass and TCSA which suggests that the vegetative growth stimulated by pruning contributed to a TCSA expansion within each treatment () (Robinson et al., Citation1993; Tucker et al., Citation1994). This so-called response to compensatory growth is in agreement with the positive correlation between the vigor of new growth and the severity of pruning found by Bevington (Citation1980) on Valencia oranges.
Similarly, canopy volume increased over the entire trial period regardless of treatment. However, canopy volumes between treatments differed significantly both for individual years and for the 3-year mean (). As a result of tree form and the subsequent amount of removed biomass after harvest, the Pyramid trees had the lowest canopy volume in all 3 years, followed by the Open Center and Control trees ().
Effect of Pruning on Yield and Production Efficiency
The effect of pruning on crop load was most visible after the first year’s pruning, where the Pyramid and Open Center trees showed significantly lower yields compared with the Control trees (). This was also confirmed by a highly significant negative correlation between removed biomass and yield in that particular year (r = −0.68446; p < .0001). After the second and third season’s pruning, the Pyramid and Control trees showed the highest yields, respectively (p > .05) (). The 3-year mean yield was highest for the Control trees, followed the Pyramid trees and the Open Center trees. These results agree with studies by Tucker et al. (Citation1994), Krajewski (Citation1996), and Krajewski and Pittaway (Citation2000) who found that the severity and type of pruning causes changes in crop load in citrus. Likewise, Hamdy (Citation2018) could show that heavy pruning of 5-year old ‘Murcott’ and ‘Fremont’ mandarin trees planted at high density, reduced yield compared with light or medium pruning and the untreated control. In contrast, Ahmad et al. (Citation2006) demonstrated that heavy pruning of 12-year old ‘Kinnow’ mandarin increased yield and fruit quality compared with light or no pruning. From the above mentioned literature, it becomes clear that the response to pruning in mandarin differs between young and mature trees and depends on pruning severity and method (i.e. type and position of branches removed) as well as the resulting changes in light interception before and after pruning. Hence, the changes in crop load as a result of pruning form, pruning severity, and increasing tree age may have been the reason for the treatment differences observed over the trial period in the present study. Interestingly, correlations between yield and canopy volume only showed positive correlations (p < .01) for the Pyramid trees, which may indicate and confirm a possible benefit of the pyramid form for fruit production of ‘Nadorcott’ ().
When reviewing yield changes within treatments over time, it was evident that the Pyramid and Open Center treatments showed a steady yield increase of 10–35% year after year, whereas the Control treatment showed signs of alternate bearing with two “on”-years and one “off”-year (). Despite the lower yield in the first year, form pruning from the first year of bearing may have delayed the onset of alternate bearing. This is in line with suggestions by Tucker et al. (Citation1994) and Khurshid and Krajewski (Citation2010) who recommended heavier pruning if an “on”-year is expected in order to alleviate alternate bearing in the following years, although these authors were referring to older trees. Nevertheless, more years of study are necessary for sound conclusions in this regard.
Production efficiencies for each treatment were calculated for a more representative comparison of treatments. Production efficiency 1 (PE1), defined as yield per TCSA, is most commonly used in horticultural studies (Robinson et al., Citation1993). In the current study, PE1 ranged on average between 1.2 and 1.4 kg/cm2 and did not show any significant difference between treatments except in the first year, where the Control treatment exhibited significantly higher PE1 compared with both the Open Center and Pyramid treatments (). The lack of differences in PE1 can be explained by the lack of differences in TCSA and yield. In a study on different lemon cultivars, Fallahi et al. (Citation1990) stated that the production efficiency expressed as yield per TCSA is not always a good indicator if TCSA is not proportional to the canopy volume. Although positive correlations between TCSA and canopy volume were found across treatments and years (r = 0.66245; p < .0001) and for the individual treatments across the 3-year period (), no correlations were found for each individual year (data not shown). Therefore, Production Efficiency 2 (PE2) (yield per canopy volume) provided a better indication because pruning strongly affected the canopy volume of each treatment. The PE2 was highest in the first year, ranging between 28 and 39 kg/m3 across treatments but decreased in the following years (). In all 3 years and the mean over 3 years, the Pyramid treatment showed the highest PE2 compared with the other treatments. This suggests that the pyramid shape appears to be the most efficient tree form for ‘Nadorcott’, most likely because it accommodates the natural growth habit of this cultivar better than the more roundish tree forms of the other treatments, resulting in more even and stable regrowth (as seen in ) and possibly better carbon partitioning to the fruit. In support of our findings, Joubert et al. (Citation2000) showed that a pyramid-like form improved light penetration and promoted the development of evenly distributed regrowth in oranges and grapefruit resulting in improved production. Assessment of light penetration could have confirmed the relationship between light and regrowth distribution in the recent study and should be included in future studies.
