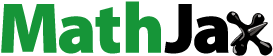
ABSTRACT
Peach is an important fruit; however, its perishability limits its availability to the consumers. The present study fosters a low-cost preservation method of peach involving osmotic dehydration at 35–45◦C using a sugar solution (50–70◦Brix) for 60–180 minutes. The conditions for osmotic dehydration (OD) of peach slices in terms of maximum water loss (WL), rehydration ratio (RR), minimum solute gain (SG) with optimum hardness, and maximum overall acceptability (OA) were optimized using Box-Behnken Design (BBD) of response surface methodology (RSM). The regression coefficient of the models indicated that the time of immersion was the most significant (p < .001) factor for WL and SG, followed by the temperature. Furthermore, immersion time had a positive effect on hardness, whereas it had a negative effect on the organoleptic properties of osmo-dried peach slices. Sucrose concentration of 63.20◦Brix for 133 minutes at 41◦C was most suitable for osmotic dehydration of peach slices to obtain maximum possible WL, minimum SG, maximum RR, optimum hardness, and maximum overall acceptability. Combined drying (OD+CAD) significantly reduced the drying time and had better retention of sensory attributes. The peach slices subjected to a combination of OD+CAD had good retention (45–50%) of bioactive components even at ambient temperature and had an overall acceptability score of above 6.0 with a 6-months shelf life of the product. The technology was demonstrated among the small-scale processors and entrepreneurs and based on the response received, more than 90% highly rated this technique as efficient, energy-conserving, and easy to adopt.
Introduction
Fruits are relished by everyone, and they are the main source of micronutrients in the human diet. Peaches are popular for their unique flavor and taste. From a nutritional point of view, peaches are an excellent source of vitamin A and C as well as rich in potassium, niacin, copper and manganese. Peaches are mainly cultivated in warm temperate and subtropical region. Shan-e-Punjab variety of peach ripens in early May with its fresh fruit quite big in size, succulent, yellow-colored with reddish blush. High moisture content of peaches leads to shorter shelf life; processing is the only option for their preservation for long-term use. Fruit juice concentrates are being adopted as osmotic agent in combination with microwave – vacuum drying commercially for more product attractiveness (Kowalska et al., Citation2020; Samborska et al., Citation2019). However, in developing countries like India, low cost and simple preservative techniques are more viable for the farming community and small entrepreneurs. Moreover, the fruit juice concentrates are not cost-effective and need to preserved at low temperatures as these are very sensitive to microbial contamination. The most common, oldest and low-cost method of preservation of food is drying (Berk, Citation2018). For perishable fruits like peach osmotic dehydration (OD) is the best among other drying methods, which involves partial dehydration of vegetables and fruits by immersion in a hypertonic solution of sugar or salt before drying (Rastogi et al., Citation2014). Many factors such as concentration of the osmotic agent, time and temperature, shape and tissue of plant material are important for optimizing the conditions for osmotic dehydration. Osmotic dehydration is not only a low-cost technology; it is also preferable pre-treatment for further drying. Earlier studies with various fruits and vegetables such as apple (Koprivica et al., Citation2014), mango (Madamba and Lopez, Citation2002), banana (Fernandes et al., Citation2006), guava (Vieira et al., Citation2012), pineapple (Zapata Montoya et al., Citation2011), cherry tomato (Derossi et al., Citation2015), carrots (Singh et al., Citation2010), green pepper (Ozdemir et al., Citation2008), yam beans (Abud et al., Citation2008) pear (Da Costa Ribeiro et al., Citation2016), ginger (Siqueira et al., Citation2019) etc. demonstrate successful use of osmotic dehydration for their preservation with retention of nutritional components, color, flavor, vitamin and overall preserve the wholesomeness of the food.
Osmotic dehydration involves three types of mass transfer, water and solutes such as sugar, acid, mineral, vitamins, etc., from the food in the osmotic solution reverse the osmotic solutes enter in the food. The commonly used solutes are salt and sugar (Ahmed et al., Citation2016; Luchese et al., Citation2015; Park et al., Citation2002). Conventional drying is the most common and economical method to combine with osmotic dehydration (Tola and Ramaswamy, Citation2010). In a study, it was observed that keeping the fruits and vegetables in osmotic solution (40◦Brix) for a time period of about 132 minutes resulted in good-quality osmotic dried product (Yadav and Singh, Citation2014).
Before using any technique, optimization is essential for technology development to get an acceptable product with desired quality parameters. The response surface methodology (RSM) is a statistical tool for optimization reported by many authors (Koprivica et al., Citation2014; Krokida and Philippopoulos, Citation2005) and different varieties of same fruit respond differentially to osmotic dehydration. The present study aimed to optimize the level of sucrose, time and temperature for osmotic dehydration of peach slices using RSM for attaining maximum water loss (WL) (%) and rehydration ratio with minimum solute gain (SG) (mg/100 g), optimum hardness (N) and maximum overall acceptability in terms of sensory attributes in the final product for commonly cultivated Shan-e-Punjab variety of peach. Further, the study compares drying time and sensory qualities of peach dried with combination of OD and CAD with only CAD dried samples. OD+CAD dried sample was stored for 6 months at ambient and refrigerated temperature for evaluating shelf life of peach slices. The optimized peach drying technology was demonstrated among small-scale peach processors and entrepreneurs in a five-day training programme. The participants were asked to rate the technology to evaluate its commercial viability.
Materials and Methods
Sample Preparation
Fresh matured under-ripe peaches each weighing 50–55 g of Shan-e-Punjab cultivar were procured from the orchard of Regional Research Station, Bathinda and stored for a day at 4–7°C in a refrigerator. Following washing the fruits were pricked, destoned and sliced into pieces (20 × 20 × 5 mm) for immersing in different osmotic solutions. Fruit slices weighing 60 g were dipped in 250 ml of sucrose solution of different °Brix as per the designed experiment with approximately 1:4 ratio of fruit to the osmotic solution. The pre- and post-treatment moisture content of peach was calculated using AOAC method (AOAC, Citation1991) and the initial moisture content in fresh fruit was 88.5 ± 1.0%.
Osmotic Solutions Preparation
Developing nations like India needs economical processing techniques. Thus, sucrose is widely used for preservation instead of fruit concentrates as it is economical, easy to handle, readily available and has widely acceptable taste. Moreover, fruit juice concentrates are expensive compared to sucrose, and they need storage facilities at low temperatures to avoid microbial spoilage, which leads to additional costs. Sucrose solutions (three replicates) varying between 50–70◦Brix were prepared from food grade sugar as osmotic solution. The peach slices were immersed in osmotic solutions according to the time and temperature combination designed by the response surface methodology. The known quantity of peach fruit (60 g) was immersed in the osmotic solutions with various concentrations preset at the desired temperature in a water bath. These slices were removed from the solutions at particular time intervals followed by blotting up with absorbent paper.
Experimental Design
The response pattern and optimum combinations of variables was studied using Box-Behnken Design (BBD) of Response Surface Methodology (RSM) with three independent variables at three different levels. Trial version of Design expert 12 (statease.com) was used to apply RSM to the experimental data, and 17 runs were designed using this software with details of various responses presented in . The analysis of variance (ANOVA) was used for statistical significance of the terms in regression equation and the models thus obtained were used to infer the effect of various variables on WL, SG and RR. The higher and lower ranges of independent variables were selected based on literature and initial trials conducted before designing the final experiment using response surface methodology ().
Table 1. Minimum and maximum ranges of independent variables
Table 2. Experimental design (Coded) of independent variables and studied responses for osmotic dehydration of peach (Shan-e-Punjab)
Water Loss and Solute Gain during Osmotic Dehydration
The water loss (WL) and solute gain (SG) were calculated by using the procedure (Ozen et al., Citation2002; Chauhan et al., Citation2011).
Where W0, Wt, S0, and St are initial weight (g) of peach slices, weight (g) of the osmotically dehydrated peach after time in minutes, initial weight of solids content in peach slices (g) and weight of solids of the osmotically dehydrated peach slices after time in minutes, respectively.
Rehydration Ratio
Rehydration measures the injuries to the plant cells/tissues occurred due to pre-treatment and drying temperature and time (Lewicki, Citation1998), which was determined by soaking 20 g of osmotically dehydrated samples in 300 ml of water at 25◦C. The peach slices were rehydrated for 15 h to reach a constant weight followed by excess removal of water using filter paper and weighing of samples. The rehydration ratio was calculated as per earlier described methodology (Mazza, Citation2007).
Textural Analysis
Hardness was measured as force (Newton) as an index of dried peach slices texture by Stable Micro System Texture Analyzer Model (TA-H di England) using settings as Test-TPA, Probe-75 mm Cylindrical, pretest speed-1 mm/s, Test speed-1 mm/s, posttest speed-1 mm, Force-5 kg.
Conventional Air Drying and Sensory Evaluation
Osmo-dried peach slices under optimized conditions using sucrose concentration of 63.20◦Brix, time of 133 minutes and temperature of 41◦C were conventionally air dried (CAD) in cabinet drier at 50◦C. The samples were cooled in a desiccator, weighed and dried to reach the moisture content below 20%. The sensory evaluation of combined dried peach slices (OD+CAD) was done in comparison with CAD sample using a 9.0-point hedonic scale.
Storage Studies
Combined dried (OD+CAD) peach slices were packed in 200-gauge high-density polyethylene (HDPE) and stored under refrigeration (4–7◦C) and ambient temperature (28 ± 5◦C). The stored product was evaluated for bioactive components like pH total phenol content (TPC), antioxidant activity, hue angle and sensory attributes for calculating overall acceptability of stored samples.
Extraction and Isolation
Dried peach slices were ground, and extracted with 80% methanol by refluxing at 50◦C temperature for 2 hours. The samples were filtered and the residue was again refluxed for an hour with 80% methanol and volume made to 100 ml with 80% methanol and collected filtrate was analyzed for antioxidant activity and total phenol content.
Determination of Total Phenolics Content
Total phenolic contents samples were determined using Folin-Ciocalteu reagent as described by (Hatami et al., Citation2014; Singleton.V.L, Citation1965). Samples were put into a test tube and mixed thoroughly with 5 ml Folin-Ciocalteu reagent (pre-diluted 10 times with distilled water). Kept it for 5 min and then added 4 ml of 7.5% sodium carbonate and allowed to react for 2 hours at room temperature. The absorbance was measured at 765 nm using a spectrophotometer. Samples were measured in three replicates. The results were expressed as mg GAE/100 g extract sample.
Determination of Free Radical Scavenging Assay
The free radical scavenging activity of the OD+CAD dried peach extract was evaluated using DPPH (1, 1-Diphenyl-2-picrylhydrazyl) method. 1 g of the sample was mixed with 3 mL of DPPH methanol solution. The reaction mixtures were incubated at room temperature and allowed to react for 30 minutes in the dark. After 30 minutes, the absorbance values were measured at 517 nm and converted into a percentage of antioxidant activity. Butylated hydroxytoluene (BHT) was used as a control. The percentage inhibition of DPPH (%) was calculated as follows:
ODc = Control solution absorbance. DB = Blank solution absorbance. ODS = Sample solution absorbance.