Effect of Pruning on Fruit Size
Fruit size plays an important role in the marketability and profitability of ‘Nadorcott’ mandarin and larger fruits are of higher commercial value. The Control trees had the lowest fruit diameters in most years and mean over 3 years compared with other treatments due to their higher crop load (). In contrast, lower crop load in the Pyramid and Open Center trees resulted in significantly larger and therefore more marketable fruit in the first year and mean over 3 years. This relationship between yield and fruit size was confirmed by highly significant negative correlations in 2015 (r = −0.66519; p < .0001) and over the 3-year mean (r = −0.67300; p < .0001). Furthermore, the effect of pruning on yield and subsequently on fruit size was also demonstrated by the highly significantly positive correlation between the 3-year mean biomass removal and the 3-year mean fruit size (r = 0.61626; p = .0003). The inverse relationship between yield and fruit size is a common and well-known phenomenon in many fruit tree species, but in particular in citrus (Guardiola and García-Luis, Citation2000; Khurshid and Krajewski, Citation2010; Krajewski and Pittaway, Citation2000; Martin-Gorriz et al., Citation2014). Predominantly cultivars with alternate bearing patterns will typically produce a large number of fruit with very small fruit size of low commercial value in “on”-years and a very small number of very large unattractive fruit in “off”-years (Verreynne and Lovatt, Citation2009). Although the trees in the current study were still young, this phenomenon could already be observed in the Control trees (). The fact that there were only slight treatment differences in fruit size in the second and third years can be attributed to fewer yield differences (). This may indicate that pruning has a bigger effect on fruit size at the beginning of an orchard life or in young trees compared with established orchards, similar to references made earlier on the effect of pruning severity on crop load in young and mature mandarin trees (Ahmad et al., Citation2006; Hamdy, Citation2018). Assessment of additional fruit quality parameters, such as color, total soluble solids, and titratable acid, could possibly have revealed stronger effects of pruning form on final crop value compared with fruit size alone, although the pruning study by Van der Merwe (Citation2012) on ‘Nadorcott’ could not show pruning effects on other fruit quality parameters.
Effect of Pruning on Leaf Starch
The ‘Nadorcott’ trees displayed distinct patterns of leaf starch accumulation and mobilization throughout each growing season (). Neither pruning itself nor the regrowth as a result of pruning appeared to have a direct effect on leaf starch during the period between pruning and early fruit growth, when most of the regrowth generally occurs (). The lack of correlations between removed biomass and leaf starch or between regrowth and leaf starch (data not shown) also suggests that pruning did not have an effect on leaf starch levels. Van der Merwe (Citation2012) also could not find any significant effect of pruning or shoot thinning on leaf carbohydrate levels of ‘Nadorcott’ mandarin in South Africa, and concluded that pruning has little or no effect on leaf carbohydrate levels. Nevertheless, pruning can have an indirect effect on tree carbohydrate reserves by influencing tree structure and light interception. As such, pruning influences the type of bearing branches as well as crop load, which then leads to changes in carbohydrate reserves (Khurshid and Krajewski, Citation2010; Krajewski, Citation1996). This also seemed to be the case in the current study. The high yield of the Control tree in June 2015 caused a depletion of leaf starch, compared with the Pyramid and Open Center trees whose lower yields resulted in much higher leaf starch levels at harvest (; ). Similarly, at harvest in July 2016, leaf starch was the lowest for the two highest yielding treatments, i.e. Pyramid and Control treatment. In 2017, differences between high and low yielding treatments were only observed during rapid fruit growth, but not at harvest. These trends were confirmed by significant negative correlations between leaf starch at harvest (June/July) and yield for all 3 years (). Similarly, Monselise and Goldschmidt (Citation1982) found that “on”-year trees of ‘Wilking’ mandarin that were completely de-fruited in summer had higher leaf starch concentration by the next spring compared with “on”-year trees that were only hand thinned. In addition, a highly significant positive correlation between leaf starch in June 2015 (harvest) and fruit size in June 2015 () demonstrates the importance of adequate carbohydrate reserves in leaves and twigs for good, marketable fruit size, and that regulating carbohydrate limitations, e.g. by improving light interception through pruning among others, can be beneficial (Goldschmidt, Citation1999; Ruiz et al., Citation2001).