Color Attributes of Peach Candy
Color of peach candy was measured in terms of L*, a* and b* color scales using a Minolta CR-300 Chroma Meter (Konica Minolta, Osaka, Japan) (Nanke et al., Citation1999). Hue angle was measured as given below:
The hue Angle (°) indicates the color spectrum, where 0(°), 90(°), 180(°), and 270(°) correspond to redness, yellowness, greenness and blueness, respectively.
Dissemination of Technology
To popularize and receive the response of the technology from end users, 36 trainees including small scale peach farmers and processors were imparted 1 week training on low cost drying technology for preserving peach. The training was a part of the skill development programme for farmers and food entrepreneurs of the local area. Most of participants were local fruit growers and processors with some being members of well-organized Self-Help Groups that were actively involved in processing and value addition of farm produce. Some of the trainees were already in practice of using combination of osmotic and convective drying for making fruit candies like aonla (Indian gooseberry) candy. The demonstration of peach candy making was aimed at to see the acceptability of technology in terms ease of adoption and sensory attributes and to aware the budding entrepreneurs about the low-cost peach preservation technique. Post training the response from trainees was obtained based on questionnaire covering different aspects of peach preservation technology (Suppl ). To avoid any biasness the response was taken anonymously.
Statistical Analysis
The data was analyzed using ANOVA at p ≤ .05 significance level using SPSS 19.0 statistical software. The results were expressed as mean ± S.D. of three replications.
Results and Discussion
Effect of Osmotic Conditions on Water Loss, Solute Gain and Rehydration Ratio
The response variables for osmotic drying of peach slices for different osmotic conditions as per the experimental design are given in . The obtained results analyzed using ANOVA depicted that, all the response models were statistically significant with no significant lack of fit (). The high R2 values of 0.99, 0.99 and 0.98 for WL, SS and RR, respectively, also validated the model in predicting the change in response variable with change in osmotic drying conditions. Furthermore, the regression equations were analyzed for studying the significant factors influencing the osmotic dehydration of peach slices (). This suggested that the immersion time to be the most significant factor for WL followed by the temperature and osmotic agent concentration. Earlier studies with Chinese ginger and west Indian cherry also suggested that immersion time was the key factor for water loss in osmotic dehydration (An et al., Citation2013; Da Conceição Silva et al., Citation2012), however, further increase in immersion time decreased the WL from these samples (Azoubel and Xidieh Murr, Citation2004). The regression equations for WL also suggested that the interactive effect of sucrose concentration and temperature had a negative effect on WL. The studies with plum also suggested that during its osmotic dehydration the most significant factor for WL was immersion time followed by sucrose concentration (Koocheki and Azarpazhooh, Citation2010). Response surface three-dimensional plots are helpful to study the interactive interactions of independent variables between two responses keeping one at fixed level (Jafari et al., Citation2020; Karabegović et al., Citation2014). The response surface plots for WL indicated that an increase in immersion time resulted in increased WL keeping sucrose percentage at a constant level (). Significance of immersion time in water loss during osmotic dehydration has been reported earlier in carrots (Singh et al., Citation2008) and peach (Yadav et al., Citation2012). Along with immersion time, temperature of the osmotic agent also plays and important role in water loss during osmotic dehydration. High temperature has been reported to increase WL by swelling and plasticizing the membranes of fruit cells (Uddin et al., Citation2004). The positive role of temperature in water loss during osmosis has also been reported in pineapple (Ramallo and Mascheroni, Citation2005), sapota (Gupta et al., Citation2014) and pointed gourd (Sharma and Kumari, Citation2018). Similarly, SG was also significantly influenced by immersion time followed by temperature (). Further, the sugar concentration had least effect on SG, and also the interactive effect of variables was non-significant for this response. Earlier studies conducted on osmotic dehydration of carrots (Singh et al., Citation2010) and Chinese ginger (An et al., Citation2013) reported that the increasing temperature and immersion time made SG grow rapidly. The increase in immersion time results in the swelling of cell membrane, which increases its permeability for osmotic solutions (Lazarides et al., Citation1997), and further the high temperature favors the transfer of osmotic solution in foods. In an earlier study on osmotic dehydration of broccoli stalk slices, temperature (42◦C) and immersion time (4 h) was found significant factors in predicting solute intake of 7% at a sucrose concentration of 56% w/v (Md Salim et al., Citation2016).
Table 3. Analysis of Variance for response variables
Table 4. Regression coefficient of dependent responses
Figure 1. Response surface plots showing effect of independent variables on studied responses a: Water loss, b: Solute gain, c: Rehydration ratio, d: Hardness and e: Overall acceptability.
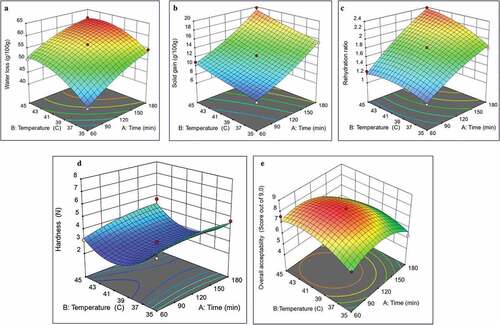
Rehydration of dried food materials involves water absorption and leaching of solutes (Krokida and Philippopoulos, Citation2005; Lewicki, Citation1998). The regression analysis also indicated that as in case of WL and SG, the immersion time was the most significant factor for predicting RR. As the immersion time increased, RR also increased at specific sugar concentrations (). During osmotic dehydration of ginger, immersion time was the most significant factor for rehydration ratio (An et al., Citation2013). The interactive effect of sucrose concentration and temperature had a positive effect on RR, while the quadratic effect of all the variables was negative as indicated by more time taken for rehydration of peach slices. This might be due the high temperature, which resulted in more solute gain during the process of osmotic drying of peach slices and causing blockage of internal pores. Earlier study on osmotic dehydration of anola slices reported that rehydration ratio decreased with an increase in temperature and sucrose concentration due to blockage of anola slices structural pores (Alam et al., Citation2010). High temperature resulted in increased solute gain in Chinese ginger, which were trapped inside and blocked the internal cellular pores of ginger. This led to higher rehydration ratio as it makes the water and solute to flow in and out difficult during the rehydration process (An et al., Citation2013).
Effect of Osmotic Conditions on Textural and Sensory Quality of Peach
Textural quality of peach slices measured in terms of hardness and overall acceptability of sensory attributes (appearance, color, flavor and texture) revealed that variance for both the responses was statistically significant, while lack of fit was found to be non-significant (). The regression coefficients, R2 and predicted R2 values for hardness and overall acceptability of peach slices () also suggested that significant change in response variable with change in osmotic drying conditions. Thus, validating both the studied models for hardness and overall acceptability by ANOVA and regression analysis. The immersion time influenced the both hardness and overall acceptability of the peach slices. As the immersion time increased, it decreased the hardness (N) of the peach slices (). Higher immersion time resulted in more solute gain and firm texture as a result of solute deposition inside the structural tissue of peach slices. The response surface plot () for hardness confirmed that the increase in immersion time resulted in decrease in hardness as a result of more solute gain in peach slices. Previous study with cherry laurel fruit also suggested that increase in immersion time resulted in more solute gain in the sample which fills the internal fruit pores, thus resulted in a firm texture with less deformed tissue (Giannakourou et al., Citation2020). Furthermore, an increase in immersion time solubilize the cell wall components and resulted in tissue softening of the strawberry fruit (Prinzivalli et al., Citation2006). The immersion time had a significantly negative impact on the sensory attributes of the peach slices (). An increase in immersion time resulted in an increased solute gain in peach slices, which might be the reason for low sensory scores for osmo-dried peach slices with higher immersion time. This might be due to higher solute gain, which made the slices sweeter and a balanced acid-sweet ratio required for an acceptable taste of the fruit product. The overall acceptability scores were directly proportional to the immersion time, response surface plot () for overall acceptability score between immersion time and temperature while keeping the sucrose at a constant level depicted the decrease in scores with an increase in immersion time. In a previous study on osmotic dehydration of anola slices immersion time and temperature were the significant factors for sensory attributes. Higher levels of process variable (immersion time and temperature) decreased the overall acceptability of anola slices (Alam et al., Citation2010).
Optimization: The criteria for optimization were maximum water loss, rehydration ratio, and overall acceptability with minimum solute gain along with optimum hardness (). The sucrose level of 63.20°Brix for 133 minutes at 41°C were found to be optimum for obtaining WL (58.91 g/100 g of fresh weight), minimum SG (13.88 g/100 of fresh weight), maximum RR (2.07), optimal hardness (2.95) and overall acceptability (8.03). The coefficient of variance (CV) for all studied response models was (<below 4.0) confirmed the close agreement between the actual values with the predicted ones suggesting the suitability of these optimized conditions for drying of peach slices.
Table 5. Optimization criteria for different factors, response and solutions
Conventional air drying (CD) and sensory evaluation dried peach slices: Osmotic-dried peach slices subjected to conventional air drying (CAD) at 50◦C showed significant reduction in drying time (). Thus, previously osmotic dried slices (drying time: 2.21 h) with moisture content (wet basis) of 31.46 g/100 g were in semidried condition and reached final moisture content of 18.1 g/100 g after 7 h with CAD. On the other hand, the control sample (CAD) with initial moisture content of 90.26 g/100 g took 14 h to reach up to 19.34 g/100 g on a wet basis. These results showed a reduction of around 34.21% for the total drying time for previously osmotic dried peach slices compared to conventional air-dried sample along with the retention of sensory attributes. Reduction in drying time resulted in energy conservation (Tola and Ramaswamy, Citation2010) and less drying time results in better retention of color, flavor and taste, as well as nutritional and bioactive components of plant tissues (Jalali et al., Citation2008; Torringa et al., Citation2001; Zhao et al., Citation2014).
Figure 2. Moisture content (g water/g dried mass) of peach slices conventionally dried at 60°C in cabinet drier. A: Previously osmo-dried using optimized conditions (sucrose concentration: 61.86 °Brix, time: 133 minutes and temperature: 41°C), B: Peach slices dried only in cabinet driver.