Table 4. Pearson’s correlation coefficients and significance levels of various tree parameters between 2015 and 2017
Figure 3. Effect of pruning treatments on percentage leaf starch content of young ‘Nadorcott’ trees at various phenological growth stage between June 2015 and July 2017. Columns indicate mean values ± SE (n = 10). Statistical significance at p = .05 is indicated as a HSD bar according to Tukey’s Studentized Range test
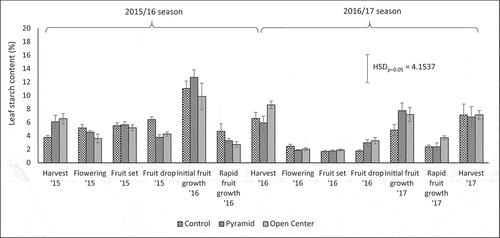
Carbohydrate reserves, in particular starch, play an important role in the expression level of alternate bearing (discussed by Van der Merwe, Citation2012). Although the current study was conducted on young trees, the first signs of alternate bearing could be observed in the Control treatment, as mentioned above and shown in . The depletion of starch reserves as a result of a high crop load in the Control treatment in the first year of bearing was most likely the reason for these trees to enter an “off”-year in the following year. According to Mazhar et al. (Citation2007), pruning is the most common method to address heavy crop load associated with small, unmarketable fruit size and carbohydrate depletion in citrus. By limiting crop load in the first year of bearing through pruning, i.e. the Open Center and Pyramid treatments, a strong imbalance between crop load and starch levels was avoided, and therefore the onset of alternate bearing was prevented or at the very least delayed. Various studies on citrus have stated that the interaction between crop load, carbohydrate levels, and alternate bearing needs to be considered when trying to maintain the balance between vegetative and reproductive growth (García-Luis et al., Citation1988; Goldschmidt, Citation1999; Iglesias et al., Citation2003; Verreynne and Lovatt, Citation2009). This is particularly important for pruning. For a cultivar like ‘Nadorcott’, which is known for its strong alternate bearing habit, early pruning of young trees in combination with modifying the tree form as in the current study, might therefore be a key to reduce its alternate bearing pattern.
Conclusion
In light of the presented results, this study showed that no or minimal pruning (Control), as is currently done commercially for ‘Nadorcott’ mandarin, achieved the highest yields over the entire trial period. Nevertheless, this was to the detriment of leaf carbohydrate reserves and good, marketable fruit size. In addition, it appeared to encourage alternate bearing by allowing a high crop load in the first year of bearing. In contrast, the removal of central branches (Open Center treatment) caused an increasing amount of regrowth with dense branch development in the canopy periphery, which caused lower yields and thus appeared to be a less beneficial tree form for ‘Nadorcott’ mandarin. Form pruning based on a pyramid shape removed the highest amount of biomass, but maintained stable regrowth throughout the trial period and had the highest production efficiency (PE2) compared with the other treatments. In addition, the pyramid form was easy to maintain after the first year of pruning. These results seem to provide sufficient evidence that a pyramid-like tree form is the most beneficial tree form for ‘Nadorcott’ mandarin as it is closest to its natural growth habit, accommodates for its strong vigor, and ensures sustainable yields with optimal fruit size. Therefore, judicious removal of lateral branches from the first year of bearing may be an adequate pruning strategy for ‘Nadorcott’ mandarin planted under high densities. In order to minimize the yield reduction in the first year, it may, however, be sensible to prune less severely than in the Pyramid treatment. In addition, assessment of fruit quality parameters are necessary for a complete and conclusive statement on the effect of pruning form on final crop value.
Acknowledgments
This study was supported by the Citrus Research International Pty (Ltd) and the Agricultural Research Council of South Africa. The authors would like to thank Indigo Fruit Farming for their on-farm support of the experiment and Dr Mardé Booyse from the ARC Biometry Unit for her assistance with statistical analysis. Dr Elliosha Hajari is thanked for critical reviewing of the manuscript.
Additional information
Funding
References
- Ahmad, S., Z.A. Chatha, M.A. Nasir, A. Aziz, N.A. Virk, and A.R. Khan. 2006. Effect of pruning on the yield and quality of Kinnow fruit. J. Agric. Social Sci. 2(1):51–53.
- Bevington, K.B. 1980. Response of Valencia orange trees in Australia to heading and topping. Proc. Fla. State Hort. Soc. 93:65–66.
- Castle, W. 1983. Growth, yield and cold hardiness of seven year old ‘Bearss’ lemon on twenty seven rootstocks. Proc. Fla. State Hort. Soc. 96:23–25.
- Dalal, R.P.S., and J.S. Brar. 2012. Relationship of trunk cross-sectional area with growth, yield, quality and leaf nutrient status in Kinnow mandarin. Indian J. Hort. 69(1):111–113.
- Davenport, T.L. 1990. Citrus flowering, p. 349–408. In: J. Janick ed.. Horticultural Reviews. Vol. 12. Timber Press, Portland, Oregon.
- Fallahi, E., D.R. Rodney, and Z. Mousavi. 1990. Growth, yield and fruit quality of eight lemon cultivars in Arizona. J. Am. Soc. Hort. Sci. 115(1):6–8. doi: https://doi.org/10.21273/JASHS.115.1.6.
- García-Luis, A., F. Fornes, A. Sanz, and J.L. Guardiola. 1988. The regulation of flowering and fruit set in citrus: Relationship with carbohydrate levels. Isr. J. Bot. 37:189–201. doi: https://doi.org/10.1080/0021213X.1988.10677099.
- Ghosh, S.N., and B. Bera. 2014. Effect of pruning on productivity in sweet orange – Short communication. J. Hort. Sci. 9(2):206–208.
- Goldschmidt, E.E. 1999. Carbohydrate supply as a critical factor for citrus fruit development and productivity. HortSci 34(6):1020–1024. doi: https://doi.org/10.21273/HORTSCI.34.6.1020.
- Guardiola, J.L., and A. García-Luis. 2000. Increasing fruit size in Citrus. Thinning and stimulation of fruit growth. Plant Growth Reg. 31:121–132. doi: https://doi.org/10.1023/A:1006339721880.
- Hamdy, A.E. 2018. Effect of pruning severity on yield and fruit quality of two mandarin cultivars. Acta. Hort. 1216:135–143. doi: https://doi.org/10.17660/ActaHortic.2018.1216.17.
- Iglesias, D., F.R. Tadeo, E. Primo-Millo, and M. Talon. 2003. Fruit set dependence on carbohydrate availability in citrus trees. Tree Physiol. 23:199–204. doi: https://doi.org/10.1093/treephys/23.3.199.
- Ikinci, A., A. Kuden, and B.R. Ak. 2014. Effects of summer and dormant pruning time on the vegetative growth, yield, fruit quality and carbohydrate contents of two peach cultivars. Afr. J. Biotech. 13(1):84–90. doi: https://doi.org/10.5897/AJB09.1614.
- Joubert, F.J., M.H. Du Plessis, and P.J.C. Stassen. 2000. Pruning strategies to alleviate overcrowding in higher density citrus orchards. J. Appl. Hort. 2(1):1–5.
- Kappel, F., M. Bouthillier, and R. Brownlee. 1997. Summer pruning young ‘Sweetheart’ sweet cherry trees affects yield and tree size. HortSci 32(6):1034–1036. doi: https://doi.org/10.21273/HORTSCI.32.6.1034.
- Khurshid, T., and A. Krajewski. 2010. Bearing branch units developed on branches hedged during flowering produce large ‘Washington’ Navel (Citrus sinensis L. Osbeck) oranges. Int. J. Fruit Sci. 10:215–227. doi: https://doi.org/10.1080/15538362.2010.510417.
- Krajewski, A. 1996. Pruning of citrus in Southern Africa: A hacker’s guide. Citrus J. 6(4):19–23.
- Krajewski, A., and T. Pittaway. 2000. Manipulation of citrus flowering and fruiting by pruning. Proc. Int. Soc. Citric. 1:357–360.
- Krajewski, A., A. Schumann, T. Ebert, C. Oswalt, R.S. Ferrarezi, and L. Waldo. 2021. Management of citrus tree canopies for fresh-fruit production. Dept. Soil Water Sci. UF/IFAS Extension, document SL485/SS698, 1/2021. doi: https://doi.org/10.32473/edis-ss698-2021.
- Levene, H. 1960. Robust test in the equality of variance, p. 278–292. In: I.P. Alto (ed.). Contributions to probability and statistics. Stanford University Press, California, USA.