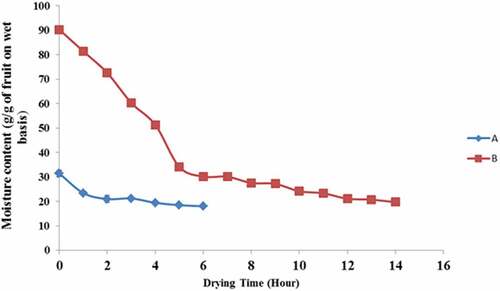
Dried peach slices analyzed for color, flavor, taste, texture, and overall acceptability using 9-point hedonic scale showed significant (p < .05) variation among combined dried sample and control (conventional dried only) for all the sensory quality attributes (). Combined drying methods have been reported beneficial for better retention of quality characteristics; osmotic dehydration as a pretreatment in dehydration of strawberry significantly reduced the drying time and improved mechanical and structural properties of the fruit (Prosapio and Norton, Citation2017). Combining osmotic and conventional air drying had a better overall acceptability score than the control. Osmotic dehydration helps in reducing the detrimental effect of conventional drying in fruit and vegetables without affecting the sensory and nutritional quality (Osorio et al., Citation2007. Beneficial role of osmotic drying as a pretreatment in dehydration of fruits has been reported in earlier studies; combined drying process (OD+ Pulse Electric field (PFE)) followed by air-drying had lower processing time (33%) with better retention of color in the drying of goji berry (Dermesonlouoglou et al., Citation2018); carrots slices dried by combining osmotic and air drying received significantly higher scores compared to the conventional air-dried carrots (Ghosh et al., Citation2006).
Bioactivity, Color and Sensory Attributes of OD+CAD Dehydrated Peach during Storage
During storage study of optimized dried peach slices, phytochemicals like total phenol content (mg GAE/100 g) and antioxidant activity (% DPPH inhibition) on dry weight basis had a significant reduction for both storage temperatures ( and 4b). Both the preservation methodologies i.e., OD and OD+ CAD dried peach candies showed no significant difference in acidity and pH (Supplementary Table 2). Moreover, the reduction of TPC and antioxidant activity at ambient temperature was higher as compared to refrigerated temperature. Oxidation of phenolic components during storage lead to decrease in TPC and antioxidant activity of stored slices as high temperature favors the oxidation reactions; this explains the higher reduction of TPC and antioxidant activity at ambient temperature. Earlier studies with cherry laurel fruit observed a decrease in total phenolics, total flavonoids, and antioxidant activity throughout the storage period (Ozturk et al., Citation2017). However, even at room temperature, dried peach slices were managed to retain more than, 50% of total phenol content () and 45% antioxidant activity () at the end of 6 months of storage. Storage temperature had a detrimental effect on color attributes of peach candies evident from the decrease in hue angle as the corresponding to the storage period (). Previous study on anthocyanin stability of strawberry nectar observed a color and phytochemical degradation during storage (Aamer et al., Citation2021). Concerning sensory attributes, stored peach slices were evaluated for color, flavor, taste, texture, flavor and their average value was taken as overall acceptability score calculated at monthly intervals and scores were in a well acceptable range (above 6.0) for the entire storage period (). Earlier study also showed storage stability of osmo-dried bilimbi (Aparna et al., Citation2018) with overall acceptability score of (8.17) after four months of storage. Evaluation of sensory attributes of fruit bar (papaya: banana) in 50:50 ratio was found in higher organoleptic scores and consumer acceptability (Vagadia et al., Citation2016). From the storage study, it was concluded that the dried peach slices had a shelf life of 6 months at both the studied temperatures. As the final product was dried peach slice or peach candy, and fruit candies are intermediate mositure foods with longer shelf life. Moreover, longer shelf life of fruit candies or osmotic dehdydrated fruits were previously reported; osmo-dehydrated carambola of had a shelf life of 10, 8 and 6 months at different temperature range at 5°C, ambient (30 ± 2°C) and 37°C, respectively (Roopa et al., Citation2014) apple leather, a dehydrated snack was stable for 7 months at 20°C (Quintero Ruiz et al., Citation2012).
Response of Marginal Peach Farmers/ Small Scale Food Processors to Low-cost Peach Preservation Technology
Based on response to questionnaire from the farmers/small scale food processors imparted training on low-cost peach preservation technology, it was observed that none of the entrepreneurs was aware of this technique (). More than 85% of the entrepreneurs strongly rated ease in adoption of this technique. It was observed that more than 90% of the entrepreneurs rated this technique as economical, effective in enhancing the shelf life with good organoleptic qualities. Moreover, use of osmotic dehydration as a pretreatment prior to CAD, reduced 50% drying time and helped in energy conservation. Therefore, this technique was rated as a best option to reduce the post-harvest losses in highly perishable peach fruit. The current study gives a complete package of developing a low-cost peach preservation technique and its application at the farm level. Most of the entrepreneurs trained are the members of well-organized Self-help groups (SHG). The SHG in Punjab are involved in multi entrepreneurial activities and have good inclination toward adoption of new value additional techniques (Singh, Citation2012). Thus, based on response received, this low-cost technique will be highly adopted and helpful for small-scale processors.
Table 6. Response of marginal peach farmers/ small scale food processors to various attributes of the low-cost peach preservation technique
Conclusions: Osmotic dehydration combined with conventional drying is an effective low cost and energy conserving technology for the preservation of peach fruit. Optimum conditions were maximum possible WL (58.91 g/100 g of fresh weight), minimum SG (13.88 g/100 of fresh weight), and maximum RR (2.07), optimum hardness (2.95) and maximum overall acceptability (8.03) by using sucrose concentration of 63.20°Brix, time of 133 minutes and temperature of 41°C. Combined drying (OD+CAD) significantly reduced the drying time by 34.21% as compared to the control sample (CAD). From a sensory point of view combined dried product had better color, flavor, texture and taste and thus accounted for a higher acceptability score than the control sample. Therefore, it was concluded that OD followed by CAD is an economical drying method to reduce drying time and to attain a better-quality product having a shelf life of 6 months. Concerning technology adoption, peach farmers and small-scale entrepreneurs highly appreciated this technology as it is economical, easy to adopt and was anticipated to decrease post-harvest losses in peach.
Disclosure Statement
No potential conflict of interest was reported by the author(s).