- Martin-Gorriz, B., I.P. Castillo, and A. Torregrosa. 2014. Effect of mechanical pruning on the yield and quality of ‘Fortune’ mandarins. Span. J. Agric. Res. 12(4):952–959. doi: https://doi.org/10.1590/S1413-70542008000100015.
- Mazhar, M.S., R. Anwar, and M. Maqbool. 2007. A review of alternate bearing in citrus, p.143–150. In: Proceedings of the International Symposium on Prospects of Horticultural Industry in Pakistan, Faisalabad, Pakistan, 28–30 March 2007. Institute for Horticultural Sciences, University of Agriculture, Faisalabad, Pakistan.
- Mesejo, C., A. Martínez-Fuentes, C. Reig, S. Balasch, E. Primo-Millo, and M. Agustí. 2020. Mechanical pruning attenuates alternate bearing in ‘Nadorcott’ mandarin. Sci. Hort. 261:108993. doi: https://doi.org/10.1016/j.scienta.2019.108993.
- Monselise, S.P., and E.E. Goldschmidt. 1982. Alternate bearing in fruit trees. Hort. Rev. 4:128–173.
- Montgomery, D.C. 2005. Design and analysis of experiments. 6th ed. John Wiley & Sons, USA, Hoboken, NJ.
- Ott, R.L., and M. Longnecker. 2010. An introduction to statistical methods and data analysis. Brooks/Cole, Cengage Learning, Belmont, USA.
- Robinson, T.L., J. Wünsche, and A. Lakso. 1993. The influence of orchard system and pruning severity on yield, light interception, conversion efficiency, partitioning index and leaf area index. Acta. Hort. 349:123–128. doi: https://doi.org/10.17660/ActaHortic.1993.349.17.
- Ruiz, R., A. García-Luis, C. Monerri, and J.L. Guardiola. 2001. Carbohydrate availability in relation to fruitlet abscission in Citrus. Ann. Bot. 87:805–812. doi: https://doi.org/10.1006/anbo.2001.1415.
- Shapiro, S.S., and M.B. Wilk. 1965. An analysis of variance test for normality (complete samples). Biometrika. 52:591–611. doi: https://doi.org/10.1093/biomet/52.3-4.591.
- Suzuki, T., S. Okamoto, and T. Seki. 1973. Effects of micro-meteorological elements and positions in the tree crown on the development of shoots, leaves and fruits of Satsuma mandarin. J. Jpn. Soc. Hort. Sci. 42(3):201–2019. doi: https://doi.org/10.2503/jjshs.42.201.
- Tucker, D.P.H., T.A. Wheaton, and R.P. Muraro. 1994. Citrus tree pruning principles and practices. Fact Sheet HS-144. Horticultural Sciences Dept., Florida Cooperative Extension Service, Institute of Food and Agricultural Sciences, University of Florida, Gainesville, Florida, USA.
- Van der Merwe, I.S. 2012. Studies on the phenology and carbohydrate status of alternate bearing ‘Nadorcott’ mandarin trees. MSc thesis. University of Stellenbosch, South Africa.
- Verreynne, J.S. 2005. The mechanism and underlying physiology perpetuating alternate bearing in ‘Pixie’ mandarin (Citrus reticulata Blanco). University of Stellenbosch, South Africa, PhD thesis.
- Verreynne, J.S., and C.J. Lovatt. 2009. The effect of crop load on budbreak influences return bloom in alternate bearing ‘Pixie’ mandarin. J. Am. Soc. Hort. Sci. 134:299–307. doi: https://doi.org/10.21273/JASHS.134.3.299.
- Verreynne, J.S., E. Rabe, and K.I. Theron. 2004. Effect of bearing position on fruit quality of mandarin types. South Afr. J. Plant Soil 21(1):1–7. doi: https://doi.org/10.1080/02571862.2004.10635014.
- Westwood, M.N., and A.N. Roberts. 1970. The relationship between trunk cross-sectional area and weight of apple trees. J. Am. Soc. Hort. Sci. 95:28–30.
- Wright, H., D. Nicols, and C. Embree. 2006. Evaluating the accountability of trunk size and canopy volume models for determining apple tree production potential across diverse management regimes. Acta. Hort. 707:237–243. doi: https://doi.org/10.17660/ActaHortic.2006.707.30.
- Xu, C.J., W.J. Chen, K.S. Chen, and S.L. Zhang. 1998. A simple method for determining the content of starch – Iodine colorimetry. Biotechnology 8(2):41–43.