References
- Aamer, R.A., W.A. Amin, and R.S. Attia. 2021. Enhancement of color stability in strawberry nectar during storage. Ann. Agric. Sci 66(2):121–130. doi: 10.1016/j.aoas.2021.08.003.
- Abud-Archila, M., M.A.R.-C. D.G.Vázquez-Mandujano, M. Moscosa-Santillán, M. Moscosa-Santillán, L. Dendooven, L. Dendooven, L. Dendooven, and L. Dendooven. 2008. Optimization of osmotic dehydration of yam bean (Pachyrhizus erosus) using an orthogonal experimental design. J. Food. Eng 84(3):413–419. doi: 10.1016/j.jfoodeng.2007.06.003.
- Ahmed, I., I.M. Qazi, and S. Jamal. 2016. Developments in osmotic dehydration technique for the preservation of fruits and vegetables. Innov. Food. Sci. Emerg.Technol. 34:29–43. doi: 10.1016/j.ifset.2016.01.003.
- Alam, M.S., S. Amarjit, and B.K. Sawhney. 2010. Response surface optimization of osmotic dehydration process for aonla slices. J. Food Sci. Technol 47(1):47–54. doi: 10.1007/s13197-010-0014-4.
- An, K., S. Ding, HongyanTao, D. Zhao, X., Z. Wang and X. Hu. 2013. Response surface optimisation of osmotic dehydration of Chinese ginger (Zingiber officinale Roscoe) slices. Int. J. Food. Sci. Technol 48:28–34.
- An, K., S. Ding, H. Tao, D. Zhao, X. Wang, Z. Wang, and X. Hu. 2013. Response surface optimisation of osmotic dehydration of Chinese ginger (Zingiber officinale Roscoe) slices. Int. J. Food. Sci. Technol 48(1):28–34. doi: 10.1111/j.1365-2621.2012.03153.x.
- AOAC, 1991. Official methods of analysis of the association of official analytical chemists. 15th ed, Analytica Chimica Acta. Washington, DC: Analytica Chimica Acta.
- Aparna, G.S., P.R.G. Lekshmi, C. Mini, and T.T. Chandran. 2018. Studies on sensory attributes and microbial analysis of stored osmo dehydrated bilimbi (Averroha bilimbi). Asian. J. Dairy Food. Res 37(2):154–157. doi: 10.18805/ajdfr.DR-1314.
- Azoubel, P.M., and F.E. Xidieh Murr. 2004. Mass transfer kinetics of osmotic dehydration of cherry tomato. J. Food Eng 61(3):291–295. doi: 10.1016/S0260-8774(03)00132-8.
- Berk, Z. 2018. Food Processing Engineering and Technology. 3rd ed. Academic Press, San Diego, CA 92101-4495, USA.
- Chauhan, O.P., S. Asha, S. Asha, P.S. Raju, and A.S. Bawa. 2011. Effects of osmotic agents on colour, textural, structural, thermal, and sensory properties of apple slices. Int. J. Food Prop 14(5):1037–1048. doi: 10.1080/10942910903580884.
- Da Conceição Silva, M.A., Z.E. da Silva, V.C. Mariani, and S. Darche. 2012. Mass transfer during the osmotic dehydration of West Indian cherry. LWT- Food Sci. Technol 45(2):246–252. doi: 10.1016/j.lwt.2011.07.032.
- Da Costa Ribeiro, A.S., E. Aguiar-Oliveira, and R.R. Maldonado. 2016. Optimization of osmotic dehydration of pear followed by conventional drying and their sensory quality. LWT - Food Sci. Technol. 72:407–415. doi: 10.1016/j.lwt.2016.04.062.
- Dermesonlouoglou, E., A. Chalkia, G. Dimopoulos, and P. Taoukis. 2018. Combined effect of pulsed electric field and osmotic dehydration pre-treatments on mass transfer and quality of air dried goji berry. Innovative Food Science & Emerging Technologies. 49:106–115. doi: 10.1016/j.ifset.2018.08.003.
- Derossi, A., C. Severini, A. Del Mastro, and T. De Pilli. 2015. Study and optimization of osmotic dehydration of cherry tomatoes in complex solution by response surface methodology and desirability approach. LWT – Food. Sci. Technol 60(2):641–648. doi: 10.1016/j.lwt.2014.10.056.
- Fernandes, F.A.N., S. Rodrigues, O.C.P. Gaspareto, and E.L. Oliveira. 2006. Optimization of osmotic dehydration of bananas followed by air-drying. J. Food Eng 77(1):188–193. doi: 10.1016/j.jfoodeng.2005.05.058.
- Ghosh, P.K., Y.C. Agrawal, D.S. Jayas, and B. Kumbhar. 2006. Process development for osmo-hot air drying of carrot. J. Food Sci. Technol 43:65–68.
- Giannakourou, M.C., A.Z. Lazou, and E.K. Dermesonlouoglou. 2020. Optimization of osmotic dehydration of tomatoes in solutions of non-conventional sweeteners by response surface methodology and desirability approach. Foods 9(10):1393. doi: 10.3390/foods9101393.
- Gupta, S.V., B. Patil, V.R. Wankhade, P.M. Nimkar, and P.A. Borkar. 2014. Modelling and optimization of osmotic dehydration of sapota using response surface methodology. J. Food Agric. Environ 12:135–140.
- Hatami, T., S.A. Emami, S.S. Miraghaee, and M. Mojarrab. 2014. Total Phenolic Contents and Antioxidant Activities of Different Extracts and Fractions from the Aerial Parts of Artemisia biennis Willd. Iranian Journal of Pharmaceutical Research: IJPR 13(2):551–559.
- Jafari, F., K. Movagharnejad, and E. Sadeghi. 2020. Infrared drying effects on the quality of eggplant slices and process optimization using response surface methodology. Food. Chem. 333:127423. doi: 10.1016/j.foodchem.2020.127423.
- Jalali, V.R.R., N. Narain, and G.F. Da Silva. 2008. Effect of osmotic predehydration on drying characteristics of banana fruits. Food Sci. Technol 28:269–273.
- Karabegović, I.T., S.S. Stojičević, D.T. Veličković, N.Č. Nikolić, and M.L. Lazić. 2014. Optimization of microwave-assisted extraction of cherry laurel fruit. Sep. Sci. Technol 49(3):416–423. doi: 10.1080/01496395.2013.838967.
- Koocheki, A., and E. Azarpazhooh. 2010. Evaluation of mass exchange during osmotic dehydration of plum using response surface methodology. Int. J. Food. Prop 13(1):155–166. doi: 10.1080/10942910802256172.
- Koprivica, G.B., L.L. Pezo, B.L. Ćurčić, L.B. Lević, and D.Z. Šuput. 2014. Optimization of osmotic dehydration of apples in sugar beet molasses. J. Food. Process. Preserv 38(4):1705–1715. doi: 10.1111/jfpp.12133.
- Kowalska, H., A. Marzec, E. Domian, E. Masiarz, A. Ciurzyńska, S. Galus, A. Małkiewicz, A. Lenart, J. Kowalska, H. Physi Kowalska, et al. 2020. Physical and sensory properties of Japanese quince chips obtained by osmotic dehydration in fruit juice concentrates and hybrid drying. Molecules. 25(23):5504. doi:10.3390/molecules25235504.
- Krokida, M.K., and C. Philippopoulos. 2005. Rehydration of dehydrated foods. Dry. Technol 23(4):799–830. doi: 10.1081/DRT-200054201.
- Lazarides, H.N., V. Gekas, and N. Mavroudis. 1997. Apparent mass diffusivities in fruit and vegetable tissues undergoing osmotic processing. J. Food. Eng 3(3):315–324. doi: 10.1016/S0260-8774(96)00084-2.
- Lewicki, P.P. 1998. Some remarks on rehydration of dried foods. J. Food. Eng 36(1):81–87. doi: 10.1016/S0260-8774(98)00022-3.
- Luchese, C.L., P.D. Gurak, and L.D.F. Marczak. 2015. Osmotic dehydration of physalis (Physalis peruviana L.): Evaluation of water loss and sucrose incorporation and the quantification of carotenoids. LWT – Food. Sci. Technol 63(2):1128–1136. doi: 10.1016/j.lwt.2015.04.060.
- Madamba, P.S., and R.I. Lopez. 2002. OPTIMIZATION OF THE OSMOTIC DEHYDRATION OF Mango (Mangifera indica L.) SLICES. Drying Technology 20(6):1227–1242. doi: 10.1081/DRT-120004049.
- Mazza, G. 2007. Dehydration of carrots. Int. J. Food Sci. Technol 18(1):113–123. doi: 10.1111/j.1365-2621.1983.tb00249.x.
- Md Salim, N.S., Y. Gariepy, and V. Raghavan. 2016. Effects of operating factors on osmotic dehydration of broccoli stalk slices. Cogent. Food. Agric 2:1134025.
- Nanke, K.E., G.S. J, and D.G. Olson. 1999. Color characteristics of irradiated aerobically packaged pork, beef, and Turkey. J. Food. Sci 64(2):272–278. doi: 10.1111/j.1365-2621.1999.tb15881.x.
- Osorio, C., M.S. Franco, M.P. Castaño, M.L. González-Miret, F.J. Heredia, and A.L. Morales. 2007. Colour and flavour changes during osmotic dehydration of fruits. Innov. Food. Sci. Emerg.Technol 8(3):353–359. doi: 10.1016/j.ifset.2007.03.009.
- Ozdemir, M., B.F. Ozen, L.L. Dock, and J.D. Floros. 2008. Optimization of osmotic dehydration of diced green peppers by response surface methodology. LWT – Food. Sci. Technol 41(10):2044–2050. doi: 10.1016/j.lwt.2008.01.010.
- Ozen, B.F., L.L. Dock, M. Ozdemir, and J.D. Floros. 2002. Processing factors affecting the osmotic dehydration of diced green peppers. Int. J. Food Sci. Technol 37(5):497–502. doi: 10.1046/j.1365-2621.2002.00606.x.
- Ozturk, B., S.M. Celik, M. Karakaya, O. Karakaya, A. Islam, and T. Yarilgac. 2017. Storage temperature affects phenolic content, antioxidant activity and fruit quality parameters of cherry laurel (Prunus laurocerasus L.). J. Food Process. Preserv 41(1):12774. doi: 10.1111/jfpp.12774.
- Park, K., A. Bin, F. Brod, and T. Park. 2002. Osmotic dehydration kinetics of pear D’anjou (Pyrus communis L.). J. Food. Eng 52(3):293–298. doi: 10.1016/S0260-8774(01)00118-2.
- Prinzivalli, C., A. Brambilla, D. Maffi, R. Lo Scalzo, and D. Torreggiani. 2006. Effect of osmosis time on structure, texture and pectic composition of strawberry tissue. Eur. Food Res. Technol 224(1):119–127. doi: 10.1007/s00217-006-0298-9.
- Prosapio, V., and I. Norton. 2017. Influence of osmotic dehydration pre-treatment on oven drying and freeze drying performance. LWT- Food. Sci. Technol. 80:401–408. doi: 10.1016/j.lwt.2017.03.012.
- Quintero Ruiz, N.A., S.M. Demarchi, J.F. Massolo, L.M. Rodoni, and S.A. Giner. 2012. Evaluation of quality during storage of apple leather. LWT- Food. Sci Technol 47(2):485–492. doi: 10.1016/j.lwt.2012.02.012.
- Ramallo, L.A., and R.H. Mascheroni. 2005. Rate of water loss and sugar uptake during the osmotic dehydration of pineapple. Brazilian Archiv. Bio. Technol 48(5):761–770. doi: 10.1590/S1516-89132005000600012.
- Rastogi, N.K., K.S.M.S. Raghavarao, and K. Niranjan. 2014. Recent developments in osmotic dehydration. In: D.W. Sun (ed.). Emerging technologies for food processing. 2nd ed. Elsevier. San Diego, USA. 181–212.
- Roopa, N., O.P. Chauhan, P.S. Raju, D.K. Das Gupta, R.K.R. Singh, and A.S. Bawa. 2014. Process optimization for osmo-dehydrated carambola (Averrhoa carambola L) slices and its storage studies. J. Food. Sci.Technol 51(10):2472–2480. doi: 10.1007/s13197-012-0756-2.
- Samborska, K., L. Eliasson, A. Marzec, J. Kowalska, D. Piotrowski, A. Lenart, and H. Kowalska. 2019. The effect of adding berry fruit juice concentrates and by-product extract to sugar solution on osmotic dehydration and sensory properties of apples. J. Food. Sci.Technol. 56(4):1927. doi:10.1007/s13197-019-03658-0. 1938.
- Sharma, P., and N. Kumari. 2018. Optimization of Osmotic dehydration process of pointed gourd by response surface methodology. J. Pharmaco. Phytochem 7:2491–2495.
- Singh, S. 2012. An Evaluation Study on Women Self-Help Groups of Punjab. Master Thesis Punjab Agricultural University. Ludhiana, India. Pp 1–98.
- Singh, B., P. Panesar, and V. Nanda. 2008. Optimization of osmotic dehydration process of carrot cubes in sucrose solution. J. Food Process Eng 31(1):1–20. 181–212. doi: 10.1111/j.1745-4530.2007.00173.x.
- Singh, B., P.S. Panesar, V. Nanda, and J.F. Kennedy. 2010. Optimisation of osmotic dehydration process of carrot cubes in mixtures of sucrose and sodium chloride solutions. Food Chem 123(3):590–600. doi: 10.1016/j.foodchem.2010.04.075.
- Singleton.V.L, J.A.R. 1965. Colorimetry of total phenolics with phosphomolybdic-phosphotungstic acid reagents. Am. J. Enol. Vitic 16:144–158.
- Siqueira, L.P., L.A. de Sarubbo, N.K.S. Shinohara, M.S. Valencia, N.M.S. Cortez, and S.A.C. Andrade. 2019. Optimization of the osmotic dehydration of ginger. Ciência. Rural 49(10):1–8. doi: 10.1590/0103-8478cr20170430.
- Tola, Y., and H. Ramaswamy. 2010. Going beyond conventional osmotic dehydration for quality advantage and energy savings. Ejast 1(1):1–15.
- Torringa, E., E. Esveld, I. Scheewe, R. van den Berg, and P. Bartels. 2001. Osmotic dehydration as a pre-treatment before combined microwave-hot-air drying of mushrooms. J. Food. Eng 49(2–3):185–191. doi: 10.1016/S0260-8774(00)00212-0.
- Uddin, M.B., P. Ainsworth, and S. İbanoğlu. 2004. Evaluation of mass exchange during osmotic dehydration of carrots using response surface methodology. J. Food. Eng 65(4):473–477. doi: 10.1016/j.jfoodeng.2004.02.007.
- Vagadia, P., A. Senapati, R. Tank, J. Mayani, and B. Koyani. 2016. Evaluation of Physico-chemical and organoleptic quality of Papaya Cv. Taiwan and Banana Cv. Grand naine based mixed fruit bar during storage. Int. J. Environ. Agric. Biotech 9(4):541–545. doi: 10.5958/2230-732X.2016.00071.1.
- Vieira, G., L. Rodrigues, and M. Hubinger. 2012. Optimisation of osmotic dehydration process of guava by response surface methodology and desirability function. Int. J. Food Sci. Technol 47(1):132–140. doi: 10.1111/j.1365-2621.2011.02818.x.
- Yadav, A.K., and S.V. Singh. 2014. Osmotic dehydration of fruits and vegetables: A review. J. Food Sci. Technol 51(9):1654–1673. doi: 10.1007/s13197-012-0659-2.
- Yadav, B.S., R.B. Yadav, and M. Jatain. 2012. Optimization of osmotic dehydration conditions of peach slices in sucrose solution using response surface methodology. J. Food Sci. Technol 49(5):547–555. doi: 10.1007/s13197-011-0298-z.
- Zapata Montoya, J.E., A. Joham Maria, and G. Gelemy. 2011. Optimization of osmotic dehydration of pineapple (Ananas comosus L.) using the response surface methodology. Agron. Colomb 29:441–448.
- Zhao, J.H., R. Hu, H.W. Xiao, Y. Yang, F. Liu, Z.L. Gan, and Y.Y. Ni. 2014. Osmotic dehydration pretreatment for improving the quality attributes of frozen Mango: Effects of different osmotic solutes and concentrations on the samples. Int. J. Food Sci. Technol 49(4):960–968. doi: 10.1111/ijfs.12388.